-
PDF
- Split View
-
Views
-
Cite
Cite
Raúl E Cian, Pablo R Salgado, Adriana N Mauri, Silvina R Drago, Pyropia columbina phycocolloids as microencapsulating material improve bioaccessibility of brewers’ spent grain peptides with ACE-I inhibitory activity, International Journal of Food Science and Technology, Volume 55, Issue 3, March 2020, Pages 1311–1317, https://doi.org/10.1111/ijfs.14397
- Share Icon Share
Abstract
The effects of wall hydrocolloids on delivery and bioaccessibility of encapsulated brewers’ spent grain ACE-I inhibitory peptides after simulated gastrointestinal digestion were assessed. Microencapsulation of peptides was carried out by spray drying using locust bean gum, P. columbina phycocolloids, or its mixtures as wall materials. Microcapsules presented round external surfaces with some concavities, negative surface charge and encapsulation efficiencies higher than 90%. The incorporation of phycocolloids to formulations increased the encapsulation efficiency, negative surface charge and resistance against digestive enzymes of microcapsules. Encapsulated peptides with P. columbina phycocolloids showed lower IC50 value of ACE-I inhibition than un-encapsulated peptides (2.4 ± 0.2 vs. 7.2 ± 0.3 mg mL−1 protein), but higher than that obtained for hydrolysate (1.5 ± 0.2 mg mL−1 protein), indicating a 75% protection of bioactivity. Electrostatic and hydrophobic interactions between P. columbina phycocolloids and brewers’ spent grain peptides could be implicated in the protection of peptides during gastrointestinal digestion.
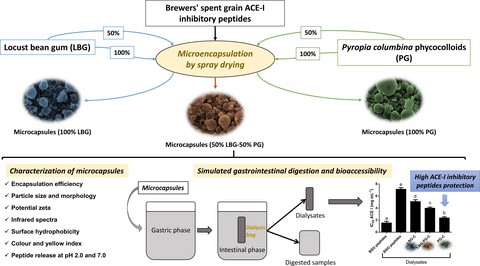
Introduction
Brewers’ spent grain (BSG) is a byproduct of the brewing process. It has been used to obtain different bioactive compounds (Lynch et al., 2016) including peptides, which have been shown to exert different in vitro bio-functional properties such as: antioxidant, anti-microbial, anti-inflammatory, hypoglycaemic (α-glucosidase, α-amylase and dipeptidyl peptidase-IV inhibitory activities), antithrombotic and angiotensin converting enzyme I (ACE-I) inhibitory activities (Connolly et al., 2015, 2017; Cian et al., 2018). On the other hand, bioactive peptides can be inactivated by proteolytic enzymes and extremely low pH in the gastrointestinal tract when they are administered orally (Wang et al., 2015). Cian et al. (2018) reported that in vitro gastrointestinal digestion degrade ACE-I inhibitory peptides from BSG hydrolysates, IC50 value being increased. In this regard, several studies have demonstrated that most bioactive peptides containing more than 2–3 amino acid residues do not withstand simulated gastrointestinal digestion (Mohan et al., 2015), losing their bio-functional properties (De Koker et al., 2011). An alternative to protect them inside the gastrointestinal tract is by spray drying microencapsulation using different biopolymers (Cian et al., 2019). This technology produces a continuous matrix containing the active substances (Nesterenko et al., 2013), enhancing the bioaccessibility of food-derived bioactive peptides.
Maltodextrin, starch, gum arabic, pectin and chitosan are the polysaccharides most studied as encapsulating agents and delivery systems, due to their abundance in nature and structural stability (Fathi et al., 2014). Recently, the encapsulation of peptides obtained from different protein sources with maltodextrin and gum arabic blend by spray drying has been reported (Yang et al., 2012; Rao et al., 2016; Cian et al., 2019). Even though carrageenans and sulphated polysaccharide extracted from red algae are extensively used in the food industry as gelling agent, stabilizers and texture enhancers (Cian et al., 2014), they have not been studied as encapsulating agents (Papoutsis et al., 2018). Locust bean gum (LBG) has not been used to encapsulate peptides by spray drying, although it has been used in drug delivery systems (Prajapatia et al., 2013). The mixture of coating agents might be more efficient than the individual compounds for the encapsulation of bioactive compounds (Papoutsis et al., 2018) if they interact in a synergistic way. In this regard, the blend of polysaccharides such as LBG and κ-carrageenan has improved the physicochemical properties of edible films and gels (Martins et al., 2012), demonstrating a synergistic effect between both polysaccharides (Arda et al., 2009). However, little attention has been paid on the properties related to these biopolymers when used as encapsulating material for bioactive peptides in spray drying process. Moreover, as far as we know, there is no research about the effects of Pyropia columbina phycocolloids (carrageenan and sulphated polysaccharide mixture) and LBG on the protection of encapsulated brewers’ spent grain bioactive peptides and delivery after in vitro gastrointestinal digestion. Therefore, the aim of this work was to assess the effects of wall hydrocolloids on delivery and in vitro bioaccessibility of encapsulated brewers’ spent grain ACE-I inhibitory peptides after simulated gastrointestinal digestion.
Materials and methods
This section is in the additional supporting information.
Results and discussion
Characterisation of microcapsules
Encapsulation efficiency of microcapsules was higher than 90% (Table S1). Although it was suggested that encapsulation efficiency of over 50% increases the risk of leakage, lower values would lead to inefficient use of the bioactive materials and imply that higher amount of encapsulated products would be required to attain the peptide quantities needed to exert physiological bioactivities (Mohan et al., 2015). On the other hand, the addition of phycocolloids from red seaweed P. columbina to microcapsule formulation increased the encapsulation efficiency (P < 0.05). This effect could be due to the good film forming properties of carrageenan and agar provided by this red seaweed (Cian et al., 2014).
Surface morphology of microcapsules is shown in Figure S1a. All microcapsules presented smooth round external surfaces and some surface dents (indentations), which are characteristics of atomised products (Mohan et al., 2015). Such dents/indentations are formed with slow processes of film formation during drying of the atomised droplets, being these surface depressions the consequence of droplets collapse during the early stages of drying process (Ré, 1998). Rocha et al. (2009) observed similar morphology for casein hydrolysate encapsulated with maltodextrin by spray drying.
Particle size of microcapsules determinates the dispersibility and solubility of the encapsulated peptides. Mohan et al. (2015) described that the particle size of above 50 μm can significantly affect the solubility, dispersion and, hence, the texture and feel of the food. In our study, the mean apparent diameters of microcapsules – estimated from the SEM images – were 5–7 μm for all samples. However, wide size distributions were observed (from 0.7 to 30 μm). The addition of phycocolloids of P. columbina to the microcapsule formulation increased the width of the particle size distribution. Therefore, the microcapsules produced with P. columbina phycocolloids as wall material (PG-C; from 0.7 to 30 μm) showed a wider but not significant particle size distribution than those obtained with LBG (LBG-C; from 0.7 to 16 μm). Moreover, all these size distributions are typically found in spray dried particles, which were determined mainly by the spray-dryer nozzle diameter used (Carneiro et al., 2013).
The FTIR spectra of microcapsules are shown in Figure S1b. For all microcapsules, a strong O−H stretching peak near 3300 cm−1 was observed, which can be attributed to hydrogen bond of hydroxyl groups present in polysaccharides molecules. In the case of LBG-C, this −OH stretching peak was broadened respect to PG-C, due to the higher –OH content of LGB. Moreover, it was observed a reduction of the intensity of this peak when BSG hydrolyzate was incorporated in capsule formulation (data not shown). This could indicate an interaction between LBG and BSG hydrolysate by hydrogen bounds through hydroxyl groups. All microcapsules showed a C−H stretching vibrations peak around 2928 cm−1. Additional characteristic bands of LBG and P. columbina phycocolloids appeared at 1420 and 1080 cm−1, attributed to C−H and O−H bending vibrations, respectively. Moreover, the bands at 930 cm−1 could be attributed to C–O–C stretching of glycosidic bonds (Kaity et al., 2013). The bands at 1370 and ~1250 cm−1 in microcapsules formulated with P. columbina phycocolloids can be attributed to the S = O vibration of the sulphate groups. Note that, these bands are not present in locust bean gum microcapsules (LBG-C). Moreover, the bands at 845, 830 and 820 cm−1 are used to infer the position of the sulphate group in agarocolloids and are assigned to the 4-sulphate, 2-sulphate and 6-sulphate of the D-galactose units, respectively (Gómez-Ordóñez & Rupérez, 2011). The FTIR spectrum of LBG:PG-C and PG-C showed a band at 850 cm−1, attributed to the sulphate substitution at the C-4 of the galactose. The absence of bands at 830 or 820 cm−1 could indicate that the sulphate substitution at the C-2 and C-6 of the galactose was not present. Similar FTIR spectrums were reported by Martins et al. (2012) for edible films developed by k-carrageenan and LBG mixtures. Finally, the bands at 1150 and 775 cm−1 can be assigned to C–O stretching vibrations of pyranose ring of hydrocolloids (Cian et al., 2014; Mathur, 2017). In addition, Amida I band (1660–1630 cm−1), with a maximum peak at 1653 cm−1, was observed in the three spectra. This band can be attributed mainly from the C = O stretching vibration with minor contributions from the out-of-phase C-N stretching vibration, the C-C-N deformation and the N-H in-plane bend (Barth, 2007). This result corroborates the presence of the peptides in the microcapsules.
Zeta potential of microcapsules obtained with different ratios of LBG and P. columbina phycocolloids is shown in Table 1. In all cases, the zeta potential of microcapsules was lower than −28 mV at pH 7.0, indicating that the microcapsules had a strong negative charge at the surface of particle. In general, a zeta potential of ± 30 mV is required as a minimum for a physical stable suspension only stabilized by electrostatic repulsion (Mohan et al., 2015). The surface charge of particles has a significant influence on the suspension stability, on the particle interactions with other charged substances and on the adherence of drug delivery systems to biological surfaces (Peres et al., 2012). The high negative zeta potential obtained for microcapsules obtained with different ratios of LBG and P. columbina phycocolloids indicates colloid stability and that there is low tendency for the formation of particle aggregates. Microcapsules added with P. columbina phycocolloids (LBG:PG-C and PG-C) had higher magnitude of zeta-potential value than those formulated with only locust bean gum (LBG-C). The higher negative surface charge can be attributed to sulphate group provided by P. columbina phycocolloids. In this regard, an increase of P. columbina phycocolloids proportion in the formulation as wall material increased sulphate content of microcapsules (P < 0.05) (Table 1), as their most important phycocolloids are sulphated polysaccharides (carrageenans and agars) (Gómez-Ordóñez & Rupérez, 2011).
Encapsulation efficiency and characteristics of BSG hydrolysate microcapsules prepared with different formulas
Microcapsules* . | Encapsulation efficiency (%) . | Zeta potential (mV) . | Sulphate content (mg 100 g−1) . | Yellow index . |
---|---|---|---|---|
LBG-C | 90.9 ± 1.4a | −28.4 ± 0.9b | 51.7 ± 2.7a | 13.4 ± 0.7c |
LBG:PG-C | 98.5 ± 1.2b | −37.4 ± 2.1a | 1826.1 ± 2.7b | 11.1 ± 0.4b |
PG-C | 99.4 ± 1.5b | −38.3 ± 1.0a | 2538.9 ± 3.5c | 7.0 ± 0.5a |
Microcapsules* . | Encapsulation efficiency (%) . | Zeta potential (mV) . | Sulphate content (mg 100 g−1) . | Yellow index . |
---|---|---|---|---|
LBG-C | 90.9 ± 1.4a | −28.4 ± 0.9b | 51.7 ± 2.7a | 13.4 ± 0.7c |
LBG:PG-C | 98.5 ± 1.2b | −37.4 ± 2.1a | 1826.1 ± 2.7b | 11.1 ± 0.4b |
PG-C | 99.4 ± 1.5b | −38.3 ± 1.0a | 2538.9 ± 3.5c | 7.0 ± 0.5a |
LBG-C, LBG:PG-C, and PG-C: capsules of BSG hydrolysate obtained with 100:0 LBG/PG, 50:50 LBG/PG, and 0:100 LBG/PG respectively. Results are expressed as mean value ± standard deviation. Different letters in the same column mean significant differences among samples (P < 0.05), according to least significant difference (LSD) test.
Encapsulation efficiency and characteristics of BSG hydrolysate microcapsules prepared with different formulas
Microcapsules* . | Encapsulation efficiency (%) . | Zeta potential (mV) . | Sulphate content (mg 100 g−1) . | Yellow index . |
---|---|---|---|---|
LBG-C | 90.9 ± 1.4a | −28.4 ± 0.9b | 51.7 ± 2.7a | 13.4 ± 0.7c |
LBG:PG-C | 98.5 ± 1.2b | −37.4 ± 2.1a | 1826.1 ± 2.7b | 11.1 ± 0.4b |
PG-C | 99.4 ± 1.5b | −38.3 ± 1.0a | 2538.9 ± 3.5c | 7.0 ± 0.5a |
Microcapsules* . | Encapsulation efficiency (%) . | Zeta potential (mV) . | Sulphate content (mg 100 g−1) . | Yellow index . |
---|---|---|---|---|
LBG-C | 90.9 ± 1.4a | −28.4 ± 0.9b | 51.7 ± 2.7a | 13.4 ± 0.7c |
LBG:PG-C | 98.5 ± 1.2b | −37.4 ± 2.1a | 1826.1 ± 2.7b | 11.1 ± 0.4b |
PG-C | 99.4 ± 1.5b | −38.3 ± 1.0a | 2538.9 ± 3.5c | 7.0 ± 0.5a |
LBG-C, LBG:PG-C, and PG-C: capsules of BSG hydrolysate obtained with 100:0 LBG/PG, 50:50 LBG/PG, and 0:100 LBG/PG respectively. Results are expressed as mean value ± standard deviation. Different letters in the same column mean significant differences among samples (P < 0.05), according to least significant difference (LSD) test.
As shown in Fig. 1a, microcapsules added with P. columbina phycocolloids had lower fluorescence intensity value than LBG-C. Moreover, those only added with PG-C showed the lowest fluorescence intensity (P < 0.05). This result suggests that during encapsulation, hydrophobic interactions occur between P. columbina phycocolloids and BSG hydrolysate decreasing the surface exposure of hydrophobic groups. Moreover, the addition of SDS increased the fluorescence intensity of LBG:PG-C and PG-C (P < 0.05), which can be due to the disruption of the hydrophobic interactions between P. columbina phycocolloids and BSG hydrolysate. Note that SDS is an anionic detergent and a powerful disruptor of hydrophobic interactions among molecules (Schmitt et al., 2010).
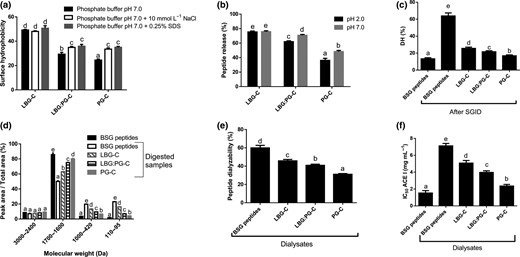
Surface hydrophobicity (a), peptide release (b), degree of hydrolysis (c), proportion of molecular weight species respect to total area obtained from FPLC gel filtration (d), peptide dialyzability (e) and Angiotensin-I converting enzyme inhibitory activity (f) of BSG hydrolysate encapsulated with different ratios of locust bean gum (LBG) and Pyropia columbina phycocolloids (PG). LBG-C, LBG:PG-C and PG-C: microcapsules of BSG hydrolysate obtained with 100:0 LBG/PG, 50:50 LBG/PG and 0:100 LBG/PG respectively. Different letters mean significant differences between samples (P < 0.05).
Similarly, fluorescence intensity of LBG:PG-C and PG-C also increased after addition of NaCl (P < 0.05), indicating that salt disrupted the electrostatic forces between P. columbina phycocolloids and BSG hydrolysate. Therefore, there are both electrostatic and hydrophobic forces between P. columbina phycocolloids and BSG hydrolysate in microcapsules. However, no effect on the fluorescence intensity of LBG-C was observed with the addition of SDS or NaCl. This result could indicate that the interaction between LBG and BSG hydrolysate is due to other intermolecular forces than electrostatic or hydrophobic, like hydrogen bonds, Van der Waals or glycosidic bounds formed by Maillard reaction. Under extreme conditions, such as high drying temperature, polysaccharides are susceptible to react with peptides to form complex products (e.g. Maillard reaction products), which can deplete the bioactivity of peptides (Mohan et al., 2015; Quintero et al., 2018).
Francis & Clydesdale (1975) proposed the use of the yellowing index as a colour measurement related to browning intensity. In the present work, yellow index was used as an indirect measurement of browning reaction among carbohydrates of wall material and BSG peptide. Table 1 shows the yellow index values of microcapsules obtained with different ratios of LBG and P. columbina phycocolloids. The increase of LBG level in capsule formulation increased yellow index (P < 0.05), indicating that this gum could react with BSG peptides more strongly than P. columbina phycocolloids during spray drying. Thus, sulphate groups from PG probably contribute to avoid browning. In this regard, it has been reported than sulphate-containing compounds could inhibit the cross-linking and the browning occurring during the advanced stages of the Maillard reaction by scavenging the free-radicals and the reactive di-carbonyls (Seo & Karboune, 2014).
Peptide release of microcapsules at pH 2.0 and pH 7.0 is shown in Fig. 1b. At both pH a higher level of P. columbina phycocolloids in capsule formulation reduced peptide release, it being inversely related to P. columbina phycocolloids content (r2 = 0.9913 and 0.9380 for pH 2.0 and 7.0, respectively). These results could indicate that P. columbina phycocolloids interact more strongly with BSG hydrolysate than LBG in aqueous medium. At pH 2.0, the sulphate groups of P. columbina phycocolloids remain negatively charged, allowing them to interact with the peptides by electrostatic forces. The basic amino acids content in BSG was higher than that of acidic amino acids, indicating a higher proportion of positive charges in the hydrolyzate (Table S2). Thus, at pH 2.0 the peptides had a very strong positive charge, which allows them to interact with the sulphate groups from PG. Moreover, hydrophobic interactions between phycocolloids and BSG hydrolysate may occur, contributing to the capsule stability at pH 2.0. In this regard, hydrophobic amino acids content in BSG was very high, exceeding 60% (Table S2). At pH 7.0, sulphate groups of P. columbina phycocolloids are strongly charged, but BSG peptides are less positively charged than at pH 2. Thus, a weaker interaction between peptides and PG contributes to a higher peptide release.
On the other hand, the stable pH range of LBG in aqueous solution is 3.0–11.0 (Dionísio & Grenha, 2012). Thus, the acidic pH could affect the capsule integrity, increasing peptide release at pH 2.0. In this sense, there was no significant difference in the peptide release of LBG-C at both pH values (Fig. 1b), indicating that a higher peptide release occurs at pH 2.0 (~80%). This result indicates that BSG peptides would be released in the gastric environment, they being susceptible to enzymatic degradation before arrive to the intestinal absorptions site, when encapsulating only with LBG.
Simulated gastrointestinal digestion, bioaccessibility and ACE-I inhibitory activity
The degree of hydrolysis (DH) obtained before and after simulated gastrointestinal digestion (SGID) of BSG hydrolysate is shown in Fig. 1c. SGID increased the DH value 5 times, indicating that pepsin and pancreatin enzymes degrade the peptides of BSG hydrolysate. This effect also was observed in the FPLC gel filtration profile where 1700–1600 Da peak area of BSG hydrolysate was significantly higher than that obtained for BSG hydrolysate after SGID (Fig. 1d). Moreover, digested non-encapsulated BSG peptides showed higher proportion of low molecular weight peptides (1000–420 Da) and free amino acids (110–95 Da) than BSG hydrolysate (P < 0.05). These results suggest that in vitro gastrointestinal digestion produced an effective degradation of intermediate molecular weight species, increasing the proportion of low molecular weight peptides. On the other hand, pepsin and pancreatin enzymes degrade the peptides of BSG encapsulated with different wall material, producing an increase of DH, low molecular weight peptides (1000–420 Da) and amino acids (110–95 Da) respect to BSG hydrolysate (P < 0.05). However, the DH and the proportion of low MW peptides obtained for all microcapsules were lower than that of BSG hydrolysate after SGID, indicating a partial protection of encapsulated peptides. Moreover, microcapsules formulated with of P. columbina phycocolloids as wall material showed the highest resistance against enzymatic degradation, DH being lower than those obtained for LBG-C and LBG:PG-C. In this regard, the 1700–1600 Da peak area of PG-C was significantly higher than obtained for LBG-C or LBG:PG-C, indicating high protection of BSG peptides against digestive enzymes.
Peptide dialyzability of BSG hydrolysate and encapsulated peptides after SGID is shown in Fig. 1e. Peptide dialyzability of digested BSG hydrolysate was higher than those obtained for encapsulated ones. This result is in agreement with FPLC gel filtration profile which showed high proportion of low molecular weight species generated by pepsin and pancreatin from BSG hydrolysate (1000–420 and 110–95 Da). Microcapsules formulated with P. columbina phycocolloids showed the lowest peptide dialyzability and lower amount of molecular weight species lower than 1000 Da, indicating that PG-C protected the BSG peptides more efficiently.
Regarding ACE-I inhibition of dialysates, all samples showed inhibitory activity (Fig. 1f). However, the IC50 value of BSG hydrolysate after SGID was higher than that obtained for BSG peptides. Thus, in vitro gastrointestinal digestion decreased ACE-I inhibitory activity of peptides. Similar results were found by Thuanthong et al. (2017) for ACE-I inhibition by peptides obtained from Nile tilapia (Oreochromis niloticus) skin gelatin. Moreover, Cian et al. (2018) reported that in vitro gastrointestinal digestion with pepsin and pancreatin enzymes degrade ACE-I inhibitory peptides from BSG hydrolysates, IC50 value of digest sample being increased. In general, small peptides (di- and tri-peptides) have shown a high ACE-I inhibitory activity (Connolly et al., 2015). Even though the peptide size distribution of the digest hydrolysate showed a lower proportion of the large peptides (1700–1600 Da) and a higher content of small peptides (1000–420 Da) than BSG hydrolysate, the small peptides produced during SGID did not present active sequences or were single amino acids without activity. Similar results were reported by Thuanthong et al. (2017). They found that SGID reduced the peptide size distribution of gelatin hydrolyzates from 880 to 210 Da, decreasing the ACE-I inhibitory activity of digested samples.
As mentioned before, peptide proteolysis was reduced by encapsulation. However, the IC50 values for all capsule formulations were higher than that obtained for BSG hydrolysate, but lower than digested BSG hydrolysate, showing a partial protection of bioactive peptides from digestive enzymes by encapsulation (Fig. 1f). Moreover, PG-C not only showed the highest resistance against enzymatic degradation (~70%), but also IC50 value was lower than those obtained for LBG-C and LBG:PG-C. These results are in agreement with the FPLC gel filtration profile of microcapsules (Fig. 1d), indicating that intermediate molecular weight peptides (1700–1600 Da) could be responsible for the ACE-I inhibitory effect. In this regard, Ciau-Solís et al. (2018) reported that peptide fractions from P. lunatus hydrolysate with molecular weight >1000 Da had higher ACE-I inhibitory activity than those with < 1000 Da. However, further purification and physicochemical characterisation studies of ACE-I inhibitory peptides from PG-C dialysate must be done to confirm these results.
Conclusion
Brewers’ spent grain peptides were significantly affected by simulated gastrointestinal digestion. These peptides were encapsulated for preserving them from the gastrointestinal environment in a way they maintain their activity intact in the absorption site. The encapsulation partially preserved the ACE-I inhibitory activity in a stronger way when P. columbina phycocolloids were in a higher level in wall hydrocolloid matrix. Electrostatic and hydrophobic interactions between P. columbina phycocolloids and brewers’ spent grain peptides were observed, indicating a possible role in the protection of peptides during gastrointestinal digestion. These studies showed that not only the feasibility of encapsulation must be investigated (protein efficiency, particle size, surface hydrophobicity, etc.) but also the bioaccessibility of the activity of the encapsulated material. In this regard, peptides encapsulated with P. columbina phycocolloids showed the lowest dialyzability but the highest ACE-I inhibitory activity in the dialysated sample, indicating that the BSG peptides maintained their potency after gastrointestinal digestion, regardless of their proportion in the dialysate. No synergistic effect on physicochemical properties of capsule was observed between locust bean gum and carrageenan/agar from red seaweed P. columbina. Moreover, the use of sulphated gum for encapsulation of peptides could be recommended.
Acknowledgments
REC, PRS and SRD carried out the experiment. All authors analysed the data and wrote the paper and had primary responsibility for final content. All authors read and approved the final manuscript. The authors are thankful to PICT-2016–2716 and PICT-2016-2879 from ANPCyT for the financial support.
Ethical guidelines
Ethics approval was not required for this research.
Conflicts of interest
The authors declare that there are no conflicts of interest.
Data availability
Research data are not shared.