-
PDF
- Split View
-
Views
-
Cite
Cite
Tülin Aydemir, Gülay Akkanlı, Partial purification and characterisation of polyphenol oxidase from celery root (Apium graveolens L.) and the investigation of the effects on the enzyme activity of some inhibitors, International Journal of Food Science and Technology, Volume 41, Issue 9, November 2006, Pages 1090–1098, https://doi.org/10.1111/j.1365-2621.2006.01191.x
- Share Icon Share
Abstract
Polyphenol oxidase (PPO) of celery root was extracted and partially purified by (NH4)2SO4 fractionation and dialysis. Optimum pH and temperature were found at pH 7.0 and 30 °C, and Km and Vmax values were 29 mm and 5560 U mL−1 min−1 with catechol, respectively. The activation energy of the enzyme with catechol was 17.9 kJ mol−1 at pH 7.0. In electrophoretic seperation, six isoenzymes were detected with Dl-dopa substrate. PPO showed activity to catechol, 4-methylcatechol, pyrogallol, gallic acid, Dl-dopa. l-Tyrosine was also tested but was not oxidised by celery root PPO. β-Mercaptoethanol was found to be the most effective inhibitor. (NH4)2SO4, NaCl, KCl and sucrose appeared to be protective agents of celery root PPO against thermal denaturation. Metal ions (Cu2+, Zn2+, Mn2+) were poor inhibitors of the celery root PPO at 1 mm. PPO activity was also inhibited by CaCl2, NaCl, BaCl2, FeSO4 and NiCl2.
Introduction
Browning usually occurs in certain fruits and vegetables during handling, processing and storage after harvest. The browning phenomenon is mainly due to the enzymatic oxidation of endogenous phenolic compounds catalysed by polyphenol oxidase (PPO; EC 1.14.18.1) or tyrosinase inherent in biological tissues (Mayer, 1987). PPO, a bifunctional copper-containing enzyme, in the presence of molecular oxygen catalyses the hydroxylation of monophenols to o-diphenols (monophenolase or cresolase activity) and the further oxidation of o-diphenols to o-quinones (diphenolase or catecholase activity). The generated, unstable highly reactive o-quinones subsequently react with themselves, amino acids or proteins, evolving into brown, black or red heterogeneous polymers responsible for quality loss in many foods (Matheis & Whitaker, 1984; Garcia-Carmona et al., 1988).
Physiologically, the function of PPO in plants and fungi is not yet understood, but it may be protective. In higher plants, the enzyme protects the plant against insects and microorganisms by catalysing the formation of an impervious scab of melanin against further attack, as is well documented for the PPO from the trichomes of Solanaceae species (Partington & Bolwell, 1996).
Polyphenol oxidase has been studied in many vegetables and fruits including potato (Chen et al., 1992), cabbage (Fujita et al., 1995), mushroom (Golan-Goldhirsh & Whitaker, 1984), eggplant (Fujita & Tono, 1988), head lettuce (Fujita et al., 1991), pears (Halim & Montgomery, 1978; Flurkey & Jen, 1980), grape (Wissemann & Lee, 1985), avocado (Kahn, 1976), sunflower seed (Raymond et al., 1993), peppermint (Kavrayan & Aydemir, 2001), artichoke head (Aydemir, 2004). However, little research has been reported on the isolation and characterisation of celery root PPO.
In the present work, PPO was isolated from celery root (Apium graveolens L.) and a number of investigations were conducted, mainly with respect to heat and chemical inactivation of the enzyme.
Material and methods
Plant material
Celery root (A. graveolens L.) of marketable quality was harvested from the region of Ege, Turkey (November–March).
Reagents
4-Methylcatechol, catechol, l-dopa, Dl-dopa, gallic acid, dithiotreitol, β-mercaptoethanol, thiourea, glutathione, Tris–HCl, sodium metabisulphite were obtained from Sigma Chemical Co. (St. Louis, MO, USA). Ammonium sulphate, sodium chloride, potassium cyanide, l-tyrosine, l-cysteine, sodium azide were purchased from Merck, Darmstadt, Germany. All other chemicals used were of analytical grade.
Partial purification of polyphenol oxidase
All extraction materials were maintained at low temperature (2–5 °C) to reduce enzymatic activity during extraction. For crude extract, 20 g of celery root tissue was quickly cut into thin slices and extracted with 0.1 m sodium phosphate buffer (pH 7.0). After filtration of the homogenate through cotton cloth, the filtrate was centrifuged at 3500 × g for 20 min. The supernatant was brought to 40% saturation with ammonium sulphate. The precipitate, which was collected by centrifugation as before, was dissolved in a small volume of 0.1 m phosphate buffer (pH 7.0) and dialysed against the same buffer at 4 °C for 3 h. After dialysis, the active fractions were used for characterisation.
Determination of polyphenol oxidase activity
Polyphenol oxidase activity was spectrophotometrically monitored by following the oxidation of substrate (catechol, 420 nm; 4-methylcatechol, 460 nm; pyrogallol, 334 nm; Dl-dopa, 460 nm, gallic acid, 420 nm; l-tyrosine, 480) in 0.2 m phosphate buffer, pH 7.0, at 30 °C.
The sample cuvette contained 0.05 mL of the enzyme and 2.95 mL of substrate solution in various concentrations. In 0.2 m phosphate buffer, the blank sample contained only 3 mL of substrate solution. One unit of PPO activity was defined as the amount of enzyme that caused an increase in the absorbance of 0.001 min−1. PPO activity was assayed in triplicate.
Effect of pH
Polyphenol oxidase activity was determined with five different substrates (catechol, 4-methylcatechol, Dl-dopa, gallic acid, pyrogallol). Appropriate buffers (0.1 m acetate for pH 3.0–5.0, 0.1 m phosphate for pH 5.0–7.0 and 0.1 m Tris–HCl for pH 7.0–10.0 at 30 °C) were used for determining the optimum pH of celery root PPO. The optimum pH values obtained from this assay were used in all the other experiments. The enzyme was preincubated in various buffers (0.1 m acetate for pH 4.0 and 5.0, 0.1 m phosphate for pH 5.0 and 7.0 and 0.1 m Tris–HCl for pH 8.0 and 9.0) to determine the PPO stability at different pH values for 60 min at 30 °C. Residual PPO activity was measured under standard assay conditions.
Effect of temperature on enzyme activity and determination of activation energy
The thermal activity profile was studied with different substrates, a reaction time of 5 min and a temperature range of 5–70 °C. Thermal stability of PPO was determined by heating the enzyme solution at various temperatures between 20 and 70 °C for 60 min at pH 7.0. In addition, to study the effect of additions on heat stability, PPO was incubated in the presence of either salt or sugar. Activation energy (Ea) was determined according to the Arrhenius equation by measuring the maximal initial rate at different temperatures and plotting the logarithmic value of Vmax vs. 1/T (Segel, 1976).
Kinetic studies
The substrate specificity of the enzyme was examined using five chemicals (catechol, 4-methylcatechol, pyrogallol, gallic acid, Dl-dopa) as substrates. Michaelis constants (Km) and maximum reaction velocities (Vmax) were calculated from Lineweaver and Burk plots (1934) using these substrates under various concentrations and in optimum conditions (pH, temperature and ionic strength).
Inhibitor effects
To determine the effects of inhibitors, reactions containing 20 mm catechol and constant amount of enzyme in 0.2 m phosphate buffer (pH 7.0) were run at 30 °C in the presence and absence of inhibitors. All enzyme activities were measured using five different concentrations of the substrate with three constant inhibitor concentrations. Values 1/v and 1/[S] were employed to draw Lineweaver–Burk graphs. Finally, Ki constant values obtained from the graphs. To determine the inhibitor concentration, which reduced the enzyme activity by 50% (I50), regression analysis graphs were drawn, using percent inhibition values, by a statistical package computer program. I50 values were determined from the graphs.
Electrophoresis and gel staining
Non-denaturing polyacrylamide gel electorphoresis (PAGE) was carried out using the method of Laemmli (1970). Crude extract sample was mixed with glycerol and bromophenol blue before being applied to 7.5% polyacrylamide gel. Electrophoresis was performed for 3 h at 4 °C with a current of 25 mA. The gel was stained at 2.5 mmDl-dopa in 0.1 m phosphate buffer pH 8.0. After 1 h of incubation of the gel, isoenzyme bands developed.
Results and discussion
Polyphenol oxidase could be extracted by buffer of low molarity (0.05–0.2 m). The maximum yield was achieved at a concentration of 0.1 m. The optimal pH conditions for PPO extraction were also investigated using different buffers in the pH range 4.0–9.0. The higher specific activity yields as a function of pH were obtained in pH 7.0 phosphate buffer (Fig. 1). Several precipitations with solid (NH4)2SO4 between 20% and 80% were tested to find the proper saturation point. As a result, PPO activity of the precipitate of 40% (NH4)2SO4 saturation was found to be the highest, and this saturation point was used in all the extraction processes. After ammonium sulphate precipitation, the dialysed enzyme extract was used for the characterisation of celery root PPO. Molarity of the reaction buffer was selected as indicated in Table 1. An increase of ionic strength, from 0.05 to 0.2 m of potassium phosphate buffer, produced almost a 20% increase in PPO enzyme activity.
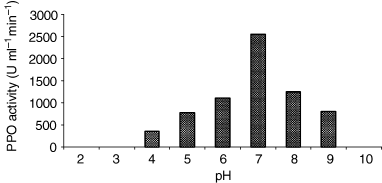
Optimum pH of extraction of celery root PPO. The tissue was extraction 0.1 m NaOAc (pH from 3.0 to 5.0), potassium phosphate (pH from 5.0 to 7.0) and Tris–HCl (pH from 7.0 to 9.0).
Buffer concentrations . | PPO activities (U mL−1 min−1) . |
---|---|
0.05 m Fosfat Tamponu (pH 7.0)a | 1950 |
0.1 m Fosfat Tamponu (pH 7.0)a | 2330 |
0.2 m Fosfat Tamponu (pH 7.0)a | 2582 |
Buffer concentrations . | PPO activities (U mL−1 min−1) . |
---|---|
0.05 m Fosfat Tamponu (pH 7.0)a | 1950 |
0.1 m Fosfat Tamponu (pH 7.0)a | 2330 |
0.2 m Fosfat Tamponu (pH 7.0)a | 2582 |
aThe pH 7.0 was selected by a previous pH study of optimal conditions for spectrophotometric PPO assay.
Buffer concentrations . | PPO activities (U mL−1 min−1) . |
---|---|
0.05 m Fosfat Tamponu (pH 7.0)a | 1950 |
0.1 m Fosfat Tamponu (pH 7.0)a | 2330 |
0.2 m Fosfat Tamponu (pH 7.0)a | 2582 |
Buffer concentrations . | PPO activities (U mL−1 min−1) . |
---|---|
0.05 m Fosfat Tamponu (pH 7.0)a | 1950 |
0.1 m Fosfat Tamponu (pH 7.0)a | 2330 |
0.2 m Fosfat Tamponu (pH 7.0)a | 2582 |
aThe pH 7.0 was selected by a previous pH study of optimal conditions for spectrophotometric PPO assay.
The effects of pH and temperature on PPO activity
pH optima for celery root PPO was found to be 7.0 using catechol, pyrogallol, 4-methylcatechol and Dl-dopa as substrates. Widely different pH optima have been reported for various plant PPOs. The pH optima of Royal Ann cherries (Benjamin & Montgomery, 1973), guava (Augustin et al., 1985) and allium sp. (Arslan et al., 1997) PPO for catechol were 7.0, 7.2 and 7.5, respectively. On the contrary, using gallic acid as the substrate, the optimal pH was found to be acidic (pH 5.0) for celery root PPO. Similar results have also been reported for potato, Japanese pear and eggplant. (Balasingam & Ferdinand, 1970; Tono et al., 1986, Fujita & Tono, 1988).
Alyward & Haisman (1965) reported that the optimum pH for maximum PPO activity in plants varies from about 4.0 to 7.0, depending on extraction methods, the substrates used for assay and localisation of the enzyme in the plant cell.
Below and above pH 7.0 the activity decreased rapidly, and at pH 4.0 and 9.0 the enzyme retained 37% and 43% of the activity observed at optimum pH, respectively.
The effect of pH on stability was also determined (Fig. 2). The enzyme showed stability at pH 7.0, where as at pH 4.0 and 9.0, using catechol as the substrate, an activity loss of about 50% occurred after 17 min and 27 min incubation at 30 °C, respectively. The optimum temperature for maximal PPO activity was 30 °C; above 40 °C the activity declined very rapidly as the temperature increased. This result was similar to that of the other optimum temperatures reported for grape (Yokotsuka et al., 1988), artichoke heads (Aydemir, 2004) and peppermint PPO (Kavrayan & Aydemir, 2001), which is between 25 and 30 °C. Study of heat stability of enzyme indicated that about 50% inactivation occurred with a treatment of 1 min at 60 °C and total inactivation could be achieved in 4 min at 70 °C (Fig. 3).
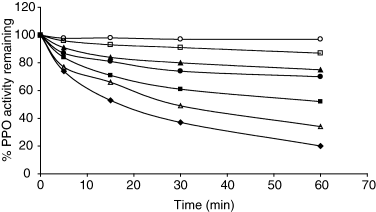
pH stability of celery root PPO with catechol as substrate. pH 4.0 (♦), pH 5.0 (■), pH 6.0 (▲), pH 7.0 (○), pH 7.5 (□), pH 8.0 (●), pH 9.0 (Δ).
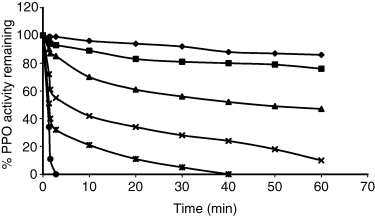
Heat inactivation of celery root PPO at various temperatures with catechol as substrate. 20 °C (♦), 30 °C (■), 40 °C (▲), 50 °C (×), 60 °C (*), 70 °C (●).
Ea value for celery root PPO with catechol substrate was found to be 17.9 kJ mol−1. This value was similar to that of banana (18.6 kJ mol−1) (Palmer, 1963), kiwi fruit (17.0 kJ mol−1) (Park & Luh, 1985) and wild rice (20.0 kJ mol−1) (Owasa-Ansak, 1989).
Effect of sucrose and salts
It has been recognised that polyhydric alcohols and sugars protect the native conformation of proteins against thermal denaturation, which explains the stabilising effect of sucrose (Lee & Timasheff, 1981; Arakawa & Timasheff, 1982a,b). In contrast to this stabilising effect, sugars have been noted to increase and decrease kinetic parameters, suggesting that protein conformation is effected (Hinton et al., 1969; Yoovidhya et al., 1986). This implies that stabilisation of an enzyme against unfolding may not necessarily mean stabilisation of an enzyme against thermal inactivation.
To investigate the effect of sucrose on thermal stability of celery root PPO, the enzyme mixture was heated in the presence of 20–40% sucrose solution. Figure 4 shows the effect of sucrose at 20% and 40% (w/w) on thermal inactivation of PPO at 50 and 60 °C. The data shown in Fig. 4 reveal that activity was more stable when PPO was heated in the presence 40% of sucrose. In this case, PPO retained 50% of the original activity after 5 min of heating at 60 °C compared with a 30% value obtained in the absence of sugar. In contrast, the enzyme heated in 20% sucrose was not markedly protected by sucrose.

Heat inactivation of celery root PPO in the presence of sucrose at 50 and 60 °C heating at different temperatures; the enzyme solution was rapidly cooled in ice and remaining activity was assayed with catechol as substrate at 30 °C. 50° C, control (○), sucrose concentrations: 20% (Δ), 40% (□); 60° C, control (●), sucrose concentrations: 20% (▲), 40% (■).
Back et al. (1979) suggested that sucrose stabilises the protein by increasing the structure of water which, in turn, increases hydrophobic interaction. Sucrose also increases the surface tension with solvent components (Lee & Timasheff, 1981; Arakawa & Timasheff, 1982a). The conformation, stability and solubility of proteins are sensitive functions of solvent composition. The effect of salts on these properties of proteins in aqueous solution is a strong function of the ionic species present.
The effect of salts on heat inactivation of PPO is shown in Fig. 5. Among the investigated, a series ranking from most to least effective was as follows: (NH4)2SO4 > Na2SO4 > NaCl > KCl.
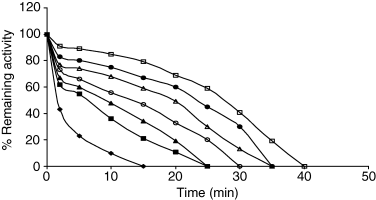
Heat inactivation of celery root PPO in the presence of salts and heating at different temperatures. Control, (♦); salt concentrations: 1 m KCl (■), 1 m NaCl (▲), 1 m Na2SO4 (●), 2 m NaCl (Δ), 2 m KCl (○), 1 m (NH4)2SO4 (□).
The results show that the protective effect on PPO activity depends on the concentration and nature of the salts. It was also observed that at identical concentrations Na2SO4 was more effective than NaCl in protecting the enzyme activity. As can be seen from Fig. 5, when comparing Na2SO4 and (NH4)2SO4 at the same concentrations, it has been found that (NH4)2SO4 is stronger than Na2SO4 against heat inactivation.
Previous studies have shown that a knowledge of preferential interactions of solvent components with proteins in three-component systems can lead to an understanding of the mechanism of the effect of solvent components on the stability and solubility of proteins (Lee & Timasheff, 1981; Arakawa & Timasheff, 1982a,b) and also, in investigating the various salt effects on proteins, can be related to the preferential interaction patterns of salts with proteins.
Recently, Melander & Horvath (1977) have concluded on the basis of the cavity theory that the solubility of proteins in aqueous salt solutions can be corrected with the surface tension increment of water induced by the addition of salts.
Kunt & Kauzman (1974) have reviewed the available data on the effect of salt on protein hydration, but the information is insufficient to interpret the widely different salt effects in terms of preferential interactions.
The effect of salts on protein stability depends on the concentration and ionic strength of the salts. At low salt concentration, the stabilising effect of electrolytes on protein conformation has been attributed to an electrostatic response. At high salt concentration, the ability of salts to stabilise protein structure has been related to the preferential hydration of the protein molecule, as a result of a salt-induced alteration of the water structure in the vicinity of the protein and can be referred to as a lyotropic effect (Arakawa & Timasheff, 1982b).
Substrate specificity
The substrate specificity of the partially purified celery root PPO is similar to most reported plant PPOs, which markedly oxidase o-diphenols such as catechol and 4-methylcatechol (Rivas & Whitaker, 1973; Kahn, 1976; Park & Luh, 1985; Wissemann & Lee, 1985a; Fujita & Tono, 1988; Augustin et al., 1985; Fujita et al., 1991; Murata et al., 1992).
Celery root PPO showed no activity towards tyrosine (the monophenols), suggesting the absence the monophenolase (cresolase) activity. This result is similar to those reported for d'Anjou (Halim & Montgomery, 1978) and yali pear PPO (Zhou & Feng, 1991).
The Km values of the enzyme for catechol, 4-methylcatechol, pyrogallol, gallic acid and Dl-dopa, determined according to the method of Lineweaver–Burk (1934), were found to be 29, 42, 50, 40, 56 mm, respectively (Table 2). The celery root Km value for catechol substrate is similar to those previously reported for PPO from other sources: Amasya apple (34 mm) (Oktay et al., 1995), Stanley plum (20 mm) (Siddig et al., 1992), peach (29 mm) (Jen & Kahler, 1974), pear (22.7 mm) (Siddig et al., 1994).
Optimum pH, temperature, Km and Vmax values of the polyphenol oxidase with different substrates
Substrate . | Optimum pH . | Optimum temperature (°C) . | Km (M) . | Vmax (U mL−1 min−1) . |
---|---|---|---|---|
Catechol | 7.0 | 30 | 29 × 10−3 | 5560 |
4-Methylcatechol | 7.0 | 30 | 42 × 10−3 | 3500 |
Dl-Dopa | 7.0 | 30 | 56 × 10−3 | 1100 |
Pyrogallol | 7.0 | 30 | 50 × 10−3 | 5000 |
Gallic acid | 5.0 | 35 | 40 × 10−3 | 1687 |
Substrate . | Optimum pH . | Optimum temperature (°C) . | Km (M) . | Vmax (U mL−1 min−1) . |
---|---|---|---|---|
Catechol | 7.0 | 30 | 29 × 10−3 | 5560 |
4-Methylcatechol | 7.0 | 30 | 42 × 10−3 | 3500 |
Dl-Dopa | 7.0 | 30 | 56 × 10−3 | 1100 |
Pyrogallol | 7.0 | 30 | 50 × 10−3 | 5000 |
Gallic acid | 5.0 | 35 | 40 × 10−3 | 1687 |
Optimum pH, temperature, Km and Vmax values of the polyphenol oxidase with different substrates
Substrate . | Optimum pH . | Optimum temperature (°C) . | Km (M) . | Vmax (U mL−1 min−1) . |
---|---|---|---|---|
Catechol | 7.0 | 30 | 29 × 10−3 | 5560 |
4-Methylcatechol | 7.0 | 30 | 42 × 10−3 | 3500 |
Dl-Dopa | 7.0 | 30 | 56 × 10−3 | 1100 |
Pyrogallol | 7.0 | 30 | 50 × 10−3 | 5000 |
Gallic acid | 5.0 | 35 | 40 × 10−3 | 1687 |
Substrate . | Optimum pH . | Optimum temperature (°C) . | Km (M) . | Vmax (U mL−1 min−1) . |
---|---|---|---|---|
Catechol | 7.0 | 30 | 29 × 10−3 | 5560 |
4-Methylcatechol | 7.0 | 30 | 42 × 10−3 | 3500 |
Dl-Dopa | 7.0 | 30 | 56 × 10−3 | 1100 |
Pyrogallol | 7.0 | 30 | 50 × 10−3 | 5000 |
Gallic acid | 5.0 | 35 | 40 × 10−3 | 1687 |
Isozyme profile
To elucidate the presence of isoenzymes in the crude extract, a native PAGE was performed and the gel was stained with Dl-dopa. The crude enzyme solution revealed the presence of six bands (Fig. 6). Park & Luh (1985) reported four forms of PPO as isozymes in kiwi fruit. Wong et al. (1971) reported the existence of two types of PPO in clingstone peach.

Native PAGE of celery root PPO. Activity staining by 2.5 mmDl-dopa.
Storage stability
The effect of different storage temperatures on partially purified celery root PPO was studied over a 25 day period at pH 7.0 in 0.1 m phosphate buffer.
At 25 °C, the enzyme completely lost its activity after 5 days. At 4 °C, 50% loss in PPO activity was observed during the first 3 days, however, at the end of 10 days more than 90% activity was lost. The enzyme was more stable at −15 °C; it lost only 20% enzyme activity after storage for 7 days and was completely inactivated after 25 days.
Kavrayan & Aydemir (2001) reported that Mentha piperita PPO enzyme completely lost activity after 21 days at −15 °C. Nagai & Suzuki (2001) reported that cabbage PPO enzyme completely lost activity after 14 days at 4 °C.
Inhibitors
The effect of various inhibitors on celery root PPO obtained, after (NH4)2SO4 precipitation and dialysed with catechol as substrate, was determined (Table 3). Several compounds reported as PPO inhibitors (Halim & Montgomery, 1978; Anosike & Ayaebene, 1981; Raymond et al., 1993) were also shown to have an inhibitory effect on the celery root PPO.
. | I (M) . | Ki (M) . | Type of inhibition . | % Inhibition . | I50 . |
---|---|---|---|---|---|
Citric acid | 2.0 × 10−2 | 3.25 × 10−2 | Competitive | 35 | 2.63 × 10−2 |
2.5 × 10−2 | 1.20 × 10−2 | 52 | |||
3.0 × 10−3 | 8.60 × 10−3 | 68 | |||
Sodium metabisulphite | 3.3 × 10−5 | 6.67 × 10−5 | Non-competitive | 20 | 5.05 × 10−5 |
1.7 × 10−4 | 2.00 × 10−5 | 55 | |||
1.0 × 10−4 | 2.23 × 10−5 | 87 | |||
Potassium cyanide | 1.7 × 10−5 | 12.0 × 10−6 | Competitive | 33 | 2.8 × 10−5 |
3.3 × 10−5 | 9.00 × 10−6 | 52 | |||
6.7 × 10−5 | 8.46 × 10−6 | 63 | |||
Glutathione | 3.3 × 10−5 | 6.60 × 10−5 | Non-competitive | 25 | 7.4 × 10−5 |
6.7 × 10−5 | 4.76 × 10−5 | 45 | |||
1.0 × 10−4 | 3.23 × 10−5 | 62 | |||
Dithiotreitol | 1.3 × 10−5 | 7.70 × 10−6 | Non-competitive | 20 | 1.52 × 10−5 |
2.0 × 10−5 | 5.64 × 10−6 | 52 | |||
3.3 × 10−5 | 5.34 × 10−6 | 70 | |||
Sodium azide | 3.3 × 10−5 | 5.26 × 10−5 | Non-competitive | 21 | 9.47 × 10−5 |
6.7 × 10−5 | 4.50 × 10−5 | 44 | |||
1.0 × 10−4 | 4.10 × 10−5 | 64 | |||
Thiourea | 3.3 × 10−5 | 1.57 × 10−4 | Competitive | 16 | 6.97 × 10−5 |
6.7 × 10−5 | 7.71 × 10−5 | 48 | |||
1.0 × 10−4 | 7.00 × 10−4 | 69 | |||
Oxalic acid | 6.7 × 10−5 | 1.56 × 10−4 | Competitive | 14 | 1.62 × 10−4 |
1.3 × 10−4 | 1.29 × 10−4 | 40 | |||
2.0 × 10−4 | 1.18 × 10−4 | 71 | |||
Sodium thiosulphite | 1.7 × 10−5 | 3.20 × 10−5 | Competitive | 19 | 2.61 × 10−5 |
2.3 × 10−5 | 1.64 × 10−5 | 44 | |||
3.3 × 10−5 | 0.64 × 10−5 | 64 | |||
Tartaric acid | 6.7 × 10−3 | 1.60 × 10−2 | Non-competitive | 32 | 7.83 × 10−3 |
8.3 × 10−3 | 9.10 × 10−3 | 53 | |||
1.0 × 10−2 | 7.10 × 10−3 | 60 | |||
β-Mercaptoethanol | 3.3 × 10−6 | 5.36 × 10−6 | Non-competitive | 20 | 7.61 × 10−6 |
6.7 × 10−6 | 4.40 × 10−6 | 44 | |||
1.0 × 10−5 | 3.50 × 10−6 | 72 | |||
Ascorbic acid | 3.3 × 10−5 | 7.33 × 10−5 | Non-competitive | 25 | 5.10 × 10−5 |
5.0 × 10−5 | 4.24 × 10−5 | 49 | |||
6.7 × 10−5 | 2.94 × 10−5 | 64 | |||
EDTA | 3.3 × 10−4 | 5.40 × 10−4 | Competitive | 38 | 4.54 × 10−4 |
5.0 × 10−4 | 3.51 × 10−4 | 55 | |||
6.7 × 10−4 | 2.70 × 10−4 | 67 | |||
l-Cysteine | 3.3 × 10−5 | 6.8 × 10−5 | Competitive | 38 | 5.5 × 10−5 |
5.0 × 10−5 | 2.8 × 10−5 | 55 | |||
6.6 × 10−5 | 1.1 × 10−5 | 76 |
. | I (M) . | Ki (M) . | Type of inhibition . | % Inhibition . | I50 . |
---|---|---|---|---|---|
Citric acid | 2.0 × 10−2 | 3.25 × 10−2 | Competitive | 35 | 2.63 × 10−2 |
2.5 × 10−2 | 1.20 × 10−2 | 52 | |||
3.0 × 10−3 | 8.60 × 10−3 | 68 | |||
Sodium metabisulphite | 3.3 × 10−5 | 6.67 × 10−5 | Non-competitive | 20 | 5.05 × 10−5 |
1.7 × 10−4 | 2.00 × 10−5 | 55 | |||
1.0 × 10−4 | 2.23 × 10−5 | 87 | |||
Potassium cyanide | 1.7 × 10−5 | 12.0 × 10−6 | Competitive | 33 | 2.8 × 10−5 |
3.3 × 10−5 | 9.00 × 10−6 | 52 | |||
6.7 × 10−5 | 8.46 × 10−6 | 63 | |||
Glutathione | 3.3 × 10−5 | 6.60 × 10−5 | Non-competitive | 25 | 7.4 × 10−5 |
6.7 × 10−5 | 4.76 × 10−5 | 45 | |||
1.0 × 10−4 | 3.23 × 10−5 | 62 | |||
Dithiotreitol | 1.3 × 10−5 | 7.70 × 10−6 | Non-competitive | 20 | 1.52 × 10−5 |
2.0 × 10−5 | 5.64 × 10−6 | 52 | |||
3.3 × 10−5 | 5.34 × 10−6 | 70 | |||
Sodium azide | 3.3 × 10−5 | 5.26 × 10−5 | Non-competitive | 21 | 9.47 × 10−5 |
6.7 × 10−5 | 4.50 × 10−5 | 44 | |||
1.0 × 10−4 | 4.10 × 10−5 | 64 | |||
Thiourea | 3.3 × 10−5 | 1.57 × 10−4 | Competitive | 16 | 6.97 × 10−5 |
6.7 × 10−5 | 7.71 × 10−5 | 48 | |||
1.0 × 10−4 | 7.00 × 10−4 | 69 | |||
Oxalic acid | 6.7 × 10−5 | 1.56 × 10−4 | Competitive | 14 | 1.62 × 10−4 |
1.3 × 10−4 | 1.29 × 10−4 | 40 | |||
2.0 × 10−4 | 1.18 × 10−4 | 71 | |||
Sodium thiosulphite | 1.7 × 10−5 | 3.20 × 10−5 | Competitive | 19 | 2.61 × 10−5 |
2.3 × 10−5 | 1.64 × 10−5 | 44 | |||
3.3 × 10−5 | 0.64 × 10−5 | 64 | |||
Tartaric acid | 6.7 × 10−3 | 1.60 × 10−2 | Non-competitive | 32 | 7.83 × 10−3 |
8.3 × 10−3 | 9.10 × 10−3 | 53 | |||
1.0 × 10−2 | 7.10 × 10−3 | 60 | |||
β-Mercaptoethanol | 3.3 × 10−6 | 5.36 × 10−6 | Non-competitive | 20 | 7.61 × 10−6 |
6.7 × 10−6 | 4.40 × 10−6 | 44 | |||
1.0 × 10−5 | 3.50 × 10−6 | 72 | |||
Ascorbic acid | 3.3 × 10−5 | 7.33 × 10−5 | Non-competitive | 25 | 5.10 × 10−5 |
5.0 × 10−5 | 4.24 × 10−5 | 49 | |||
6.7 × 10−5 | 2.94 × 10−5 | 64 | |||
EDTA | 3.3 × 10−4 | 5.40 × 10−4 | Competitive | 38 | 4.54 × 10−4 |
5.0 × 10−4 | 3.51 × 10−4 | 55 | |||
6.7 × 10−4 | 2.70 × 10−4 | 67 | |||
l-Cysteine | 3.3 × 10−5 | 6.8 × 10−5 | Competitive | 38 | 5.5 × 10−5 |
5.0 × 10−5 | 2.8 × 10−5 | 55 | |||
6.6 × 10−5 | 1.1 × 10−5 | 76 |
. | I (M) . | Ki (M) . | Type of inhibition . | % Inhibition . | I50 . |
---|---|---|---|---|---|
Citric acid | 2.0 × 10−2 | 3.25 × 10−2 | Competitive | 35 | 2.63 × 10−2 |
2.5 × 10−2 | 1.20 × 10−2 | 52 | |||
3.0 × 10−3 | 8.60 × 10−3 | 68 | |||
Sodium metabisulphite | 3.3 × 10−5 | 6.67 × 10−5 | Non-competitive | 20 | 5.05 × 10−5 |
1.7 × 10−4 | 2.00 × 10−5 | 55 | |||
1.0 × 10−4 | 2.23 × 10−5 | 87 | |||
Potassium cyanide | 1.7 × 10−5 | 12.0 × 10−6 | Competitive | 33 | 2.8 × 10−5 |
3.3 × 10−5 | 9.00 × 10−6 | 52 | |||
6.7 × 10−5 | 8.46 × 10−6 | 63 | |||
Glutathione | 3.3 × 10−5 | 6.60 × 10−5 | Non-competitive | 25 | 7.4 × 10−5 |
6.7 × 10−5 | 4.76 × 10−5 | 45 | |||
1.0 × 10−4 | 3.23 × 10−5 | 62 | |||
Dithiotreitol | 1.3 × 10−5 | 7.70 × 10−6 | Non-competitive | 20 | 1.52 × 10−5 |
2.0 × 10−5 | 5.64 × 10−6 | 52 | |||
3.3 × 10−5 | 5.34 × 10−6 | 70 | |||
Sodium azide | 3.3 × 10−5 | 5.26 × 10−5 | Non-competitive | 21 | 9.47 × 10−5 |
6.7 × 10−5 | 4.50 × 10−5 | 44 | |||
1.0 × 10−4 | 4.10 × 10−5 | 64 | |||
Thiourea | 3.3 × 10−5 | 1.57 × 10−4 | Competitive | 16 | 6.97 × 10−5 |
6.7 × 10−5 | 7.71 × 10−5 | 48 | |||
1.0 × 10−4 | 7.00 × 10−4 | 69 | |||
Oxalic acid | 6.7 × 10−5 | 1.56 × 10−4 | Competitive | 14 | 1.62 × 10−4 |
1.3 × 10−4 | 1.29 × 10−4 | 40 | |||
2.0 × 10−4 | 1.18 × 10−4 | 71 | |||
Sodium thiosulphite | 1.7 × 10−5 | 3.20 × 10−5 | Competitive | 19 | 2.61 × 10−5 |
2.3 × 10−5 | 1.64 × 10−5 | 44 | |||
3.3 × 10−5 | 0.64 × 10−5 | 64 | |||
Tartaric acid | 6.7 × 10−3 | 1.60 × 10−2 | Non-competitive | 32 | 7.83 × 10−3 |
8.3 × 10−3 | 9.10 × 10−3 | 53 | |||
1.0 × 10−2 | 7.10 × 10−3 | 60 | |||
β-Mercaptoethanol | 3.3 × 10−6 | 5.36 × 10−6 | Non-competitive | 20 | 7.61 × 10−6 |
6.7 × 10−6 | 4.40 × 10−6 | 44 | |||
1.0 × 10−5 | 3.50 × 10−6 | 72 | |||
Ascorbic acid | 3.3 × 10−5 | 7.33 × 10−5 | Non-competitive | 25 | 5.10 × 10−5 |
5.0 × 10−5 | 4.24 × 10−5 | 49 | |||
6.7 × 10−5 | 2.94 × 10−5 | 64 | |||
EDTA | 3.3 × 10−4 | 5.40 × 10−4 | Competitive | 38 | 4.54 × 10−4 |
5.0 × 10−4 | 3.51 × 10−4 | 55 | |||
6.7 × 10−4 | 2.70 × 10−4 | 67 | |||
l-Cysteine | 3.3 × 10−5 | 6.8 × 10−5 | Competitive | 38 | 5.5 × 10−5 |
5.0 × 10−5 | 2.8 × 10−5 | 55 | |||
6.6 × 10−5 | 1.1 × 10−5 | 76 |
. | I (M) . | Ki (M) . | Type of inhibition . | % Inhibition . | I50 . |
---|---|---|---|---|---|
Citric acid | 2.0 × 10−2 | 3.25 × 10−2 | Competitive | 35 | 2.63 × 10−2 |
2.5 × 10−2 | 1.20 × 10−2 | 52 | |||
3.0 × 10−3 | 8.60 × 10−3 | 68 | |||
Sodium metabisulphite | 3.3 × 10−5 | 6.67 × 10−5 | Non-competitive | 20 | 5.05 × 10−5 |
1.7 × 10−4 | 2.00 × 10−5 | 55 | |||
1.0 × 10−4 | 2.23 × 10−5 | 87 | |||
Potassium cyanide | 1.7 × 10−5 | 12.0 × 10−6 | Competitive | 33 | 2.8 × 10−5 |
3.3 × 10−5 | 9.00 × 10−6 | 52 | |||
6.7 × 10−5 | 8.46 × 10−6 | 63 | |||
Glutathione | 3.3 × 10−5 | 6.60 × 10−5 | Non-competitive | 25 | 7.4 × 10−5 |
6.7 × 10−5 | 4.76 × 10−5 | 45 | |||
1.0 × 10−4 | 3.23 × 10−5 | 62 | |||
Dithiotreitol | 1.3 × 10−5 | 7.70 × 10−6 | Non-competitive | 20 | 1.52 × 10−5 |
2.0 × 10−5 | 5.64 × 10−6 | 52 | |||
3.3 × 10−5 | 5.34 × 10−6 | 70 | |||
Sodium azide | 3.3 × 10−5 | 5.26 × 10−5 | Non-competitive | 21 | 9.47 × 10−5 |
6.7 × 10−5 | 4.50 × 10−5 | 44 | |||
1.0 × 10−4 | 4.10 × 10−5 | 64 | |||
Thiourea | 3.3 × 10−5 | 1.57 × 10−4 | Competitive | 16 | 6.97 × 10−5 |
6.7 × 10−5 | 7.71 × 10−5 | 48 | |||
1.0 × 10−4 | 7.00 × 10−4 | 69 | |||
Oxalic acid | 6.7 × 10−5 | 1.56 × 10−4 | Competitive | 14 | 1.62 × 10−4 |
1.3 × 10−4 | 1.29 × 10−4 | 40 | |||
2.0 × 10−4 | 1.18 × 10−4 | 71 | |||
Sodium thiosulphite | 1.7 × 10−5 | 3.20 × 10−5 | Competitive | 19 | 2.61 × 10−5 |
2.3 × 10−5 | 1.64 × 10−5 | 44 | |||
3.3 × 10−5 | 0.64 × 10−5 | 64 | |||
Tartaric acid | 6.7 × 10−3 | 1.60 × 10−2 | Non-competitive | 32 | 7.83 × 10−3 |
8.3 × 10−3 | 9.10 × 10−3 | 53 | |||
1.0 × 10−2 | 7.10 × 10−3 | 60 | |||
β-Mercaptoethanol | 3.3 × 10−6 | 5.36 × 10−6 | Non-competitive | 20 | 7.61 × 10−6 |
6.7 × 10−6 | 4.40 × 10−6 | 44 | |||
1.0 × 10−5 | 3.50 × 10−6 | 72 | |||
Ascorbic acid | 3.3 × 10−5 | 7.33 × 10−5 | Non-competitive | 25 | 5.10 × 10−5 |
5.0 × 10−5 | 4.24 × 10−5 | 49 | |||
6.7 × 10−5 | 2.94 × 10−5 | 64 | |||
EDTA | 3.3 × 10−4 | 5.40 × 10−4 | Competitive | 38 | 4.54 × 10−4 |
5.0 × 10−4 | 3.51 × 10−4 | 55 | |||
6.7 × 10−4 | 2.70 × 10−4 | 67 | |||
l-Cysteine | 3.3 × 10−5 | 6.8 × 10−5 | Competitive | 38 | 5.5 × 10−5 |
5.0 × 10−5 | 2.8 × 10−5 | 55 | |||
6.6 × 10−5 | 1.1 × 10−5 | 76 |
Sulphhydryl compounds have been shown to be effective in the control of enzymatic browning in fruit products. Several thiol-containing compounds such as l-cysteine, glutathione, β-mercaptoethanol and thiourea have been investigated as inhibitors of enzymatic browning (Halim & Montgomery, 1978; Golan-Goldhirsh & Whitaker, 1984). The result from inhibitor studies in other plant tissues showed the thiol reagents as the most effective inhibitors for those enzymes. Similar PPO inhibition by thiol compounds were recorded, both Aydemir (2004) and Lourenco et al. (1992) for artichoke heads PPO and sweet potato PPO. Golan-Goldhirsh & Whitaker (1984) observed a direct inhibition by thiol-containing compounds and have shown changes in the electrophoretic pattern of PPO after incubation with thiol. l-Cysteine is the most effective in this group (Dudley & Hotchkiss, 1989; Molnar-Perl & Friedman, 1990a,b; Richard-Forget et al., 1992; Özoǧlu & Bayındırlı, 2002). The action of cysteine is complex. Sulphhydryl-containing amino acids like l-cysteine prevent brown pigment formation by reacting with the quinone intermediates to form stable colourless compounds. Cysteine–quinone adducts have proved to be competitive inhibitors of PPO. Direct inhibition of PPO by cysteine, through the formation of stable complexes with copper, has also been proposed (Nicholas et al., 1994).
NaN3 was able to inhibit the celery root PPO.
Ascorbic acid is the most frequently used chemical product for the chemical reduction of o-quinones back into o-diphenolic compounds for the browning control of fruits and vegetable products. But, its effect is temporary (Nicholas et al., 1994; Özoǧlu & Bayındırlı, 2002). A Lineweaver–Burk (1934) plot for celery root PPO reaction using different concentrations of catechol in the absence and presence of different concentrations of ascorbic acid was typical of a non-competitive inhibition. The calculated inhibitor dissociation constant (Kiaverage) was 4.83 × 10−5 m.
The inhibition of celery root PPO with sodium metabisulphite was also non-competitive according to the Lineweaver–Burk plot. Sodium metabisulphite can act as a reducing agent for o-benzoquinones (Wong et al., 1971).
This effect decreases the observed extent of oxidation of o-phenols, as measured at 420 nm, and was dependent on inhibitor concentration. Embs & Markakis (1965) showed that sodium metabisulphite acted as inhibitor of mushroom PPO through binding to the o-quinones, thus preventing the formation of melanin. Although the bisulphites are effective in preventing browning, they could be harmful to human health, especially in asthmatic patients (FDA, 1986, 1987).
Six amino acids, l-aspartic acid, l-glutamic acid, l-arginine, l-phenylalanine, l-cysteine and glycine, were assayed as possible inhibitors of celery root PPO with catechol as substrate. Among the amino acids chosen, l-cysteine was the most effective inhibitor. The most recent evidence that PPO is inhibited by proteins, peptides and amino acids was obtained by Kahn (1985) for the enzyme from mushroom, avocado and banana. In addition, Kahn reported that l-phenylalanine, l-histidine, glycine and l-lysine, in decreasing order of effectiveness, inhibited the dopa oxidation by mushroom tyrosinase. Proteins and amino acids can affect PPO activity by reacting with o-quinone products of PPO activity and by chelating the essential copper at the active site of PPO. Mason & Peterson (1965) reported that N-terminal primary amino group, aliphatic amino groups and thiol-containing amino acids react with o-quinones to give red or brown products. In addition, certain amino acids are able to form stable complexes with Cu2+ at the active site of PPO and hence make the enzyme ineffective (Mathew & Parpia, 1971; Kahn, 1985).
The effects of various compounds on the partially purified celery root PPO activity are listed in Table 4. The enzyme was markedly inhibited by CoNO3, Hg(CH3COO)2, SnCl2, BaCl2. These compounds also inhibited PPO in head lettuce (Lactuca sativa) (Fujita et al., 1991). CuSO4, ZnSO4, NH4Cl and MnCl2 were poor inhibitors of the enzyme at 1 mm.
Compounds . | Relative activity (%) . | |
---|---|---|
1 mMa . | 10 mMa . | |
None | 100 | 100 |
(NH4)2SO4 | 104 | 100 |
CuSO4 | 96 | 66 |
ZnSO4 | 98 | 71 |
CoNO3 | 41 | 5 |
CaCl2 | 65 | 40 |
BaCl2 | 71 | 21 |
NaF | 71 | 53 |
Hg(CH3COO)2 | 45 | 13 |
FeSO4 | 88 | 35 |
NaCl | 85 | 75 |
NH4Cl | 94 | 57 |
NiCl2 | 80 | 49 |
MnCl2 | 97 | 70 |
SnCl2 | 0 | 0 |
Compounds . | Relative activity (%) . | |
---|---|---|
1 mMa . | 10 mMa . | |
None | 100 | 100 |
(NH4)2SO4 | 104 | 100 |
CuSO4 | 96 | 66 |
ZnSO4 | 98 | 71 |
CoNO3 | 41 | 5 |
CaCl2 | 65 | 40 |
BaCl2 | 71 | 21 |
NaF | 71 | 53 |
Hg(CH3COO)2 | 45 | 13 |
FeSO4 | 88 | 35 |
NaCl | 85 | 75 |
NH4Cl | 94 | 57 |
NiCl2 | 80 | 49 |
MnCl2 | 97 | 70 |
SnCl2 | 0 | 0 |
aFinal concentration of various compounds.
Compounds . | Relative activity (%) . | |
---|---|---|
1 mMa . | 10 mMa . | |
None | 100 | 100 |
(NH4)2SO4 | 104 | 100 |
CuSO4 | 96 | 66 |
ZnSO4 | 98 | 71 |
CoNO3 | 41 | 5 |
CaCl2 | 65 | 40 |
BaCl2 | 71 | 21 |
NaF | 71 | 53 |
Hg(CH3COO)2 | 45 | 13 |
FeSO4 | 88 | 35 |
NaCl | 85 | 75 |
NH4Cl | 94 | 57 |
NiCl2 | 80 | 49 |
MnCl2 | 97 | 70 |
SnCl2 | 0 | 0 |
Compounds . | Relative activity (%) . | |
---|---|---|
1 mMa . | 10 mMa . | |
None | 100 | 100 |
(NH4)2SO4 | 104 | 100 |
CuSO4 | 96 | 66 |
ZnSO4 | 98 | 71 |
CoNO3 | 41 | 5 |
CaCl2 | 65 | 40 |
BaCl2 | 71 | 21 |
NaF | 71 | 53 |
Hg(CH3COO)2 | 45 | 13 |
FeSO4 | 88 | 35 |
NaCl | 85 | 75 |
NH4Cl | 94 | 57 |
NiCl2 | 80 | 49 |
MnCl2 | 97 | 70 |
SnCl2 | 0 | 0 |
aFinal concentration of various compounds.
Polyphenol oxidase was also inhibited by CaCl2, NaCl, BaCl2, FeSO4 and NiCl2. Similar effects of these compounds were found on purified PPO from eggplant (Solanum melongema) (Fujita & Tono, 1988).