-
PDF
- Split View
-
Views
-
Cite
Cite
Yanhua Liu, Kunli Yang, Ling Wang, Jinfang Yang, Yang Wang, Hu Luo, Peng Li, Yaling Yin, Vitamin B6 prevents Isocarbophos-induced posterior cerebral artery injury in offspring rats through up-regulating S1P receptor expression, Acta Biochimica et Biophysica Sinica, Volume 53, Issue 12, December 2021, Pages 1691–1701, https://doi.org/10.1093/abbs/gmab150
- Share Icon Share
Abstract
We have previously reported that the long-term exposure of Isocarbophos, a kind of organophosphorus compounds, induces vascular dementia (VD) in rats. Studies have also shown that organophosphorus compounds have adverse effects on offsprings. Vitamin B6 is a coenzyme mainly involved in the regulation of metabolism and has been demonstrated to ameliorate VD. Sphingosine-1-phosphate (S1P), a biologically active lipid, plays a vital role in the cardiovascular system. However, whether S1P is involved in the therapeutic effects of Vitamin B6 on posterior cerebral artery injury has yet to be further answered. In the present study, we aimed to explore the potential influence of Vitamin B6 on Isocarbophos-induced posterior cerebral artery injury in offspring rats and the role of the S1P receptor in this process. We found that Vitamin B6 significantly improves the vasoconstriction function of the posterior cerebral artery in rats induced by Isocarbophos by the blood gas analysis and endothelium-dependent relaxation function assay. We further demonstrated that Vitamin B6 alleviates the Isocarbophos-induced elevation of ICAM-1, VCAM-1, IL-1, and IL-6 by using the enzyme-linked immunosorbent assay kits. By performing immunofluorescence and the western blot assay, we revealed that Vitamin B6 prevents the down-regulation of S1P in posterior cerebral artery injury. It is worth noting that Fingolimod, the S1P inhibitor, significantly inhibits the Vitamin B6-induced up-regulation of S1P in posterior cerebral artery injury. Collectively, our data indicate that Vitamin B6 may be a novel drug for the treatment of posterior cerebral artery injury and that S1P may be a drug target for its treatment.
Introduction
Organophosphorus compounds have become the main body of the pesticide industry in social agriculture, which are used to control insects, pests, and plant diseases. Organophosphorus compounds can enter the body through the digestive tract, respiratory tract, intact skin, and mucous membranes. Furthermore, they cause a series of nerve poisoning symptoms such as sweating, mental disorder, and speech disorder [1–3].
Previous studies have shown that organophosphorus compounds could not only cause a variety of neurotoxic diseases but also have the ability to directly cause neurotoxicity to a certain extent [4]. Isocarbophos is an organophosphorus insecticide used worldwide in agriculture, which has good control effects on mites in arachnida and lepidoptera and homoptera in insecta. It can cause human poisoning through the esophagus, skin, and respiratory tract. Our previous studies also demonstrated a strong association between long-term low-dose Isocarbophos treatment and vascular dementia (VD) in rats [5–7]. Endothelial cells (ECs) cover the inner surface of blood vessels and play an important role in maintaining the normal functions of biological vascular systems [8]. It has been reported that certain common clinical cardiovascular and cerebrovascular diseases, such as coronary heart disease, diabetes, and atherosclerosis, are closely related to vascular endothelial injury [9]. However, the possibility of Isocarbophos to induce vascular injury in offspring rats has been barely explored.
Vitamin B6 is a metabolic essential nutrient that plays a key role in nervous system development and function, as well as in the treatment of neurological diseases [10–12]. Phosphate derivative pyridoxal 5ʹ-phosphate (PLP) is the biologically active form of Vitamin B6 that reflects long-term body storage. Low plasma levels of PLP have been proven to be associated with the increased risk of cardiovascular diseases [13–15], and thus, supplementation of Vitamin B6 can improve vascular endothelial dysfunction in mice fed with high-fat diet [16]. In addition, Vitamin B6 can promote the biosynthesis of endogenous nitric oxide (NO) and platelet-derived NO to improve the endothelial dysfunction. However, it is unknown whether Vitamin B6 has a protective effect on vascular endothelial injury induced by Isocarbophos in offspring rats.
Sphingosine-1-phosphate (S1P) participates in many important biological processes as a biologically active lipid messenger. On the one hand, as a metabolite of sphinolipin, S1P can specifically bind to receptors to trigger biological reactions and play a role in cardiovascular formation and regulation as well as the immune system. On the other hand, as a typical second messenger, S1P can regulate intracellular biological processes through changes in concentration [17]. When cells are disturbed by the outside world, S1P concentration will increase rapidly, thus causing a series of cellular reactions. In addition, S1P can directly activate the calcium channel in the endoplasmic reticulum and increase the intracellular S1P level and Ca2+ level. S1P is involved in the pathophysiological processes of apoptosis, neurogenesis, immunosuppression, and injury repair in the central nervous system. Moreover, S1P has important physiological functions as a phospholipid metabolite for cardiovascular diseases [18] and can specifically bind to S1P receptors (S1PRs) expressed on the cell surface to trigger a wide range of biological activities, including angiogenesis, endothelial protection, myocardial ischemia reperfusion injury, adhesion molecule expression, and immune activation [19–21]. In light of the importance of S1P, compounds that are capable of regulating S1P may hold translational potential as novel therapeutic drugs for vascular injury. Fingolimod is an immunomodulating drug, mostly used for the treatment of multiple sclerosis. It reduces the rate of relapses in relapsing–remitting multiple sclerosis by approximately one-half over a 2-year period. Fingolimod is an S1PR inhibitor that sequesters lymphocytes in lymph nodes, preventing them from contributing to an autoimmune reaction [22,23]. However, none of the above compounds are undergoing clinical trials in the vascular injury patients. Therefore, further research is needed to explore effective S1P regulatory agents for vascular injury treatment. Vitamin B6, as a metabolic essential nutrient, plays a protective role by regulating S1P and meets the urgent needs of patients with vascular injury.
In the present study, a model of the Isocarbophos-induced posterior cerebral artery injury in offspring rats was established. By detecting the content of oxidative stress index, inflammatory factors, and the expression of SIP protein, we analyzed the mechanism of Vitamin B6 in the posterior cerebral artery injury of offspring rats, clarified the role of Vitamin B6 in the posterior cerebral artery injury of offspring rats, and investigated the interaction between Vitamin B6 and S1P in the posterior cerebral artery injury of offspring rats.
Materials and Methods
Reagents
Vitamin B6, acetylcholine, ethylenediaminetetraacetic acid, and tetramethylethylenediamine were purchased from Sigma-Aldrich (St Louis, USA). Radioimmunoprecipitation lysis buffer, loading buffer, anti-β-actin antibody, BCA protein assay kit, anti-horseradish peroxidase antibody, hematoxylin and eosin (H&E) staining kit, and BeyoECL plus were purchased from Beyotime Biotechnology (Shanghai, China). Paraformaldehyde, glycine, Tris, and Tween-20 were purchased from Solarbio (Beijing, China). Protein marker, Fingolimod, ICAM-1, VCAM-1, IL-1, and IL-6 mouse enzyme-linked immunosorbent assay (ELISA) kits were purchased from Thermo Fisher Scientific (San Jose, USA). Anti-S1P1 antibody, anti-S1P2 antibody, and anti-Caspase-3 antibody were purchased from Santa Cruz Biotechnology (Santa Cruz, USA). Acetylcholine assay kit, total superoxide dismutase (SOD) assay kit, NO assay kit (Microwell plate method), and malondialdehyde (MDA) assay kit were purchased from Nanjing Jiancheng Bioengineering Institute (Nanjing, China). S1P mouse ELISA kit was purchased from Tripbiotech (Shanghai, China).
Animals
Male and female Sprague Dawley rats (160±20 g) were purchased from Animal Experimental Center of Zhengzhou University (Zhengzhou, China). They were kept in a suitable place with room temperature of 22°C–24°C and 45%–65% relative humidity, with a day/dark cycle for 12 h and free access to food and water. The experimental protocol was approved by the Animal Care Committee of Xinxiang Medical University, Henan Province and was in line with the Guidelines of the National Institutes of Health for Laboratory Animal Care and Use.
Animal model
Animals were randomly divided into six groups (10 male and 20 female rats per group) as follows: Control group; Vitamin B6 group (50 mg/kg/day); Fingolimod group (100 mg/kg/day); Isocarbophos group (0.5 mg/kg every two days); Isocarbophos+Vitamin B6 (50 mg/kg/day) group; and Isocarbophos+Fingolimod (100 mg/kg/day)+Vitamin B6 (50 mg/kg/day) group. When the animals were administered with the drug continuously for 16 weeks, the female rats and male rats in each group were caged together to propagate offspring. After 8 weeks, eight offspring rats of the same age were randomly selected from each group for assessment of posterior cerebral artery injury.
Blood gas analysis
The offspring rats were anesthetized with 10% chloral hydrate. After the toe reflexes disappeared, the thoracic cavities of the offspring rats were opened with tissue scissors to expose the hearts. Then, 2 ml of blood was extracted from the left ventricle using blood gas analysis needles (Shanghai, China) for blood gas analysis (PL2200; Perlove Medical, Nanjing, China).
Measurement of endothelium-dependent relaxation function
The posterior cerebral arteries of offspring rats were isolated carefully by removing the adhering perivascular tissue and then cut into vascular rings (3–5 mm in length). The obtained vascular rings were transferred into baths containing Krebs’ solution (37°C, 95% O2, and 5% CO2). Phenylephrine (Phe, 1 µM) was used to evoke the contractile response of arterial vasoconstriction. Once phenylephrine induced a stable tension, acetylcholine (ACh) at a cumulative concentration (0.003∼3 μM) was added to the bath, and the relaxation response induced by 3 μM ACh was recorded as the maximum relaxation effect (Emax). The endothelium-dependent relaxation (EDR) response induced by ACh at this concentration range was recorded. The diastolic percentage of ACh was calculated with the maximum tension of phenyladrenaline excitation of 100%. The half effective amount (EC50), the required ACh concentration to cause 50% of the maximum diastolic rate, was determined from the dose–response curve by linear regression.
Measurement the activities of SOD, MDA, and NO
The samples of the blood and posterior cerebral arteries were collected from offspring rats. The contents of MDA, SOD, and NO in blood and tissue samples were measured using the the MDA assay kit, SOD assay kit, and NO assay kit according to the manufacturer’s instructions, respectively.
Inflammatory factor measurement
The collected blood samples in anticoagulation tubes were centrifuged at 157 g for 15 min to obtain the supernatant. The collected posterior cerebral arteries were homogenized and the supernatant was obtained. The levels of intercellular adhesion molecule-1 (ICAM-1), vascular cell adhesion molecule-1 (VCAM-1), interleukin-1 (IL-1), and interleukin-6 (IL-6) were measured using commercial ELISA kits according to the manufacturer’s protocols.
Hematoxylin & eosin staining
H&E staining was used to detect the overall morphology of the tissue [24]. The tissue specimens of the brains were fixed with 4% paraformaldehyde. The fixed tissues were dehydrated with a series of sucrose solution, embedded in frozen optimal cutting temperature medium (Shanghai, China), and cut into sections at a thickness of 10 µm using a cryostat. The obtained sections were dried at 60°C for 2 h and then used for H&E staining. The dried sections were washed with water three times, and the washed sections were immersed in hematoxylin working solution for 10 min and then in eosin working solution for 3 min. The stained sections were washed with water again, dehydrated with a series of ethanol solution, and then immersed in xylene for 10 min. A Pannoramic SCAN II microscope (3D HISTECH Corporation, Budapest, Hungary) was used to capture the images of stained sections.
Immunohistochemical analysis
The sections were incubated with H2O2/ethanol solution (V/V=1/50) at room temperature for 30 min and then washed with water. Then, the sections were immersed in 20 mM phosphate buffered saline (PBS) solution (pH 7.2–7.6) and heated to boiling for antigen retrieval. After that, the sections were blocked with 5% bovine serum albumin (Beyotime Biotechnology, Shanghai, China) at 37°C for 30 min and incubated with anti-caspase-3 antibody at 4°C overnight. Subsequently, the sections were washed three times with PBS and incubated with peroxidase-conjugated secondary antibody (59d2394; Beyotime Biotechnology) at 37°C for 1 h. The sections were stained by incubation with 3,3ʹ-diaminobenzidine substrate solution (Solarbio, Beijing, China). The stained sections were washed with water, dehydrated with a series of ethanol solution, and then immersed in xylene for 10 min. An Axio A1 photomicroscope (Carl Zeiss, Yena, Germany) was used to capture the images of stained sections.
Immunofluorescence staining
The sections were fixed with cold acetone for 10 min and washed three times with PBS, followed by incubation with 2% goat serum albumin (Beyotime Biotechnology) at room temperature for 30 min. After being washed with PBS, the sections were incubated with anti-S1P1 or anti-S1P2 antibody at 4°C for 12 h, respectively. Subsequently, the sections were washed with PBS and incubated with secondary antibody at 37°C for 1 h. The stained sections were washed with PBS again and then observed under the Pannoramic SCAN II microscope.
Western blot analysis
The collected posterior cerebral arteries were frozen, triturated, and homogenized to extract protein, and the total protein content was quantified using a BCA protein assay kit. The extracted protein samples were mixed with loading buffer and sodium dodecyl sulfate (SDS) solution, and the resulting solution was heated at 98°C for 10 min. Then, 10 μl of the samples was subject to 8% SDS-polyacrylamide gel electrophoresis and transferred onto nitrocellulose filter (NC) membranes (Santa Cruz Biotechnology, Beijing, China). The membranes were blocked and incubated with anti-S1P1 antibody (1:1000; Santa Cruz Biotechnology) or anti-β-actin antibody (1:2000; Beyotime Biotechnology), followed by incubation with the peroxidase-conjugated secondary antibody at 38°C for 1 h; the protein bands were visualized via chemiluminescence using the ECL Kit (catalog number: KF003; dilution 1/5000; Affinity) and then measured by ImageJ software (National Institutes of Health, New York, USA). β‐Actin was utilized as an internal control for equal loading.
Statistical analysis
Data were analyzed using SPSS 22.0 software. All data were presented as the mean±SD. Differences among the multiple treatment groups were evaluated by one-way analysis of variance. The comparison between the two groups was performed by the Student’s t-test. A P value <0.05 was regarded as statistically significant.
Results
Vitamin B6 regulated the acid–base imbalance and hypoxia induced by Isocarbophos
To investigate the influence of Isocarbophos and Vitamin B6 on the amount of the blood gas, blood gas analysis in the offspring rats after treatments was performed. As shown in Table 1, the total blood CO2 concentration (tCO2) in the Isocarbophos group was significantly increased compared with that in the saline-treated control group, while the tCO2 in the Isocarbophos+Vitamin B6 group was significantly decreased compared with that in the Isocarbophos group. This suggests that co-treatment with Vitamin B6 significantly attenuates the increase in tCO2 induced by Isocarbophos. However, it should be noted that the Isocarbophos+Vitamin B6+Fingolimod group showed a 1.7-fold higher tCO2 than the Isocarbophos+Vitamin B6 group, suggesting that the inhibitory effect of Vitamin B6 on Isocarbophos may be disrupted by Fingolimod, a specific S1P inhibitor. Meanwhile, other blood gas indexes, including actual bicarbonate (AB), standard bicarbonate (SB), base excess extracellular fluid [BE(ecf)], and base excess of blood [BE(B)] in the rats after different treatments showed no significant changes among different treatments. These data indicate that Vitamin B6 can significantly recover the acid–base imbalance and rescue the hypoxia induced by Isocarbophos.
Blood gas analysis of arterial blood in offspring rats after different treatments
Group . | AB (mM) . | SB (mM) . | BE(ecf) (mM) . | BE(B) (mM) . | tCO2 (mM) . |
---|---|---|---|---|---|
Saline | 22.83±4.82 | 17.55±3.62 | −4.32±0.63 | −6.73±0.62 | 22.33±5.41 |
Vitamin B6 | 23.25±5.43 | 15.64±4.89 | −4.58±0.75 | −6.46±0.84 | 23.54±4.66 |
Fingolimod | 22.55±4.67 | 16.84±6.86 | −4.74±0.54 | −6.92±0.26 | 38.33±6.46# |
Isocarbophos | 24.61±6.56 | 18.12±5.73 | −4.12±0.58 | −6.33±0.56 | 39.53±6.12# |
Isocarbophos+Vitamin B6 | 21.22±6.25 | 17.65±3.74 | −4.91±0.66 | −5.92±0.74 | 29.13±5.35* |
Isocarbophos+Vitamin B6+Fingolimod | 22.34±5.63 | 16.73±4.66 | −4.82±0.69 | −6.61±0.76 | 37.86±6.92& |
Group . | AB (mM) . | SB (mM) . | BE(ecf) (mM) . | BE(B) (mM) . | tCO2 (mM) . |
---|---|---|---|---|---|
Saline | 22.83±4.82 | 17.55±3.62 | −4.32±0.63 | −6.73±0.62 | 22.33±5.41 |
Vitamin B6 | 23.25±5.43 | 15.64±4.89 | −4.58±0.75 | −6.46±0.84 | 23.54±4.66 |
Fingolimod | 22.55±4.67 | 16.84±6.86 | −4.74±0.54 | −6.92±0.26 | 38.33±6.46# |
Isocarbophos | 24.61±6.56 | 18.12±5.73 | −4.12±0.58 | −6.33±0.56 | 39.53±6.12# |
Isocarbophos+Vitamin B6 | 21.22±6.25 | 17.65±3.74 | −4.91±0.66 | −5.92±0.74 | 29.13±5.35* |
Isocarbophos+Vitamin B6+Fingolimod | 22.34±5.63 | 16.73±4.66 | −4.82±0.69 | −6.61±0.76 | 37.86±6.92& |
After Isocarbophos treatment, arterial blood of offspring rats was collected from left ventricles for blood gas analysis. AB: actual bicarbonate; SB: standard bicarbonate; [BE(ecf)]: base excess extracellular fluid; [BE(B)]: base excess of blood; tCO2: total carbon dioxide. Data are expressed as the mean±SD, n = 8.
P<0.05 vs Saline group.
P<0.05 vs Isocarbophos group.
P<0.05 vs Isocarbophos+Vitamin B6.
Blood gas analysis of arterial blood in offspring rats after different treatments
Group . | AB (mM) . | SB (mM) . | BE(ecf) (mM) . | BE(B) (mM) . | tCO2 (mM) . |
---|---|---|---|---|---|
Saline | 22.83±4.82 | 17.55±3.62 | −4.32±0.63 | −6.73±0.62 | 22.33±5.41 |
Vitamin B6 | 23.25±5.43 | 15.64±4.89 | −4.58±0.75 | −6.46±0.84 | 23.54±4.66 |
Fingolimod | 22.55±4.67 | 16.84±6.86 | −4.74±0.54 | −6.92±0.26 | 38.33±6.46# |
Isocarbophos | 24.61±6.56 | 18.12±5.73 | −4.12±0.58 | −6.33±0.56 | 39.53±6.12# |
Isocarbophos+Vitamin B6 | 21.22±6.25 | 17.65±3.74 | −4.91±0.66 | −5.92±0.74 | 29.13±5.35* |
Isocarbophos+Vitamin B6+Fingolimod | 22.34±5.63 | 16.73±4.66 | −4.82±0.69 | −6.61±0.76 | 37.86±6.92& |
Group . | AB (mM) . | SB (mM) . | BE(ecf) (mM) . | BE(B) (mM) . | tCO2 (mM) . |
---|---|---|---|---|---|
Saline | 22.83±4.82 | 17.55±3.62 | −4.32±0.63 | −6.73±0.62 | 22.33±5.41 |
Vitamin B6 | 23.25±5.43 | 15.64±4.89 | −4.58±0.75 | −6.46±0.84 | 23.54±4.66 |
Fingolimod | 22.55±4.67 | 16.84±6.86 | −4.74±0.54 | −6.92±0.26 | 38.33±6.46# |
Isocarbophos | 24.61±6.56 | 18.12±5.73 | −4.12±0.58 | −6.33±0.56 | 39.53±6.12# |
Isocarbophos+Vitamin B6 | 21.22±6.25 | 17.65±3.74 | −4.91±0.66 | −5.92±0.74 | 29.13±5.35* |
Isocarbophos+Vitamin B6+Fingolimod | 22.34±5.63 | 16.73±4.66 | −4.82±0.69 | −6.61±0.76 | 37.86±6.92& |
After Isocarbophos treatment, arterial blood of offspring rats was collected from left ventricles for blood gas analysis. AB: actual bicarbonate; SB: standard bicarbonate; [BE(ecf)]: base excess extracellular fluid; [BE(B)]: base excess of blood; tCO2: total carbon dioxide. Data are expressed as the mean±SD, n = 8.
P<0.05 vs Saline group.
P<0.05 vs Isocarbophos group.
P<0.05 vs Isocarbophos+Vitamin B6.
Vitamin B6 significantly improved the vasoconstriction function of the posterior cerebral artery in rats induced by Isocarbophos
Previous studies have reported that long-term low-dose intragastric administration of Isocarbophos induces abnormalities of vascular structures and endothelium-dependent diastolic functions in the middle and posterior cerebral arteries of rats [25]. Therefore, we evaluated the function of vasoconstriction by measuring the ACh-induced EDR in posterior cerebral artery. As shown in Fig. 1A, Isocarbophos treatment significantly reduced the Emax compared with the control group. Emax in the Isocarbophos+Vitamin B6 co-treated group was increased by 1.9 folds compared with in the Isocarbophos-treated group, suggesting the protective role of Vitamin B6. However, Emax in the Isocarbophos+Vitamin B6+Fingolimod co-treated group was still very low, which indicated that Fingolimod treatment could abolish the protective role of Vitamin B6. EC50 in the treated groups was found to be completely opposite to Emax (Fig. 1B). Collectively, these results suggest that Vitamin B6 has an obvious protective effect on the vasoconstriction function of the posterior cerebral artery in offspring rats.
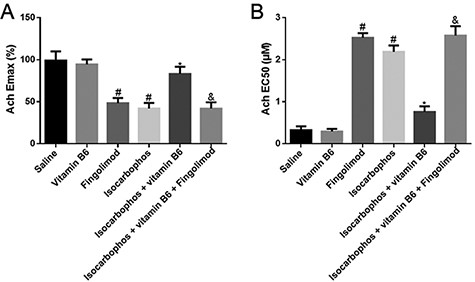
EDR measurement Ach-induced Emax (A) and EC50 (B) of posterior cerebral artery in different treatment groups. n=8. #P<0.05 vs Saline group; *P<0.05 vs Isocarbophos group; &P<0.05 vs Isocarbophos+Vitamin B6.
Vitamin B6 significantly improved the injury of posterior cerebral artery in offspring rats induced by Isocarbophos
Long-term low-dose exposure of Isocarbophos could cause vascular injury in offspring rats. To confirm this, posterior cerebral arteries of offspring rats in different treatment groups were observed under a posture microscope. As shown in Fig. 2A, the endothelium of the posterior cerebral arteries was rough and uneven with congestion and bleeding points in the Isocarbophos group compared with that in the saline-treated control group, while the rough and uneven endothelium with congestion and bleeding points in the Isocarbophos+Vitamin B6 group was significantly improved compared with that in the Isocarbophos group. However, the Isocarbophos+Vitamin B6+Fingolimod group still had different degrees of roughness and uneveness with congestion and bleeding points in the posterior cerebral artery endothelium compared with the Isocarbophos+Vitamin B6 group. Then, H&E staining was used to investigate the changes of vascular endothelial and smooth muscle structure in different treatment groups. As shown in Fig. 2B, compared with the saline-treated group, in the Isocarbophos group, the ECs of the vascular wall were detached, the inner wall was rough, the smooth muscle cells were hyperproliferative disorderly, and some elastic membranes were broken. Meanwhile, compared with the Isocarbophos group, vascular ECs were lost and smooth muscle cell proliferation disorder was significantly improved in the Isocarbophos+Vitamin B6 group. More importantly, it should be noted that the Isocarbophos+Vitamin B6+Fingolimod group showed a significant increase in ECs detached from the vascular wall, rough inner wall, smooth muscle cell disorder, and rupture of part of the elastic membrane, compared with the Isocarbophos+Vitamin B6 group.
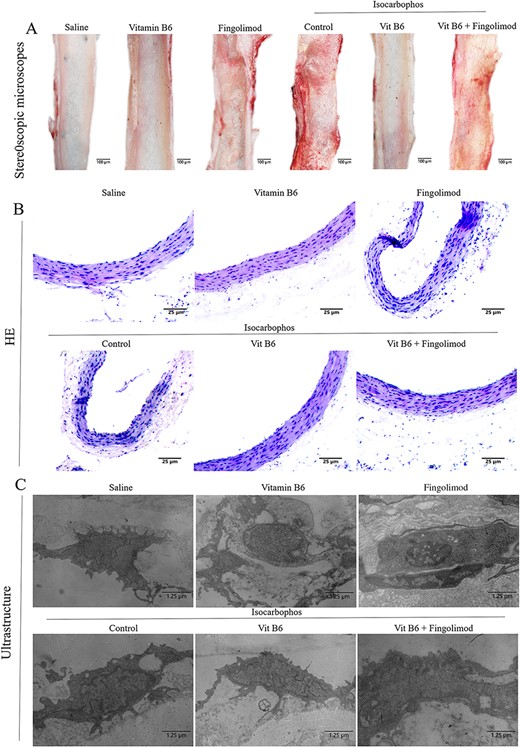
Injury analysis of posterior cerebral artery (A) Optical images of endothelial structure of posterior cerebral artery in offspring rats. (B) H&E staining images of posterior cerebral artery in offspring rats after different treatments. (C) Electron microscopy images of vascular endothelium of posterior cerebral artery in offspring rats.
Furthermore, we observed the ultrastructural changes of posterior cerebral artery endothelium in offspring rats. As shown in Fig. 2C, compared with the saline-treated group, the number of phagocyte vesicles was decreased, the cytoplasm was deeply stained, the mitochondria were obviously swollen with vacuolization change, and the rough endoplasmic reticulum had a slight degranulation phenomenon in the Isocarbophos group. Compared with those in the Isocarbophos group, the protrusions on the surface of vascular ECs in the Isocarbophos+Vitamin B6 group were significantly reduced, the number of phagocyte vesicles was increased, and the rough endoplasmic reticulum degranulation phenomenon was significantly improved. More importantly, EC protrusions and the decrease in the number of phagocytes in the Isocarbophos+Vitamin B6+Fingolimod group were similar to those in the Isocarbophos group, compared with the Isocarbophos+Vitamin B6 group.
These data indicate that Vitamin B6 attenuates Isocarbophos-induced vascular endothelial injury, but this improvement is eliminated by Fingolimod.
Vitamin B6 significantly decreased the MDA content and increased the SOD activity and NO content in plasma and vascular tissue of offspring rats
Some studies have demonstrated that long-term exposure to organophosphorus compounds causes vascular dysfunction, inflammatory oxidative damage, and cardiovascular diseases [26–28]. In order to evaluate the oxidative damage induced by Isocarbophos, the contents of MDA and NO and the activity of SOD in plasma and vascular tissue of offspring rats were determined. The results showed that compared with those in the saline-treated group, the levels of NO and SOD in the Isocarbophos group were significantly decreased, while the content of MDA was significantly increased. In addition, co-treatment with Vitamin B6 significantly reversed the decrease of NO and SOD and the increase of MDA compared with Isocarbophos only, suggesting a protective effect of Vitamin B6 against oxidative injury. However, compared with those in the Isocarbophos+Vitamin B6 group, the levels of NO and SOD were significantly decreased and the content of MDA was significantly increased in the Isocarbophos+Vitamin B6+Fingolimod group, indicating that Fingolimod diminished the protective effect of Vitamin B6. The trend of oxidation indexes in vascular tissue is consistent with that in plasma (Fig. 3B). These results suggest the anti-oxidative effects of Vitamin B6.
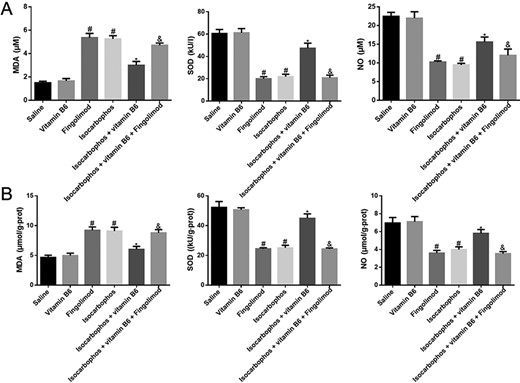
Quantification of the NO content, SOD activity, and MDA content in plasma blood and vascular tissue (A) Plasma oxidation indexes in offspring rats. (B) Oxidative indexes of vascular tissue in offspring rats. n=8. #P<0.05 vs Saline group; *P<0.05 vs Isocarbophos group; &P<0.05 vs Isocarbophos+Vitamin B6.
Vitamin B6 significantly reduced the elevation of inflammatory cytokines induced by Isocarbophos
Next, we measured the levels of inflammatory factors in the blood and vascular tissues of offspring rats. Similarly, Isocarbophos-induced oxidative damage of plasma and vascular tissues was observed. Results showed that treatment with Isocarbophos significantly increased the levels of ICAM-1, VCAM-1, IL-1, and IL-6 in plasma and vascular tissues compared with those in the saline-treated group (Fig. 4), while the levels of ICAM-1, VCAM-1, IL-1, and IL-6 in plasma and vascular tissues in the Isocarbophos+Vitamin B6 group were significantly decreased compared with those in the Isocarbophos group. More importantly, the levels of ICAM-1, VCAM-1, IL-1, and IL-6 were remarkably lower in the Isocarbophos+Vitamin B6 group than in the Isocarbophos+Vitamin B6+Fingolimod group. These results indicated that Vitamin B6 could alleviate the Isocarbophos-induced inflammatory factor elevation, which suggested the anti-inflammatory effects of Vitamin B6, but this activity could be abolished by Fingolimod.

Quantification of the levels of ICAM-1, VCAM-1, IL-1, and IL-6 in plasma blood and vascular tissue (A) Plasma inflammatory factor in offspring rats. (B) Inflammatory factors of arteriae cerebral posterior vascular in offspring rats. n=8. #P<0.05 vs Saline group; *P<0.05 vs Isocarbophos group; &P<0.05 vs Isocarbophos+Vitamin B6.
Vitamin B6 significantly reduced the overexpression of Caspase-3 and cell apoptosis
Studies have shown that Isocarbophos can significantly induce the increase of vascular injury, oxidation index,and inflammatory factors and may lead to cell apoptosis and necrosis[29].Therefore, in this study,we used immunohistochemical staining of Caspase-3 to investigate apoptosis of posterior cerebral artery cells. As shown in Fig. 5,the expression of Caspase-3 in the Isocarbophos group was significantly higher than that in the saline-treated control group, while the expression of Caspase-3 in the Isocarbophos+Vitamin B6 group was significantly decreased compared with that in the Isocarbophos group. Moreover, the Isocarbophos+Vitamin B6+Fingolimod group showed serious cell apoptosis compared with the Isocarbophos+Vitamin B6 group.These results indicated that Vitamin B6 has an effective anti-apoptosis ability, but this ability could be abolished by Fingolimod.
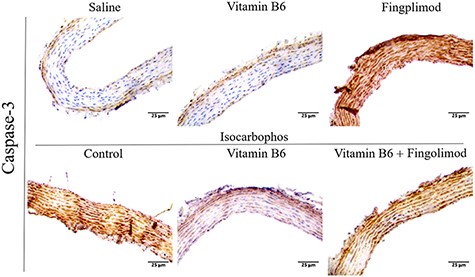
Expression of Caspase-3 in the posterior cerebral arteries of offspring rats in different treatment groups
Vitamin B6 significantly inhibits the decrease of S1P content induced by Isocarbophos
Subsequently, the S1P level was measured in plasma and vascular tissues of offspring rats. As shown in Fig. 6, S1P levels in the Isocarbophos group was obviously decreased compared with that in the saline-treated control group, while under Vitamin B6 combination therapy, S1P levels in the plasma and blood vessel tissues were increased obviously compared with those in the Isocarbophos group. S1P level was significantly higher in the Isocarbophos+Vitamin B6 group than that in the Isocarbophos+Vitamin B6+Fingolimod group. These results suggest that Vitamin B6 can effectively inhibit the decrease of the S1P content induced by Isocarbophos and that the beneficial effect of Vitamin B6 can be eliminated by Fingolimod.

Quantification of S1P contents in plasma blood and vascular tissue (A) Plasma S1P contents in offspring rats. (B) S1P contents of arteriae cerebral posterior vascular in offspring rats. n=8. #P<0.05 vs Saline group; *P<0.05 vs Isocarbophos group; &P<0.05 vs Isocarbophos+Vitamin B6.
Vitamin B6 increased the protein level of S1P1 in vascular tissues of offspring rats
To explore the mechanism of Vitamin B6-mediated inhibition for Isocarbophos-induced vascular injury, the S1PR expression in vascular tissues of offspring rats after different treatments was studied. Immunofluorescence staining images showed that the expression of the S1P1 receptor (red fluorescent signal) was significantly inhibited in the Isocarbophos group compared with that in the saline-treated control group, while the expression of the S1P1 receptor in the Isocarbophos+Vitamin B6 group was markedly increased compared with that in the Isocarbophos group (Fig. 7A). However, the expression of the S1P1 receptor in the Isocarbophos+Vitamin B6 group was significantly higher than that in the Isocarbophos+Vitamin B6+Fingolimod group. The expression of the S1P2 receptor (green fluorescent signals) in these treated groups did not have obvious changes as compared with the control group.
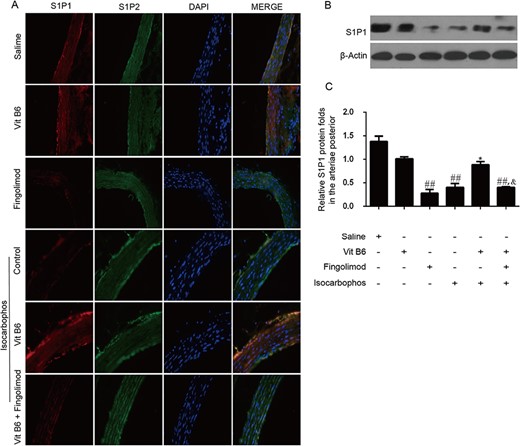
S1P receptor expression in different treatment groups (A) Immunofluorescence staining images of S1P1 and S1P2 receptor expression in the vascular tissues of offspring rats after different treatments. Red fluorescence signals indicate the S1P1 receptor expression, green fluorescence signals indicate the S1P2 receptor expression, and blue fluorescence signals are the cell nuclei stained by DAPI. (B) Expression of S1P1 receptor determined by western blot analysis. (C) Quantitative analysis of the S1P1 receptor expression in (B). ##P<0.01 vs Saline group; *P<0.05 vs Isocarbophos group; &P<0.05 vs Isocarbophos+Vitamin B6.
The expression of the S1P1 receptor was further confirmed by western blot analysis. As compared with that in the saline-treated control group, the expression of the S1P1 receptor was decreased in the Isocarbophos group (Fig. 7B,C). In particular, the expression of the S1P1 receptor in the Isocarbophos+Vitamin B6 group was significantly increased compared with that in the Isocarbophos group. More importantly, the expression of the S1P1 receptor in the Isocarbophos+Vitamin B6+Fingolimod group was significantly lower than that in the Isocarbophos+Vitamin B6 group.
The results of immunofluorescence staining and western blot analysis indicated that Isocarbophos inhibited the expression of the S1P1 receptor in vascular tissues, while Vitamin B6 had a protective role against this inhibition. However, as Fingolimod compromised the protective effect of Vitamin B6, the expression of the S1P1 receptor was inhibited.
Discussion
In this study, we investigated in detail the effects of Vitamin B6 and high levels of S1P on vascular injury in offspring rats. Results showed that compared with Isocarbophos, Vitamin B6 significantly alleviated vascular injury by increasing antioxidant indexes and reducing the amount of inflammatory factors. In addition, we also found that the expression of Caspase-3 was decreased obviously after the combined treatment with Vitamin B6, indicating that Vitamin B6 has an effective anti-apoptosis ability. Moreover, Vitamin B6 can effectively inhibit the decrease of the S1P content induced by Isocarbophos, but Fingolimod can eliminate the beneficial effect of Vitamin B6. In general, our study suggests that Vitamin B6 has an effective vascular protection activity. In addition, we established a chronic poisoning model of Isocarbophos to elucidate the mechanism of organophosphorus compounds on posterior cerebral artery injury in offspring rats. We found that Vitamin B6 protects against vascular injury by inhibiting S1P1 damage. This result provides a preliminary basis for Vitamin B6 as a potential target for the treatment of Isocarbophos-induced vascular injury in offspring rats.
Vitamin B6, as an essential cofactor for enzymes involved in various metabolic activities, participates in various metabolic reactions, which has rarely been reported in the field of cardiovascular diseases. Our data provide the evidence that Vitamin B6 inhibits vascular injury by modulating S1P expression. Although our previous study showed the protective effect of Vitamin B6 on VD, this study focused on the regulatory effect of Vitamin B6 on posterior cerebral artery injury in offspring rats and its potential mechanism.
We found that Vitamin B6 significantly improved vascular injury in rats induced by Isocarbophos, which is consistent with the results of previous studies. Many investigations indicated that S1P can induce vascular maturation, angiogenesis, and regulate vascular permeability [30–35]. The regulation of these functions mainly depends on the S1PR, which exists in vascular ECs and smooth muscle cells. Previous studies have found that S1P can induce EC migration and adhesion and promote the formation of tubular structures [36–39]. S1P1 also plays an important role in the integrity and survival of vascular ECs [40]. In cardiovascular ECs, S1P inhibits ceramide-induced apoptosis of vascular ECs through the mitogen-activated protein kinase signaling pathway [41]. On the cardiovascular endothelial, S1P can also stimulate the production of NO and make the blood vessels dilate, thereby regulating the vascular tension [42]. Since S1P plays such an important role in regulating vascular function, this study aimed to investigate whether S1P is related to the inhibitory effect of Vitamin B6 on posterior cerebral artery injury. H&E staining showed that Fingolimod could eliminate the beneficial effect of Vitamin B6.
In addition, immunofluorescence and western blot analysis of the posterior cerebral artery of offspring rats further confirmed that S1P plays an important role in Vitamin B6-induced inhibition of vascular injury, suggesting that S1P is involved in the protective effect of Vitamin B6 on the posterior cerebral artery injury. In this experiment, we found that Isocarbophos treatment could induce the increase of CO2 content in blood and the increase of NO content and SOD activity in blood and vascular tissues, but it could induce the decrease of MDA content and inflammatory factor levels in blood and vascular vessels. Macrophages can be activated by the stimulation of organophosphorus compounds, inducing the release of various cytokines such as IL-1β, TNF-α, ICAM-1, and VCAM-1 [43]. Similar results were observed in this study. We propose that IL-1β, TNF-α, ICAM-1, and VCAM-1 should be considered as sensitive indicators to reflect the status of organophosphorus poisoning and evaluate the prognosis. However, S1P can also induce coronary artery contraction [44] and middle cerebral artery contraction [45], which may be related to the differential distribution and expression of S1PR on the vascular wall, thus achieving different physiological functions [46]. By detecting the S1P content in the plasma blood and posterior cerebral arteries of offspring rats, we found that the Isocarbophos treatment significantly reduced the S1P content, while Vitamin B6 was able to inhibit this Isocarbophos-induced S1P content decrease. Therefore, our data provide a basis for the treatment of vascular injury.
To investigate whether S1PR is a target for Vitamin B6-mediated injury inhibition, Fingolimod [47,48] was used to block the S1PR. We found that the offspring rats with blocked S1PR showed blood and vascular morphological injuries similar to those treated with Isocarbophos, which indicated that S1PR is closely related to Vitamin B6-mediated inhibition of vascular function injury. Tamama et al. [49] verified that S1P inhibited the PDGF-induced vascular smooth muscle cell migration through the S1P2 receptor pathway. Since S1P1 and S1P2 are very important in the development of vessels, it is necessary to determine which subtype of S1PR is responsible for the Vitamin B6-mediated inhibition of vascular dysfunction. Immunofluorescence staining of the posterior cerebral arteries showed that Isocarbophos significantly down-regulated the expression of S1P1, while the S1P2 expression did not change significantly. The combined treatment of Vitamin B6 increased the S1P1 expression without affecting the S1P2 expression. However, after combined treatment of Isocarbophos+Vitamin B6+Fingolimod, S1P1 expression was decreased. Similar results were obtained by western blot analysis. These results suggested that Vitamin B6 inhibited Isocarbophos-induced vascular injury possibly through affecting S1P1.
In summary, we confirmed that a high expression of S1P1 could significantly improve the vascular injury induced by Isocarbophos in offspring rats. Nevertheless, our study still has some limitations that should be further investigated in the future. For example, the exact mechanism by which Vitamin B6 regulates S1P expression in cerebral artery injury and the detection of S1PR in the artery. To better understand the mechanism by which Vitamin B6 alleviates Isocarbophos-induced cerebral artery injury in offspring rats, future studies may focus on the relationship between Vitamin B6 and the targets identified in this study.
Funding
This work was supported by the grants from the National Natural Science Foundation of China (Nos. 81874312 and U1804197), the Research Foundation of Henan Province (Nos. 202300410308, 2018GGJS102, 18HASTIT047, ZD2020006, 219906, and 192102310083), and the Research Foundation of Xinxiang Medical University (Nos. XYBSKYZZ201626, XYBSKYZZ505319, and YJSCX202041Y).
Conflict of Interest
The authors declare that they have no conflict of interest.
References
Author notes
Yanhua Liu1, Kunli Yang contributed equally to this work.