-
PDF
- Split View
-
Views
-
Cite
Cite
Yifei Zhu, Lei Wen, Sheng Wang, Ka Zhang, Yue Cui, Chi Zhang, Lei Feng, Fan Yu, Yongquan Chen, Ruxing Wang, Xin Ma, Omega-3 fatty acids improve flow-induced vasodilation by enhancing TRPV4 in arteries from diet-induced obese mice, Cardiovascular Research, Volume 117, Issue 12, 1 November 2021, Pages 2450–2458, https://doi.org/10.1093/cvr/cvaa296
- Share Icon Share
Abstract
Previous studies have shown the intake of omega-3 polyunsaturated fatty acids is associated with low rates of obesity and ischaemic pathologies. Omega-3 also have anti-inflammatory and plaque-stabilization effects and regulate vasodilation and constriction. However, there are few studies of the role of omega-3 in flow-induced vasodilation involving Ca2+-permeable ion channel TRPV4 in high-fat diet-induced obese (DIO) mouse. Here, we determined whether omega-3 protect against vascular dysfunction induced by a high-fat diet by enhancing TRPV4 activity and subsequently improving flow-mediated vasodilation.
Flow-mediated vasodilation in second-order mesenteric arteries from mice was measured using a pressure myograph. The intracellular Ca2+ concentration in response to flow and GSK1016790A (a TRPV4 agonist) was measured by Fluo-4 fluorescence. Whole-cell current was measured by patch clamp. Cell membrane tether force was measured by atomic force microscopy. Impairment of flow-mediated vasodilation in arteries and Ca2+ influx in endothelial cells from DIO mice was restored by omega-3 treatment. The improved flow-induced vasodilation was inhibited by the TRPV4 antagonist HC067047 and in TRPV4−/− mice. Omega-3 treatment enhanced endothelial TRPV4 activity and altered cell membrane mechanic property, as indicated by enhanced GSK1016790A-induced Ca2+ influx and whole-cell current and altered membrane mean tether force in endothelial cells from DIO mice.
Omega-3 improve vascular function by improving flow-induced vasodilation via enhancing TRPV4 activity in the endothelium of obese mice which may be related to improved cell membrane physical property. Activation of TRPV4 in endothelium plays an important role in the protective mechanisms of omega-3 against vascular dysfunction in obesity by improving flow-mediated vasodilation.
1. Introduction
It is well known that high-fat diet-induced obesity (DIO) is strongly associated with impairment of endothelial function as well as endothelium-dependent vascular tone.1 Clinical studies have shown that obese patients present increased vasomotor tone and hypertension.2
Polyunsaturated fatty acids (PUFAs), such as eicosapentaenoic acid (EPA) and docosahexaenoic acid (DHA), are widely regarded as protectors against cardiovascular dysfunction, and this is attributed to the regulation of vasodilation.3–7 However, little is known about the role of omega-3 PUFAs in flow-induced vasodilation. Previous studies have shown that the intake of omega-3 PUFAs is associated with low rates of obesity and ischaemic pathologies such as myocardial infarction and atherosclerosis.7,8 The precise molecular mechanisms underlying the PUFAs-mediated vascular protection have not been fully determined. It has been reported that omega-3 PUFAs affect cell membrane fluidity, regulate membrane Na+ and Ca2+ channels,3 and regulate arterial wall remodeling.9 They also have anti-inflammatory and plaque-stabilization effects in endothelial cells (ECs).10,11 The target of PUFAs in the vascular system seems to be the non-selective Ca2+ channel transient receptor potential channel subfamily V member 4 (TRPV4), which is known to be a mechano-activated channel that is activated by shear stress (flow).12 Ca2+ signals of TRPV4 channels regulate vascular function.13 Moreover, TRPV4 was shown to be impaired in cardiovascular disease including obesity.14,15 But little is known about its role in the improvement of endothelial function induced by PUFAs in obesity.
The aim of this work was to determine whether TRPV4 plays a role in the vascular protective effect of PUFAs in obesity. We addressed the following questions: (i) do PUFAs improve the flow-induced vasodilation of resistance arteries in obesity and (ii) does TRPV4 play a vital role in the PUFAs-induced improvement of vascular function in obesity, which reveal a protective mechanism?
We investigated the effect of PUFAs on flow-induced vasodilation in mouse mesenteric artery, analysed the expression and activity of TRPV4 as well as NO production in PUFAs-treated ECs of DIO mouse mesenteric artery, and used TRPV4−/− mice to establish that omega-3 PUFAs improve flow-induced vasodilation by enhancing TRPV4 activity in resistance arteries from DIO mouse.
2. Methods
2.1 Animal protocols
TRPV4 knockout (TRPV4−/−) mice on the C57BL/6J background were obtained from Dr. Makoto Suzuki (Jichi Medical School, Tochigi, Japan and RIKEN BioResource Center, Japan) and maintained at Jiangnan University. The mice were kept at 22–23°C under a 12 h light/dark cycle. From the age of 6 weeks, male TRPV4−/− or wild-type (WT) mice were fed with standard chow (Control) or a high-fat diet (45% kCal from fat, TP23200, Trophic Animal Feed High-tech Co., Ltd., Nantong, China) for 3 months to develop DIO. TRPV4−/− and WT littermates were selected by an established polymerase chain reaction genotyping method (Supplementary material online, Figure S1) for use in this study. CO2 inhalation was used as the method for euthanasia. All animal experiments were performed in accordance with the guidelines of the National Institutes of Health, USA, and were approved by the Animal Experimentation Ethics Committee of Jiangnan University (the approval number: JN.No20190430c1801231[86]).
2.2 Primary cells
Mouse mesenteric artery endothelial cells (MMAECs) were isolated and cultured in EC medium (ScienCell, Carlsbad, CA, USA). Confluent cells at passage 3 or less were used. To obtain cells, mice were killed by CO2 asphyxiation. For MMAECs, the small intestine was dissected, venous branches were rapidly excised, and arterial branches were digested with 0.02% collagenase type IA (Sigma) in phosphate-buffered saline [PBS (in mmol/L); 140 NaCl, 3 KCl, 10 Na2HPO4, and 1.8 KH2PO4; pH 7.4] for 35 min at 37°C. The digested tissues were centrifuged at 1600 g for 5 min, and pelleted cells were re-suspended in Dulbecco’s modified Eagle’s medium (DMEM; Gibco) supplemented with 10% fetal bovine serum, 100 µg/mL penicillin, and 100 U/mL streptomycin. The medium was changed 2 h later and the remaining adherent ECs were cultured at 37°C under 5% CO2. Cells were incubated with or without 30 µmol/L EPA and 30 µmol/L DHA overnight at 37°C under 5% CO2 if necessary.
2.3 Organ culture of mesenteric arterial rings
Mouse mesenteric arterial rings were dissected and incubated in fresh sterile Krebs solution supplemented with or without 30 µmol/L EPA and 30 µmol/L DHA for 3 h at 37°C under 5% CO2. After the incubation, the rings were transferred to a chamber filled with fresh Krebs solution and mounted in a pressure myograph (Danish Myo Technology, Aarhus, Denmark) for measurements of vessel diameter.
2.4 Pressure myography
Vessel diameters were measured with a pressure myograph as described elsewhere.16 Briefly, second-order mesenteric arteries were dissected in Krebs solution from control, DIO, TRPV4 WT, and TRPV4−/− mice and mounted on two glass micropipettes seated in a chamber (Danish Myo Technology, Aarhus, Denmark) filled with Krebs solution bubbled with 95% O2/5% CO2 and maintained at 37°C. In the experiments, the endothelium was mechanically denuded by gently rubbing the lumen with a steel wire if necessary. Successful removal of the endothelium was verified by the lack of a relaxant response to 100 nmol/L bradykinin. The cannulated artery was connected to a separate reservoir of Krebs solution with 2% bovine serum albumin (BSA). Under an inverted microscope (Zeiss Axiovert 40, Model 110 P) with a video camera, the external diameter of each artery was measured using MyoView software (version 1.1 P, 2000, Photonics Engineering, Denmark). Vessels were pre-contracted with 1 µmol/L phenylephrine (Phe) at 70 mmHg intraluminal pressure. Phe (1 mmol/L) was dissolved in deionized water and diluted in Krebs which bathing the blood vessel in the myographic chamber to obtain 1 μmol/L. After reaching a stable baseline, the flow was increased in a stepwise manner by changing the pressure of the inflow and outflow, creating a pressure difference across the blood vessel without altering the intravascular pressure. Flow-mediated vasodilation was induced at an initial shear stress of ∼10 dynes/cm2. At the end of each experiment, passive dilation was induced by changing the bathing solution to a Ca2+-free Krebs solution. HC067047 (10 µmol/L) was added to the bathing Krebs solution if necessary. Vasodilation in response to flow was calculated as a percentage of the diameter differences: (flow-induced dilation—Phe tone)/(passive dilation—Phe tone).
2.5 Ca2+ measurement
Cells were seeded on coverslips, placed in a plate flow chamber, and loaded with Fluo-4 AM (Thermo) for 15 min. Laminar shear stress was generated using the precise peristaltic pump (BT100-2J, LongerPump, UK) with a normal physiological saline solution containing (in mmol/L): 140 NaCl, 5 KCl, 1 CaCl2, 1 MgCl2, 10 glucose, 5 HEPES, and 2% BSA (pH 7.4). Live-cell images of the intracellular Ca2+ concentration ([Ca2+]i) were recorded and analysed using a Zeiss confocal microscope. [Ca2+]i changes were quantified as the ratio of real-time fluorescence (F1) relative to the intensity immediately before GSK1016790A application or flow (F0). The cells were challenged with GSK1016790A (100 nmol/L) or shear stress induced by flow at ∼5 dyne/cm2. 5–15 randomly chosen cells were evaluated in each replication.
2.6 Immunofluorescence
Briefly, cultured cells or frozen sections of mesenteric artery segments were fixed with 4% paraformaldehyde (Sigma–Aldrich), washed in PBS, permeabilized by 0.1% Triton-X-100, blocked in 2% BSA, and then incubated with rabbit anti-TRPV4 (1:500, ACC-034, Alomone) and/or mouse anti-CD144 (1:500, sc-52751, Santa Cruz) antibodies overnight at 4°C, followed by AlexaFluor 488 anti-rabbit and/or 546 anti-mouse secondary antibodies. Nuclei were stained with 4’, 6-diamidino-2-phenylindole. Samples were imaged by a Zeiss confocal microscope.
2.7 Western blot
Primary cultured MMAECs were lysed with lysis buffer (P0013C, Beyotime) supplemented with the protease inhibitor phenylmethylsulfonyl fluoride (Beyotime) and then the lysates were collected by centrifugation. Protein samples were electrophoresed on a 10% SDS-poly-acrylamide gel and transferred to an immobilon-P polyvinylidene difluoride membrane (Millipore Corp., Bedford, MA, USA). Non-specific binding was blocked with 5% BSA in 0.1% Tween-20 TBS. Blots were incubated overnight at 4°C with the primary antibodies anti-TRPV4 (1:1000, ACC-034, Alomone) and anti-GAPDH (1:5000, sc-47724, Santa Cruz) followed by horseradish peroxidase-conjugated secondary antibody (Beyotime). Anti-GAPDH was used as a housekeeping protein. The densitometric analysis was performed using Image J software. The protein expression level was normalized to GAPDH. The specificity of TRPV4 antibody was confirmed (Supplementary material online, Figure S2).
2.8 Total internal reflection fluorescence microscopy
The fluorescently labelled TRPV4 on the cell membrane was observed by total internal reflection fluorescence microscopy (TIRFM). The TIRFM setup was as described elsewhere.17 Briefly, cells were fixed in 4% formaldehyde. TRPV4 was detected with anti-TRPV4 antibody (1:200, ACC-034, Alomone). A secondary antibody was then used with Donkey anti-Rabbit IgG (H + L) highly cross-adsorbed secondary antibody, AF488 (catalog no. A21206, Thermo Fisher Scientific, Shanghai, PR China). The fluorescently labelled TRPV4 on the cell membrane was observed by TIRFM. The TIRFM setup was based on Nikon N-STORM microscope system (Tokyo, Japan), equipped with an argon (488 nm) laser line, a 60× oil-immersion objective. Images were acquired using NIS-elements. The TIRFM fluorescence density was analysed using Image J software. For data recording, each condition was replicated three times. Five cells were randomly chosen and evaluated in each replication.
2.9 Whole-cell patch clamp
MMAECs were cultured and supplemented with or without 30 μmol/L EPA and 30 μmol/L DHA overnight prior to electrophysiological measurements. Whole-cell patch clamping was conducted using an EPC10 patch clamp amplifier (HEKA, Holliston, MA, USA) as described previously.18 The pipette solution contained (in mmol/L): 140 CsCl, 5 EGTA, and 10 HEPES (pH 7.2). The bath solution contained (in mmol/L): 140 NaCl, 6 KCl, 1 MgCl2, 10 glucose, and 10 HEPES (pH 7.4). For the desensitization experiments, the bath solution contained 5 mmol/L CaCl2. The TRPV4 I–V relationship of primary mesenteric endothelial cells was obtained using a 500-ms ramp protocol from −80 mV to +80 mV from a holding potential of −60 mV. The cells were treated with GSK1016790A (100 nmol/L), HC067047 (10 μmol/L), or ruthenium-red (5 µmol/L) in the bath solution if necessary.
2.10 Atomic force microscopy
Primary isolated mouse endothelial cells were cultured and pretreated with EPA (30 μmol/L) and DHA (30 μmol/L) overnight if necessary. Atomic force microscopy (AFM) experiments were performed as previously described19 with Dimension Icon & FastScan Bio. AFM cantilevers were coated for 20 min with 0.1 mg/mL Poly-l-Lysine solution (Sigma) and then air-dried. The cantilever was positioned above the cell and tether events were yielded. 15–50 cells were analysed for each group, with ∼30 force/distance curves acquired from each cell. Tether force were determined using NanoScope Analysis software.
2.11 Statistics
All data are presented as the mean ± the standard error of the mean (SEM). Statistical analyses were performed using GraphPad Prism 6.0 software. For data that passed normality and equal variance tests, comparisons between two groups were measured by Student’s unpaired two-tailed t-test, and differences among three or more groups were assessed by one-way analysis of variance followed by Dunnett’s multiple comparison test or Tukey's multiple comparisons test. When either test failed, the Mann–Whitney U test was used when comparing between groups, and Kruskal–Wallis and Dunn’s post hoc non-parametric test was performed when comparing more than two groups. P-values <0.05 were considered to be significantly different.
3. Results
3.1 Role of diet-induced obesity in endothelial dysfunction of mouse mesenteric arteries
The endothelium-dependent flow-induced vasodilation in mesenteric arteries from DIO mice was reduced compared with those from control mice (Figure 1A and B). However, endothelium-independent flow-induced vasodilation was at a low level in both DIO and control groups (Figure 1B) suggesting the involvement of ECs. Therefore, to further determine whether DIO is associated with impairment of endothelial mechanotransduction, we tested the effect of DIO on shear stress-induced endothelial responses in MMAECs. We found that MMAECs from DIO mice showed a significantly reduced shear stress-induced [Ca2+]i rise (Figure 1C and D).
![Diet-induced obesity attenuates flow-induced vasodilation in mouse mesenteric arteries and intracellular Ca2+ concentration ([Ca2+]i) in mouse endothelial cells. (A and B) Representative trace (A) and data analysis (B) showing mesenteric artery with or without endothelial cells (ECs) dilation in response to flow challenge in control [CTL, N = 10; CTL(-ECs), N = 5] and diet-induced obese [DIO, N = 12; DIO(-ECs), N = 6] mice. Vessels were pre-constricted with phenylephrine (Phe, 1 µmol/L) to ∼60% of the pre-constriction diameter. Solid bars above traces show the period of the flow. (C and D) Representative trace (C) and data summary (D) of the flow-induced [Ca2+]i rise in primary cultured mesenteric artery endothelial cells (MMAECs) from CTL (N = 6) and DIO (N = 9) mice. (Mean ± SEM; B, *P = 0.0394 vs. CTL, **P = 0.0027 vs. CTL, NS, no significant difference vs. DIO, Kruskal–Wallis and Dunn’s post hoc test; D, ***P = 0.0005 vs. CTL, Student’s unpaired two-tailed t-test).](https://oup.silverchair-cdn.com/oup/backfile/Content_public/Journal/cardiovascres/117/12/10.1093_cvr_cvaa296/3/m_cvaa296f1.jpeg?Expires=1747881847&Signature=PKfsAKsa48gf1eEk~0yHylMAX4WVOzqiFUqENWAJEqKcT57s388Hwhkd6uyvyoOr2vtefTwpM96cLxPXWrI5t73Ko~4~n572G-Dac19s3xH~arl3qUFiOaNFAWnyviOjdNAgOeX8~FKQmB7cRoC8FMtQLXxX43mv7cKZzfzOd0pX2MP63czMLtAqlOtreB7n5WG5dB09tUepbCdNn630gaRNnjdVuUaLEmRq7jBDwjS1F~HY7QKoWLvhKlXqkm8tS3E3lvmadD74kba1mwOa7Ebn0gkG~TK7YFReliBRxAeVHTsZd-~YZeZ0OZ5h2cWyP2LYy3Au5p4xeo5e0Zy7Gw__&Key-Pair-Id=APKAIE5G5CRDK6RD3PGA)
Diet-induced obesity attenuates flow-induced vasodilation in mouse mesenteric arteries and intracellular Ca2+ concentration ([Ca2+]i) in mouse endothelial cells. (A and B) Representative trace (A) and data analysis (B) showing mesenteric artery with or without endothelial cells (ECs) dilation in response to flow challenge in control [CTL, N = 10; CTL(-ECs), N = 5] and diet-induced obese [DIO, N = 12; DIO(-ECs), N = 6] mice. Vessels were pre-constricted with phenylephrine (Phe, 1 µmol/L) to ∼60% of the pre-constriction diameter. Solid bars above traces show the period of the flow. (C and D) Representative trace (C) and data summary (D) of the flow-induced [Ca2+]i rise in primary cultured mesenteric artery endothelial cells (MMAECs) from CTL (N = 6) and DIO (N = 9) mice. (Mean ± SEM; B, *P = 0.0394 vs. CTL, **P = 0.0027 vs. CTL, NS, no significant difference vs. DIO, Kruskal–Wallis and Dunn’s post hoc test; D, ***P = 0.0005 vs. CTL, Student’s unpaired two-tailed t-test).
3.2 Omega-3 PUFAs improve flow-induced vasodilation of resistance arteries in diet-induced obese mice
Omega-3 PUFAs treatment augmented the endothelial-dependent flow-induced vasodilation in mesenteric arteries from DIO mice (Figure 2A and B). When primary cultured MMAECs from DIO mice were pre-incubated with EPA (30 µmol/L) and DHA (30 µmol/L) overnight, Ca2+ imaging showed that the [Ca2+]i rise in response to flow was also improved (Figure 2C and D). These results demonstrated that omega-3 PUFAs improve endothelial function in mesenteric arteries by regulating the flow-mediated [Ca2+]i rise in DIO mouse ECs.
![Role of omega-3 polyunsaturated fatty acids in endothelial dysfunction induced by diet-induced obesity. (A and B) Representative traces (A) and data analysis (B) showing mesenteric arterial dilation in response to flow challenge in diet-induced obese (DIO, N = 17) and EPA (30 µmol/L)/DHA (30 µmol/L)-pretreated DIO (N = 8) mice. Vessels were pre-constricted with phenylephrine (Phe, 1 µmol/L) to ∼60% of the pre-constriction diameter. (C and D) Representative trace (C) and data summary (D) of the flow-induced [Ca2+]i rise in DIO (N = 6) and EPA (30 µmol/L)/DHA (30 µmol/L)-pretreated DIO (N = 7) mouse mesenteric artery endothelial cells (MMAECs). (Mean ± SEM; B, ****P < 0.0001 vs. DIO, Mann–Whitney U test; D, ****P < 0.0001 vs. DIO, Student’s unpaired two-tailed t-test).](https://oup.silverchair-cdn.com/oup/backfile/Content_public/Journal/cardiovascres/117/12/10.1093_cvr_cvaa296/3/m_cvaa296f2.jpeg?Expires=1747881847&Signature=WkhlUhAGkiGgazoMPhmNHBUJrmttOiJxlKGU6vohXLNfVCuqkt3NVsFAP5ROUrTf335k-GjzRs6hQw9ue9DddpA0JMxqBRj8oW4KxWwgR9hCcByMaYvYCVGrMcrisCAhBCs2~qMvbbHlq5NaljCFZ0eSpC8HoHAzRYair2Oz6fjLHfxkO-urJzb0zQBEPKIaV9xBWHDg7CIPSD9T6XOtpzoDU0TfVzM9dESLJGgqgtkuTs8agBfQIeJptMbPgJf1WkV6LuxlQ8gk78F-Xiv2R-zgS-Uo49NSCWjdMCIQG6RqnLDObQ3GmQcKtAYGfKdZrO4GgQO5a34IT~GuZFaIIQ__&Key-Pair-Id=APKAIE5G5CRDK6RD3PGA)
Role of omega-3 polyunsaturated fatty acids in endothelial dysfunction induced by diet-induced obesity. (A and B) Representative traces (A) and data analysis (B) showing mesenteric arterial dilation in response to flow challenge in diet-induced obese (DIO, N = 17) and EPA (30 µmol/L)/DHA (30 µmol/L)-pretreated DIO (N = 8) mice. Vessels were pre-constricted with phenylephrine (Phe, 1 µmol/L) to ∼60% of the pre-constriction diameter. (C and D) Representative trace (C) and data summary (D) of the flow-induced [Ca2+]i rise in DIO (N = 6) and EPA (30 µmol/L)/DHA (30 µmol/L)-pretreated DIO (N = 7) mouse mesenteric artery endothelial cells (MMAECs). (Mean ± SEM; B, ****P < 0.0001 vs. DIO, Mann–Whitney U test; D, ****P < 0.0001 vs. DIO, Student’s unpaired two-tailed t-test).
3.3 Role of TRPV4 in the improvement of flow-induced vasodilation in diet-induced obese mice by PUFAs
A previous study has shown that PUFAs are required for TRPV4 activity in Caenorhabditis elegans and are associated with endothelial TRPV4 activity.12 We thus used TRPV4−/− mice and the TRPV4 pharmacological inhibitor HC067047 to study the role of TRPV4 in the vascular protective effect of PUFAs in DIO mice.
The effect on flow-induced vasodilation was investigated by treatment with the TRPV4 inhibitor HC067047 as well as in WT and TRPV4−/− mice. The results showed that flow-induced vasodilation was attenuated by the inhibitor and by knockout of TRPV4 (Figure 3A). As expected, the flow-mediated [Ca2+]i rise was also markedly decreased in mouse ECs treated with 10 µmol/L HC067047 and in TRPV4−/− mice ECs (Figure 3B). Treatment with 30 µmol/L EPA and 30 µmol/L DHA increased the vascular diameter on challenged with the flow in DIO mice (Figure 3C), and this effect was inhibited by the pharmacological inhibition of TRPV4 and in TRPV4−/− mice (Figure 3C). TRPV4−/− mice were treated with a high-fat diet for 4 weeks to establish the TRPV4−/− DIO mouse model. The lack of improvement of flow-induced vasodilation by EPA/DHA in vessels isolated from TRPV4−/− mice strongly suggests a key role of TRPV4 channels in the protective effect of PUFAs on vascular function.
![Effect of TRPV4 inhibition on flow-induced vasodilation and the endothelial cell [Ca2+]i rise. (A) Representative traces (left) and data summary (right) showing that flow-induced vasodilation is attenuated by the TRPV4 inhibitor HC067047 (10 µmol/L) and in TRPV4−/− mice. CTL, N = 7; HC067047, N = 6; TRPV4−/−, N = 11. (B) Representative traces (left) and data summary (right) showing the flow-induced [Ca2+]i rise in CTL (N = 6), HC067047 (10 µmol/L)-pretreated (N = 5) and TRPV4−/− (N = 5) mouse mesenteric artery endothelial cells (MMAECs). (C) Representative traces (left) and data summary (right) showing that flow-induced vasodilation is attenuated by HC067047 (10 µmol/L) and the knockout of TRPV4 in EPA (30 µmol/L)/DHA (30 µmol/L)-treated DIO mice. CTL, N = 5; HC067047, N = 7; TRPV4−/−, N = 9. (Mean ± SEM; A, ****P < 0.0001 vs. CTL, one-way analysis of variance test followed by Dunnett’s multiple comparisons test; B, **P = 0.0014, HC067047 vs. CTL, **P = 0.0011, TRPV4−/− vs. CTL, one-way analysis of variance test followed by Dunnett’s multiple comparisons test; C, **P = 0.0016, HC067047 vs. CTL, ***P = 0.0002, TRPV4−/− vs. CTL, one-way analysis of variance test followed by Dunnett’s multiple comparisons test).](https://oup.silverchair-cdn.com/oup/backfile/Content_public/Journal/cardiovascres/117/12/10.1093_cvr_cvaa296/3/m_cvaa296f3.jpeg?Expires=1747881847&Signature=PehY5ibhBspRUfvpn3Ah3-FPip6b1mqgc3Cg7nFM0rYXrWS6nUcuvnKUOM5qVeGN4faWl5LirJ~KuNmtOdmw-PdjTa79DbJP1ghVZXNFMsljdlEmnzAHqnP82RgVk66DgQjXDDqGFTVH2CeVaqpL7T3SDC6wmePSRKQzp88E1TRbFcCDU2RVqxLCO0dC5qh6lp4Bti-65KmBUof~7a4lfHvjMfAVQ3xAHJIMjq4kRw9BnmHLwkRYu~21xn~ytGEY8B9piKTlladj1ejNoYt1ObC6O9TAKdEn0HFEaHMcsMZbV346Tt6hX4Imr97kAtshH8DSaeghhCPLR40Y6G9PPw__&Key-Pair-Id=APKAIE5G5CRDK6RD3PGA)
Effect of TRPV4 inhibition on flow-induced vasodilation and the endothelial cell [Ca2+]i rise. (A) Representative traces (left) and data summary (right) showing that flow-induced vasodilation is attenuated by the TRPV4 inhibitor HC067047 (10 µmol/L) and in TRPV4−/− mice. CTL, N = 7; HC067047, N = 6; TRPV4−/−, N = 11. (B) Representative traces (left) and data summary (right) showing the flow-induced [Ca2+]i rise in CTL (N = 6), HC067047 (10 µmol/L)-pretreated (N = 5) and TRPV4−/− (N = 5) mouse mesenteric artery endothelial cells (MMAECs). (C) Representative traces (left) and data summary (right) showing that flow-induced vasodilation is attenuated by HC067047 (10 µmol/L) and the knockout of TRPV4 in EPA (30 µmol/L)/DHA (30 µmol/L)-treated DIO mice. CTL, N = 5; HC067047, N = 7; TRPV4−/−, N = 9. (Mean ± SEM; A, ****P < 0.0001 vs. CTL, one-way analysis of variance test followed by Dunnett’s multiple comparisons test; B, **P = 0.0014, HC067047 vs. CTL, **P = 0.0011, TRPV4−/− vs. CTL, one-way analysis of variance test followed by Dunnett’s multiple comparisons test; C, **P = 0.0016, HC067047 vs. CTL, ***P = 0.0002, TRPV4−/− vs. CTL, one-way analysis of variance test followed by Dunnett’s multiple comparisons test).
3.4 PUFAs enhance TRPV4 activity but do not affect TRPV4 expression in mouse endothelial cells
We then asked whether the important role of TRPV4 in endothelial function and its improvement by PUFAs is reflected in the increased expression of TRPV4. First, we used immunofluorescence to assess the expression and distribution of TRPV4 in mesenteric arteries in control and DIO mice. Co-staining of the endothelium marker CD144 with TRPV4 showed that TRPV4 was expressed in endothelium and its expression level did not significantly differ between the control and DIO groups (Figure 4A), and the results of western blot analysis of TRPV4 at the cellular level were the same (Figure 4B). More importantly, the treatment with EPA/DHA also did not change the total TRPV4 level in ECs (Figure 4C). TIRFM is a common imaging method for observing single molecules on the plasma membrane of a cell.20 We thus used TIRFM to determine the TRPV4 expression level on the EC plasma membrane and found that the membrane also showed similar TRPV4 expression levels in control, DIO, and PUFAs-treated DIO MMAECs (Figure 4D). This strongly suggested that TRPV4 activity is impaired in DIO mice, and the supplying PUFAs improve TRPV4 activity without affecting its expression level in ECs.
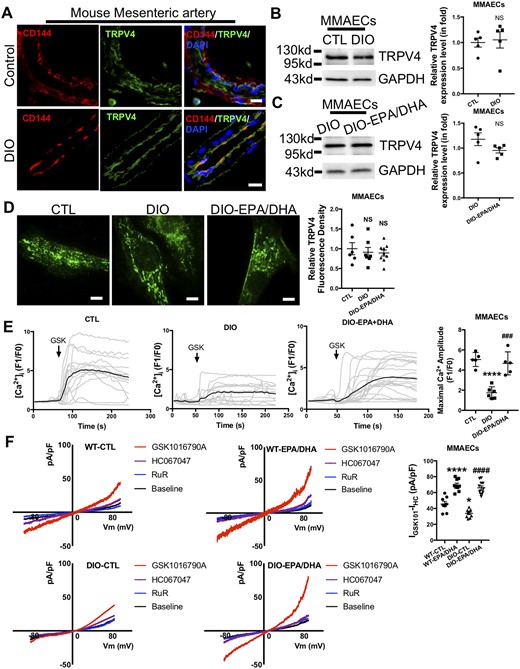
Omega-3 PUFAs enhance endothelial TRPV4 activity in diet-induced obese (DIO) mice. (A) Co-immunostaining of TRPV4 and CD144 in mouse mesenteric artery. Scale bar: 15 µm. (B and C) Western blots and analyses for anti-TRPV4 antibody in CTL, DIO (B), and DIO, EPA (30 µmol/L)/DHA (30 µmol/L)-treated DIO (C) mouse mesenteric artery endothelial cells (MMAECs). N = 5. (D) Representative images (left) and data summary (right) of TIRFM of TRPV4 in CTL (N = 6), DIO (N = 7), and EPA (30 µmol/L)/DHA (30 µmol/L)-treated DIO (N = 9) MMAECs. Scale bar: 10 µm. (E) Representative Ca2+ traces (left) and data summary (right) of CTL (N = 5), DIO (N = 6), and EPA (30 µmol/L)/DHA (30 µmol/L)-pretreated DIO (N = 5) MMAECs challenged with GSK1016790A (100 nmol/L). (F) Representative I-V curves from whole-cell patch-clamp recordings (left) and summary data (right) from WT and DIO mouse endothelial cells treated with or without EPA (30 µmol/L)/DHA (30 µmol/L) challenged with GSK1016790A (100 nmol/L), HC067047 (10 µmol/L) and ruthenium-red (5 µmol/L). WT-CTL, N = 8; WT-EPA/DHA, N = 9; DIO-CTL, N = 10; DIO-EPA/DHA, N = 11. (Mean ± SEM; B and C, NS, no significant difference vs. CTL, Student’s unpaired two-tailed t-test; D, NS, no significant difference vs. CTL, one-way analysis of variance test followed by Tukey's multiple comparisons test; E, ****P < 0.0001 vs. CTL, ###P = 0.0002 vs. DIO, one-way analysis of variance test followed by Tukey's multiple comparisons test; F, ****P < 0.0001 vs. WT-CTL, *P = 0.0127 vs. WT-CTL, ####P < 0.0001 vs. DIO-CTL, one-way analysis of variance test followed by Tukey's multiple comparisons test).
In order to investigate the activity of TRPV4 in ECs from control and DIO mice, we first assessed the response of TRPV4 to its activator GSK1016790A by measuring the fluorescence changes in MMAECs loaded with a Ca2+-sensitive dye (Fluo-4 AM) as a readout of the [Ca2+]i level. The results showed that the [Ca2+]i rise in response to GSK1016790A (100 nmol/L) was significantly attenuated in ECs from DIO mice compared to those from control mice (Figure 4E), demonstrating that TRPV4 activity and function was impaired in DIO mouse ECs. These data suggested that, in obese mice, impaired endothelial TRPV4 function contributes to the inhibited flow-induced vasodilation.
To further determine whether PUFAs supplementation affect TRPV4 activity, we assessed the mediation of the [Ca2+]i level by TRPV4 using GSK1016790A. We found that the decreased TRPV4 activity in ECs from DIO mice was restored by PUFAs supplementation (Figure 4E). Furthermore, PUFAs supplementation led to a marked increase of TRPV4 ionic currents compared to controls without supplementation when cells were challenged with GSK1016790A in both WT and DIO mice (Figure 4F). Besides, this effect did not occur in the presence of the TRPV4 antagonist HC067047 (Figure 4F). We also determined whether PUFAs supplementation affected TRPV4 desensitization, since TRPV4 channels desensitize in the presence of Ca2+ (5 mmol/L) in response to heat.21 The results showed that TRPV4 Ca2+-dependent desensitization was decreased in PUFAs-treated ECs from DIO mice compared to controls (Supplementary material online, Figure S3). These results revealed that supplying of PUFAs makes more TRPV4 channels available for activation, thus enhancing TRPV4 activity in DIO mice.
3.5 PUFAs improve membrane mechanical property of DIO mouse ECs
It has been reported that PUFAs could regulate TRPV4 activity by altering the physical properties of the cell membrane.12 We hypothesized that PUFAs may enhance TRPV4 activity by increasing cell membrane fluidity and decreasing the membrane stiffness of DIO mouse ECs. To study the underlying mechanism of PUFAs-induced TRPV4 activation in DIO mouse ECs, we firstly investigated the effect of DIO on EC membrane physical property. We performed atomic force microscopy (AFM) to detect the cell membrane tether force, allowing determination of the fluidity and stiffness of the plasma membrane. The result showed that the mean tether force of DIO MMAECs was increased compared to control ECs suggesting membrane fluidity was decreased and bending stiffness was increased under the DIO condition (Figure 5A and B). Moreover, we found that PUFAs treatment significantly attenuated tether force in DIO mouse ECs which means that the plasma membrane enriched with PUFAs show higher membrane fluidity and lower stiffness (Figure 5A and B). These results support the hypothesis that PUFAs improve the TRPV4 channel function may not through direct activation mechanism but more rely on altering cell membrane mechanics which is also mentioned in the previous study.12
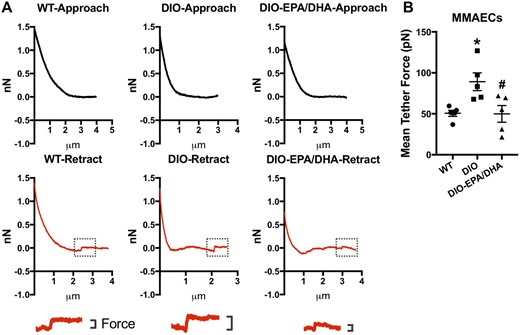
Effect of omega-3 PUFAs on endothelial cell membrane fluidity and stiffness. (A) Representative force distance traces acquired at 10 µm/s. Magnification of the force step of WT, DIO and EPA (30 µmol/L)/DHA (30 µmol/L)-treated DIO mouse mesenteric artery endothelial cells (MMAECs) were shown below. (B) Data analysis of mean tether force of WT, DIO, and EPA/DHA-treated DIO MMAECs. Mean ± SEM; *P = 0.0237 vs. WT, #P = 0.0213 vs. DIO, one-way analysis of variance test followed by Tukey's multiple comparisons test. N = 5.
4. Discussion
The present study demonstrates a key role of omega-3 PUFAs in protecting endothelial function as well as vascular function via enhancing TRPV4 activity in obese mice. The actions of PUFAs and TRPV4 were examined in isolated ex vivo mesenteric artery segments. This showed that PUFAs supplementation improved flow-induced vasodilation and the endothelial [Ca2+]i rise in obese mice, whereas the knockout or inhibition of TRPV4 weakened these protective effects. PUFAs supplementation did not alter the whole-cell or cell membrane expression level of TRPV4 in ECs, but it markedly enhanced the TRPV4 activity in ECs from obese mice. Our data are in line with previous studies showing that omega-3 PUFAs can induce vasodilation in rat aorta and mesenteric and cerebral arteries,4,6 as well as those reporting that PUFAs enhance TRPV4 activity.12 We found that shear-stress-induced vasodilation (flow-mediated vasodilation) declined after a high-fat diet, consistent with previous reports,22 and this was rescued by TRPV4 activation mediated by PUFAs supplementation.
PUFAs are known to protect endothelial function and play a role in vasodilation.23 TRPV4 has been demonstrated to regulate the endothelium-dependent relaxation associated with nitric oxide release under physiological conditions and endothelium-dependent contraction in hypertension.24,25 Whether TRPV4 activation has a relaxation or contraction effect in obesity was unclear. Moreover, it was also unclear whether a linkage exists between PUFAs and TRPV4 in regulating endothelium-dependent vasodilation in obesity. TRPV4 channels have been reported to play a protective role against obesity as well as the related hyperleptinaemia.26 TRPV4 deficiency increases the susceptibility of mice to obesity; they show more severe symptoms of obesity.27 We showed for the first time the beneficial effect of TRPV4 induced by omega-3 PUFAs supplementation which may be related to increase NO production (Supplementary material online, Figure S4) in obese mice using loss-of-function approaches. However, further studies are needed to verify the beneficial effect of omega-3-TRPV4 in clinical obese patients. Importantly, our study showed that omega-3 PUFAs did not affect TRPV4 expression in both conduit and resistance artery ECs, but enhanced the TRPV4 activity in response to GSK1016790A. This effect may rely on improved endothelial cell membrane mechanics such as increased membrane fluidity and decreased membrane stiffness. In our study, we showed that instant delivery of omega-3 PUFAs-induced rapid responses of vasodilation, Ca2+ influx, and TRPV4 currents and these effects will only last for a short period of time (Supplementary material online, Figures S5 and S6). Addition of EPA/DHA induces a slight increase in TRPV4 ionic currents which last for 2–3 min in WT mouse endothelial cells (∼30% of total GSK1016790A currents; Supplementary material online, Figure S5), while this effect was absent in TRPV4−/− mice (Supplementary material online, Figure S5). This result suggesting that PUFAs may have little effect in direct activating TRPV4 in ECs if any. All these results support the hypothesis that PUFAs improve TRPV4 channel function may not only through direct activation mechanism but more rely on altering cell membrane mechanics and our findings agree with a previous report12 and suggest that the treatment with omega-3 PUFAs increase the number of TRPV4 channels available for activation in the ECs of obese mice.
In sum, we investigated the role of omega-3 PUFAs-related TRPV4 channels in endothelial function by measuring the vasomotor responses of resistance arteries in response to flow in TRPV4−/− mice under a high-fat diet. The results demonstrated that TRPV4 activation is a protective mechanism underlying omega-3 PUFAs treatment in obesity.
Data availability
The data underlying this article are available in the article and in its online supplementary material.
Supplementary material
Supplementary material is available at Cardiovascular Research online.
Author contributions
Y.Z. and S.W. performed cell culture, WB, pressure myography, Ca2+ image, TIRFM and AFM experiments; S.W., F.Y., and L.F. contributed to animal housing. K.Z., Y.Cui and C.Z. contributed to WB and patch clamp analysis. L.W., Y.Chen and R.W. provided a critical reading of the manuscript. Y.Z. and X.M. initiated the project, designed the study, and wrote the article.
Acknowledgements
We greatly appreciate the contribution of Professor Iain C. Bruce (University of Hong Kong, China) for his support in manuscript writing. We thank School of Medicine, Zhejiang University for sharing the use of total internal reflection fluorescence microscope. We thank Instrumental Analysis Center, Shanghai Jiao Tong University for providing the use of atomic force microscope.
Conflict of interest: none declared.
Funding
This work was supported by the National Natural Science Foundation of China (81622007 and 81960662), the Chang Jiang Scholars Program (Q2015106), Fundamental Research Funds for the Central Universities (JUSRP51704A), the National First-class Discipline Program of Food Science and Technology (JUFSTR20180101), Fundamental Research Funds for Young Scholars of Jiangnan University (JUSRP12046), and the Natural Science Foundation for Young Scholars of Jiangsu Province (BK20190596).
References
Omega-3 improve the endothelial function via enhancing TRPV4 activity and augmenting the endothelial-dependent flow-induced vasodilation in DIO mice resistance arteries. This study provides a strategy of improving vascular function under obesity, namely, by targeting TRPV4 via the usage of omega-3.
Author notes
The first three authors contributed equally to the study.