-
PDF
- Split View
-
Views
-
Cite
Cite
Ying Sun, Zhengfu Wang, Jihong Wu, Fang Chen, Xiaojun Liao, Xiaosong Hu, Optimising enzymatic maceration in pretreatment of carrot juice concentrate by response surface methodology, International Journal of Food Science and Technology, Volume 41, Issue 9, November 2006, Pages 1082–1089, https://doi.org/10.1111/j.1365-2621.2006.01182.x
- Share Icon Share
Abstract
In this paper, enzyme preparation for carrot pulp maceration was screened out and enzymatic maceration processing condition of the carrot pulp was optimised by response surface methodology for carrot juice concentrate. Pectinex Smash XXL was the best commercial enzyme preparation than Pectinex Ultra SP-L, Pectinase FNP-1 and cellulose FNC-1 employed in the carrot juice processing in the study. The effect of enzyme concentration and incubation time and their complex interaction on juice β-carotene content, juice yield and viscosity in the maceration process was studied by using experiments of central composite rotatable design. The results indicated that under the optimal conditions that the enzyme concentration was 100 mL t−1 and incubation time was 80 min, the juice β-carotene content was ≥54.2 mg kg−1, the juice yield ≥63.5% and the juice viscosity ≤2.1 mPa S.
Introduction
Carrot is an important vegetable crop world-wide and is rich in carotenoids, which are a natural bioactive compound. Of the various carotenoids in carrots, β-carotene constitutes a large portion. The beneficial effect of β-carotene on human health has been well established (Chen et al., 1996). Recently Stoll et al. (2003) recommended that daily intake of isolated β-carotene should not exceed 2 mg. In contrast, it has been recognised that intake of carotenes from fruits and vegetables is safe and beneficial (Stoll et al., 2003). Carrot juices and derived products such as ATBC (Alpha-Tocopheral, Beta-Carotene, vitamin supplement) and breakfast drinks represented an emerging market with growing economic importance (Schieber et al., 2001). In recent years, as an important nutritional source of carotene, the consumption of carrot juices in China has been increasing steadily.
Conventional carrot juice production with mechanical pressing of the mash results in a slightly cloudy juice and a carotene-rich pomace, and the carotene insoluble in water remains in the carrot cells. Mash treatments with pectolytic enzymes are now essential in juice industry in order to get high yield of juice with high aromatic quality in a short processing time, to increase the carotene content and to reduce the amount of waste pomace (Sarioglu et al., 2001; Demir et al., 2004).
Juice β-carotene, juice yield and juice viscosity are of importance in carrot juice concentrate manufactured with enzyme maceration. In order to obtain higher juice yield and higher β-carotene from carrot pulp, pectinase is employed to break down pectin and facilitate separation of carrot juice and release of β-carotene (Mutlu et al., 1999). This process will reduce the viscosity of carrot juice. A lower viscosity beneficially increases the process of evaporation, preventing the formation of gells. Cloudy stability is a great concern for carrot juice because carrot juice is generally a cloudy juice, and it is important to prevent loss of cloudy stability in the extraction of carrot juice. In the process of enzyme maceration if the enzyme preparation is badly selected or over-maceration occurs the loss of juice cloudy stability was caused although a higher juice yield was reached. Researches have indicated that enzyme maceration is influenced by enzyme preparation, enzyme concentration, incubation time and hydrolysis temperature.
Although there are many reports on enzymatic maceration, little information on optimisation of processing condition is available. The general practice for determining the optimal operating conditions is by means of varying one parameter while keeping the others at a constant level. The major disadvantage of this single-variable optimisation is that it does not include interactive effects among the variables, and therefore it does not depict the net effects of various parameters on the reaction rate (Rai et al., 2004). When many factors and their interactions affect a desired response, the response surface methodology (RSM) is an effective tool for optimising the process conditions. RSM is a statistical method for determining and simultaneously solving multivariate equations (Roy et al., 2002). It usually uses an experimental design such as a central composite rotatable design (CCRD) to fit a first- or second-order polynomial by a least significance technique. An equation is used to describe how the test variables affect the response, and to determine the interrelationship among the test variables in the response. The contour plots can be usefully employed to study the response surfaces and locate the optimal parameters. This graphical optimisation technique has been used in other juice treatments such as mango juice (Rastogi & Rashmi, 1999), mosambi juice (Roy et al., 2002). Also it could be used to maximise juice yield and β-carotene content of carrot juice and to minimise apparent viscosity of carrot pulp.
The objective of this study was to optimise the enzyme concentration and incubation time in the carrot pulp maceration by RSM for carrot juice concentrate.
Materials and methods
Materials
Enzyme preparations
Pectinex Smash XXL and Pectinex Ultra SP-L were obtained from Novozymes Co. (Beijing, China), which are produced from Aspergillus aculeatus with activity 30 000 UPTE mL−1, and is brown in liquid state. The suitable maceration temperature is 40–45 °C and the optimal pH 4.5–5.5.
Pectinase FNP-1 and ellulase FNC-1 were from Beijing Funong Food Co. (Beijing, China). It is brown in liquid state. The suitable maceration temperature is 40–45 °C and the optimal pH is 4.5–5.5.
Citric acid was purchased from Beijing Chemicals Co. (Beijing, China), which is of analytical grade. Fresh and mature carrot was from China Agricultural Science Academy (CASA, Beijing, China) at the late autumn in 2003, the cultivar name is defined as No. 1 Orange-red carrot, cultured by CASA.
Methods
Fresh carrots were washed with tap water, and blemished carrots were discarded, then carrots of good quality were sliced into about 2 cm thickess, acid-blanched with citric acid, and pulped by a pulp processor (Ouke, ZHJ-308A1l; Fushan Ouke Electric Appliance Co., Tushan, China). The pH of carrot pulp was adjusted from 5.0 to 4.8 and macerated for enzyme preparations, and heated with a microwave oven (Sanyo, EM-8518, Hefei Rongshida & Sanyo Co., Hefei, China) and then cooled. The juice was then pressed with hand and centrifuged (Feige,TDL-5-A, Beijing Feige Instrument Co., Beijing, China). Carrot juice extraction process is shown in Fig. 1.
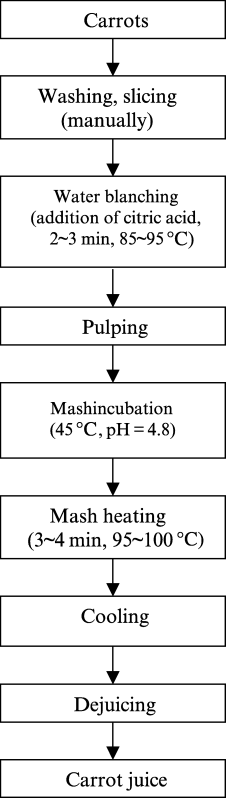
Determination of β-carotene content
A volume of 10 mL carrot juice was extracted for three to four times in an amber glass separatory funnel with petroleum ether and acetone (1:1, v:v) until the sample was colourless. After separation, butylated hydroxytoluene was added as an antioxidant to reach a final concentration of 0.1%, and the extract was dehydrated with anhydrous sodium sulphate. The petroleum ether and acetone was evaporated in a rotary evaporator (40–45 °C), the residue was dissolved in 1 mL mobile phase (acetonitrile:methanol:di-chlormethane = 75:20:5), and was filtered through 0.45-μm Millipore membrane. β-Carotene was analysed using an HPLC system (HP-1100; HP Inc., Palo Alto, CA, USA). The HPLC system was equipped with an autoinjector and a diode array detector; the reverse phase column C18 (150 × 3.9 mm) with a particle size 5 μm (waters symmetry). The mobile phase was acetonitrile:methanol:di-chlormethane =75:20:5 with a flow rate of 1.5 mL min−1. Cantification was carried out by external standards (Sigma Chemical Company, St Louis, MO, USA). The purity of β-carotene standard was 95%.
Estimation of juice yield
The juice yield was estimated as a weight percentage of the juice obtained to the initial pulp. The formula is:

Measurement of juice viscosity and turbidity
Viscosities of juice samples were determined at room temperature by means of a rotary viscometer (Fungilab, Barcelona, Spain) with spindle at 100 r.p.m. (Model No. LCP). A volume of 10 mL carrot juice in each run was sampled; all measurements were performed in triplicate. Turbidities of carrot juice were measured with a turbiditymeter (Shanke Instrument Co., Shanghai, China) at room temperature; all measurements were performed in triplicate.
Measurement of total soluble solids
The total soluble solids (TSS) of carrot juice was measured with a WAY-2S digital Abbe Refractionmeter (Shanghai Precision & Scientific Instrument Co., Shanghai, China) at room temperature; all measurement were performed in triplicate.
Scheme of the screening of enzyme preparation
Nine experiments were designed to select one kind of enzyme based on preliminary experiments, which should fit the production of cloudy carrot juice. The numbers of experiments and the enzyme preparation are shown in Table 1.
Experiment no. . | Pectinex Smash XXL . | Pectinex Ultra SP-L . | Pectinase FNP-1 . | Cellulase FNC-1 . |
---|---|---|---|---|
1 | – | – | – | – |
2 | 0.10 | – | – | – |
3 | – | 0.10 | – | – |
4 | 0.025 | 0.075 | – | – |
5 | 0.05 | 0.05 | – | – |
6 | 0.075 | 0.025 | – | – |
7 | – | – | 0.10 | – |
8 | – | – | 0.10 | 0.05 |
9 | – | – | 0.10 | 0.10 |
Experiment no. . | Pectinex Smash XXL . | Pectinex Ultra SP-L . | Pectinase FNP-1 . | Cellulase FNC-1 . |
---|---|---|---|---|
1 | – | – | – | – |
2 | 0.10 | – | – | – |
3 | – | 0.10 | – | – |
4 | 0.025 | 0.075 | – | – |
5 | 0.05 | 0.05 | – | – |
6 | 0.075 | 0.025 | – | – |
7 | – | – | 0.10 | – |
8 | – | – | 0.10 | 0.05 |
9 | – | – | 0.10 | 0.10 |
‘–’, No added enzyme preparation.
Experiment no. . | Pectinex Smash XXL . | Pectinex Ultra SP-L . | Pectinase FNP-1 . | Cellulase FNC-1 . |
---|---|---|---|---|
1 | – | – | – | – |
2 | 0.10 | – | – | – |
3 | – | 0.10 | – | – |
4 | 0.025 | 0.075 | – | – |
5 | 0.05 | 0.05 | – | – |
6 | 0.075 | 0.025 | – | – |
7 | – | – | 0.10 | – |
8 | – | – | 0.10 | 0.05 |
9 | – | – | 0.10 | 0.10 |
Experiment no. . | Pectinex Smash XXL . | Pectinex Ultra SP-L . | Pectinase FNP-1 . | Cellulase FNC-1 . |
---|---|---|---|---|
1 | – | – | – | – |
2 | 0.10 | – | – | – |
3 | – | 0.10 | – | – |
4 | 0.025 | 0.075 | – | – |
5 | 0.05 | 0.05 | – | – |
6 | 0.075 | 0.025 | – | – |
7 | – | – | 0.10 | – |
8 | – | – | 0.10 | 0.05 |
9 | – | – | 0.10 | 0.10 |
‘–’, No added enzyme preparation.
Experiment design
Table 2 shows a CCRD with two variables which was used to study the response pattern and to determine the optimum combination of the variables. The optimised variables were enzyme concentration (29.3–170.7) and time of reaction (32.02–88.28) each at five levels: −1.414, −1, 0, 1 and 1.414. Table 1 shows the symbols and levels.
Variable . | Symbols . | Levels . | |||||
---|---|---|---|---|---|---|---|
Coded . | Uncoded . | −1.414 . | −1 . | 0 . | 1 . | 1.414 . | |
Enzyme conc. (mL t−1) | X1 | x1 | 29.3 | 50 | 100 | 150 | 170.7 |
Time (min) | X2 | x2 | 32.02 | 40 | 60 | 80 | 88.28 |
Variable . | Symbols . | Levels . | |||||
---|---|---|---|---|---|---|---|
Coded . | Uncoded . | −1.414 . | −1 . | 0 . | 1 . | 1.414 . | |
Enzyme conc. (mL t−1) | X1 | x1 | 29.3 | 50 | 100 | 150 | 170.7 |
Time (min) | X2 | x2 | 32.02 | 40 | 60 | 80 | 88.28 |
X1 = (x1 − 100)/50 and X2 = (x2 − 60)/20.
Variable . | Symbols . | Levels . | |||||
---|---|---|---|---|---|---|---|
Coded . | Uncoded . | −1.414 . | −1 . | 0 . | 1 . | 1.414 . | |
Enzyme conc. (mL t−1) | X1 | x1 | 29.3 | 50 | 100 | 150 | 170.7 |
Time (min) | X2 | x2 | 32.02 | 40 | 60 | 80 | 88.28 |
Variable . | Symbols . | Levels . | |||||
---|---|---|---|---|---|---|---|
Coded . | Uncoded . | −1.414 . | −1 . | 0 . | 1 . | 1.414 . | |
Enzyme conc. (mL t−1) | X1 | x1 | 29.3 | 50 | 100 | 150 | 170.7 |
Time (min) | X2 | x2 | 32.02 | 40 | 60 | 80 | 88.28 |
X1 = (x1 − 100)/50 and X2 = (x2 − 60)/20.
The CCRD shown in Table 3 was arranged to fit an appropriate regression model by using a multiple regression program. Five replicates (treatments 9–13) at the centre of design were used to estimate the pure error sum of squares. Experiments were randomised in order to maximise the effects of unexplained variability in the observed responses due to extraneous factors.
Experiment no. . | Enzyme conc. (mL t−1) X1 . | Time (min) X2 . | β-Carotene (mg kg−1) Y1 . | Yield (%) Y2 . | Viscosity (mPa s) Y3 . |
---|---|---|---|---|---|
1 | 1 | 1 | 52.96 | 62.64 | 1.99 |
2 | 1 | −1 | 46.20 | 59.53 | 1.79 |
3 | −1 | 1 | 47.21 | 60.80 | 2.52 |
4 | −1 | −1 | 43.33 | 61.13 | 2.42 |
5 | 1.414 | 0 | 45.62 | 60.12 | 2.08 |
6 | −1.414 | 0 | 42.50 | 57.07 | 2.60 |
7 | 0 | 1.414 | 55.80 | 64.40 | 2.20 |
8 | 0 | −1.414 | 45.72 | 53.53 | 2.35 |
9 | 0 | 0 | 54.79 | 63.95 | 2.03 |
10 | 0 | 0 | 55.02 | 63.70 | 2.04 |
11 | 0 | 0 | 54.95 | 63.76 | 2.02 |
12 | 0 | 0 | 54.66 | 63.82 | 2.03 |
13 | 0 | 0 | 54.78 | 63.77 | 2.03 |
Experiment no. . | Enzyme conc. (mL t−1) X1 . | Time (min) X2 . | β-Carotene (mg kg−1) Y1 . | Yield (%) Y2 . | Viscosity (mPa s) Y3 . |
---|---|---|---|---|---|
1 | 1 | 1 | 52.96 | 62.64 | 1.99 |
2 | 1 | −1 | 46.20 | 59.53 | 1.79 |
3 | −1 | 1 | 47.21 | 60.80 | 2.52 |
4 | −1 | −1 | 43.33 | 61.13 | 2.42 |
5 | 1.414 | 0 | 45.62 | 60.12 | 2.08 |
6 | −1.414 | 0 | 42.50 | 57.07 | 2.60 |
7 | 0 | 1.414 | 55.80 | 64.40 | 2.20 |
8 | 0 | −1.414 | 45.72 | 53.53 | 2.35 |
9 | 0 | 0 | 54.79 | 63.95 | 2.03 |
10 | 0 | 0 | 55.02 | 63.70 | 2.04 |
11 | 0 | 0 | 54.95 | 63.76 | 2.02 |
12 | 0 | 0 | 54.66 | 63.82 | 2.03 |
13 | 0 | 0 | 54.78 | 63.77 | 2.03 |
Experiment no. . | Enzyme conc. (mL t−1) X1 . | Time (min) X2 . | β-Carotene (mg kg−1) Y1 . | Yield (%) Y2 . | Viscosity (mPa s) Y3 . |
---|---|---|---|---|---|
1 | 1 | 1 | 52.96 | 62.64 | 1.99 |
2 | 1 | −1 | 46.20 | 59.53 | 1.79 |
3 | −1 | 1 | 47.21 | 60.80 | 2.52 |
4 | −1 | −1 | 43.33 | 61.13 | 2.42 |
5 | 1.414 | 0 | 45.62 | 60.12 | 2.08 |
6 | −1.414 | 0 | 42.50 | 57.07 | 2.60 |
7 | 0 | 1.414 | 55.80 | 64.40 | 2.20 |
8 | 0 | −1.414 | 45.72 | 53.53 | 2.35 |
9 | 0 | 0 | 54.79 | 63.95 | 2.03 |
10 | 0 | 0 | 55.02 | 63.70 | 2.04 |
11 | 0 | 0 | 54.95 | 63.76 | 2.02 |
12 | 0 | 0 | 54.66 | 63.82 | 2.03 |
13 | 0 | 0 | 54.78 | 63.77 | 2.03 |
Experiment no. . | Enzyme conc. (mL t−1) X1 . | Time (min) X2 . | β-Carotene (mg kg−1) Y1 . | Yield (%) Y2 . | Viscosity (mPa s) Y3 . |
---|---|---|---|---|---|
1 | 1 | 1 | 52.96 | 62.64 | 1.99 |
2 | 1 | −1 | 46.20 | 59.53 | 1.79 |
3 | −1 | 1 | 47.21 | 60.80 | 2.52 |
4 | −1 | −1 | 43.33 | 61.13 | 2.42 |
5 | 1.414 | 0 | 45.62 | 60.12 | 2.08 |
6 | −1.414 | 0 | 42.50 | 57.07 | 2.60 |
7 | 0 | 1.414 | 55.80 | 64.40 | 2.20 |
8 | 0 | −1.414 | 45.72 | 53.53 | 2.35 |
9 | 0 | 0 | 54.79 | 63.95 | 2.03 |
10 | 0 | 0 | 55.02 | 63.70 | 2.04 |
11 | 0 | 0 | 54.95 | 63.76 | 2.02 |
12 | 0 | 0 | 54.66 | 63.82 | 2.03 |
13 | 0 | 0 | 54.78 | 63.77 | 2.03 |
A second-order polynomial equation was used to fit the experimental data obtained according to the design in Table 2. The model proposed for the response was:

where Y is the predicted responses for juice yield, β-carotene content and viscosity, respectively. A0 is the value of the fitted response at the centre point of the design, and Ai, Aii and Aij are the coefficients of linear, quadratic and cross-product terms, respectively. Optimisation of the fitted polynomials was done by means of a graphic technique. The optimum condition was verified by conducting experiments at that condition. Responses were monitored and results were compared with model predictions.
The fitted polynomial equations were expressed as surfaces and contour plots by using the Statistica software version 6.0 (Stasoft Inc., Tulsa, OK, USA) in order to visualise the relation between the response and experimental levels of each factor and to deduce the optimum condition.
Results and discussion
Screening of the enzyme preparations for pulp maceration
Table 4 shows the results of the carrot pulp maceration. Juice yield and TSS increased significantly (P < 0.05), and viscosity decreased very significantly (P < 0.01), when compared with control experiment 1. However, the performance of juice turbidity and β-carotene is different depending on the enzyme preparations. The mash treatment with Pectinex Smash XXL increased not only the juice yield significantly, but also the β-carotene content up to 59.12 ± 1.88 mg kg−1 in all the experiments. Moreover, the juice turbidity (NTU =305.2 ± 12.5) has a little decrease and is not significant from the control experiment 1, but the juice turbidity from other experiments is reduced significantly. The decrease in β-carotene content in experiments 8 and 9 may be due to the increase in juice yield. During this process proto-pectin and pectin are degraded into galacturonic acid, thereby viscosity and turbidity are decreased reasonably (Sarioglu et al., 2001). Only the carrot juice obtained from Pectinex Smash XXL was stable in relation to cloudiness and the carrot juice from other experiments was gradually clarified or nearly clarified after a 5-month storage at room temperature. So, Pectinex Smash XXL should be the most suitable enzyme preparation for the production of cloudy carrot juice in our study.
Experiment no. . | Yield (%) . | Total soluble solid (°Bx) . | Viscosity (mPa s) . | Turbidity (NTU) . | β-Carotene (mg kg−1) . |
---|---|---|---|---|---|
1 | 40.86 ± 2.27 | 6.0 ± 0.2 | 2.55 ± 0.02 | 320.1 ± 15.2 | 40.75 ± 1.19 |
2 | 57.21 ± 2.56* | 6.9 ± 0.3* | 2.01 ± 0.02** | 305.2 ± 12.5 | 59.12 ± 1.88** |
3 | 60.53 ± 2.34** | 6.5 ± 0.2* | 1.87 ± 0.01** | 170.5 ± 12.7* | 44.39 ± 2.26* |
4 | 57.12 ± 2.63* | 6.6 ± 0.2* | 1.93 ± 0.03** | 170.4 ± 13.6* | 41.61 ± 2.44 |
5 | 54.95 ± 2.35* | 6.8 ± 0.1* | 1.98 ± 0.05** | 187.3 ± 14.2* | 44.54 ± 2.88* |
6 | 52.11 ± 2.08* | 6.8 ± 0.2* | 2.00 ± 0.05** | 190.2 ± 15.1* | 46.96 ± 1.62* |
7 | 67.34 ± 2.31** | 6.5 ± 0.2* | 1.81 ± 0.04** | 170.4 ± 12.2* | 40.23 ± 2.68 |
8 | 68.64 ± 2.05** | 6.6 ± 0.1* | 1.76 ± 0.02** | 120.1 ± 8.8** | 38.07 ± 2.49** |
9 | 70.06 ± 2.44** | 5.9 ± 0.2* | 1.64 ± 0.02** | 110 ± 9.3** | 34.30 ± 2.74** |
Experiment no. . | Yield (%) . | Total soluble solid (°Bx) . | Viscosity (mPa s) . | Turbidity (NTU) . | β-Carotene (mg kg−1) . |
---|---|---|---|---|---|
1 | 40.86 ± 2.27 | 6.0 ± 0.2 | 2.55 ± 0.02 | 320.1 ± 15.2 | 40.75 ± 1.19 |
2 | 57.21 ± 2.56* | 6.9 ± 0.3* | 2.01 ± 0.02** | 305.2 ± 12.5 | 59.12 ± 1.88** |
3 | 60.53 ± 2.34** | 6.5 ± 0.2* | 1.87 ± 0.01** | 170.5 ± 12.7* | 44.39 ± 2.26* |
4 | 57.12 ± 2.63* | 6.6 ± 0.2* | 1.93 ± 0.03** | 170.4 ± 13.6* | 41.61 ± 2.44 |
5 | 54.95 ± 2.35* | 6.8 ± 0.1* | 1.98 ± 0.05** | 187.3 ± 14.2* | 44.54 ± 2.88* |
6 | 52.11 ± 2.08* | 6.8 ± 0.2* | 2.00 ± 0.05** | 190.2 ± 15.1* | 46.96 ± 1.62* |
7 | 67.34 ± 2.31** | 6.5 ± 0.2* | 1.81 ± 0.04** | 170.4 ± 12.2* | 40.23 ± 2.68 |
8 | 68.64 ± 2.05** | 6.6 ± 0.1* | 1.76 ± 0.02** | 120.1 ± 8.8** | 38.07 ± 2.49** |
9 | 70.06 ± 2.44** | 5.9 ± 0.2* | 1.64 ± 0.02** | 110 ± 9.3** | 34.30 ± 2.74** |
Significant at *0.05 and **0.01.
Experiment no. . | Yield (%) . | Total soluble solid (°Bx) . | Viscosity (mPa s) . | Turbidity (NTU) . | β-Carotene (mg kg−1) . |
---|---|---|---|---|---|
1 | 40.86 ± 2.27 | 6.0 ± 0.2 | 2.55 ± 0.02 | 320.1 ± 15.2 | 40.75 ± 1.19 |
2 | 57.21 ± 2.56* | 6.9 ± 0.3* | 2.01 ± 0.02** | 305.2 ± 12.5 | 59.12 ± 1.88** |
3 | 60.53 ± 2.34** | 6.5 ± 0.2* | 1.87 ± 0.01** | 170.5 ± 12.7* | 44.39 ± 2.26* |
4 | 57.12 ± 2.63* | 6.6 ± 0.2* | 1.93 ± 0.03** | 170.4 ± 13.6* | 41.61 ± 2.44 |
5 | 54.95 ± 2.35* | 6.8 ± 0.1* | 1.98 ± 0.05** | 187.3 ± 14.2* | 44.54 ± 2.88* |
6 | 52.11 ± 2.08* | 6.8 ± 0.2* | 2.00 ± 0.05** | 190.2 ± 15.1* | 46.96 ± 1.62* |
7 | 67.34 ± 2.31** | 6.5 ± 0.2* | 1.81 ± 0.04** | 170.4 ± 12.2* | 40.23 ± 2.68 |
8 | 68.64 ± 2.05** | 6.6 ± 0.1* | 1.76 ± 0.02** | 120.1 ± 8.8** | 38.07 ± 2.49** |
9 | 70.06 ± 2.44** | 5.9 ± 0.2* | 1.64 ± 0.02** | 110 ± 9.3** | 34.30 ± 2.74** |
Experiment no. . | Yield (%) . | Total soluble solid (°Bx) . | Viscosity (mPa s) . | Turbidity (NTU) . | β-Carotene (mg kg−1) . |
---|---|---|---|---|---|
1 | 40.86 ± 2.27 | 6.0 ± 0.2 | 2.55 ± 0.02 | 320.1 ± 15.2 | 40.75 ± 1.19 |
2 | 57.21 ± 2.56* | 6.9 ± 0.3* | 2.01 ± 0.02** | 305.2 ± 12.5 | 59.12 ± 1.88** |
3 | 60.53 ± 2.34** | 6.5 ± 0.2* | 1.87 ± 0.01** | 170.5 ± 12.7* | 44.39 ± 2.26* |
4 | 57.12 ± 2.63* | 6.6 ± 0.2* | 1.93 ± 0.03** | 170.4 ± 13.6* | 41.61 ± 2.44 |
5 | 54.95 ± 2.35* | 6.8 ± 0.1* | 1.98 ± 0.05** | 187.3 ± 14.2* | 44.54 ± 2.88* |
6 | 52.11 ± 2.08* | 6.8 ± 0.2* | 2.00 ± 0.05** | 190.2 ± 15.1* | 46.96 ± 1.62* |
7 | 67.34 ± 2.31** | 6.5 ± 0.2* | 1.81 ± 0.04** | 170.4 ± 12.2* | 40.23 ± 2.68 |
8 | 68.64 ± 2.05** | 6.6 ± 0.1* | 1.76 ± 0.02** | 120.1 ± 8.8** | 38.07 ± 2.49** |
9 | 70.06 ± 2.44** | 5.9 ± 0.2* | 1.64 ± 0.02** | 110 ± 9.3** | 34.30 ± 2.74** |
Significant at *0.05 and **0.01.
Model fitting and response surface plotting
By using the Statistica program, three second-order polynomial equations can be obtained. The three fitted models are shown as follows:



Here, Y1 is juice β-carotene content, Y2 is juice yield, Y3 is juice viscosity, X1 is enzyme concentration, and X2 is incubation time.
Let the partial derivative of these equations be zero, the following conclusions can be derived from the above equations. To obtain highest β-carotene content, the enzyme concentration and incubation time should be 104.9 mL t−1 and 81.08 min, respectively. To obtain the highest juice yield the enzyme concentration and incubation time should be 114 mL t−1 and 73.6 min, respectively. To obtain the lowest viscosity the enzyme concentration and incubation time should be 149 mL t−1 and 63.4 min, respectively.
The effect of the enzymatic liquefaction conditions on the responses could be represented by the coefficients of the second-order polynomials. The high values of the coefficient of determination (R2) indicate that the models fit the experiments data well as the R2 is the proportion of variability in the response values explained by the model (Rastogi & Rashmi, 1999). Obviously, the above models have a satisfactory adequacy in fitting the experimental data.
Table 5 illustrates the significance of regression coefficient for β-carotene content (using Student's t-test and P-value). The corresponding variables will be more significant if the absolute t value becomes larger and the P-value becomes smaller (Nor Aishah Saidina Amin & Didi Dwi Anggoro, 2004). It can be seen that the variable with the largest effect was the quadratic of the enzyme concentration (), followed by the linear term of incubation time (X2), and the linear term of enzyme concentration (X1). The factor t-test value (−4.1616) and P-value (0.0252) correspond to
, while the t-test values of 3.5853 and 3.0065 for X2 and X1, respectively, are smaller, but the P-values of 0.0371 and 0.0574 respectively are still significant. The total determination coefficient R2 (92.91%) implies that the sample variation of 92.91% for β-carotene content is attributable to the independent variables, enzyme concentration and incubation time.
Variables . | Regression coefficient . | Standard error . | Computed t-value . | P-value . |
---|---|---|---|---|
Constant | 67.307 | 1.6979 | 32.2994 | 0.0001 |
X1 | 1.271 | 0.6003 | 3.0065 | 0.0574 |
X2 | 7.525 | 0.6004 | 3.5853 | 0.0371 |
![]() | −7.455 | 0.8489 | −4.1616 | 0.0252 |
![]() | −3.579 | 0.9957 | −2.4988 | 0.0878 |
X1X2 | 0.175 | 0.9816 | 0.2533 | 0.0816 |
Variables . | Regression coefficient . | Standard error . | Computed t-value . | P-value . |
---|---|---|---|---|
Constant | 67.307 | 1.6979 | 32.2994 | 0.0001 |
X1 | 1.271 | 0.6003 | 3.0065 | 0.0574 |
X2 | 7.525 | 0.6004 | 3.5853 | 0.0371 |
![]() | −7.455 | 0.8489 | −4.1616 | 0.0252 |
![]() | −3.579 | 0.9957 | −2.4988 | 0.0878 |
X1X2 | 0.175 | 0.9816 | 0.2533 | 0.0816 |
X1 represents enzyme concentration and X2 incubation time.
Variables . | Regression coefficient . | Standard error . | Computed t-value . | P-value . |
---|---|---|---|---|
Constant | 67.307 | 1.6979 | 32.2994 | 0.0001 |
X1 | 1.271 | 0.6003 | 3.0065 | 0.0574 |
X2 | 7.525 | 0.6004 | 3.5853 | 0.0371 |
![]() | −7.455 | 0.8489 | −4.1616 | 0.0252 |
![]() | −3.579 | 0.9957 | −2.4988 | 0.0878 |
X1X2 | 0.175 | 0.9816 | 0.2533 | 0.0816 |
Variables . | Regression coefficient . | Standard error . | Computed t-value . | P-value . |
---|---|---|---|---|
Constant | 67.307 | 1.6979 | 32.2994 | 0.0001 |
X1 | 1.271 | 0.6003 | 3.0065 | 0.0574 |
X2 | 7.525 | 0.6004 | 3.5853 | 0.0371 |
![]() | −7.455 | 0.8489 | −4.1616 | 0.0252 |
![]() | −3.579 | 0.9957 | −2.4988 | 0.0878 |
X1X2 | 0.175 | 0.9816 | 0.2533 | 0.0816 |
X1 represents enzyme concentration and X2 incubation time.
Table 6 illustrates the significance of regression coefficient for juice yield (using Student's t-test and P-value). It can be seen that the variable with the largest effect was the linear term of the incubation time (X2), followed by the linear term of enzyme concentration (X1) and the quadratic of the incubation time (). The factor t-test value (5.1770) and P-value (0.0139) correspond to X2, while the t-test values of 4.3319 and −3.6654 for X1 and
, respectively, are smaller, but the P-values of 0.0227 and 0.0351, respectively, are still significant. The total determination coefficient R2 (95.24%) implies that the sample variation of 95.24% for yield is attributable to the independent variables, enzyme concentration and incubation time.
Variables . | Regression coefficient . | Standard error . | Computed t-value . | P-value . |
---|---|---|---|---|
Constant | 63.799 | 1.1758 | 55.1130 | 0.0001 |
X1 | 0.569 | 0.4157 | 4.3319 | 0.0227 |
X2 | 2.269 | 0.4157 | 5.1770 | 0.0139 |
![]() | −2.041 | 0.6895 | −3.6654 | 0.0351 |
![]() | −1.856 | 0.6895 | −2.3887 | 0.0969 |
X1X2 | 0.86 | 0.5879 | 1.0334 | 0.3774 |
Variables . | Regression coefficient . | Standard error . | Computed t-value . | P-value . |
---|---|---|---|---|
Constant | 63.799 | 1.1758 | 55.1130 | 0.0001 |
X1 | 0.569 | 0.4157 | 4.3319 | 0.0227 |
X2 | 2.269 | 0.4157 | 5.1770 | 0.0139 |
![]() | −2.041 | 0.6895 | −3.6654 | 0.0351 |
![]() | −1.856 | 0.6895 | −2.3887 | 0.0969 |
X1X2 | 0.86 | 0.5879 | 1.0334 | 0.3774 |
X1 represents enzyme concentration and X2 incubation time.
Variables . | Regression coefficient . | Standard error . | Computed t-value . | P-value . |
---|---|---|---|---|
Constant | 63.799 | 1.1758 | 55.1130 | 0.0001 |
X1 | 0.569 | 0.4157 | 4.3319 | 0.0227 |
X2 | 2.269 | 0.4157 | 5.1770 | 0.0139 |
![]() | −2.041 | 0.6895 | −3.6654 | 0.0351 |
![]() | −1.856 | 0.6895 | −2.3887 | 0.0969 |
X1X2 | 0.86 | 0.5879 | 1.0334 | 0.3774 |
Variables . | Regression coefficient . | Standard error . | Computed t-value . | P-value . |
---|---|---|---|---|
Constant | 63.799 | 1.1758 | 55.1130 | 0.0001 |
X1 | 0.569 | 0.4157 | 4.3319 | 0.0227 |
X2 | 2.269 | 0.4157 | 5.1770 | 0.0139 |
![]() | −2.041 | 0.6895 | −3.6654 | 0.0351 |
![]() | −1.856 | 0.6895 | −2.3887 | 0.0969 |
X1X2 | 0.86 | 0.5879 | 1.0334 | 0.3774 |
X1 represents enzyme concentration and X2 incubation time.
Table 7 illustrates the significance of regression coefficient for viscosity (using Student's t-test and P-value). It can be observed that, the variable with the largest effect was the linear term of the enzyme concentration (X1), followed by the quadratic of enzyme concentration () and the quadratic of the incubation time (
). The factor t-test value (−4.6698) and P-value (0.0185) correspond to X1, while the t-test values of 1.6229 and 1.2150 for
and
, respectively, are smaller, but the P-values of 0.0203 and 0.0311, respectively, are still significant. The total determination coefficient R2 (2.19%) implies that the sample variation of 92.19% for viscosity is attributable to the independent variables, enzyme concentration and incubation time.
Variables . | Regression coefficient . | Standard error . | Computed t-value . | P-value . |
---|---|---|---|---|
Constant | 2.03 | 0.1359 | 14.9344 | 0.0001 |
X1 | −0.237 | 0.0481 | −4.6698 | 0.0185 |
X2 | 0.011 | 0.0481 | −0.3150 | 0.0977 |
![]() | 0.123 | 0.0797 | 1.6229 | 0.0203 |
![]() | 0.091 | 0.0797 | 1.2150 | 0.0311 |
X1X2 | 0.025 | 0.0680 | 0.0689 | 0.0525 |
Variables . | Regression coefficient . | Standard error . | Computed t-value . | P-value . |
---|---|---|---|---|
Constant | 2.03 | 0.1359 | 14.9344 | 0.0001 |
X1 | −0.237 | 0.0481 | −4.6698 | 0.0185 |
X2 | 0.011 | 0.0481 | −0.3150 | 0.0977 |
![]() | 0.123 | 0.0797 | 1.6229 | 0.0203 |
![]() | 0.091 | 0.0797 | 1.2150 | 0.0311 |
X1X2 | 0.025 | 0.0680 | 0.0689 | 0.0525 |
X1 represents enzyme concentration and X2 incubation time.
Variables . | Regression coefficient . | Standard error . | Computed t-value . | P-value . |
---|---|---|---|---|
Constant | 2.03 | 0.1359 | 14.9344 | 0.0001 |
X1 | −0.237 | 0.0481 | −4.6698 | 0.0185 |
X2 | 0.011 | 0.0481 | −0.3150 | 0.0977 |
![]() | 0.123 | 0.0797 | 1.6229 | 0.0203 |
![]() | 0.091 | 0.0797 | 1.2150 | 0.0311 |
X1X2 | 0.025 | 0.0680 | 0.0689 | 0.0525 |
Variables . | Regression coefficient . | Standard error . | Computed t-value . | P-value . |
---|---|---|---|---|
Constant | 2.03 | 0.1359 | 14.9344 | 0.0001 |
X1 | −0.237 | 0.0481 | −4.6698 | 0.0185 |
X2 | 0.011 | 0.0481 | −0.3150 | 0.0977 |
![]() | 0.123 | 0.0797 | 1.6229 | 0.0203 |
![]() | 0.091 | 0.0797 | 1.2150 | 0.0311 |
X1X2 | 0.025 | 0.0680 | 0.0689 | 0.0525 |
X1 represents enzyme concentration and X2 incubation time.
The three-dimensional surface plots are drawn by Statistica program according to Table 4, which illustrate the main and interactive effects of the independent variables on the dependent one. The response surfaces based on experiments data are shown in Fig. 2a–c. The effect of enzyme concentration and incubation time on response β-carotene content, juice yield and viscosity are represented (Tables 5–7) by the coefficients of second-order polynomials. The response surfaces indicate a complex interaction between the variables.
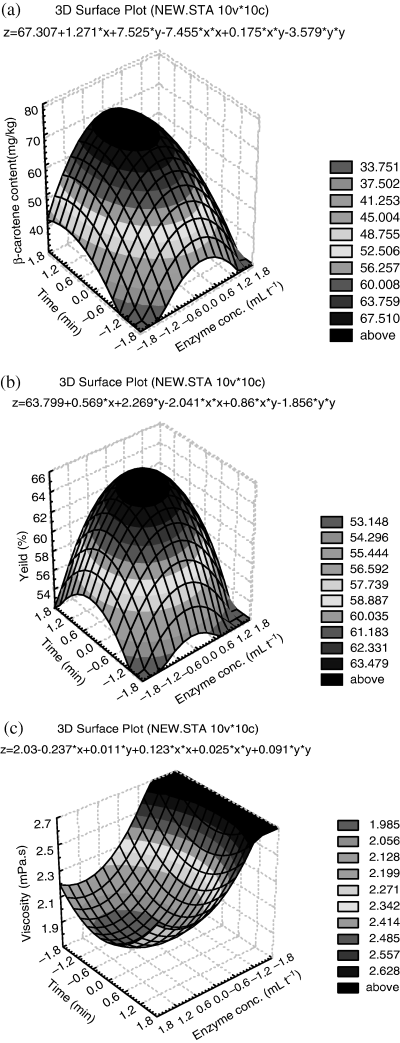
Response surfaces showing effect of enzyme concentration and incubation time on (a) juice β-carotene content, (b) juice yield and (c) juice viscosity.
Optimisation
The graphic optimisation technique is adopted to determine the workable optimum conditions for the enzymatic liquefaction of carrot pulp. This technique dramatically reduces the amount of time and effort required for the investigation of multifactor and multiple-response systems. It also provides comprehensive and informative insight into the system, which leads to a faster optimisation process and may replace other complex statistical analyses. The specification necessary for each of the responses was first set up and also served as constraints on optimisation (Floros & Chinnan, 1988). An acceptable compromise is made based on the following criteria: Y1 ≥ 54.19 mg kg−1, Y2 ≥ 63.479% and Y3 ≤ 2.128 mPa s. The contour plots for each of the responses generated are shown in Fig. 3 and can be compared visually. The contour plots for estimating activity surface over independent variable x1 and x2 are shown in Fig. 3a–c for β-carotene, juice yield and viscosity. The stationary point is the point at which the slope of the response surface is zero in all directions. All the contour plots are elliptical, and the maximum optimum point is attained at the centre as moving along the major and minor axis of ellipse.
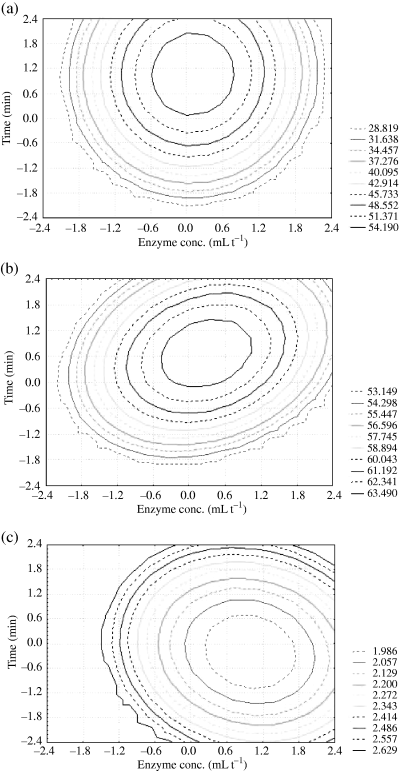
Contour plots showing effect of enzyme concentration and incubation time on (a) juice β-carotene content, (b) juice yield and (c) juice viscosity.
The change in scope of the two variables x1 and x2 affecting the result of the incubation can be observed from contour plots. Figure 3a shows the time at which the enzyme concentration is in the range of −0.6 to 0.7 (70–135 mL t−1) and the incubation time is in the range of 0.1–2.0 (62–100 min), β-carotene content is ≥54.19 mg kg−1. Figure 3b shows the time at which the enzyme concentration is in the range of −0.5 to 1.0 (75–150 mL t−1) and the incubation time is in the range of −0.1–1.4 (58–88 min), the juice yield is ≥63.479%. Figure 3c shows the time at which the enzyme concentration is in the range of −0.3 to 2.3 (85–215 mL t−1) and the incubation time is in the range of −1.5 to 1.3 (30–86 min), the juice viscosity is ≤2.128 mPa s.
A combination of the optimum conditions can be selected. The point at which the enzyme concentration is 100 mL t−1 and incubation time is 80 min can be recommended as the practical optimal condition.
Verification of results
The suitability of the model equation for predicting the optimum response values are tested by using the recommended optimum conditions. This set of conditions are determined to be optimal by the RSM optimisation approach, which is also used to validate experimentally and predict the value of the responses by using model equations. It is because a fractional factorial design was used as the experimental design. A model is considered an adequate one if the predicted values of the model are close to the experimental values observed during the validation tests (Madamda, 2002).Table 8 shows the predicted and observed values for the responses at optimum conditions for enzyme concentration and incubation time, respectively. The experimental values in both cases are averages of three replicates and are very close to the predicted values. Therefore, predictions are within statistically acceptable limits.
Optimum condition . | Coded levels . | Actual levels . | |
---|---|---|---|
Enzyme concentration | 0.0 | 100 mL t−1 | |
Incubation time | 1.0 | 80 min | |
Responses | Predicted value | Experimental value | |
Mean | Range | ||
β-Carotene content (mg kg−1) | 54.19 | 56.88 ± 0.11 | 55.03–57.11 |
Yield (%) | 63.48 | 64.44 ± 0.12 | 64.26–65.32 |
Viscosity (mPa s) | 2.13 | 2.10 ± 0.02 | 2.00–2.20 |
Optimum condition . | Coded levels . | Actual levels . | |
---|---|---|---|
Enzyme concentration | 0.0 | 100 mL t−1 | |
Incubation time | 1.0 | 80 min | |
Responses | Predicted value | Experimental value | |
Mean | Range | ||
β-Carotene content (mg kg−1) | 54.19 | 56.88 ± 0.11 | 55.03–57.11 |
Yield (%) | 63.48 | 64.44 ± 0.12 | 64.26–65.32 |
Viscosity (mPa s) | 2.13 | 2.10 ± 0.02 | 2.00–2.20 |
Optimum condition . | Coded levels . | Actual levels . | |
---|---|---|---|
Enzyme concentration | 0.0 | 100 mL t−1 | |
Incubation time | 1.0 | 80 min | |
Responses | Predicted value | Experimental value | |
Mean | Range | ||
β-Carotene content (mg kg−1) | 54.19 | 56.88 ± 0.11 | 55.03–57.11 |
Yield (%) | 63.48 | 64.44 ± 0.12 | 64.26–65.32 |
Viscosity (mPa s) | 2.13 | 2.10 ± 0.02 | 2.00–2.20 |
Optimum condition . | Coded levels . | Actual levels . | |
---|---|---|---|
Enzyme concentration | 0.0 | 100 mL t−1 | |
Incubation time | 1.0 | 80 min | |
Responses | Predicted value | Experimental value | |
Mean | Range | ||
β-Carotene content (mg kg−1) | 54.19 | 56.88 ± 0.11 | 55.03–57.11 |
Yield (%) | 63.48 | 64.44 ± 0.12 | 64.26–65.32 |
Viscosity (mPa s) | 2.13 | 2.10 ± 0.02 | 2.00–2.20 |
Conclusions
The processing parameters of carrot juice produced from carrot pulp could be optimised by experiments of CCRD and the data could be treated by the software of Statistica version 6.0. Three second-order polynomial models are derived by combinational use of the RSM approach and CCRD. In the β-carotene content model the variable with the largest effect is the quadratic of the enzyme concentration, in the yield model it is the linear term of the incubation time, and in the viscosity model it is the linear term of the enzyme concentration. The study indicated that the two independent variables involved in optimising carrot juice production optimisation are enzyme concentration and incubation time. The three models have high values of the coefficient of determination (R2), which indicates that the models fit the experiment data well. According to the RSM results and the graphic optimisation technique the enzyme concentration of 100 mL t−1 and incubation time of 80 min can be recommended as the practical optimum condition. Correspondingly, juice β-carotene content is ≥54.19 mg kg−1, juice yield is ≥63.479% and juice viscosity is ≤2.128 cP. The validity tests prove that the adequacy of the above models is satisfactory.