-
PDF
- Split View
-
Views
-
Cite
Cite
Anca Remes, Rawa Arif, Maximilian Franz, Andreas Jungmann, Marcin Zaradzki, Thomas Puehler, Markus B Heckmann, Norbert Frey, Matthias Karck, Klaus Kallenbach, Markus Hecker, Oliver J Müller, Andreas H Wagner, AAV-mediated AP-1 decoy oligonucleotide expression inhibits aortic elastolysis in a mouse model of Marfan syndrome, Cardiovascular Research, Volume 117, Issue 12, 1 November 2021, Pages 2459–2473, https://doi.org/10.1093/cvr/cvab012
- Share Icon Share
Abstract
Marfan syndrome is one of the most common inherited disorders of connective tissue caused by fibrillin-1 mutations, characterized by enhanced transcription factor AP-1 DNA binding activity and subsequently abnormally increased expression and activity of matrix-metalloproteinases (MMPs). We aimed to establish a novel adeno-associated virus (AAV)-based strategy for long-term expression of an AP-1 neutralizing RNA hairpin (hp) decoy oligonucleotide (dON) in the aorta to prevent aortic elastolysis in a murine model of Marfan syndrome.
Using fibrillin-1 hypomorphic mice (mgR/mgR), aortic grafts from young (9 weeks old) donor mgR/mgR mice were transduced ex vivo with AAV vectors and implanted as infrarenal aortic interposition grafts in mgR/mgR mice. Grafts were explanted after 30 days. For in vitro studies, isolated primary aortic smooth muscle cells (SMCs) from mgR/mgR mice were used. Elastica-van-Giesson staining visualized elastolysis, reactive oxygen species (ROS) production was assessed using dihydroethidine staining. RNA F.I.S.H. verified AP-1 hp dON generation in the ex vivo transduced aortic tissue. MMP expression and activity were assessed by western blotting and immunoprecipitation combined with zymography.
Transduction resulted in stable therapeutic dON expression in endothelial and SMCs. MMP expression and activity, ROS formation as well as expression of monocyte chemoattractant protein-1 were significantly reduced. Monocyte graft infiltration declined and the integrity of the elastin architecture was maintained. RNAseq analysis confirmed the beneficial effect of AP-1 neutralization on the pro-inflammatory environment in SMCs.
This novel approach protects from deterioration of aortic stability by sustained delivery of nucleic acids-based therapeutics and further elucidated how to interfere with the mechanism of elastolysis.
1. Introduction
Marfan syndrome is a rare genetic disorder characterized by loss-of-function mutations in the fibrillin-1 gene,1 affecting the skeletal, ocular and most importantly, the cardiovascular system represented by life-threatening rupture of aortic aneurysms.2 The main events that accompany the formation of aortic aneurysms are the degradation of elastic fibres, smooth muscle cell (SMC) apoptosis, immune cell infiltration,3 and excessive secretion of matrix-metalloproteinases (MMPs).4,5
The fibrillin-1 gene (FBN1) has been proven to control transforming growth factor-β (TGF-β) activation and signalling. TGF-β plasma levels were demonstrated to be highly increased in both Marfan syndrome patients and the fibrillin-1 hypomorphic mgR/mgR murine model of Marfan syndrome as compared to control animals.6 Consequently, the administration of a TGF-β neutralizing antibody could improve the architecture of elastin fibres in mgR/mgR mice.7
TGF-β promotes the activation of MMPs, which in turn correlates with the degree of elastic fibre fragmentation in aortic tissue isolated from mgR/mgR mice8 and Marfan patients.9 MMPs degrade a high variety of extracellular matrix components, such as collagen, elastin, fibronectin, and gelatine, consequently lowering the stability of the aortic wall.10 Moreover, MMP2 and MMP9 have been shown to decrease SMCs contractility, increase SMCs apoptosis and promote elastin fragmentation in the context of thoracic aneurysm in mgR/mgR mice.8 Previous studies have validated the beneficial effect of MMP inhibition on aortic aneurysm dissection through doxycycline, a non-specific inhibitor of these enzymes.11
The induction of MMP expression by TGF-β has been demonstrated to be regulated by the activator protein 1 (AP-1) transcription factor family.12 Also, AP-1 proteins mediate expression of pro-inflammatory cytokines such as IL-1β, IL-6, or IL-10, which were shown to be increased in the plasma of Marfan patients.3 Collectively, these processes promote elastin degradation and subsequent weakening of the aortic wall.
The decoy oligodeoxynucleotide (dODN) technology is an efficient method to reduce abnormal gene expression associated with disease development.13 DNA AP-1 dODNs were previously used in a rat model of transplant vasculopathy.14 Additionally, we previously could show that single short-term incubation with AP-1 dODNs was sufficient to inhibit MMP expression and activity, and significantly improved elastin structure in aortic grafts of mgR/mgR mice in the long run.15 However, clinical translation might be hampered by the stability and duration of action of dODNs. To allow a more sustained effect, this study aimed to develop an approach for expression of a self-hybridizing RNA AP-1 hairpin (hp) decoy oligonucleotide (dON) by using an adeno-associated virus (AAV)-mediated delivery into the aortic tissue of Marfan mice. Therefore, aortic grafts from donor mgR/mgR mice were transduced ex vivo with AAV9SLR vectors, an engineered AAV variant previously reported to efficiently transduce endothelial cells and SMCs16 and implanted as infrarenal aortic interposition grafts in mgR/mgR recipient mice. Because of the non-pathogenic nature and low immunogenicity of AAVs,17 this method could be an attractive novel approach towards gene therapy of Marfan syndrome.
2. Methods
2.1 Mouse model
All animal experiments were performed with the permission of the local animal welfare committee (Regional Council Karlsruhe, Germany, permission number G-99/14 and G52/17) and conform to the guidelines from Directive 2010/63/EU of the European Parliament on the protection of animals used for scientific purposes or the current NIH guidelines. Animals were kept in the Interfaculty Biomedical Facility of Heidelberg University under standard conditions with 12-h light and 12-h dark cycle. Water and chow were offered ad libitum. Six 9-week-old female Marfan mgR/mgR mice were used for our experiments in each experimental group. Aortae were treated with either AAV9SLR expressing AP-1 consensus or mutant control hp dONs. After euthanasia using carbon dioxide (CO2) inhalation, the descending aorta was harvested from donor mgR/mgR mice and incubated for 30 min in NaCl (B. Braun, Melsungen, Germany) as control, or 50 µL AAV9SLR solution (1012 vp/mL), respectively. During incubation age- and gender-matched mgR/mgR recipient mice were anaesthetized by inhalation of 5% isoflurane and intraperitoneal injection of Temgesic® (0.3 mg/mL Buprenorphine; 2 mg/100g body weight). After laparotomy, the infrarenal abdominal vessels were carefully separated, vascular clamps were applied and the aorta was dissected between the clamps. The transduced grafts were then transplanted as end-to-end infrarenal interposition grafts into the mice as described.15 Wild type mice served as controls. Euthanasia was carried out with CO2 and grafts were explanted 4 weeks after surgery for analysis. Age-matched wild type littermates served as controls.
Besides, the AAV9SLR solution was directly applied to the lumen of the aorta of the donor animal before the graft was excised and transplanted infrarenal into the recipient animal. Therefore, after median laparotomy and thoracotomy, the entire aorta was prepared from the part of the ascending aorta to the division of the arteria iliaca communis. After an incision of the liver, the lumen of the aorta was flushed with 5 ml sterofundine (B. Braun Melsungen, Germany) by injection into the left ventricle. After suprarenal cross-clamping of the aorta descendens, the AAV solution was injected into the aorta just below the aortic arch by using a 23-gauge microcannula. After injection, the puncture site was also clamped. After 30 min incubation time, the aorta was excised and used as a free graft for aortic transplantation.
2.2 Isolation, culture and treatment of primary SMCs
Primary aortic SMCs were isolated from aortae of mgR/mgR mice using the explant method, as previously described.15 Cells were cultured in complete DMEM medium supplemented with 15% FBS, 50 U/mL penicillin and 50 μg/mL streptomycin (Thermo-Fischer Scientific, Darmstadt, Germany). SMCs were used up to passage 5 in all experiments, to prevent the phenotypic changes that emerge upon long-term culture. SMCs were plated either on plastic cell culture dishes or on gelatin-coated glass coverslips for imaging experiments. AP-1 transcription factors activation was induced by treatment with IL1-β 100 nmol/L (R&D Systems, Wiesbaden, Germany) in serum-free medium for 8 h for gene expression evaluation, or 24 h for protein analysis.
Human primary SMCs were isolated as previously described with slight modifications.18 Aortic tissue was collected from a Marfan patient and a healthy control subject undergoing surgical treatment (University Medical Center Schleswig Holstein, Clinic for Heart Surgery) following written consent under ethics vote D495/19 by the Ethics Committee of the University Medical Centre Schleswig–Holstein, Kiel. All samples were collected according to a protocol in compliance with the Declaration of Helsinki. The vascular tissue was quickly rinsed with PBS under sterile conditions and media was cut into small pieces (1–2 mm2). Afterward, tissue was incubated in a solution containing 0.1% collagenase I (Thermo Fischer Scientific) for 4 h at 37°C. Next, tissue was filtered, centrifuged and cells were resuspended into complete DMEM medium containing 10% FCS (Thermo Fischer Scientific). Cells were passaged after reaching 90% confluency.
Treatment with TGF-β neutralizing antibody (R&D Systems, MAB1835) was conducted at a concentration 0.25 µg/mL under serum-free conditions.
2.3 Monocyte transmigration assay
Human umbilical vein endothelial cells (HUVECs) were isolated from freshly obtained umbilical cords provided by local hospitals with the Ethics Committee permission (S-383/2013) and cultured as previously described.19 The study was in accordance with the Declaration of Helsinki and all patients written informed consents have been obtained. Cells up to passage 3 were used for all experiments. THP1 cells (ATCC, Germany) were maintained as described previously.19 Transmigration assay was performed according to the manufacturer’s instruction (CytoSelect Leukocyte Transmigration Assay Kit, Biocat, Heidelberg). In brief, HUVECs were seeded on each transwell insert (100 000 cells/well) and cultured for 48 h to reach a monolayer. Afterward, IL1-β 30 nmol/L was added to the medium and cells were further incubated for 24 h. MMP9 inhibitor (Santa Cruz, CAS 1177749-58-4) was added to a final concentration of 20 ng/mL. Next, LeukoTracker (provided in the kit)-stained THP cells were added to endothelial cells and cultured for a further 24 h to allow leukocyte transmigration. Media was aspirated, non-migratory THP cells were removed and the lower side of the insert was lysed together with the medium residing in the lower side of the well. Fluorescence was measured (Ex/Em: 480 nm/520 nm) using Cytation5 Imaging Reader (Biotek, Berlin, Germany).
2.4 Decoy technology
Sequences of AP-1 hp dODNs (Biomers, Ulm, Germany) were designed to form a stem-loop structure and to contain the consensus binding site of the transcription factor family as follows: hpAP-1 cons dODN 5′-CTGCGGTGCTGA CTCAGCACGAAACGTGCTGAGTCAG CACCGCAG-3′. hpAP-1 mut dODN 3′-CTGCGGTGC TTACTTAGCACGAAACGTGCTAAGTAAGCACCGCAG-5′ represents the non-functional mutated control dODN (mutations are set in bold and italics). Hp dODNs were solubilized in sterile TEN buffer to a concentration of 500 µmol/L and were hybridized by incubation at 95°C for 5 min followed by gradual cooling, to induce complementary base pairing. The hybridization efficiency was subsequently proven by 2.5% agarose gel electrophoresis.
2.5 Generation and production of AAV vectors
DNA sequences encoding AP-1 cons hp dON and AP-1 mut hp dON were synthetized (GeneArt, Thermo Fischer Scientific, Darmstadt, Germany) and cloned under control of the H1-promoter sequence, between XhoI and SalI restriction sites, as previously described.20 The construct contains an EGFP reporter cassette, with expression driven by the CMV promoter, to allow the detection of successfully transduced cells (see Supplementary material online, Figure S4A). Vector genome plasmids were packaged into retargeted AAV9 capsids displaying an SLRSPPS peptide motive (AAV9SLR) previously reported to efficiently transduce endothelial cells and SMCs in vitro,16 resulting in AAV9SLRcons or AAV9SLRmut. As additional controls without hairpin sequences, pdsCMV-EGFP was packed both into wild type AAV9 capsids (without targeting capsid, only low transduction of ECs and SMCs) or AAV9SLR (target the vector to specific cell types, as previously described16) resulting in AAV9WT-EGFP and AAV9SLR-EGFP, respectively. Production, purification, and titration of high titer adeno-associated viral vectors of serotype 6 (AAV-6) have been described previously.16
2.6 HP dODNs and AAV treatment
SMC treatment with hp dODNs or AAVs were performed at 80% cell confluency. Hairpin dODNs were applied to cell culture medium to a final concentration of 10 µmol/L and incubated for 2 h before IL1-β stimulation. AAV9SLR transduction was conducted at MOI = 105 virus particles/cell and EGFP fluorescence was monitored after 3 days.
2.7 Fluorescent in situ hybridization
In situ hybridization was used to detect AP-1 RNA hp dONs in AAV9SLR transduced SMCs and aortic grafts according to standard protocols21 with minor modifications. In brief, cells cultured on gelatine-coated glass coverslips or 5-µm thick aortic cryosections were fixed with 4% PFA (Sigma-Aldrich, Munich, Germany) and permeabilized by incubation with 20 µg/mL proteinase K (Sigma-Aldrich) diluted in DEPC-treated PBS at 37°C. As a probe, we used a molecular beacon containing 5′-Cy5 labelling and 3′-BHQ-2 (Black Hole Quencher) as a quencher (Biomers, Ulm, Germany) with a complementary sequence to the AP-1 RNA hp dON. The hybridization was accomplished in 4x SSC (saline-sodium citrate) buffer containing 50% deionized formamide (Sigma-Aldrich) and 1× Denhard’s solution in the presence of salmon DNA (Thermo Fischer Scientific) and yeast tRNA (Sigma-Aldrich) as blocking reagents, at 55°C for 16 h. Afterward, a series of intensive washing steps with hybridization buffer were conducted to prevent non-specific binding of the probe. During the procedure, cells expressing EGFP exhibited a pronounced decrease in green fluorescence, presumably due to the formamide present in the hybridization buffer. Therefore, EGFP immunocytochemistry was conducted to determine transduced cells. The localization of AP-1 RNA hp decoy ONs was determined using confocal microscopy (Leica TCS SP8, Leica Microsystems, Mannheim, Germany).
2.8 Gel and in situ zymography
Gelatin zymography was performed as previously described.22 The concentration of MMPs present in the cell culture supernatant was accomplished by incubation with gelatine-coated beads (Sepharose 4B Beads, Sigma Aldrich) for 24 h at 4°C, followed by elution in a non-reducing sample buffer and loaded onto 12% acrylamide gels containing 5 mg/mL gelatine (Sigma Aldrich). Following electrophoresis washing in Triton-X100 containing buffer for 2 h induced SDS removal and enzyme renaturation. Subsequently, gels were incubated at 37°C in developing buffer overnight to allow gelatine lysis and stained with Coomassie Brilliant Blue containing buffer. White bands, representing the degraded gelatine substrate, were then analysed by ImageJ. In situ zymography was used to detect the intracellular MMP activity in both cultured primary SMCs and aortic frozen sections. For this purpose, SMCs or tissue cryosections were treated with 0.1 µg/mL 520 MMP FRET Substrate XIV (Anaspec, Göttingen, Germany) which upon enzymatic cleavage by MMP-1, 2, 3, 7, 8, 9, 12, or 13 leads to the separation of the quencher (QXL) and the fluorophore 5-FAM (5-carboxyfluorescein, Ex/Em = 494/521 nm). The resulting fluorescence was visualized by confocal microscopy and mean intensities of the different treatment groups were analysed using ImageJ.
2.9 Quantitative reverse transcriptase PCR
Total RNA was extracted from cells and tissues using RNeasy Mini kit (Qiagen, Hilden, Germany) according to the instructions provided by the manufacturer. First-strand cDNA synthesis was achieved using the Omniscript Reverse Transcriptase kit (Qiagen) and OligodT primers (Promega, Mannheim, Germany). Genomic DNA was extracted from aortic tissue using the PureLink™ Genomic DNA Mini kit (Thermo Fischer Scientific). Quantitative PCR was executed using SYBR Green dye (Qiagen) and specific primers for the genes of interest (sequences presented in Table 1 and see Supplementary material online, Table S1), by using the Qiagen Rotor-Gene device. Gene expression was normalized to RPL32 or GAPDH.
Gene . | Sequence . | Annealing temperature . |
---|---|---|
EGFP | forward: 5′-AAGCAGCACGACTTCTTCAAGTC-3′ reverse: 5′-TCGCCCTCGAACTTCACCTC-3′ | 60°C |
MCP-1 | forward: 5′-TTCCTCCACCACCATGCAG-3′ reverse: 5′-CCAGCCGGCAACTGTGA-3′ | 60°C |
MMP9 | Qiagen (QT00108815) | 55°C |
RPL32 | forward: 5′-GGGAGCAACAAGAAAACCAA-3′ reverse: 5′-ATTGTGGACCAGGAACTTGC-3′ | 55°C |
Gene . | Sequence . | Annealing temperature . |
---|---|---|
EGFP | forward: 5′-AAGCAGCACGACTTCTTCAAGTC-3′ reverse: 5′-TCGCCCTCGAACTTCACCTC-3′ | 60°C |
MCP-1 | forward: 5′-TTCCTCCACCACCATGCAG-3′ reverse: 5′-CCAGCCGGCAACTGTGA-3′ | 60°C |
MMP9 | Qiagen (QT00108815) | 55°C |
RPL32 | forward: 5′-GGGAGCAACAAGAAAACCAA-3′ reverse: 5′-ATTGTGGACCAGGAACTTGC-3′ | 55°C |
Gene . | Sequence . | Annealing temperature . |
---|---|---|
EGFP | forward: 5′-AAGCAGCACGACTTCTTCAAGTC-3′ reverse: 5′-TCGCCCTCGAACTTCACCTC-3′ | 60°C |
MCP-1 | forward: 5′-TTCCTCCACCACCATGCAG-3′ reverse: 5′-CCAGCCGGCAACTGTGA-3′ | 60°C |
MMP9 | Qiagen (QT00108815) | 55°C |
RPL32 | forward: 5′-GGGAGCAACAAGAAAACCAA-3′ reverse: 5′-ATTGTGGACCAGGAACTTGC-3′ | 55°C |
Gene . | Sequence . | Annealing temperature . |
---|---|---|
EGFP | forward: 5′-AAGCAGCACGACTTCTTCAAGTC-3′ reverse: 5′-TCGCCCTCGAACTTCACCTC-3′ | 60°C |
MCP-1 | forward: 5′-TTCCTCCACCACCATGCAG-3′ reverse: 5′-CCAGCCGGCAACTGTGA-3′ | 60°C |
MMP9 | Qiagen (QT00108815) | 55°C |
RPL32 | forward: 5′-GGGAGCAACAAGAAAACCAA-3′ reverse: 5′-ATTGTGGACCAGGAACTTGC-3′ | 55°C |
2.10 MMP9 western blot analysis
Total protein was extracted using reducing RIPA lysis buffer (Sigma-Aldrich), supplemented with 0.1 mol/L DTT, 25 mol/L protease inhibitors and 50 mol/L Pefabloc SC (Sigma-Aldrich). Proteins were separated using SDS-polyacrylamide gel electrophoresis and transferred onto polyvinylidene difluoride membrane (Merck Millipore, Darmstadt, Germany). Blocking of non-specific binding was carried out by incubation with 5% non-fat dry milk (Roth, Karlsruhe, Germany). MMP9 primary antibody (Abcam, Cambridge, UK) was used to a dilution of 1:1000 and the detection was made by enhanced chemiluminescence method using ImageQuant LAS 4000 mini system (GE Healthcare Life Sciences, Munich, Germany). β-Actin (Abcam) was used as a loading control.
2.11 Immunofluorescence analysis
Cells and 5-µm thick aortic frozen sections were fixed with 4% PFA (Sigma-Aldrich) or Zinc fixative, respectively. Primary antibodies used and specific dilutions were as follows: EGFP and Ki67 (Abcam, 1:1000), F4/80 (Dianova, Hamburg, Germany, 1:500), CD31 (Santa Cruz, Heidelberg, Germany, 1:500), MMP2, MMP9, and MMP12 (Abcam, 1:1000), SMA (Abcam, 1:500), ZO-1 (Thermo Fischer Scientific, Darmstadt, Germany, 1:500). Corresponding fluorescence dye-labelled secondary antibodies (Dianova, Hamburg, Germany) were diluted 1:300 before use. Images were recorded using confocal microscopy and analysed by ImageJ. Colocalization was assessed using Coloc2 plugin (ImageJ).
2.12 Histological analysis
Verhoeff-van Gieson Staining was performed using the Elastic Stain kit (Sigma Aldrich) according to the manufacturer’s instructions. The degree of fragmentation of elastic fibres was assessed by counting the number of ‘islands of damage’ per field of view, as previously described.23
2.13 Reactive oxygen species measurement
The amount of reactive oxygen species (ROS) generated in situ in SMCs or aortic cryosections was detected using the dihydroethidine (DHE) dye as previously reported.24 Fluorescence images were captured using a confocal microscope and mean fluorescence intensity was assessed by ImageJ (version 1.52e, 2018).
2.14 RNA sequencing and analysis
RNA sequencing was performed from 800 ng starting material (Novogene, Cambridge). RNA sequencing data were analysed using R (version 3.6.3). Transcripts were aligned to the human genome (hg38) using the Rsubread package (version 2.2.4).25 Differentially expressed genes were calculated using DESeq2 (version 1.28.1).26 Genes were ranked using the apeglm algorithm.27 Gene set enrichment analyses for KEGG, GO and Reactome terms were performed with 100 000 permutations using the ClusterProfiler (version 3.16.0)28 and ReactomePA (version 1.32.0)29 packages. All genes with a significant expression were subjected to a promoter analysis using TFBSTools (version 1.26.0).30 The promoter region was determined 500 bp upstream and 100 bp downstream of the transcription start site. The following motifs were attributed to AP-1: MA0476.1, MA0488.1, MA0489.1, MA0491.1, MA0492.1 (JASPAR2020 version 0.99.10).31 The number of non-overlapping sites was calculated for each gene using a minimal score of 95% for each motif. Genes with two or more distinct sites were considered target genes. Clustered heatmaps were created using heatmap3 (version 1.1.7). KEGG pathways were visualized using pathview 1.28.1.
2.15 Statistical methods
Data are presented as means ± standard deviation and graphs were generated using GraphPad Prism 7 (GraphPad Software, USA). Statistical differences between treatment groups were analysed by employing one-way analysis of variance followed by Tukey’s multiple comparisons test for particular pairs of groups using GraphPad InStat 3.06 software. Normal distribution was assessed by the D'Agostino–Pearson test. Mann–Whitney U test was used to compare two groups. A P-value lower than 0.05 was considered statistically significant.
3. Results
3.1 AP-1 hp dODN decreased MMP gene expression and activity in vascular SMCs of Marfan mice
We have recently shown that primary aortic SMCs from mgR/mgR mice can take up AP-1 dODNs without the use of transfection reagents, such as Lipofectamine, leading to effective nuclear import.15 Therefore, to verify AP-1 neutralization by the hpAP-1 dODNs, these SMCs were treated with the naked nucleic acid. Transcription factor activation was induced by exposure to IL1-β for 8 h, which caused a significant increase in mRNA levels of AP-1 target genes MMP9 and MCP-1 as compared to non-stimulated controls, whereas treatment with the consensus hpAP-1 dODN before IL1-β exposure caused a significant decrease in expression of both AP-1 target genes (see Supplementary material online, Figure S1A and B). The mutant control dODN did not affect IL1-β stimulated MMP9 or MCP-1 gene expression, confirming the specificity of our approach.
To further confirm these findings on the protein and enzyme activity level, total lysates of SMCs treated with dODNs were subjected to MMP9 Western blot analysis (see Supplementary material online, Figure S1E). Gelatinase activity was determined in cell culture supernatants (see Supplementary material online, Figure S1F). In our hands, the most prominent band detected in the western blot presumably corresponded to the MMP9 homodimer (approximately 200–300 kDa). To determine the nature of the detected band, cells were treated with a small molecule (N-[4-(difluoromethoxy)phenyl]-2-[(4-oxo-6-propyl-1H-pyrimidin-2-yl)sulfanyl]-acetamide) which potently and specifically decreases MMP9 dimerization through blocking its PEX domain.32 When primary SMCs were incubated with the described compound for 24 h but not with DMSO as control, MMP9 dimerization was completely abolished and the high molecular band was not present (see Supplementary material online, Figure S2). Thus, the 250 kDa protein band was used for further analysis.
Our results show that pre-incubation with the AP-1 cons hp dODNs causes a significant decrease in IL1-β up-regulation of MMP9 protein whereas the mutated control dODN did not influence MMP9 protein abundance (see Supplementary material online, Figure S1C). Gelatinase activity in the supernatant of the SMCs was further investigated by gel zymography. According to this method, the 105 kDa band corresponding to the monomeric form of MMP9 had the highest gelatinolytic activity (see Supplementary material online, Figure S1F). In agreement with the results on MMP9 protein abundance, exposure to the AP-1 hp dODN but not the mutant control dODN led to a substantial reduction in the activity of secreted MMP9, as shown in see Supplementary material online, Figure S1D. The same result was obtained concerning total intracellular MMP activity, as detected by employing a broad-spectrum FRET substrate (see Supplementary material online, Figure S3A and B).
3.2 AP-1 hp dONs are expressed in vascular SMCs after AAV9SLR transduction and decrease AP-1 target genes and MMP activity
AP-1 hp dONs are encoded as hairpin RNAs under the control of the H1 promoter (see Supplementary material online, Figure S4A and B). EGFP expression, driven by the CMV promoter could be detected in successfully transduced medial cells. Subsequently, to confirm the generation of the hpAP-1 dON after adeno-associated viral transduction, we performed RNA F.I.S.H. using a molecular beacon with a complementary sequence to the target hairpin RNA molecule. Red fluorescence emitted by specific binding of the probe to the hairpin RNA molecule was observed only in SMCs transduced with AAV9SLR expressing the AP-1 hp dON but not in AAV9SLR-EGFP treated cells (see Supplementary material online, Figure S4C). No fluorescence was noted in the non-transduced control SMCs accounting for the specificity of this method.
Similar to treatment with the naked AP-1 hp dODN, transduction with AAV9SLRcons significantly decreased expression of AP-1 target genes MMP9 (Figure 1A) and MCP-1 (Figure 1B). To corroborate the gene expression analysis results, we conducted MMP9 immunocytochemistry following AAV9 treatment and IL1-β stimulation. Red fluorescence corresponding to MMP9 protein was analysed in transduced EGFP-positive cells. Through this method, we could detect a robust decrease by 50% in MMP9 protein following transduction with AAV9SLRcons but not with AAV9SLRmut (Figure 1D and E). The same observations were made about the MMP9-specific activity in SMC supernatants. The results of both gel and in situ zymography verified that AAV9SLR-mediated generation of AP-1 hp dON abrogates IL1-β-induced MMP activity, as shown in Figure 1C and see Supplementary material online, Figure S3C and D.
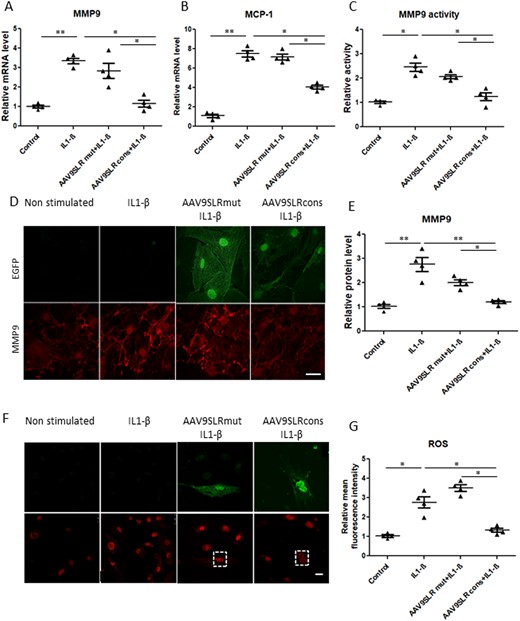
AAV9SLRcons significantly decreases AP-1 target genes. Evaluation of (A) MMP9 and (B) MCP-1 gene expression in transduced SMCs by quantitative RT-PCR. Messenger RNA levels were normalized to RPL32 as a housekeeping gene (n = 4, *P < 0.05). (C) Relative gelatinolytic capacity of secreted MMP9 in the different treatment groups with the non-stimulated control value set to 1 (n = 4, *P < 0.05). (D) Representative images of MMP9 immunocytochemistry (red) and corresponding statistical quantification (E) of the fluorescence intensity. (F) Representative images showing DHE fluorescence in vascular SMCs of the indicated treatment groups. Transduced cells were visualized in the green channel. (G) Statistical quantification of mean red fluorescence intensity as a measure of generated ROS in SMCs in different treatment groups. The non-stimulated group served as a control. Scale bar represents 25 μm (n = 4, 20 images analysed/group, *P < 0.05). Data were analysed using one-way analysis of variance (ANOVA), followed by a post hoc Tukey multiple comparison test.
Furthermore, we could detect a marked increase in contractility markers SMA, SM22a and calponin and reduced proliferative capacity following AP-1 neutralization by AAV9SLR. On the other hand, apoptosis remained unchanged (see Supplementary material online, Figure S5A–E), Treatment with IL1-β led to a marked increased cell proliferation, evidenced by elevated mRNA levels of Ki67, PCNA, and CDK5, while AP-1 neutralization decreased cytokine-induced cell growth (see Supplementary material online, Figure S5F–H). Furthermore, by immunocytochemistry we could detect a significantly higher percentage of Ki67-positive cells following IL1-β addition, which was blunted by AAV9SLR cons transduction (see Supplementary material online, Figure S5I and J). The mutated AAV did not affect the analysed parameters.
To extend our study and to show the transferability to the human system, we performed a set of experiments in which we compared human SMCs isolated from aortic tissue of a Marfan patient and controls. As expected, we noted an increased MMP9 expression in SMCs isolated from patients on both protein and mRNA levels. However, the delivery of AP-1 dON by AAV transduction significantly reduced MMP9 levels, as shown in see Supplementary material online, Figure S6. Additionally, our designed gene therapy approach markedly decreased MCP-1 mRNA levels in human SMCs isolated from diseased aortae. Hence, we conclude that AAV9SLR cons do not only decrease IL1-β-induced MMP9 expression in mouse SMCs, but also stabilizes its level in human SMCs.
Additionally, we aimed to analyse the effect of AP-1 neutralization on TGF-β signalling, taking into account the well-established role of this cytokine in aneurysm progression in Marfan syndrome. For this purpose, human aortic SMCs were subjected to transduction with AAV9SLRcons and AAV9SLRmut as a control in serum-free conditions. To determine the efficacy of the proposed treatment in comparison with TGF-β neutralization, a neutralizing antibody was applied to the cell culture medium. TGF-β was measured in supernatants three 3 days after transduction by ELISA.
Our results show that the application of AAV9SLRcons leads to a marked decrease in TGF-β concentration in the SMC-conditioned medium 3 days after transduction. Similarly, TGF-β neutralization by treatment with a neutralizing antibody caused normalization of cytokine levels close to basal values of control cells (see Supplementary material online, Figure S7A). Furthermore, we determined the phosphorylation status of Smad3, a well-known downstream target of TGF-β. As expected, we could observe a significant rise in phosphorylated Smad3 (P-Smad3) levels in primary human SMCs isolated from the aorta of Marfan patient as compared to healthy control cells. In addition, application of the neutralizing anti-TGF-β antibody caused normalization of P-Smad3 levels, while decreasing the transcriptional activity of AP-1 also significantly reduced downstream TGF-β signalling (see Supplementary material online, Figure S7B and C). To show whether our AAV construct affects the expression of genes involved in the pathogenesis of Marfan syndrome similarly as TGF-β inhibition, we next measured MCP-1 mRNA levels and MMP9 protein and mRNA among the different treatment groups. Interestingly, our findings suggest that expression of the AP-1 dON by the targeted SMCs is as effective as the neutralizing anti-TGF-β antibody in decreasing MMP9 and MCP-1 expression in SMCs isolated from the aorta of Marfan mice (see Supplementary material online, Figure S7D–F) and may thus stabilize aortic aneurysms in vivo in a similar manner as the neutralizing anti-TGF-β antibody. Consistently, RNA sequencing data confirmed that dON-based neutralization of AP-1 negatively impacts on activation of the TGF-β pathway in vitro (see Supplementary material online, Figure S7G). In addition, we could identify a dramatic effect by transduction with the AAV9SLRcons on distinct signalling pathways in the SMCs isolated from the aorta of Marfan mice (see Supplementary material online, Figure S8A and Table S2). Furthermore, we could validate that pro-inflammatory cytokines and well established pro-inflammatory pathways are strongly affected by our nucleic acid-based treatment approach (see Supplementary material online, Figure S8B). To confirm the RNA sequencing results, we performed real-time qPCR experiments aiming to characterize inflammation (IL-6 and IL-10) and apoptosis (cIAP1 and cIAP2)-related markers. As proven in see Supplementary material online, Figure S8C–F, we found significantly increased mRNA levels of both interleukins and cIAP-related markers in aortic SMCs isolated from Marfan patients as compared to controls.
3.3 AAV9SLR-mediated AP-1 consensus hp dON generation decreases IL1-β induced superoxide production in vascular SMCs from Marfan mice
To determine the effect of AP-1 neutralization on ROS production in SMCs, we performed DHE staining after IL1-β stimulation. As anticipated, quantification of the fluorescence images revealed a strong increase in the amount of superoxide generated in the presence of IL-1β, which could be markedly reduced by prior transduction of the cells with AAV9SLRcons but not AAV9SLRmut (Figure 1F and G).
3.4 AP-1 hp dONs are expressed following ex vivo transduction of aortic grafts from mgR/mgR mice
The aforementioned in vitro findings prompted us to explore the effects of AAV9SLR-mediated AP-1 hp dONs delivery to aortic SMCs in vivo using the mgR/mgR mouse model for Marfan syndrome. We proceeded with ex vivo AAV9SLR transduction of thoracic aortic grafts followed by infrarenal reimplantation into recipient mgR/mgR mice. This way we could achieve transduction of both endothelial cells and the target SMCs, as shown in Figure 2A. Ex vivo application of the control non-modified AAV9 (AAV9 WT) did not yield EGFP-positive cells. To further prove that the capsid peptide SLR increases ex vivo transduction efficiency of AAV9 in the aortic tissue, we extracted genomic DNA from treated aortic grafts. EGFP expression was assessed by qPCR using specific primers. The results support the data of the immunohistochemistry analysis revealing an up to 30fold increase in EGFP expression in AAV9SLR transduced aortic grafts in comparison to the control AAV9 WT treated grafts (Figure 2B). Using RNA F.I.S.H. we additionally verified the generation of the AP-1 hp dON in the aortic tissue 30 days post-transduction (Figure 2C). To show specifically the transduction route of the AAV vector, grafts were incubated intraluminal with a solution containing AAV9SLR EGFP and transplanted into recipient mice. As shown in see Supplementary material online, Figure S9, our results prove that 2 weeks after transduction, we could achieve efficient transduction of both ECs and SMCs, suggesting that AAV9SLR primarily reaches target cells through the endothelial layer.
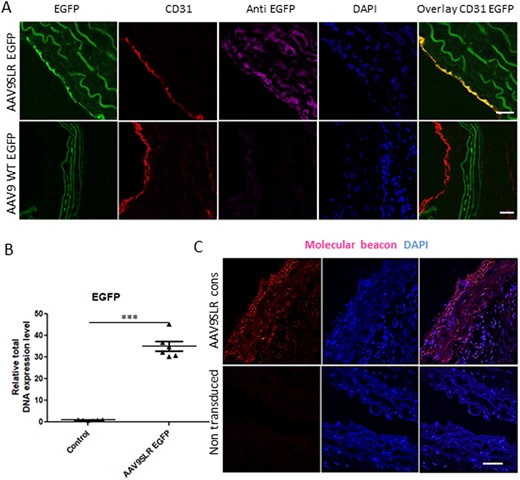
AAV9SLR-mediated AP-1 hp dONs generation in aortic grafts of mgR/mgR mice following transduction. (A) EGFP immunohistochemistry (magenta) was performed on aortic cryosections from mgR/mgR mice 30 days after ex vivo transduction with AAV9SLR. Positive staining was obtained in CD31 positive endothelial cells (red), as well as in the medial SMCs. (B) Quantification of EGFP vector genome copies among total DNA, confirming successful transduction of aortic grafts (n = 6, ***P < 0.001). (C) Illustrative images showing the formation of RNA AP-1 hp dONs 4 weeks post-transduction of aortic grafts with AAV9SLR, documented by RNA F.I.S.H. Red fluorescence indicates specific hybridization of the molecular beacon to the hairpin RNA target. Nuclei were stained with DAPI (blue). Scale bar represents 25 µm. Data were analysed using Mann–Whitney U-test.
3.5 AAV9SLR-mediated AP-1hp dONs expression decreased AP-1 target gene expression in transduced aortic grafts
Immunohistochemistry experiments demonstrated a significant decrease in MMP9, MMP2 and MMP12 protein abundance in aortic grafts after single treatment with the AP-1 hp dON-expressing AAV9SLR. As expected, transduction with the AAV9SLR expressing the mutant control dON did not affect the expression of gelatinases or elastase in the transduced aortic tissue (Figure 3A–C).
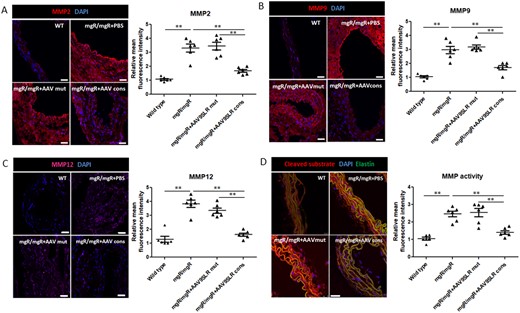
AAV9SLR-mediated AP-1 cons hp dONs delivery significantly down-regulates the expression and activity of MMPs in ex vivo treated aortic grafts of mgR/mgR mice. Immunofluorescent analysis (red) of MMP2 (A), MMP9 (B), and MMP12 (C) protein abundance in aortic cryosections of the different treatment groups. (D) Representative images of in situ zymography using a broad-spectrum FRET MMP substrate. Red fluorescence represents the cleaved compound and was quantified as the level of MMP activity. Elastin autofluorescence was recorded in the green channel and DAPI (blue) was used to counterstain nuclei. The graphs represent the statistical quantification of red fluorescence in confocal microscopy images. The values of wild type mice served as controls and were set to 1. Scale bar represents 25 μm (n = 6, **P < 0.01). Data were analysed using one-way analysis of variance (ANOVA), followed by a post hoc Tukey multiple comparison test.
Moreover, we used a broad-spectrum FRET substrate to determine global MMP activity present in the aortic sections of mice derived from the different treatment groups. The in situ zymography results revealed a significant decrease in enzymatic activity following AAV9SLRcons-mediated AP-1 neutralization, whereas AAV9SLRmut treatment had no effect (Figure 3D). This method allowed us to determine not only the gelatinases present in the samples but also other MMPs potentially important for aortic aneurysm formation in Marfan syndrome.
3.6 AAV9SLR-mediated AP-1 hp dON expression significantly improved elastic fibre structure in transduced aortic grafts
To test whether the down-regulation of MMP abundance and activity in the aortic grafts affects elastin architecture, we executed elastic van Giesson staining and counted the number of ‘islands of damage’ as a measure of fibre fragmentation. An island of damage was defined as an isolated area of the aortic wall where two adjacent elastic fibres were fragmented with interposed excessive connective tissue.23 As expected, non-treated and control AAV9SLR transduced aortic tissue from mgR/mgR mice presented with a significant degree of elastin degradation and a high number of islands of damage. In contrast, 4 weeks after transduction with AAV9SLRcons a significant improvement in elastin fibre architecture (Figure 4A). This was reflected by a significant decrease in the number of islands of damage, defined as breaks in two neighbouring elastic fibres surrounded by conjunctive tissue as published previously,23 in the treated grafts (Figure 4B).
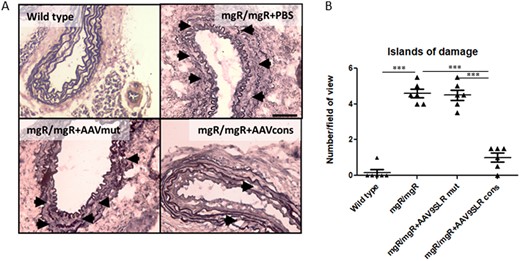
AAV9SLR-mediated hpAP-1 cons hp dONs delivery in aortic grafts from mgR/mgR mice improves elastin architecture. (A) Representative bright-field microscopy images of elastic van Gieson staining of aortic cryosections of the indicated treatment groups, explanted 30 days after surgery. Elastin is stained in black, collagen appears in red and cell nuclei are black-brown. Black arrows indicate ‘islands of damage’, defined as an isolated area of the aortic wall where two adjacent elastic fibres were fragmented with interposed excessive connective tissue.23 (B) Statistical quantification of the number of ‘islands of damage’ in different treatment groups (n = 6, 30 images analysed/group, ***P < 0.001). Scale bar represents 50 μm. Data were analysed using one-way analysis of variance (ANOVA), followed by a post hoc Tukey multiple comparison test.
3.7 AAV9SLR-mediated AP-1 hp dON generation by medial SMCs decreases monocyte infiltration and ROS formation in transduced aortic grafts
Inflammatory cell infiltration in the aortic wall has been proven to be a hallmark of aortic aneurysm in the context of Marfan syndrome and correlates with the severity of dilatation.3 According to real-time PCR analysis, there was a substantial down-regulation of MCP-1 mRNA expression in aortic tissues treated with AAV9SLRcons (Figure 5A). As expected, transduction with AAV9SLRcons but not AAV9SLRmut also led to a significant decrease in macrophage counts (Figure 5B and C). Interestingly, monocyte recruitment was noted primarily in the adventitia in our model.

AAV9SLR-mediated generation of AP-1 cons hp dONs in aortic grafts of mgR/mgR mice significantly decreases monocyte infiltration in the aortic wall. (A) MCP-1 mRNA level was assessed in transduced tissue and compared to the age-matched wild type values as control. RPL32 was used as a housekeeping gene (n = 6, ***P < 0.001). (B) Representative confocal images showing F4/80 (red) immunohistochemistry demonstrating macrophage presence in the aortic sections of the indicated treatment groups. Elastin autofluorescence was recorded in the green channel and DAPI (blue) was used to counterstain nuclei. (C) Statistical quantification of macrophage density in the adventitia of aortic cryosections from mgR/mgR mice (n = 6 for mgR/mgR mice, 20 images analysed/group, **P < 0.01). The scale bar represents 50 μm. Data were analysed using one-way analysis of variance (ANOVA), followed by a post hoc Tukey multiple comparison test.
As already demonstrated with cultured SMCs, the amount of oxidative stress detected by the fluorescent probe DHE was significantly decreased by 40% in grafts transduced with AAV9SLRcons in comparison to AAV9SLRmut transduction and non-treated aortic grafts (Figure 6).
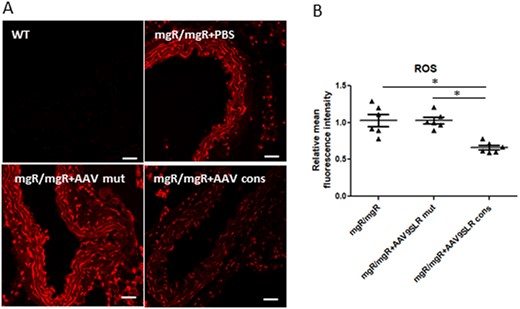
AAV9SLR-mediated AP-1 cons hp dONs expression significantly decrease the amount of superoxide in ex vivo transduced aortic grafts from mgR/mgR mice. (A) ROS production in transduced aortic cryosections was assessed using DHE staining (red). Scale bar represents 25 μm. (B) Analysis of DHE-derived mean fluorescence intensity with the aortic frozen sections from non-treated mgR/mgR mice set to 1 (n = 6, 20 images analysed/group, *P < 0.05). The complete data set of the original images is presented in the Supplementary material online, SupplMat_Figure6.pdf.
MMP9 was shown to affect endothelial tight junction integrity in various models, hence promoting endothelial permeability.33 Therefore, we hypothesize that AAV-mediated reduction in MMP9 activity increases the ZO-1 protein level, hence improving endothelial function. For this purpose, we transduced HUVECs with the designed viral vectors, and applied a specific MMP9 inhibitor (CAS 1177749-58-4, Santa Cruz) in addition to IL1-β (see Supplementary material online, Figure S10A and B). As expected, IL1-β stimulation led to a marked decrease in ZO-1 protein level, as proved by immunocytochemistry. HUVEC transduction with AAV9SLR cons led to normalization of ZO-1 expression, while AAV9SLR mut did not affect the expression of the tight junction protein. MMP9 inhibition led to a similar effect, proving that the matrix-metalloprotease is a critical factor leading to ZO-1 degradation (see Supplementary material online, Figure S10D and E).
Moreover, to assess whether increased endothelial tight junctions by AP-1 neutralization protects against monocyte transmigration, we used a transwell-based assay in which we added THP monocytic cells to a confluent HUVEC layer. Our results prove that IL1-β caused a significant increase in endothelial cell permeability, as shown by an elevated THP cell transmigration to the lower well of the insert. However, transduction with AAV9SLR cons and MMP9 inhibition before cytokine addition significantly reduced the number of migrated cells. Addition of MMP9 inhibitor had a similar effect, and potently decreased monocyte transmigration through the endothelial barrier (see Supplementary material online, Figure S10C).
3.8 AAV9SLR-mediated AP-1 hp dONs delivery increased the endothelial tight junction protein ZO-1 in endothelial cells of transduced grafts
In addition, we noted that the capacity of AAV9SLR to transduce medial SMCs in aortic grafts of mgR/mgR mice is considerably higher in contrast to WT mice (cf. Figure 2A). On the other hand, endothelial cells, identified by CD31 immunohistochemistry, were equally efficiently transduced in mgR/mgR and WT mice (Figure 7A). Presumably, the increased permeability of the endothelial cell monolayer in mgR/mgR mice enables the viral vector to reach the medial SMCs more easily (quantification of EGFP immunofluorescence intensity presented in Figure 7B and C). To prove this hypothesis, we performed immunohistochemistry using a specific antibody for the tight junction protein ZO-1, a pivotal regulator of the integrity of the endothelial cell monolayer.34 In fact, there was a significant 60% decrease in ZO-1 protein abundance in endothelial cells of mgR/mgR mice as compared to wild type animals. AP-1 neutralization through transduction with AAV9SLRcons significantly restored ZO-1 protein levels, as shown in Figure 7D and E.
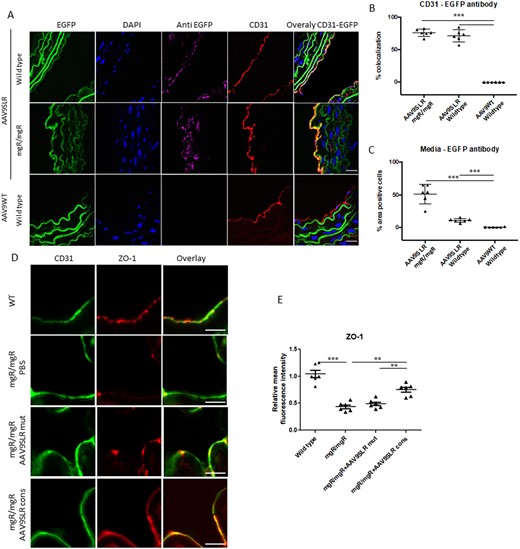
AAV9SLR-mediated AP-1 cons hp dONs generation significantly increases the expression of tight junction protein ZO-1 in ex vivo transduced aortic grafts from mgR/mgR mice. (A) Representative images showing AAV9SLR-EGFP transduction efficiency in aortic grafts from mgR/mgR and wild type mice. CD31 (red) staining was used to mark endothelial cells, and EGFP immunohistochemistry (magenta) was employed to determine EGFP positive cells. Elastin fibre autofluorescence (green) and DAPI nuclear staining (blue) are also shown. The scale bar represents 25 μm (n = 3, exemplary images). (B) Statistical quantification of percentage colocalization of CD31 and EGFP signal, as a marker of endothelial cell transduction. (C) Evaluation of EGFP positive cells in the media of transduced aortic tissue from mgR/mgR and wild-type mice, quantified as percentage area. (D) Representative confocal images of aortic cryosections from mgR/mgR mice stained with antibodies recognizing CD31 (green) and ZO-1 (red) as well as statistical quantification of ZO-1 protein abundance (E). The scale bar represents 75 μm (n = 6 for WT mice, n = 6 for mgR/mgR mice, 20 images were analysed/group, **P < 0.01, ***P < 0.001).
4. Discussion
Aortic aneurysm dissection represents the main mortality risk of patients with Marfan syndrome. Current treatment options such as the administration of β-adrenergic blocking compounds or prophylactic aortic root replacement solely offer either a limited or highly invasive solution for this cardiovascular complication35 and therefore the advancement of new therapeutic strategies is essential. In this study, we delineate a novel single treatment approach of preserving the structural integrity of the aortic wall and reducing the concomitant inflammatory reaction.
Our results strongly support a pivotal role of the AP-1 transcription factor family in elastin fibre degradation, which contributes both to aortic fragility and dissection of aneurysms. Previous studies employing the mgR/mgR mouse model of Marfan syndrome revealed some of the molecular mechanisms involved in the enlargement of the ascending aorta.36 Apart from its structural role in the assembly of the microfibrils,37 fibrillin-1 is a critical protein that keeps TGF-β in an inactive state.38 AP-1 transcription factors are key regulators of MMP gene expression hence activity.39 Activation of AP-1 is elevated in the presence of TGF-β12 and controls expression of gene products that are involved in the formation of thoracic aneurysms, such as MMPs and pro-inflammatory cytokines. Accordingly, we have previously shown a strong intrinsic activation of this transcription factor family in aortic SMCs isolated from mgR/mgR mice.15 Neutralization of AP-1 in aortic grafts from these animals through administration of a naked dODN led to normalization of the elastin structure, down-regulation of MMPs and a substantially reduced infiltration of pro-inflammatory cells into the treated grafts.15 These results indicate that such a strategy may be feasible for delaying aortic aneurysm dissection in Marfan syndrome. The present study advances this approach to a single long-term treatment option by using AP-1 hp dONs expressed as hairpin RNAs under the control of the H1 promoter via AAV-mediated targeted transduction of medial SMCs. Its possible benefit is that one-time treatment with the viral vectors may be sufficient for persistent generation of the active nucleic acid-based drugs in the aortic tissue.
Recent investigations on molecular mechanisms indicate that increased TGF-β activity also plays a crucial role in the pathogenesis of MFS-related disorders, such as Loeys–Dietz syndrome (LDS), caused by mutation in the mentioned TGF-β signalling-related genes.40 Enhanced MMP9 and MMP2 activity is a hallmark of non-genetic types of aortic aneurysms.41,42 Hence, our therapeutic approach might be a therapeutic option for LDS or aneurysms of different aetiology.
Another valuable observation of our study is the dramatic effect of AP-1 hpdONs on TGF-β signalling, a classical pathway dysregulated in Marfan syndrome patients. Not only our treatment could decrease downstream signalling and secreted cytokine concentration, but we could also prove that our strategy is as effective in down-regulating MCP-1 and MMP9 as the application of a TGF-β antibody in vitro.
The intracellular production of transcription factors dONs through a plasmid or viral vector is a feasible method of dON delivery to cells.43 By using an ssDNA expression vector, the generation of any ssDNA is possible.43 Nevertheless, the expression of a reverse transcriptase must be considered a drawback for further clinical application due to potential side effects. To overcome this drawback, a new strategy has been described in the literature, in which dONs are generated using a hairpin RNA-expressing plasmid vector.44 In our study, AAV9SLR vectors were used to express the AP-1 hp dONs in the aortic tissue of wildtype and mgR/mgR mice. This particular AAV vector contains an engineered capsid variant that previously derived from a randomized library and efficiently transduces ECs and SMCs of human origin in vitro16 and can transduce ECs after local intradermal injection in mice.45 Here, we show that AAV9SLR is an optimal vector for the generation of hairpin RNA-based dONs in SMCs derived from the aorta of mgR/mgR mice in vitro. Furthermore, we confirmed that AAV9SLR successfully transduces both ECs and SMCs cells in aortic grafts of these animals when administered ex vivo, i.e. during the period of warm ischaemia. In strong contrast, AAV9WT did not lead to vascular cell transduction, as expected.
Furthermore, using RNA F.I.S.H. we could verify the generation of the AP-1 hp dONs in the ex vivo transduced aortic tissue and, more importantly, a significant down-regulation in the expression of the AP-1 target genes MCP-1 and MMP9 both in the cultured cells and in the aortic grafts. These observations were validated on the protein level both in cultured SMCs and in aortic cryosections. In situ zymography using a broad-range MMP substrate that is cleaved by MMP1, 2, 3, 7, 8, 9, 12, and 13 confirmed a significant reduction of total MMP activity.46
The contribution of ROS, mainly produced by macrophages and SMCs,47 in the pathogenesis of aortic aneurysm formation, has already been extensively investigated and shown to be dependent on AP-1.48,49 Correspondingly, we could show that treatment with an AAV9SLR neutralizing AP-1 could normalize enhanced oxidative stress levels in aortic grafts of mgR/mgR mice. Disturbances in ROS homeostasis increase the expression of chemokines that in turn reinforce pro-inflammatory cell infiltration into the aortic vessel wall.50 The transmigration of monocytes into the adventitia and media where they differentiate into macrophages is thought to be an initial step in the progression towards aortic aneurysm formation in patients with Marfan syndrome,51 and is enhanced by elastin strand breaks.52 On the other hand, macrophages can lead to exacerbation of extracellular matrix degradation through MMP secretion.3 Infection with AAV9SLR expressing the consensus AP-1 hp dON resulted in a decline in macrophage abundance in the adventitia of the mgR/mgR mouse aortae. Similar to previous studies targeting inflammation in Marfan syndrome by treatment with indomethacin,53 our data show that macrophage-derived elastase MMP12 protein abundance is down-regulated in AAV9SLRcons transduced aortic grafts. Furthermore, we could demonstrate decreased activation of pro-inflammatory pathways in cultured SMCs isolated from Marfan patients upon treatment with our viral construct. These results point to the beneficial effect of AP-1 neutralization on the pro-inflammatory environment in the aortic vessel wall in Marfan syndrome, as also confirmed by RNAseq analysis. Notably, we could detect a marked decrease in IL-6 and IL-10 mRNA levels following AAV9SLRcons transduction, proving that our gene therapy approach can limit the exacerbated pro-inflammatory mediators leading to aneurysm progression.3 Especially the observed IL-6 reduction is remarkable, as this interleukin has been described as a biomarker reflecting the dilation progression of the patient aorta.54 Interestingly, cIAP-2 was previously demonstrated to be up-regulated in human abdominal aortic aneurysm samples as a hypoxia-induced pro-survival stimulus.55 Apart from their role in inhibiting apoptosis, the IAP family was proven to induce the activation of the NF-κB pathway and activate transcription of TGF-β in a Smad4-dependent manner.56
Together with the observed direct effects on TGF-β itself and the related down-stream SMAD proteins, our broad-spectrum approach to Marfan syndrome is one that addresses multiple pathways and prevents elastolysis indicating a clinical rationale for using this new approach instead of single TGF-β neutralization. The AP-1 hp dON AAV approach may also be combined with other potential therapies that have already been shown to improve the signs and symptoms of Marfan syndrome.
In strong contrast to the mgR/mgR mouse grafts, we demonstrated that the capacity of AAV9SLR to transduce SMCs in the media of wild type mouse grafts is significantly reduced. Thus, there seems to be a problem with endothelial cell integrity in the aorta of the Marfan mice15,57 which is governed by the tight junction protein ZO-1.34 The existing endothelial barrier dysfunction has already been documented in these mice by a significant increase of albumin diffusion through the endothelial barrier.57 In fact, decreased expression of ZO-1 has been shown in rat aneurysm tissue causing enhanced infiltration of monocytes into the aortic vessel wall.58 Oxidative stress has also been shown to impair endothelial cell function by reducing the expression of tight junction proteins.50 In line with these findings, we detected a significant decline in ZO-1 protein abundance in the endothelial cell monolayer of mgR/mgR aortae as compared to their wild type counterparts, reinforcing the notion of a reduced integrity of the endothelial cell monolayer. Increased permeability of the monolayer correlates with pro-inflammatory cell recruitment in various models of vascular disease.59 Moreover, MMP9 and MMP2 have been shown to degrade ZO-1,33 and thus may explain increased permeability in the aortic grafts derived from the Marfan mice which explains AAV-mediated transduction of SMCs in aortas from Marfan but not wild type mice. Also, the absence of SMC transduction in healthy aortas may constitute thus preventing elastolysis and possibly allowing long-term reduction of aneurysm formation at an early stage of the disease. Since gene transfer of the AAV9SLRcons but not the AAV9SLRmut vector restored ZO-1 protein abundance and prevented aortic elastolysis in our animal model, this approach may stabilize the aortic vessel wall in patients with Marfan syndrome and delay progression and dissection of aortic aneurysms.
Despite clear effects of the AP-1 hp dOND expressing viral vector on the aortic grafts from the mgR/mgR mice, the approach as such cannot be transferred into clinical practice due to the ex vivo application of the vector, and thus needs refinement. In addition to vascular mechanical wall stress, haemodynamics force or kinetic energy produced by the blood through the arteries also plays a key role in aneurysm growth and rupture.60 Thus, given the increased haemodynamic forces affecting the ascending aorta where the majority of aneurysms forms in Marfan syndrome patients occur, mechanical forces and constraints on the aorta at the infrarenal position are less important. Further studies are necessary to show whether the protective effect of our gene transfer approach is also effective in the ascending aorta. Future studies may also address the infiltration of TH17 cells and neutrophils as these cell types are also involved in the onset and progression of aortic aneurysm. With the current experimental protocol, we also could not monitor the long-term survival rate of the Marfan mice. Such experiments would be rather meaningful taking into account that the life span of these animals is significantly reduced due to thoracic aorta aneurysms dissection.61
In conclusion, our study provides the basis for a novel and innovative approach to treat aortic aneurysms associated with Marfan syndrome. Although we focused on this particular disorder herein, this approach could be feasible for the treatment of other vascular remodelling processes with a strong pro-inflammatory component as well.
Supplementary material
Supplementary material is available at Cardiovascular Research online.
Authors’ contributions
A.R., M.H., O.J.M., and A.H.W. wrote the manuscript. R.A., M.Z., T.P., N.F., M.K., K.K., M.H., O.J.M., and A.H.W. designed the experiments. R.A., M.F., A.J., M.Z., M.B.H., O.J.M., and A.H.W. performed the experiments and analysed the data. All authors read the manuscript and provided critical input.
Time for primary review: 20 days
Acknowledgements
We are grateful for the expert technical assistance and help provided by Antje Weber and Franziska Mohr.
Conflict of interest: The authors declare that they have no conflicts of interest and that they have no affiliations with or involvement in any organization or entity with any financial interest.
Funding
This work was supported by grants from the German Marfan Foundation, Eutin, Germany to O.J.M. and K.K., and from the German Centre for Cardiovascular Research, Berlin, Germany to O.J.M.
Data availability
All data underlying the findings described in this manuscript and materials are fully available without restriction and will be shared on request to the corresponding author.
References
This study provides a novel single treatment option to achieve long-term expression of a transcription factor AP-1 neutralizing decoy oligonucleotide in the aorta of mgR/mgR mice with the potential to prevent life-threatening elastolysis and aortic complications.
Author notes
Oliver J. Müller and Andreas H. Wagner contributed equally to this work.