-
PDF
- Split View
-
Views
-
Cite
Cite
Lijun You, Yufeng Gong, Laihao Li, Xiao Hu, Charles Brennan, Viktoryia Kulikouskaya, Beneficial effects of three brown seaweed polysaccharides on gut microbiota and their structural characteristics: An overview, International Journal of Food Science and Technology, Volume 55, Issue 3, March 2020, Pages 1199–1206, https://doi.org/10.1111/ijfs.14408
- Share Icon Share
Abstract
The gut microbiota appears critical in the metabolic health and anti-disease activity. In this review, we discuss three brown seaweed polysaccharides (alginate, laminarin, fucoidan) for their structural information, the digestive behaviour and the effects on gut microbiota. Bioactivities are associated with various physicochemical properties, like solubility, viscosity, hydration properties, molecular weight, monosaccharide composition, and so on. Brown seaweed polysaccharides can be completely utilised by microbes in the large bowel, and they can regulate the gut microbiota. The ultimate metabolite of these polysaccharides is mainly short-chain fatty acids, which are able to regulate the ecology of the gut microbiota by increasing the growth of beneficial bacteria and inhibiting the growth of some harmful bacteria. In addition, this article also discusses the relationship between the structure and activity of modulating the gut microbiota. Results show that polysaccharides with low molecular weight are more conductive to modulate gut microbiota.
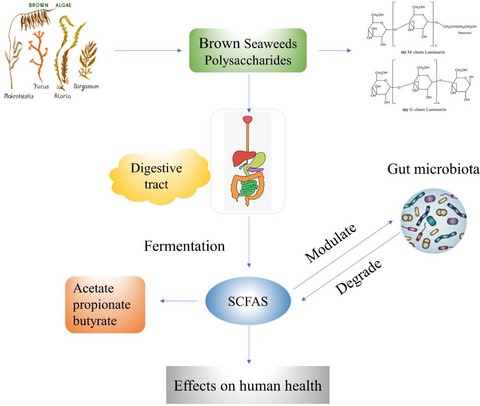
Introduction
The human gut microbiota consists of trillions of microorganisms as a hidden metabolism organ (Nicholson et al., 2012). Growing evidence indicates that gut microbiota appears critical in maintaining intestinal homeostasis and improving metabolism health. It is well known that intestinal flora dysbiosis is closely related to the development of various chronic diseases (Hand et al., 2016), such as metabolic syndrome (Chassaing et al., 2015), colorectal cancer (Wang et al., 2012), angiocardiopathy (Tang & Hazen, 2014), type II diabetes (Li et al., 2018a), adiposis (Chang et al., 2015), inflammatory bowel disease (Tao et al., 2017) and cancer (Ohtani et al., 2014). The occurrence of these diseases may be due to the damage of intestinal mucosal and decrease of bacteria abundance. The gut microbiota can maintain the health of the host by regulating development and function of metabolism, epithelial barrier integrity and immune system (Artis 2008; Bäckhed et al., 2005). Bifidobacteria is one key genus in the gut microbiota of mammals. In a highly competitive environment, bifidobacteria adopts various strategies to survive in the intestinal environment of mammals, including absorption of glycan, decomposition of glycan and cross-feeding. Additionally, the conditions can lead to some changes in microbial composition and microbial metabolism, such as short-chain fatty acid production and carbohydrate utilisation (Turroni et al., 2016). Recently, with the development of low-cost genomic sequencing technology, there has been a deeper and clearer understanding of gut microbiota. Gut microbiota may have the potential to be a drug target for both precaution and treatment of some diseases.
Seaweed is a significant component of marine biological resource and the most basic producer in the ocean. People divide marine algae into three main categories at phylum level on the basis of their natural pigment: the brown seaweeds Phaeophyta, red seaweeds Rhodophyta and green seaweeds Chlorophyta. Among them, brown seaweeds are the richest and have a relatively high diversity (Ngo & Kim, 2013). Polysaccharide accounts for more than 76% of the dry mass. Seaweeds also include some bioactive substances: polyphenol, proteins, polyunsaturated fatty acids (PUFAs), bioactive peptides (Holdt & Kraan 2011).
Polysaccharides, for example, agars, laminarin, fucoidan and alginate, most of them can reach the large bowel to exert the beneficial effects on gut microbiota (Gupta & Abughannam 2011; O'Sullivan et al., 2010). Among the seaweeds species, brown seaweed polysaccharides (BSP) have attracted a lot of attention and have been studied frequently, because they are proved to be easily acquired and grown in a large scale, with abundant polysaccharides (Charoensiddhi et al., 2016). The complex structure of BSP has been studied, due to its various bioactivities, such as anti-infection, anti-ageing, anti-inflammatory, antioxidant, anti-obesity and immunomodulatory properties (Wang et al., 2017). Brown seaweed polysaccharides may exert prebiotic activity, serving as dietary fibres regulating gut microbiota by stimulating the growth of beneficial bacteria and inhibiting the growth of harmful bacteria (Windey et al., 2012). They are difficult to be degraded by enzymes in the human body, almost remain unchanged in the mouth, stomach, small intestine without being hydrolysed. Polysaccharides would safely reach the large bowel to exert the beneficial bioactivity impacts on the gut function and microbiota (Cardona et al., 2013). In this review, we discuss the relationship between the structure and bioactivities of brown seaweed polysaccharides, the digestion behaviour and the effects on the gut microbiota.
The brown seaweed polysaccharides
The high complexity of polysaccharides arises from the stereochemical diversity of their constitutive monosaccharide residues and the various ways to connect these residues through glucosidal linkages (Mathieu et al., 2018). Alginate, laminarin and fucoidan are three main types of structural polysaccharides, usually applied in food and nutrition products as functional components (O'Sullivan et al., 2010).
Alginate
Alginate is a class of unbranched cell-wall polysaccharides in some bacteria, consisting of two uronic acids connected in a linear form, 1,4-β-D-mannuronic acid (M) and α-L-guluronic acid (G). It comprises of three residues sequences: M-blocks, G-blocks and alternating MG-blocks (Draget & Taylor, 2011; Kabisch et al., 2014). The building units (sugars) are illustrated in Fig. 1, where (a) presents β-D-mannuronic acid and (b) shows α-L-guluronic acid (Davis et al., 2003).
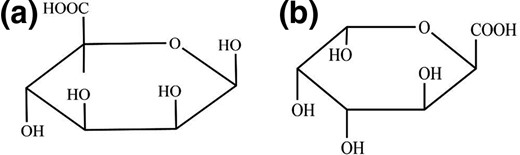
Constituent acids of alginic acid, where (a) is β-D-mannuronic acid and (b) is α-L-guluronic acid.
The current research on alginate about gut microbiota mainly focused on inhibiting the production of putrefactive substances present in the gut (An et al., 2013; Nakata et al., 2016). Bioactivities of alginates are mainly related to their arrangement of monomeric structure (Draget & Taylor, 2011). It has been reported that polysaccharides from Grateloupia filicina and Eucheuma spinosum have been shown to significantly promote the proliferation of bifidobacterium, with the highest proliferation rates of beneficial bacteria at 0.1% polysaccharides from E. spinosum and 0.4% polysaccharides from G. filicina (Chen et al., 2018b).
Laminarin
Laminarin is basically a class of low-molecular-weight polysaccharides, which is lower than polysaccharides prepared from other seaweeds. It contains β-glucans, which are in the form of β-(1,3) link accompanying β-(1,6)-linked side chains of different distributions and lengths (approximately 20–25 glucose moieties; Walsh et al., 2013). The two kinds of basic structures of laminarin (M and G chain) are presented in Fig. 2, which are classified in the reducing end (Okolie et al., 2017). Laminarin is located in cell vacuoles, found in the fronds of Laminaria and Saccharina species (Kadam et al., 2015a; Kadam et al., 2015b). Studies on laminarin have shown prospects due to various bioactivities, including alleviating metabolic syndrome (Duan et al., 2019), antioxidant (Choi et al., 2012), immunomodulating, anti-obesity (Yang et al., 2017) and antibacterial activities (Kadam et al., 2015a).
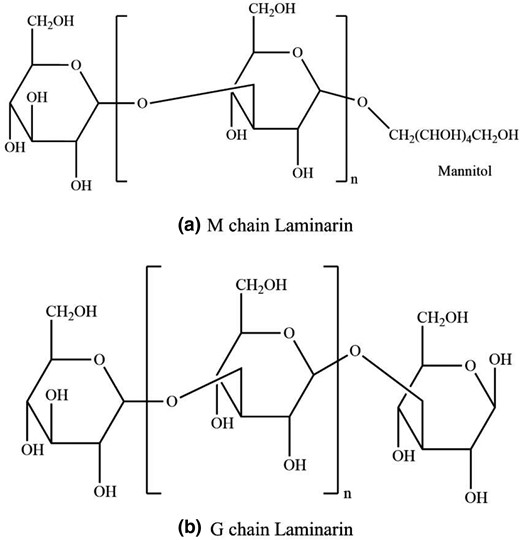
The two kinds of basic structures of laminarin (M and G chain).
There is a potential link between obesity and gut microbiota. Studies have found that obese individuals and models show gut microbiota dysbiosis, including the decreased ratio of Bacteroides and Firmicutes (Krznaric et al., 2012). There are also some differences in functional genes and metabolic activities between obese and thin individuals (Gerard, 2016). Studies have shown that laminarin can change the composition of gut microbiota in mice fed with high-fat diet, with a notable increased ratio of Bacteroides and Firmicutes (Nguyen et al., 2016). There was a lower level of carbohydrate active enzymes secreted from many of the Bacteroides species in the mice, although there was a demonstrable increase in glycoside hydrolase and polysaccharide lyases with laminarin supplementation (Flint et al., 2012). Polysaccharides could reduce obesity in obese models not only by modulating gut microbiota, but also by repairing intestinal barrier and reducing inflammations (Chang et al., 2015; Li et al., 2018b). An et al. (2013) observed that the abundance of bacterial was increased with laminarin supplementation. There were primarily two bacteria, including Clostridium spp. and P. distasonis, detected in rats fed with alginate and laminarin. These results revealed that alginate and laminarin can create a healthy intestinal environment by altering the composition of gut microbiota.
Fucoidan
Fucoidan is a kind of polysaccharide with more sulphates, primarily exited in edible brown algae. Natural fucoidan has two types of chains, type I chain consists of α- (1 → 3)-linked fucose and type II chain consists of altering α-(1 → 3) and α (1 → 4)-linked fucose (Cumashi et al., 2007). Molecular weight and sulphate content of fucoidan from Ascophyllum nodosum with type II chain were 1330 kDa and 21.0%, but MW and sulphate content of fucoidan from Laminaria japonica with type I chain were merely 310 kDa and 18.4% (Shang et al., 2017), respectively. The differences in the position of sulphate group of fucoidan with classical structures are shown in Fig. 3 (Ale et al., 2011). They possessed diverse biological activities, including anti-inflammatory (Park et al., 2011), anticoagulant (Menshova et al., 2016), anti-virus (Senthilkumara et al., 2013), immunomodulating (Kim & Joo 2008), anti-cancer (Vishchuk et al., 2016) and anti-oxidative activities (Koh et al., 2019).
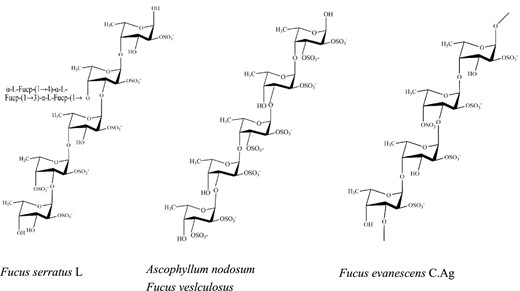
Classical structures of fucoidan showing differences in the position of sulfate group.
Changes in the interactions between the gut microbiota, intestinal epithelial cells and host immune system are related to many diseases, including cancer (Zitvogel et al., 2015). It has been studied that fucoidan could prevent breast cancer by increasing the ratio of Bacteroides and Firmicutes, and repairing the intestinal barrier by intensifying the expression of the tight junction proteins via increasing the phosphorylation of p38 MAPK and ERK1/2 (Xue et al., 2018). Reports have also indicated that fucoidan from Undaria pinnatifida alleviated dyslipidemia and modulated gut microbiota in mice with high-fat diet, by increasing the abundance of Bacteroidetes and Alloprevotella, conversely decreasing the abundance of Firmicutes, Staphylococcus and Streptococcus (Liu et al., 2018). Interestingly, dietary fucoidan was also observed to increase the concentration of short-chain fatty acids. Fucoidan has been used as commercial prebiotics in Russia (Zaporozhets et al., 2014), due to the ability of increasing the abundance of B. longum B379M and B. bifidum 791B, while other fucoidan do not have such benefits to modulate gut microbiota. For example, Shang et al. (2016) found that fucoidan from Ascophyllum nodosum had the potential to stimulate the activity of Lactobacillus spp. in the intestine, while fucoidan from Laminaria japonica had no similar effects. Therefore, more caution should be paid to the chemistry structure of fucoidan when studying its effects on the gut microbiota.
Physicochemical properties of BSP
Corresponding physicochemical properties of BSP are helpful to understand the digestion behaviour and the mechanism of bioactivities. They are mainly including solubility, viscosity, hydration properties, molecular weight, monosaccharide composition, and so on (Tungland & Meyer, 2002). Most of polysaccharides are water soluble, due to abundant hydroxyl (-OH) groups (O'Sullivan et al., 2010). Viscosity is usually known as the resistance to flow, which is related to molecular weight and concentration of polysaccharides (Capuano, 2017). Hydration properties partly influence polysaccharides' fermentability in the digestive tract (Tungland & Meyer, 2002). Different physicochemical properties influence the digestion and the colonic fermentation. Main bioactivities are from the fermentation of gut microbiota. Therefore, physicochemical properties are associated with various bioactivities (Guillon & Champ, 2000). For example, many studies found that the anticoagulant activity of fucoidan might be associated with the content and position of sulphate, molecular weight and monosaccharide composition (Li et al., 2008). Fucoidan and laminarin are usually used as functional ingredients (Kadam et al., 2015a). Alginate has excellent solubility, ionic crosslinking and alginic acid gels in physical properties, extensively used as thickeners, stabilisers, gel formers and film formers in the food and pharmaceutical industries (Szekalska et al., 2016). But we also need a further understanding about the relationships between structures and bioactivities, and the mechanism of modulating effect on gut microbiota.
The digestive behaviour of BSP
The digestive tract is a place where nutrients can be absorbed from food, especially the large bowel. Recently, our knowledge about the behaviour of polysaccharides in the digestive tract has increased, due to the development of digestion model in vitro (Capuano, 2017). There are mainly discussions about changes of the molecular weight and free monosaccharides of polysaccharides in the simulating digestive system in vitro.
The digestive tract mainly includes oral cavity, oesophagus, stomach, intestine and large bowel. A great deal of evidence has demonstrated that natural polysaccharides can reach the large bowel safely without being hydrolysed by human enzymes in the saliva, stomach and small intestine (Flint et al., 2012; Liu et al., 2018). The molecular weight and free monosaccharides of BSP did not change in the saliva, stomach and small intestine, when polysaccharides were digested in vitro. However, the fermentation of gut microbiota leads to a significant change in the molecular weight and reducing sugar content. In addition, the pH and the contents of SCFAs were found to have some significant changes in the fermentation of human faecal samples. (Kong et al., 2016; Chen et al., 2018a). There are similar results in the simulated digestion of other polysaccharides in vitro (Gao et al., 2018; Hu et al., 2018; Xu et al., 2019). Little is known about the specific mechanism of degrading complex seaweed polysaccharides (EI et al., 2013). It is still worthy of a further research on the digestive behaviour of BSP.
The effects on the gut microbiota of BSP
The effects on the gut microbiota are generally evaluated by the contents of SCFAs, the composition and abundance of beneficial intestinal bacteria (Flint et al., 2008).
SCFAs production
The main product of polysaccharides fermented by intestinal bacterial is short-chain fatty acids, including acetate, propionate and butyrate acids (Flint et al., 2008). They are rapidly absorbed and metabolised in the host (Yang et al., 2013), principal energy sources for host cells to synthesise lipids and glucose (Wolever et al., 1989). Studies have indicated that SCFAs might play a critical role in the improvement of metabolic syndrome, bowel disorders and cancer (Harig et al., 1989; Donohoe et al., 2011; Fukuda et al., 2011). Acetate acid in oral administration can ameliorate glucose tolerance and inhibit obesity. It is a critical source for cholesterol synthesis (Yamashita et al., 2007). Propionic acid is the main metabolite of Bacteroides, Phascolarctobacterium, Dialister, Veillonella, which can regulate insulin and blood sugar level to protect against diet-induced obesity (Gao et al., 2009). The succinate pathway is the most common synthetic pathway of propionate, firstly generating oxaloacetate from pyruvate, converted into succinate, succinyl-CoA, propionyl-CoA and eventually changing into propionate (Louis et al., 2014; Reichardt et al., 2014). Butyrate acid is the main metabolite of Firmicutes, regulating the gut hormones and improving gut inflammation (Lin et al., 2012).
On the other hand, SCFAs arising from metabolism of polysaccharides can stimulate the growth of beneficial bacteria and suppress the growth of harmful bacteria. SCFAs have the potential to stimulate the intestinal epithelial cells, which can synthesise mucin and antimicrobial substance (Correa et al., 2016; Kamada et al., 2013). Brown seaweed polysaccharides can be fermented by microorganism (Lactobacillus, Bifidobacterium, Roseburia, Faecalibacterium, Anaerostipes, Coprococcus, etc.) in the large bowel to generate metabolites (SCFAs) to stimulate relational gene expression (Fernández et al., 2016). SCFAs have the potential to be therapeutic targets for obesity and type II diabetes. They play a significant part in maintaining the host health and preventing diseases, as primary products in the fermentation of brown seaweed polysaccharides.
Stimulation the activity/growth of gut microbiota
Prebiotic activity can be shown by stimulating the activity or increasing the abundance of beneficial bacteria. The differences in the composition of gut microbiota could result from differences in diet (Albenberg & Wu 2014). Brown seaweed polysaccharides have the potential to change gut microbiota composition, which could be detected by bacterial 16S ribosomal RNA (16S rRNA) sequencing by faeces fermenting (An et al., 2013). Faeces are usually used to evaluate most of bacterial species by simulating colon experimental models in vitro (Roberfroid, 2007). Table 1 illustrates the source of brown seaweed polysaccharides, research methods and evidence of beneficial effects on gut microbiota.
The source of brown seaweed polysaccharides, research methods and evidence of beneficial effects on gut microbiota
BSP . | Source . | Research methods . | Evidence of beneficial effects on gut microbiota . | References . |
---|---|---|---|---|
Alginate | Gracilaria spp., Gelidium sesquipedale and Ascophyllum nodosum | In vitro | Stimulating the growth of Bifidobacterium spp. and Lactobacillus spp. in the gut | Ramnani et al. (2012) |
Alginate | Grateloupia filicina and Eucheuma spinosum | In vitro | Promoting proliferation of bifidobacterium | Chen et al. (2018b) |
Laminarin | Tokyo Kasei, Tokyo, Japan | In vivo | Stimulating the growth of Clostridium spp. and P. distasonis | An et al. (2013) |
Laminarin | Sigma | In vivo | A significant decrease in Firmicutes and an increase in the Bacteroidetes phylum | Nguyen et al. (2016) |
Fucoidan | Fucus evanescens | In vivo | Stimulating the growth of B. longum B379M and B. bifidum 791B | Zaporozhets et al. (2014) |
Fucoidan | Ascophyllum nodosum and Laminaria japonica | In vivo | Increased Akkermansia population and Alloprevotella, Blautia and Bacteroides which are SCFAs producers. | Shang et al. (2017) |
Fucoidan | Ascophyllum nodosum and Laminaria japonica | In vivo | Increased the abundance of Lactobacillus and Ruminococcaceae, decreased the pathogenic bacteria—Peptococcus. | Shang et al. (2016) |
Fucoidan | Undaria pinnatifida | In vivo | Promoted the abundance of Bacteroidetes and Alloprevotella, suppressed the richness of Firmicutes, Staphylococcus and Streptococcus | Liu et al. (2018) |
Brown seaweed polysaccharides | Ecklonia radiata | In vitro | Promoted the growth of beneficial bacteria population (Bifidobacteria and Lactobacilli) as well as SCFAs production | Charoensiddhi et al. (2016) |
Polysaccharide-rich extracts | Laminaria digitata | In vitro | Stimulating the growth of Parabacteroides as well as SCFAs production | Strain et al. (2019) |
BSP . | Source . | Research methods . | Evidence of beneficial effects on gut microbiota . | References . |
---|---|---|---|---|
Alginate | Gracilaria spp., Gelidium sesquipedale and Ascophyllum nodosum | In vitro | Stimulating the growth of Bifidobacterium spp. and Lactobacillus spp. in the gut | Ramnani et al. (2012) |
Alginate | Grateloupia filicina and Eucheuma spinosum | In vitro | Promoting proliferation of bifidobacterium | Chen et al. (2018b) |
Laminarin | Tokyo Kasei, Tokyo, Japan | In vivo | Stimulating the growth of Clostridium spp. and P. distasonis | An et al. (2013) |
Laminarin | Sigma | In vivo | A significant decrease in Firmicutes and an increase in the Bacteroidetes phylum | Nguyen et al. (2016) |
Fucoidan | Fucus evanescens | In vivo | Stimulating the growth of B. longum B379M and B. bifidum 791B | Zaporozhets et al. (2014) |
Fucoidan | Ascophyllum nodosum and Laminaria japonica | In vivo | Increased Akkermansia population and Alloprevotella, Blautia and Bacteroides which are SCFAs producers. | Shang et al. (2017) |
Fucoidan | Ascophyllum nodosum and Laminaria japonica | In vivo | Increased the abundance of Lactobacillus and Ruminococcaceae, decreased the pathogenic bacteria—Peptococcus. | Shang et al. (2016) |
Fucoidan | Undaria pinnatifida | In vivo | Promoted the abundance of Bacteroidetes and Alloprevotella, suppressed the richness of Firmicutes, Staphylococcus and Streptococcus | Liu et al. (2018) |
Brown seaweed polysaccharides | Ecklonia radiata | In vitro | Promoted the growth of beneficial bacteria population (Bifidobacteria and Lactobacilli) as well as SCFAs production | Charoensiddhi et al. (2016) |
Polysaccharide-rich extracts | Laminaria digitata | In vitro | Stimulating the growth of Parabacteroides as well as SCFAs production | Strain et al. (2019) |
The source of brown seaweed polysaccharides, research methods and evidence of beneficial effects on gut microbiota
BSP . | Source . | Research methods . | Evidence of beneficial effects on gut microbiota . | References . |
---|---|---|---|---|
Alginate | Gracilaria spp., Gelidium sesquipedale and Ascophyllum nodosum | In vitro | Stimulating the growth of Bifidobacterium spp. and Lactobacillus spp. in the gut | Ramnani et al. (2012) |
Alginate | Grateloupia filicina and Eucheuma spinosum | In vitro | Promoting proliferation of bifidobacterium | Chen et al. (2018b) |
Laminarin | Tokyo Kasei, Tokyo, Japan | In vivo | Stimulating the growth of Clostridium spp. and P. distasonis | An et al. (2013) |
Laminarin | Sigma | In vivo | A significant decrease in Firmicutes and an increase in the Bacteroidetes phylum | Nguyen et al. (2016) |
Fucoidan | Fucus evanescens | In vivo | Stimulating the growth of B. longum B379M and B. bifidum 791B | Zaporozhets et al. (2014) |
Fucoidan | Ascophyllum nodosum and Laminaria japonica | In vivo | Increased Akkermansia population and Alloprevotella, Blautia and Bacteroides which are SCFAs producers. | Shang et al. (2017) |
Fucoidan | Ascophyllum nodosum and Laminaria japonica | In vivo | Increased the abundance of Lactobacillus and Ruminococcaceae, decreased the pathogenic bacteria—Peptococcus. | Shang et al. (2016) |
Fucoidan | Undaria pinnatifida | In vivo | Promoted the abundance of Bacteroidetes and Alloprevotella, suppressed the richness of Firmicutes, Staphylococcus and Streptococcus | Liu et al. (2018) |
Brown seaweed polysaccharides | Ecklonia radiata | In vitro | Promoted the growth of beneficial bacteria population (Bifidobacteria and Lactobacilli) as well as SCFAs production | Charoensiddhi et al. (2016) |
Polysaccharide-rich extracts | Laminaria digitata | In vitro | Stimulating the growth of Parabacteroides as well as SCFAs production | Strain et al. (2019) |
BSP . | Source . | Research methods . | Evidence of beneficial effects on gut microbiota . | References . |
---|---|---|---|---|
Alginate | Gracilaria spp., Gelidium sesquipedale and Ascophyllum nodosum | In vitro | Stimulating the growth of Bifidobacterium spp. and Lactobacillus spp. in the gut | Ramnani et al. (2012) |
Alginate | Grateloupia filicina and Eucheuma spinosum | In vitro | Promoting proliferation of bifidobacterium | Chen et al. (2018b) |
Laminarin | Tokyo Kasei, Tokyo, Japan | In vivo | Stimulating the growth of Clostridium spp. and P. distasonis | An et al. (2013) |
Laminarin | Sigma | In vivo | A significant decrease in Firmicutes and an increase in the Bacteroidetes phylum | Nguyen et al. (2016) |
Fucoidan | Fucus evanescens | In vivo | Stimulating the growth of B. longum B379M and B. bifidum 791B | Zaporozhets et al. (2014) |
Fucoidan | Ascophyllum nodosum and Laminaria japonica | In vivo | Increased Akkermansia population and Alloprevotella, Blautia and Bacteroides which are SCFAs producers. | Shang et al. (2017) |
Fucoidan | Ascophyllum nodosum and Laminaria japonica | In vivo | Increased the abundance of Lactobacillus and Ruminococcaceae, decreased the pathogenic bacteria—Peptococcus. | Shang et al. (2016) |
Fucoidan | Undaria pinnatifida | In vivo | Promoted the abundance of Bacteroidetes and Alloprevotella, suppressed the richness of Firmicutes, Staphylococcus and Streptococcus | Liu et al. (2018) |
Brown seaweed polysaccharides | Ecklonia radiata | In vitro | Promoted the growth of beneficial bacteria population (Bifidobacteria and Lactobacilli) as well as SCFAs production | Charoensiddhi et al. (2016) |
Polysaccharide-rich extracts | Laminaria digitata | In vitro | Stimulating the growth of Parabacteroides as well as SCFAs production | Strain et al. (2019) |
It has been reported that more than 90% of colonic microbiota were two phyla of Firmicutes and Bacteroidetes (Faith et al., 2013). Shang et al. (2016) found that polysaccharide from Enteromorpha Clathrata could modulate gut microbiota, with a significant increase in the growth of beneficial bacteria (Bacteroides, B. thetaiotaomicron, B. distasonis and B. fragilis) and a significant decrease in the growth of harmful bacteria (Firmicutes, Alloprevotella and Blautia). Bacteroidetes and Firmicutes also have a beneficial impact on gut health (Mariat et al., 2009). Bifidobacterium, Anaerostipes and Lactobacillus are also typical health-promoting gut microbiota (Fernández et al., 2016). Currently, there has been a novel-generation probiotic bacterium, Akkermansia muciniphila, related to obesity and metabolic syndrome (Sheng et al., 2018). Microbiota composition in high-fat diet mice supplemented with dietary fucoidan from Ascophyllum nodosum and Laminaria japonica, showed an increase in the relative abundance of Akkermansia muciniphila, Alloprevotella, Blautia and Bacteroides (short-chain fatty acid producers; Shang et al., 2016; Shang et al., 2017). Polysaccharides from Enteromorpha clathrate could modulate gut microbiota by increasing the abundances of Bifidobacterium spp. and Akkermansia muciniphila in female mice. Thus, brown seaweed polysaccharides have potential prebiotic activity by changing the composition and increasing the abundance of gut microbiota.
Conclusion
This review has highlighted the relationship between structure and bioactivities of three brown seaweed polysaccharides (alginate, laminarin, fucoidan), the digestive behaviour and evidence of modulating the gut microbiota. Various physicochemical properties of BSP have an impact on the fermentability. Brown seaweed polysaccharides can reach large bowel safely without being hydrolysed by human enzymes in the saliva, stomach and small intestine. Brown seaweed polysaccharides play a critical role in modulating gut microbiota by producing SCFAs which can maintain the host health and prevent colonic diseases. They could stimulate the activity and growth of beneficial gut bacterial and change the composition of gut microbiota. Recently, the bioactivities of polysaccharides and the bacteria abundance have gradually been a hot research. However, there are still many other microbes that could degrade polysaccharides in the human intestine, which remains unclear. The mechanism of prebiotic activities is still not clear currently. Therefore, brown seaweed polysaccharides will be potential to be a research object with promising prospects.
Acknowledgments
The work was funded by Fund of Key Laboratory of Aquatic Product Processing, Ministry of Agriculture and Rural Affairs, China (NYJG201902), National Natural Science Foundation of China (31972011), Guangzhou Science and Technology Program (201907010035), Guangdong Special Support Program (2015TQ01N670), Pearl River S&T Nova Program of Guangzhou (201610010096), the Group Program of Natural Science Foundation of Guangdong Province (2016A030312001), the 111 project (B17018) and Fundamental Research Funds for the Central Universities (2019MS101).
Disclosure statement
The authors do not know any affiliations, memberships, funding, or financial holdings that might be thought as influencing the objectivity of this review.
Data availability statement
Figure 1 (Davis et al., 2003). The data that support the findings of this study are openly available in Web of Science at https://doi.org/10.1016/S0043-1354(03)00293-8 (Chen et al., 2018b). The data that support the findings of this study are openly available in Web of Science at https://doi.org/10.1016/j.ijbiomac.2018.01.183.
Figure 2 (Okolie et al., 2017). The data that support the findings of this study are openly available in Web of Science at https://doi.org/10.1111/jfbc.12392 (Shang et al., 2017). The data that support the findings of this study are openly available in Web of Science at https://doi.org/10.1016/j.jff.2016.11.002.
Figure 3 (Ale et al., 2011). The data that support the findings of this study are openly available in Web of Science at https://doi.org/10.3390/md9102106.
Ethical guidelines
Ethics approval was not required for this research.