-
PDF
- Split View
-
Views
-
Cite
Cite
Kaiyue Su, Ningning Lin, Shouqiang Xie, Yabo Han, Zaiming Yang, Hongmin Zhang, Hongpeng He, S a Zhou, Wenjian Ma, Tongcun Zhang, Nan Wang, DNMT3A inhibits E2F1-induced arterial marker expression and impairs angiogenesis in human umbilical artery endothelial cells, Acta Biochimica et Biophysica Sinica, Volume 52, Issue 11, November 2020, Pages 1236–1246, https://doi.org/10.1093/abbs/gmaa109
- Share Icon Share
Abstract
Arterial marker genes EphrinB2 and HEY2 are essential for cardiovascular development and postnatal neovascularization. Our previous study confirmed that E2F1 could activate the transcription of EphrinB2 and HEY2 in human mesenchymal stem cells; however, the detailed mechanism has not been resolved yet. In this study, we focused on the interaction between E2F1 and DNMT3A, a de novo DNA methyltransferase, on regulating the expression of EphrinB2 and HEY2, and explored the potential mechanisms. Gain- and loss-of-function experiments implicated the positive effect of E2F1 on the expression of EphrinB2 and HEY2 and tube formation in human umbilical artery endothelial cells. Accumulation of DNMT3A decreased the levels of EphrinB2 and HEY2, and impaired tube formation induced by E2F1, while inhibiting DNMT3A by RNA interference augmented their expression and angiogenesis in E2F1-trasfected cells. We then asked whether the low expressions of EphrinB2 and HEY2 induced by DNMT3A are related to the methylation status of their promoters. Surprisingly, the methylation status of the CpG islands in the promoter region was not significantly affected by overexpression of exogenous DNMT3A. Furthermore, the interaction between E2F1 and DNMT3A was confirmed by co-immunoprecipitation. DNMT3A could inhibit the transcription of EphrinB2 and HEY2 promoters by affecting the binding of E2F1 to its recognition sequences as revealed by luciferase reporter assay and chromatin immunoprecipitation. These results identified a novel mechanism underlying the cooperation of DNMT3A with E2F1 on regulating target gene expression, and revealed their roles in the angiogenic process.
Introduction
Angiogenesis plays an important role in a wide variety of physiological and pathological processes, such as fetal development, wound healing, and primary tumor growth. EphrinB2 and HEY2 are specifically expressed in arterial endothelial cells [1]. A vascular-specific deletion of EphrinB2 leads to peripheral angiogenic defects, including yolk sac, anterior cardinal vein, and head and intersomitic vessel, as well as cardiac defect [2]. EphrinB2 alone promotes postnatal arterial and venous new blood vessel formation in vivo [3]. EphrinB2 homozygous mutant embryos die at embryonic day 9.5 (E9.5) due to severe cardiovascular defect [4,5]. HEY1 and HEY2 play critical roles in embryonic vascular development and arterial fate decision [6]. Deletion of HEY2 in the mouse leads to cardiac defects and high postnatal mortality. HEY1 single knockout mice have no obvious phenotypic defect, while HEY1 and HEY2 double knockout embryos die after E9.5 and display multiple vascular abnormalities and defects [7].
E2F1, frequently upregulated in a variety of human malignancies, is a critical regulator for tumor vascularization in vivo [8]. E2F1 could regulate multiple target gene expressions by binding to the promoter region [9]. Daniel et al. [10] reported that the ablation of individual E2Fs led to striking reductions in tumor metastatic capacity and angiogenesis by decreasing the expression of VEGF and Cyr61, etc. E2F1 mediates transcriptional regulation of multiple target genes associated with the process of angiogenesis, including angiogenic factors VEGFA, VEGFB, VEGFC, and PDGF-B, as well as their receptors VEGFR1, VEGFR2, VEGFR3, and FGFR-2 [8,11,12]. Our previous studies also confirmed that E2F1 could mediate transcriptional regulation of EphrinB2 and HEY2 in human mesenchymal stem cells [13]. Here, we further explored the regulatory effect of E2F1 on the transcription of EphrinB2 and HEY2 and angiogenesis in human umbilical artery endothelial cells (HUAECs).
DNA methylation, a major epigenetic modification of the mammalian genome, is established and maintained by three DNA methyltransferases (DNMT1, DNMT3A, and DNMT3B) [14]. De novo methyltransferases DNMT3A and DNMT3B are able to methylate previously unmethylated CpG sequences during embryogenesis [15]. However, DNMT3A and DNMT3B might have different DNA methylation targets according to cell type and development stage [16]. DNMT3A-deficient mice and DNMT3B-deficient mice showed different developmental defects [17]. Human DNMT3A and DNMT3B have been shown to have obvious and distinct flanking sequence preferences for the target CpG sites [18]. DNMT3B leads to colon tumorigenesis in ApcMin/+ mice by silencing tumor suppressor genes, whereas DNMT3A has no effect [19,20]. In contrast to DNMT3B, DNMT3A functions as a tumor suppressor gene in lung cancer progression. DNMT3A deficiency significantly promotes tumor growth in mutant K-ras-induced lung tumors and affects the genes closely related to angiogenesis [21]. However, the effect of DNMT3A on regulating the expression of EphrinB2 and HEY2 in HUAECs has not been reported.
In this study, we found that E2F1 upregulated the expression of EphrinB2 and HEY2 and promoted angiogenesis in HUAECs. DNMT3A could inhibit the expression of arterial marker genes and impair tube formation induced by E2F1. Furthermore, our studies showed that DNMT3A could affect the binding of E2F1 to its recognition sequence within the arterial marker gene promoters by interacting with E2F1 and thus led to the transcriptional inhibition of EphrinB2 and HEY2.
Materials and Methods
Cell culture
HUAECs were purchased from Shanghai Huiying Biological Technology Co. (Shanghai, China), and COS-7 cells were previously preserved in our laboratory. HUAECs were cultured in Dulbecco’s modified Eagle’s medium (DMEM)-high glucose (Gibco, New York, USA) supplemented with 15% fetal bovine serum (FBS) (AusGeneX, Gold Coast, Australia) in humidified air with 5% CO2. COS-7 cells were cultured in DMEM/F-12 (Gibco) supplemented with 10% FBS.
Plasmids and cell transfection
The pCMV-HA-E2F1 expression plasmid encoding human E2F1 was purchased from Addgene (Watertown, USA). The pCMV-Tag2B-DNMT3A plasmid encoding human DNMT3A, the pGL3-pHEY2-luc plasmid containing the promoter regions of human HEY2 (−620/ + 200), and the pGL3-pEphrinB2-luc plasmid containing the promoter regions of human EphrinB2 (−553/ + 200) were constructed by GENEWIZ (South Plainfield, USA). The DNMT3A interfering plasmid (shDNMT3A) was designed and constructed by MiaoLingBio (Wuhan, China). The interfering small interfering RNA (siRNA) duplexes for DNMT3A (target sequence: 5ʹ-CCACCAAAGCAGGCGATGA-3ʹ) and E2F1 (target sequence: 5ʹ-CCTGATGAATATCTGTACT-3ʹ) were designed and synthesized by RiboBio (Guangzhou, China), and control siRNA sequence (NC; 5ʹ-ACTGTTCTATGACTTGTCGTGAATA-3ʹ) was also from RiboBio. For transfection experiments, all plasmids and siRNAs were transiently transfected using TurboFect Transfection Reagent (Thermo Fisher, Waltham, USA) in serum-free medium for 6 h. After the medium was removed, normal culture medium was added and cells were cultured for another 48 h. Then quantitative real-time PCR (RT-PCR), western blot analysis, tube formation assay, analysis of DNA methylation, co-immunoprecipitation (Co-IP), luciferase reporter analysis, and chromatin immunoprecipitation (ChIP) were performed as follows.
Extraction of total RNA and quantitative RT-PCR
Total RNA was isolated from HUAECs using Trizol reagent (Invitrogen, Carlsbad, USA), and 2 μg of the RNA sample was reverse-transcribed using M-MLV reverse transcriptase (Promega, Madison, USA). RT-PCR was performed on an Applied Biosystems StepOneTM RT-PCR System (Applied Biosystems, Foster City, USA). Fast SYBR1 Green Master Mix was obtained from Applied Biosystems. Data were shown as relative expression level after being normalized by GAPDH.
The PCR primers used in the experiment are as follows: E2F1, forward 5′-TCTCCGAGGACACTGACA-3′ and reverse 5′-GTTCTCCGAAGAGTCCAC-3′; DNMT3A, forward 5′-TGAGG AGGACCGAAAGGACG-3′ and reverse 5′-CCATTGGGTAATAG CTCTGAGGC-3′; HEY2, forward 5′-TTCAAGGCAGCGGTTCA ACTGAC-3′ and reverse 5′-CATACTGATGCACTGCTGGATGG-3′; EphrinB2, forward 5′-CTCCTCAACTGTGCCAAACCA-3′ and reverse 5′-GGTTATCCAGGCCCTCCAAA-3′; GAPDH, forward 5′-CGAGATCCCTCCAAAATCAA-3′ and reverse 5′-TTCACACC CATGACGAACAT-3′.
Western blot analysis
After treatment, HUAECs were collected and lysed in lysis buffer. Protein extracts were subject to western blot analysis. Briefly, proteins were immunoblotted with the primary antibodies including anti-E2F1 (Santa Cruz, Dallas, USA), anti-DNMT3A (Santa Cruz), anti-EphrinB2 (Santa Cruz), anti-HEY2 (Bioss, Beijing, China), and anti-β-actin (Signalway Antibody, Peel, USA) antibodies overnight at 4°C, followed by incubation with IRDye-800-conjugated anti-mouse or IRDye-680-conjugated anti-rabbit secondary antibodies (EarthOx, Millbrae, USA) for 2 h at room temperature. The specific protein bands were visualized with the Odyssey Infrared Imaging System (Gene Company Limited, Beijing, China). Band intensity was analyzed using ImageJ software.
Tube formation assay
Matrigel basement membrane matrix (BD Biosciences, New York, USA) was thawed at 4ºC overnight. Before cell seeding, 96-well plates were coated with 50 μl of Matrigel and then allowed to polymerize at room temperature for 30 min. After transfection with the corresponding plasmids, 2 ∼ 3 × 104 HUAECs were resuspended in 50 μl of endothelial basal medium and then plated on Matrigel in the incubator. Tube formation was observed for 6 h, and images were acquired under an inverted phase contrast microscope (Nikon Eclipse Ti, Tokyo, Japan). Total tubule length, the number of tube formation, and branching points were analyzed by ImageJ software. Images of 10 representative fields were used for quantification.
Wound healing assay
For wound healing assay, HUAECs were seeded in six-well plates. When cells reached 70% ∼ 80% confluence, cells were transfected with the corresponding plasmids and cultured for another 24 h. Then a straight scratch was created in the plate using pipette tip, and the plate was placed in a 5% CO2 incubator chamber at 37ºC. Pictures were taken at the initial and final time points using a phase-contrast microscope (Nikon Eclipse Ti). The area filled by migrating cells was calculated using ImageJ software by comparing the images from the initial to the final time points.
Cell viability assay
Cell viability was examined by MTT assay. HUAECs were seeded in a 96-well plate and cultured overnight. Forty-eight hours after transfection with the corresponding plasmids, cells were treated with 5 mg/ml of 3-(4,5-dimethyl-thiazol-2-yl)-2,5-diphenyltetrazolium (MTT; Solarbio, Beijing, China) solution (20 μl per well) and then cultured for another 4 h. The medium was then discarded and 100 μl dimethyl sulfoxide (DMSO) was added to each well. The absorbance of each well was measured using a Synergy 4 plate reader (Bioteck, Winooski, USA) with a wavelength of 490 nm. Absorbance was directly proportional to the number of survival cells.
Analysis of DNA methylation
Genomic DNA (gDNA) was extracted from cells using the Universal Genomic DNA kit (Cwbio, Beijing, China) and quantified with the UV-Vis Spectrophotometer (Merinton, Ann Arbor, USA). gDNA was then modified with bisulfite using the EZ DNA Methylation-GoldTM kit (Zymo Research, Orange, USA) according to the manufacturer’s directions. The modified DNA was amplified using Taq plus DNA polymerase (Sangon Biotech, Shanghai, China).
The specific primers for bisulfite-converted DNA are as follow: EphrinB2, forward 5ʹ-GTTTTGGGGGTC/TGGGAGT-3ʹ and reverse 5ʹ-AATACA/GAACTAAAACACAAAAACCC-3ʹ; HEY2, fo- rward 5ʹ-AGTAGTTTGGATTTAGATAGGGGAT-3ʹ and reverse 5ʹ-TAAACA/GCAATCTTACTAACCCCTAC-3ʹ. Bisulfite sequencing was performed with the 3730xl DNA Analyzer (ABI, Waltham, USA).
Co-immunoprecipitation (Co-Ip) analysis
Co-IP was performed to detect the interaction between E2F1 and DNMT3A in HUAECs. The protein extracts were isolated from cells using RIPA buffer (Solarbio, Beijing, China). The proteins were incubated with either anti-E2F1 antibodies or IgG (Bioss) overnight at 4°C, followed by the addition of Protein A/G Mix Magnetic Beads (Merck KGaA, Darmstadt, Germany) and subsequent incubation for 12 h. The protein extracts (input) and immunoprecipitates were then analyzed by immunoblotting.
Luciferase reporter assay
Luciferase reporter assay was performed in COS-7 cells. Cells infected with EphrinB2 or HEY2 promoter-luciferase plasmid (pEphrinB2-luc or pHEY2-luc) were cotransfected with empty vector, pCMV-HA-E2F1 or/and pCMV-Tag2B-DNMT3A. After 24 h, cellular lysates were collected and analyzed for luciferase activity using a luciferase reporter assay system (Promega, Madison, USA) on the Synergy 4 plate reader. Luciferase measurement was normalized to total protein concentrations. All experiments were performed independently at least three times.
Chromatin immunoprecipitation
ChIP assay was carried out following standard protocol [22]. Cellular lysates were cross-linked with 1% formaldehyde for 15 min at room temperature and sonicated on ice to shear DNA to lengths between 200 and 800 bp. Immunoprecipitations were conducted using anti-E2F1 antibody or mouse IgG antibodies. ProteinG sepharose beads (Merck KGaA) were added to the immunocomplexes, followed by washing and elution. The crosslinking was reversed with 0.2 M NaCl, and samples were incubated with Proteinase K (Promega) overnight at 65°C. DNA was subsequently purified by phenol-chloroform extraction and ethanol precipitation, and then RT-PCR was performed using the following primers: EphrinB2 (E2F1 binding site), forward 5′-ATTCCCTCTTTCCTGCCCTG-3′ and reverse 5′-GAGCACGGAGCGGATTAG-3′; HEY2 (E2F1 binding site), forward 5′-AGGTCAGCCAGTCAGCAGT-3′ and reverse 5′-GGAGTGTAAAGAATAACCCCAAGC-3′.
Statistical analysis
GraphPad Prism 6 (GraphPad Software Inc., La Jolla, USA) was applied to analyze all data in this study. Student’s t-test was used to assess the significance of differences among groups. Data were presented as the mean ± SD (standard variation) from more than three independent experiments. P < 0.05 was considered to be statistically significant.
Results
Overexpression of E2F1 upregulated the expression of arterial marker genes and promoted angiogenesis in HUAECs
To investigate the effect of E2F1 on the expression of arterial marker genes, HUAECs were transfected with E2F1. Forty-eight hours after transfection, the mRNA and protein levels of EphrinB2 and HEY2 were detected by RT-PCR and western blot analysis, respectively. The results showed that the mRNA levels of EphrinB2 and HEY2 were significantly upregulated in E2F1-transfected HUAECs (Fig. 1A, P < 0.05). Similar results were obtained at the protein level. Western blot analysis showed that the transfection of E2F1 obviously augmented the protein levels of EphrinB2 and HEY2 in HUAECs (Fig. 1B,C, P < 0.05). Considering the important role of EphrinB2 and HEY2 in angiogenesis, we next explored the effect of E2F1 on the angiogenesis of HUAECs using an in vitro Matrigel tube formation assay. HUAECs transfected with E2F1 could form more tubular structures than the control cells within the same time (Fig. 1D). Increased total tubule length (P < 0.05), number of tube formation (P < 0.01), and branching points (P < 0.05) were observed in HUAECs transfected with E2F1 (Fig. 1E), indicating the pro-angiogenic effects of E2F1. Wound healing assay data showed that the rate of wound closure was increased in cells transfected with E2F1 (Fig. 1F,G, P < 0.05). Overexpression of E2F1 significantly promoted cell proliferation in HUAECs at 48 h (Fig. 1H, P < 0.01). These data indicated that overexpression of E2F1 could increase the expressions of EphrinB2 and HEY2, as well as angiogenesis.
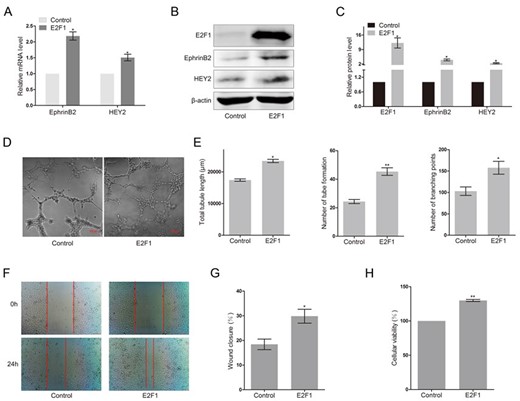
Overexpression of E2F1 upregulated the expression of arterial marker genes and promoted angiogenesis in HUAECs (A) RT-PCR analysis of EphrinB2 and HEY2 in HUAECs transfected with E2F1 or empty vector. Western blots (B) and quantification (C) of EphrinB2 and HEY2 in HUAECs transfected with E2F1 or empty vector. Matrigel assay (D) and quantification (E) of HUAECs transfected with E2F1 or empty vector. (F) Migration of HUAECs transfected with E2F1 or empty vector detected by wound healing assay. (G) Analysis of wound closure percentage. (H) Proliferation of HUAECs transfected with E2F1 or empty vector detected by MTT assay. Data were presented as the mean ± SD, n = 3. *P < 0.05, **P < 0.01.
Knockdown of E2F1 decreased the expression of arterial marker genes and abolished angiogenesis in HUAECs
Considering that E2F1 is expressed in HUAECs, knockdown of E2F1 using a special siRNA targeting E2F1 was performed to further evaluate the role of E2F1 in HUAECs. Forty-eight hours after transfection with E2F1 siRNA, HUAECs exhibited low levels of EphrinB2 and HEY2 mRNA (Fig. 2A, P < 0.01). As shown in Fig. 2B,C, knockdown of E2F1 remarkably decreased the expression of EphrinB2 protein (P < 0.01), and also reduced the expression of HEY2 protein, but not significantly. Meanwhile, tube formation capability of endothelial cells was remarkably abolished when the level of the endogenous E2F1 in HUAECs was decreased by the action of E2F1 RNAi (Fig. 2D). Loss of E2F1 significantly decreased the total tubule length, number of tube formation, and branching points (Fig. 2E, P < 0.01). Loss of E2F1 also decreased cell migration (P < 0.01) and proliferation (P < 0.05) in HUAECs (Fig. 2F–H). The above results showed that E2F1 is a critical factor regulating the expressions of EphrinB2 and HEY2, as well as angiogenesis.
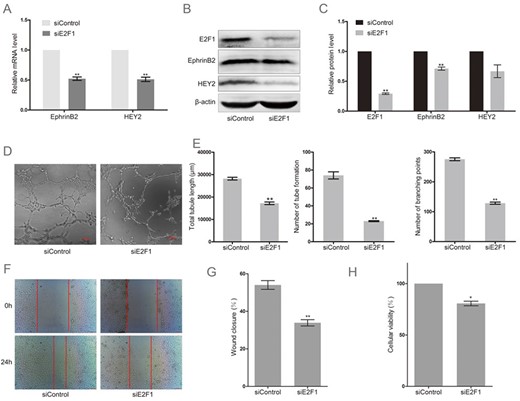
Knockdown of E2F1 decreased the expression of arterial marker genes and abolished angiogenesis in HUAECs (A) RT-PCR analysis of EphrinB2 and HEY2 in HUAECs transfected with a special siRNA targeting E2F1 (siE2F1) or non-targeting siRNA (siControl). Western blots (B) and quantification (C) of EphrinB2 and HEY2 in HUAECs transfected with siE2F1 or siControl. Matrigel assay (D) and quantification (E) of HUAECs transfected with siE2F1 or siControl. (F) Migration of HUAECs transfected with siE2F1 or siControl detected by wound healing assay. (G) Analysis of wound closure percentage. (H) Proliferation of HUAECs transfected with siE2F1 or siControl detected by MTT assay. Data were presented as the mean ± SD, n = 3.*P < 0.05, **P < 0.01.
Overexpression of DNMT3A suppressed E2F1-induced expression of arterial marker genes and impaired angiogenesis in HUAECs
To explore the possible role of DNMT3A in the activation of EphrinB2 and HEY2 induce by E2F1, the levels of EphrinB2 and HEY2 were examined in E2F1-expressing HUAECs co-transfected with exogenous DNMT3A gene. As shown in Fig. 3A, accumulated DNMT3A impaired the expression of EphrinB2 (P < 0.01) and HEY2 (P < 0.05) mRNA induced by E2F1. Similarly, E2F1 enhanced the levels of EphrinB2 and HEY2 protein and this regulation could be attenuated by DNMT3A expression (Fig. 3B,C, P < 0.05). Next, we tested the effect of DNMT3A in tube formation of E2F1-expressing HUAECs. As shown in Fig. 3D, overexpression of exogenous DNMT3A attenuated the effect of E2F1 on promoting tube formation of endothelial cells. Reductions in the total tubule length (P < 0.01), branching points (P < 0.05), and number of tube formation (P < 0.05) were found in DNMT3A-expressing HUAECs, when compared with the cells transfected with the empty vector (Fig. 3E). DNMT3A also inhibited the E2F1-mediated increase in the total tubule length (P < 0.01), number of tube formation (P < 0.05), and branching points (P < 0.05) (Fig. 3E). DNMT3A inhibited E2F1-induced cell migration (P < 0.05), but did not affect cell proliferation of HUAECs (Fig. 3F–H).
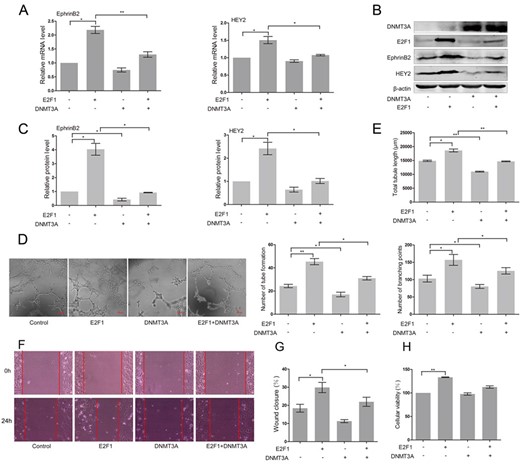
Overexpression of DNMT3A suppressed E2F1-induced expression of EphrinB2 and HEY2 and inhibited angiogenesis in HUAECs (A) RT-PCR analysis of EphrinB2 and HEY2 in HUAECs co-transfected with vector, E2F1 and/or DNMT3A. Western blots (B) and quantification (C) of EphrinB2 and HEY2 in HUAECs co-transfected with vector, E2F1 and/or DNMT3A. Matrigel assay (D) and quantification (E) of HUAECs co-transfected with vector, E2F1 and/or DNMT3A. (F) Migration of HUAECs co-transfected with vector, E2F1 and/or DNMT3A detected by wound healing assay. (G) Analysis of wound closure percentage. (H) Proliferation of HUAECs co-transfected with vector, E2F1 and/or DNMT3A detected by MTT assay. Data were presented as the mean ± SD, n = 3. *P < 0.05, **P < 0.01.
Knockdown of DNMT3A enhanced the expression of arterial marker genes and stimulated angiogenesis in E2F1-expressing HUAECs
To further support a role for DNMT3A in the regulation of EphrinB2 and HEY2 expression, we attempted to knockdown DNMT3A in HUAECs transfected with the empty vector or E2F1. Introduction of DNMT3A siRNA into HUAECs obviously enhanced the expression of EphrinB2 (P < 0.01) and HEY2 mRNA (P < 0.05) (Fig. 4A). Knockdown of DNMT3A significantly upregulated the mRNA level of HEY2 (P < 0.05) in E2F1-expressing cells and also elevated the level of EphrinB2, but not significantly (Fig. 4A).
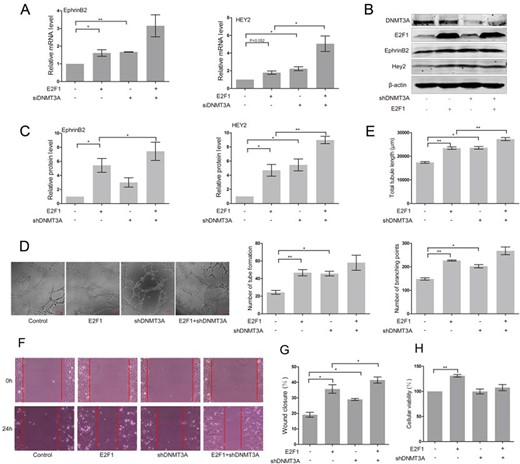
Knockdown of DNMT3A enhanced the expression of EphrinB2 and HEY2 and stimulated angiogenesis in E2F1-expressing HUAECs (A) RT-PCR analysis of EphrinB2 and HEY2 in E2F1-expressing HUAECs co-transfected with DNMT3A siRNA or control siRNA. Western blots (B) and quantification (C) of EphrinB2 and HEY2 in E2F1-expressing HUAECs co-transfected with non-targeting shRNA vector or DNMT3A targeting shRNA. Matrigel assay (D) and quantification (E) of E2F1-expressing HUAECs co-transfected with non-targeting shRNA vector or DNMT3A targeting shRNA. (F) Migration of E2F1-expressing HUAECs co-transfected with non-targeting shRNA vector or DNMT3A targeting shRNA detected by wound healing assay. (G) Analysis of wound closure percentage. (H) Proliferation of E2F1-expressing HUAECs co-transfected with non-targeting shRNA vector or DNMT3A targeting shRNA detected by MTT assay. Data were presented as the mean ± SD, n = 3. *P < 0.05, **P < 0.01.
HUAECs were then subject to a transient transduction with either non-targeting short hairpin RNA (shRNA) vector or DNMT3A targeting shRNA, followed by western blot analysis. The results showed that silencing of DNMT3A expression notably augmented the expression of HEY2 (P < 0.01) and EphrinB2 (P < 0.05) in HUAECs transfected with E2F1 (Fig. 4B,C). Furthermore, loss of DNMT3A led to the increase of tube formation in the presence or absence of E2F1 (Fig. 4D). In addition, increase in the number of tube formation (P < 0.05), branching points (P < 0.05), and total tubule length (P < 0.05) were found in HUAECs with DNMT3A knockdown, when compared with cells transfected with the empty vector (Fig. 4E). Silencing of DNMT3A expression remarkably enhanced E2F1-mediated increase in the total tubule length (P < 0.01), and also upregulated the number of tube formation and branching points, but not significantly (Fig. 4E). Silencing of DNMT3A increased E2F1-induced cell migration (P < 0.05), but did not affect cell proliferation of HUAECs (Fig. 4F–H). Taken together, these data indicated the negative regulation of DNMT3A in arterial marker gene expression and angiogenesis in HUAECs in response to E2F1.
DNMT3A negatively regulated the expression of HEY2 and EphrinB2 in a promoter methylation-independent manner in HUAECs
DNMT3A might repress gene transcription by direct methylation of the promoter to which it is recruited [23]. Thus, to directly show methylation of the HEY2 and EphrinB2 genes, bisulfite modification and DNA sequencing were used to analyze the methylation status of a 228 bp (EphrinB2) or 256 bp (HEY2) CpG island within the promoter of these two genes. As shown in Fig. 5, methylation in the promoter regions of HEY2 and EphrinB2 was not observed in normal HUAECs. Surprisingly, overexpression of DNMT3A did not significantly affect the methylation status of the CpG islands in the promoter regions of HEY2 and EphrinB2 in the presence or absence of E2F1 (Fig. 5). Elevated expression of DNMT3A only partially enhanced the methylation of CpG island at − 432 bp. Thus, these data suggested that DNMT3A negatively regulated the expression of HEY2 and EphrinB2 in a promoter methylation-independent manner in HUAECs.
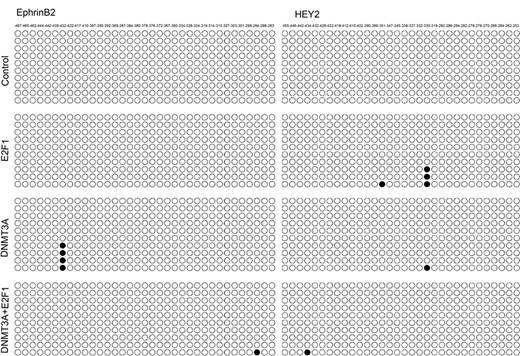
Promoter CpG island methylation status analysis of EphrinB2 and HEY2 in HUAECs co-transfected with vector, E2F1 and/or DNMT3A DNMT3A negatively regulated the expression of HEY2 and EphrinB2 in a promoter methylation-independent manner in HUAECs.
Protein-protein interaction between DNMT3A and E2F1
DNMT1 has been reported to interact with some transcription factors including p53 [24], E2F1 [25] and stat3 [26], etc. This interaction induces the recruitment of DNMT1 on DNA sites usually bound by transcription factors and maintains the methylation status of CpG located on loci or nearby sites. However, whether DNMT3A could interact with E2F1 is still not clear. Thus, we next examined protein-protein interaction between DNMT3A and E2F1 by Co-IP. HUAECs were co-transfected with DNMT3A and E2F1, and then coimmunoprecipitation assay was performed using anti-E2F1 antibodies. As shown in Fig. 6, Co-IP experiment confirmed a direct physical interaction between DNMT3A and E2F1 in vivo.
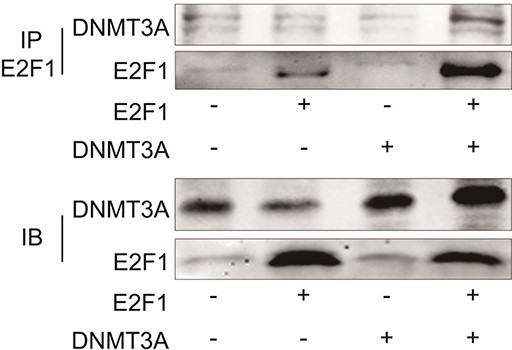
Interaction between DNMT3A and E2F1 in HUAECs co-transfected with vector, DNMT3A and/or E2F1 There is a direct physical interaction between DNMT3A and E2F1 in vivo.
DNMT3A repressed the transcription of E2F1 target genes through affecting the binding of E2F1 to its recognition sequence within gene promoters
To explore the role of DNMT3A-E2F1 interaction in the transcriptional regulation of HEY2 and EphrinB2, the HEY2 and EphrinB2 reporter plasmids were constructed and luciferase reporter assay was performed in HUAECs (Fig. 7A). As shown in Fig. 7B, E2F1 significantly activated the transcription of HEY2 and EphrinB2, while the ability of E2F1 to activate the promoter of HEY2 and EphrinB2 was greatly reduced (P < 0.05) in the presence of DNMT3A. Since DNMT3A negatively regulates the transcription of HEY2 and EphrinB2 in a promoter methylation-independent manner in HUAECs, we next explored whether DNMT3A could inhibit the access of E2F1 to E2F1-targeted promoters. Chromatin immunoprecipitation analysis indicated that E2F1 was enriched in the E2F1 binding sites of HEY2 (P < 0.05) and EphrinB2 (P < 0.01) promoters in E2F1-transfected HUAECs, compared with the control cells (Fig. 7C,D). Elevated DNMT3A expression significantly decreased the levels of E2F1 around the E2F1-binding sites (Fig. 7D). These results suggested that DNMT3A could affect the binding of E2F1 to its recognition sequences within the promoters of HEY2 and EphrinB2.
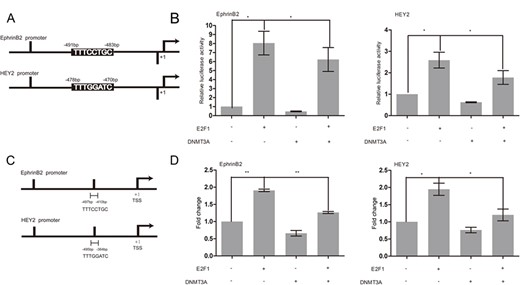
DNMT3A repressed the transcription of E2F1 target genes through affecting the binding of E2F1 to its recognition sequence within gene promoters (A) Schemes of EphrinB2 and HEY2 promoter constructs with relative locations of predicted E2F1 binding sites. (B) Cos-7 cells were cotransfected with EphrinB2 or HEY2 promoter vector and DNMT3A and/or E2F1 expression plasmids for 24 h, and then luciferase activity was measured. (C) A schematic diagram showing the relative positions of the sites in EphrinB2 or HEY2 promoter. (D) HUAECs were cotransfected with vector, E2F1, DNMT3A, and E2F1/DNMT3A, and ChIP assays were performed using a rabbit IgG as a control and with anti-E2F1 antibody as indicated. Data are presented as the mean ± SD relative to the total DNA input signal, n = 3. *P < 0.05, **P < 0.01.
Discussion
E2F1 has been reported to play an important role in regulating G1/S transition and promoting cell proliferation [27]. E2F1 is negatively regulated by tumor suppressor protein retinoblastoma (Rb) [28]. The frequent loss and mutation of Rb in tumor often lead to the high expression of E2F1. Compared to the well-established role of E2F1 in tumor and cell proliferation, its involvement in the regulation of angiogenesis has not been fully elucidated. Recent studies have revealed that E2F1 plays a critical role in the transcription regulation of multiple genes associated with angiogenesis. Analysis of gene expression profiles using cDNA microarrays revealed several upregulated genes associated with angiogenesis, including FGF2, MMP16, and VEGFB in Saos-2 cells following E2F1 activation [29]. Engelmann et al. [8] demonstrated that E2F1 bound to and activated human VEGFC and VEGFR3 gene promoters. VEGFC/VEGFR3 upregulation driven by E2F1 stimulated cultured ECs to form tubule-like structures in vitro and promoted formation of new blood vessels in vivo [8]. E2F1 was also found to activate the transcription activity of the other two important VEGF receptors FLT-1 and KDR by binding to their promoters, and its binding to the promoters and the recruitment of histone acetyl transferases like CBP, p300, and PCAF were enhanced upon VEGF stimulation [12]. Furthermore, transcription of mouse and human FGFR-2 was also activated by E2F1 [11]. In a previous study, we identified two novel E2F1 target genes EphrinB2 and HEY2 in human mesenchymal stem cells [13]. EphrinB2 and HEY2 are specially expressed in artery endothelial cells. Herein, we demonstrated that E2F1 could activate the transcription of EphrinB2 and HEY2, and promote angiogenesis in HUAECs. Luciferase reporter assay and ChIP determined that E2F1 could bind to the promoters of EphrinB2 and HEY2 and upregulate their promoter activities.
DNMT3A is a major de novo DNA methyltransferase essential for embryonic development in mammals. However, the role of DNMT3A in angiogenesis is rarely reported. Gao et al. [21] recently reported the role of DNMT3A in tumor angiogenesis. DNMT3A deletion facilitates lung tumor progression and invasion. Transcriptional profiling analyses by using whole mouse genome microarrays demonstrated that loss of DNMT3A affected multiple genes associated with blood vessel morphogenesis, blood vessel development, angiogenesis and vasculature development. Gene Ontology enrichment analysis revealed that the top biological process affected most significantly by these genes was angiogenesis, a key step of cancer progression. Our previous studies confirmed that DNMT3A knockdown contributes to endothelial differentiation and angiogenesis of MSCs. In this study, we found that overexpression of DNMT3A in HUAECs attenuated the mRNA and protein expression of EphrinB2 and HEY2 induced by E2F1. In vitro angiogenesis assays with HUAECs revealed that accumulation of DNMT3A significantly abolished E2F1-induced tube formation. Studies utilizing shRNA knockdown of DNMT3A in arterial endothelial cells showed that DNMT3A knockdown significantly upregulated the expression of EphrinB2 and HEY2. Targeted knockdown of DNMT3A also enhanced angiogenesis in the presence or absence of E2F1.
DNMT3A catalyzes the methylation of cytosine residues in CpG dinucleotide in genome, and thus silences gene expression. To determine whether the inhibitory effect of DNMT3A depends on its methyltransferase activity, a 228 bp (EphrinB2) or 256 bp (HEY2) section of bisulfite converted DNA extracted from HUAECs transfected with empty vector or DNMT3A was sequenced. We found that the methylation status of EphrinB2 and HEY2 in HUAECs was not significantly affected by exogenous DNMT3A. The CpG islands in the promoter regions of HEY2 and EphrinB2 were less methylated in cells transfected with DNMT3A in the presence or absence of E2F1. Similar to our results, Conrad et al. [30] showed that DNMT1 could function in a methylation-independent manner. Aberrant hypermethylation of the androgen receptor (AR) promoter led to its silence in AR-negative metastatic prostate cancer cell line DU-145. DNMT1 deletion restored AR gene expression, but did not change the methylation status of the AR minimal promoter associated region [30].
In the past few years, DNMT3A was found to participate in transcription repression of genes by methylation-independent mechanisms, and DNMT3A could also suppress gene transcription by recruiting transcriptional repressor protein complexes, like histone deacetylase (HDAC) [31]. It has been reported that DNMT1 could form a complex with Rb/E2F1 and HDAC1, and this complex could repress transcription of promoters containing E2F-binding sites [25]. However, whether DNMT3A interacts with E2F1 is still unclear. Our results indicated that DNMT3A could form a complex with E2F1 and that this association was capable of repressing the binding of E2F1 to its recognition sequence within the promoters of HEY2 and EphrinB2. Thus accumulated expression of DNMT3A impaired the transcription of EphrinB2 and HEY2 promoters induced by E2F1 and led to the transcriptional inhibition. Taken together, our data showed that DNMT3A, in cooperation with E2F1, inhibited EphrinB2 and HEY2 gene expression in a methylation-independent manner. Our results identified a novel mechanism underlying the cooperation of DNMT3A and E2F1 in regulating target gene expression and revealed their roles in the angiogenic process. Therefore, these findings may provide a framework for our understanding of the mechanisms underlying angiogenesis in arterial endothelial cells and contribute to future development of new strategies for therapeutic angiogenesis.
Funding
This work was supported by the grants from the National Key R&D Program of China (No. 2017YFD0400304), the Innovative Research Team of Tianjin Municipal Education Commission (No. TD13-5015), and the Tianjin Natural Science Foundation (No. 17JCZDJC33600).