-
PDF
- Split View
-
Views
-
Cite
Cite
Rewati Raman Bhattarai, T Jayasree Joshi, N U Sruthi, Pavuluri Srinivasa Rao, Stuart Johnson, Effects of extrusion cooking on nutritional and health attributes of sorghum and millets: special reference to protein and starch digestibility, International Journal of Food Science and Technology, Volume 60, Issue 1, January 2025, vvae093, https://doi.org/10.1093/ijfood/vvae093
- Share Icon Share
Abstract
Millets, including sorghum grain, are gaining global interest for their rich nutritional profile and potential to develop healthy staple foods. They also have a propensity for low protein and starch digestibility and are high in polyphenolics. These properties provide challenges for designing nutritious and consumer-acceptable foods from sorghum that meet the needs of those under energy/protein malnourishment. In contrast, the high nutritional and polyphenolic profiles and low digestibility make sorghum the ideal grain food to assist those over-energy, malnourished and at risk of obesity and related chronic diseases, including those linked to oxidative stress. Sorghum lacks gluten-forming proteins; therefore, producing sorghum foods with desirable textures is challenging. Extrusion cooking of sorghum may be one processing solution to overcome these barriers and design staple ready-to-eat food products with consumer acceptability. This paper critically reviews the current state of knowledge on the influence of extrusion cooking on protein quality and starch digestibility of sorghum and millet-composite extruded foods.
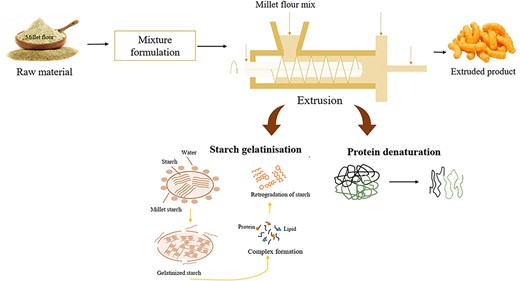
Introduction
Sorghum and millets are often considered together as they are all classified into C4 (tropical) cereals. However, there are some key differences between these cereal grains. The term millet does not refer to a single species but is used collectively for tiny-grained cereals, which are generally smaller and harder than sorghum (Taylor & Duodu, 2019). Sorghum is a distinct species often grouped with millets due to similar agronomic traits, nutritional profiles, and applications, thus usually used interchangeably. They are both climate-resilient food grains with significant potential in the food and beverage industries. They are gluten-free and high in nutrition. Bioactive phytochemicals like phenolic acids, procyanidins, flavonoids, etc. are abundant in sorghum and millets (Kaufman et al., 2013; Shen et al., 2015). These grains can be processed into grits, starch, flour, and flakes, and are used to produce diverse industrial products. The grains have also been reported to have free radical scavenging activity, high antimicrobial properties, anti-inflammatory activity, reduced oxidative stress, and cancer prevention properties (Rao et al., 2017). However, sorghum and other millet grains in the food processing industry are still limited due to antinutritional factors responsible for decreased mineral availability and low protein and starch digestibility (Alfieri et al., 2017). Besides this, starch granules in millets are encased in a dense protein matrix, rendering them highly resistant to microbial and enzymatic action in the small intestine (Mahasukhonthachat et al., 2010a). The highly resistant structure must be destroyed or reduced to increase millet starch’s digestibility.
Processing is vital in altering millet flour’s physical, organoleptic, and functional attributes (Sruthi & Rao, 2021). Traditional methods used in processing these grains include fermentation, soaking, germination, and malting. However, these techniques have significant drawbacks regarding storage stability, consumer acceptability, convenience, and product versatility. Consequently, it is imperative to invest in process technologies that enhance the functional and nutritional characteristics of millet products employed in the production of various health-promoting food items (Joshi et al., 2023). Enhanced functionality is vital for the mass production of convenient food items. The extrusion process, which uses heat, pressure, and shear forces, has been shown to alter starch’s physicochemical attributes. During the process, gelatinisation of starch occurs due to heating and shearing. Extrusion cooking also destroys antinutrients in food materials, increasing the bioaccessibility of macronutrients (Nikmaram et al., 2017). The process of extrusion markedly enhances the viscoelastic properties of dough by modifying the molecular arrangement of starch and protein constituents in unprocessed flour (Kumar et al., 2021; Patil et al., 2016). Numerous researchers have effectively demonstrated that the properties related to pasting and rheology of millet flour can be controlled and modulated through the application of the extrusion technique, thereby influencing its overall quality and performance in various culinary applications (Gulati et al., 2016; Kumar et al., 2021). This paper focuses on the latest advancements and gaps in comprehending the impact of extrusion process parameters on major nutrients and phytochemical components of foods made with millet flours, more specifically (a) protein and starch digestibility, and (b) nutritional quality of millet-based extruded foods. These food attributes are relevant to two important nutritional/health paradigms, specifically (a) the formulation of millet-based extruded products aimed at satisfying the dietary needs of swiftly expanding urban populations in Africa, particularly vulnerable groups such as children under 5 years of age and lactating or pregnant women who are at a heightened risk for protein–energy malnutrition, and (b) the management of chronic lifestyle-related diseases associated with overnutrition, such as type 2 diabetes mellitus.
Sorghum and millet proteins
The protein concentration found in sorghum and millet grains is approximately 8%–15% (db), comparable with cereal grains (Taylor & Taylor, 2017). Among the various major and minor millet species, pearl millet is noted for possessing the highest protein content. In contrast, finger millet is the least owing to their respective germ and endosperm sizes. In the case of sorghum, the kernel endosperm is the major protein repository. Its nutrient availability and functional attributes are greatly affected by the nature of these proteins within the endosperm (Bean & Ioerger, 2014). Proteins derived from cereal grains have been categorised based on their solubility in aqueous environments, with classifications including globulins, albumins, prolamins, and glutelins. The water-soluble albumins and salt-soluble globulins predominate the sorghum germ and comprise 10%–30% of the total grain protein. However, the prolamin kafirin dominates, comprising about 80% of the endosperm protein (Belton et al., 2006) and serving as the primary storage protein. As Belton and Taylor (2004) discussed, kafirins are a type of prolamin that contains high amounts of nonessential amino acids such as glutamine and proline. Similar to other cereals, these proteins exhibit suboptimal levels of essential amino acids, notably lysine, threonine, tryptophan, and methionine. To ameliorate this deficiency, legumes such as cowpea, soybean, and groundnut are frequently paired with cereals like sorghum, maize, and rice, resulting in food products that boast enhanced protein and lysine levels compared with those derived solely from cereals (Asare et al., 2010a; Pelembe et al., 2002).
Kafirin proteins in sorghum are categorised into four types: α-, β-, γ-, and δ-kafirins. Among these, both β- and γ-kafirins exhibit a significant abundance of the sulphur-containing amino acid cysteine (Shull et al., 1992), which facilitates the formation of various disuphide cross-linked polymers (El Nour et al., 1998). The protein architecture in sorghum grains is characterised by spherical entities, featuring an α-kafirin core surrounded by a β- and γ-kafirin outer layer that is extensively cross-linked (Shull et al., 1992). These protein entities are situated within a network of starch granules and glutelin protein (de Mesa Stonestreet et al., 2011). Upon the cooking of sorghum, the quantity of these cross-links may escalate, consequently diminishing the protein’s digestibility (Oria et al., 1995). As reviewed by Duodu et al. (2003), the poor digestibility of sorghum protein can be ascribed to multiple factors: the significant cross-linking of kafirins, the hydrophobic features of these proteins, the structural arrangement of protein bodies within the grain, and their interactions with polyphenols, starch, and phytic acid.
Similar to sorghum, the distribution and relative composition of proteins in millets are influenced by various factors such as environment, cropping practices, and variety (Bean & Ioerger, 2014). The aggregate protein concentration in millets is documented to vary significantly, ranging between 4% and 20%. In contrast to sorghum, which possesses a relatively small germ and, as a result, lower lysine concentrations, both pearl millet and finger millet are noted to exhibit a more elevated germ-to-endosperm ratio, thereby suggesting a greater potential for lysine levels (Bean et al., 2018). Apart from the basic class of proteins, a prolamin-like fraction (cross-linked prolamins) was also prominent in pearl millet but less than its content in sorghum. This could suggest that the pearl millet prolamins are less susceptible to cross-linking and are more nutritionally available than sorghum. A similar composition was also found for finger millet grains, with the prolamin and prolamin-like fraction forming around 46.6%, followed by 41.17% glutelin and glutelin-like proteins, and much smaller fractions of albumins and globulins (Dharmaraj & Malleshi, 2011).
Notably, millet prolamins exhibit distinct solubility characteristics compared with sorghum kafirin, which might have arisen from variations in polymerisation or hydrophobic properties. Albumin and globulin proteins remain inadequately characterised in both sorghum and millet species; however, their amino acid profiles suggest that these fractions possess superior nutritional quality relative to other protein categories (Bean et al., 2018). Despite having elevated lysine concentrations compared with other protein fractions, the reduced overall proportion of these proteins within the total grain protein limits their influence on nutritional quality.
Nonetheless, from a nutritional and functional standpoint, sorghum and millet proteins do not play a significant role in flour functionality because most of their proteins are either isolated or entrapped in protein bodies, making them unavailable as functional ingredients (Hamaker & Bugusu, 2003). Upon cooking, the protein digestibility was reported to decrease, and factors such as the structure of protein and the presence of antinutrients contribute to the observed effect, with the formation of disulphide bonds proving to be the major factor (Duodu et al., 2003). Moreover, reports indicate that reduced kafirin solubility and heightened levels of oligomeric/polymeric proteins following wet cooking have influenced the functional properties of sorghum and millet proteins.
Sorghum and millet starch
Starch is found in mature sorghum and millet grains as discrete, water-insoluble granules with a size range of 4–35 μm with spherical or polyhedral shapes, which vary according to the location of the endosperm. The structure and distribution of these granules can also vary greatly (Benmoussa et al., 2006). Millets exhibit a starch content ranging from 51% to 79%, which is comparable with that of other principal cereals, and consist of amylose (AM) and amylopectin (AP). The starch content in sorghum varies between 56% and 76%. Specifically, the AM content in pearl, finger, and proso millet has been documented to be 4%–38%, 14%–39%, and 0%–34%, respectively (Choi et al., 2004; Suma & Urooj, 2015). The molecular weight (MW) of AM and AP in sorghum starch has been indicated to range from 6 × 106 to 1 × 107 Da and from 8.5 × 107 to 3 × 108 Da, respectively. Likewise, the average degree of polymerisation for AM and AP has been reported as 1,100–1,400 and 9,000–13,000, respectively (Gaffa et al., 2004; Matalanis et al., 2009). The starch derived from millet displays a Type A diffraction pattern characterised by four principal peaks: a single peak (at 2θ = 23°), a double peak (at 2θ = 17°, 18°), and a pronounced sharp peak (at 2θ = 15°) (Wu et al., 2014). The crystallinity patterns of sorghum starch are akin to those of cereal grains, with a crystallinity percentage ranging from 23% to 31% (Boudries et al., 2009; Sun et al., 2014). An additional critical factor that delineates the molecular architecture of starch molecules is the chain length (CL). The CL significantly influences the crystallisation, gelatinisation, retrogradation, and pasting attributes of starch (Cai & Shi, 2010). The AM fraction within millet starch is categorised into long-chain amylose (LCAM) and short-chain amylose (SCAM) based on CL. Starches from pearl millet and finger millet are characterised by a high concentration of LCAM, with the LCAM: SCAM ratio exceeding that of foxtail millet (0.60) and proso millet (0.63) (Gaffa et al., 2004).
The characteristics of millet starches differ based on their AM content, CL, and degree of polymerisation. The waxy variety of proso millet has significantly greater crystallinity and enthalpy (ΔH) than its nonwaxy variety. This is attributed to its high AP content and degree of polymerisation (Yang et al., 2019). It indicates that waxy starches are more stable than non-waxy ones. The gelatinisation temperature (Tg) of starches increases as branch CL increases. However, increasing the amount of AP and short branch chains reduces their gelatinisation time (Chandra et al., 2014). The size of AM and AP units influences their functional attributes and possible applications as elements in diverse food formulations. Environmental conditions, the localisation of starch granules within the grain endosperm, and the nature of any treatments or modifications done significantly impact starch morphology (Wen et al., 2014). Indentations may appear randomly on the granule surface owing to the existence of protein groups and surface openings/pores (Zhu, 2014). Specific molecules can enter the granule matrix through these openings, which connect the central cavity of the granule to its surroundings. This occurrence is advantageous for altering starches. These openings permit water molecules and other hydroxyl ions to penetrate, disrupting the amorphous zones that encompass AM and diminishing AM’s limiting characteristics, enhancing starch hydration and expansion (Nor Nadiha et al., 2010). Such properties can be helpful in the manufacture of gelling agents and thickeners, requiring high water binding.
The functional, thermal, gelatinisation, rheological, and digestibility attributes of millet starch influence its applications in the food processing industry. Swelling and solubility patterns show how chains interact between the amorphous and crystalline regions and form associative bonding within the starch granules. Millet starches were found to have a lower swelling power than potato, rye, and wheat starches (Hoover, 2001). Due to strong granule bonding forces, millet starches are more swelling-resistant. The onset gelatinisation temperature (To) of different millet starches was reported to be 68.4, 66.7, 63.9, and 62.8 °C for proso, foxtail, finger, and pearl millet, respectively (Annor et al., 2014). The enthalpy (ΔH) of finger, proso, pearl, and foxtail millet was 13.2, 13.1, 12.3, and 11.8 J/g, respectively. The value of ΔH specifies the heat energy required to break intermolecular bonds; a higher ΔH demonstrates stronger bonding and vice versa.
Extrusion processing
Extrusion processing is a multipurpose technique that integrates mixing, crushing, cooking, sterilisation, expansion moulding, drying, baking, drying, and cooling operations (Wang et al., 2022). The continuous processing eventually results in product expansion, the characteristics of which depend on the feed moisture, barrel temperature, screw diameter and speed, and other extrusion parameters. Extrusion causes several modifications in the microstructure of products, including gelatinisation of starch, loss of crystallinity, protein denaturation, destruction of granule structure, degradation of glycosidic bonds, and formation of starch–protein and starch–lipid complexes. With the high temperature, pressure, and shear inside the barrel, the complex protein structure is expected to unfold, linearise, and recross link, which weakens the binding forces required to maintain the quaternary structures of the protein (Gao et al., 2022). Changes in protein conformation also occur owing to the reorganisation of spherical aggregation to fibrous, finally causing protein denaturation.
Similarly, a decrease in crystallinity can be observed in product starch with extrusion, with the starch getting partially or almost completely gelatinised after the process (Wang et al., 2022). Extrusion facilitates the breakdown of starch into low-molecular-weight entities, and these resultant fragments possess the potential to reorganise into quantifiable starch fractions. Insights were also drawn into the effect of extrusion on the phenolic composition of food, where depolymerisation of phenolic compounds was reported, affecting the bioaccessibility of proteins and other essential nutrients (Paucar-Menacho et al., 2022). The following sections detail the impact of extrusion processing on protein and starch digestibility’s and the bioactive composition of sorghum and millets.
Effect of extrusion on protein digestibility
Numerous processing methods have been explored to examine the impact of extrusion on the protein digestibility and amino acid content in sorghum, millets, and related food products. These extrusion studies are outlined in Table 1, and Figure 1 illustrates the significant structural changes in proteins caused by extrusion. A notable study from the 1980s, conducted by Maclean et al. (1983), highlighted the effectiveness of combining extrusion with decortication to enhance the digestibility and protein quality of sorghum-based weaning foods. In this study, nine malnourished male infants, aged 7–24 months, who were undergoing nutritional rehabilitation in a hospital setting, were given gelatinised liquid diets in a crossover design: 9 days on a control diet, followed by 9 days on a sorghum-based diet, and then back to the control diet for another 9 days. Subsequently, using a pepsin digestion assay, Mertz et al. (1984) evaluated the in vitro protein digestibility of extruded sorghum used in the Maclean study. They discovered that extrusion increased the digestibility of cooked sorghum gruel from 56.8% to 79%. Additionally, extrusion improved the solubility of kafirin in the sorghum, making it more soluble in aqueous ethanol, whereas raw sorghum required a reducing agent for solubilisation (Hamaker et al., 1994). These results underscore the potential benefits of extruding decorticated sorghum and millet grains to enhance the protein quality of weaning and infant foods. However, they do not provide detailed insights into how grain variety, extrudate formulation, or processing conditions affect protein quality.
Evidence of the effect of extrusion cooking on protein level, protein digestibility, and essential amino acid content of sorghum- and millet-containing foods.
Formulation . | Product . | Extrusion conditions . | Effect of extrusion . | Reference . |
---|---|---|---|---|
Sorghum only | ||||
White sorghum flour | Extruded flour | Moisture: 10%, 14%, and 18% db Temperature: 110 and 160 °C Die: 4 mm Feed rate: 40 kg/h Screw speed: 150 rpm | Change in sorghum protein conformation Secondary structures of native protein changed from α-helix to random conformation Decrease in protein bodies observed in sorghum flour owing to the shearing force during the extrusion cooking | Jafari et al. (2017) |
Whole grain red sorghum flour | Expanded extrudate | Single screw extruder Moisture: 14% Temperature: 182 °C Screw speed: 150 rpm Die: 3 mm | Extrusion increased IVPD but reduced available lysine | Llopart et al. (2014) |
Decorticated white sorghum α-amylase (thermostable) | Protein concentrate | Twin screw extruder (laboratory scale) Moisture: 12.0%–14.4% Screw speed: 350 rpm Flow rate: 1.48–2.25 kg/h Die: 2 mm circular | Protein content inversely related to moisture level in barrel and quadratically related to enzyme level in barrel (RSM modelling) Protein content enhanced by post-extrusion enzyme treatment when no enzyme in barrel Protein digestibility of concentrate was affected by moisture content in barrel and the interaction of moisture content with enzyme level in barrel (RSM modelling) | de Mesa-Stonestreet et al. (2012) |
Milled sorghum grain | Expanded extrudate | Twin-screw extruder Die: 2 mm Length: diameter ratio of 40:1 Feed rate: 2 kg/h Moisture content: 25%, 30%, and 40% Screw speed: 150, 220, and 300 rpm | Extrusion did not completely disrupt starch–protein interactions Certain extrusion conditions denatured protein bodies | Mahasukhonthachat et al. (2010a) |
Sorghum-containing composites | ||||
Sorghum Mung bean | Extruded snacks | Corotating and intermeshing twin-screw extruder Barrel dia: 25 mm Length: diameter ratio of 16:1 | Elevated IVPD values with increase in barrel temperature owing to the alteration in noncovalent interaction which opens the protein structure | Kaur et al. (2023) |
White, nontannin and red, tannin-containing sorghum flour Corn flour | Ring-shaped RTE cereals | Co-rotating twin screw pilot scale extruder Screw dia: 57 mm Length: diameter ratio of 13.5 | Cereals made from tannin containing sorghum showed low in vitro protein digestibility | Mkandawire et al. (2015) |
Wholegrain red sorghum flour (tannin-free) Maize flour | Extrudate (prototype healthy food) | Co-rotating twin screw extruder Moisture: 21.4%, 25.8% Temperature: 120, 150 °C Screw speed: 250, 450 rpm Flow rate: 2.3, 6.8 kg/h Die: 3 mm cylindrical | In vitro protein digestibility negatively associated with sorghum level and positively with final barrel zone temperature (factorial analysis) | Licata et al. (2014) |
Formulation . | Product . | Extrusion conditions . | Effect of extrusion . | Reference . |
---|---|---|---|---|
Sorghum only | ||||
White sorghum flour | Extruded flour | Moisture: 10%, 14%, and 18% db Temperature: 110 and 160 °C Die: 4 mm Feed rate: 40 kg/h Screw speed: 150 rpm | Change in sorghum protein conformation Secondary structures of native protein changed from α-helix to random conformation Decrease in protein bodies observed in sorghum flour owing to the shearing force during the extrusion cooking | Jafari et al. (2017) |
Whole grain red sorghum flour | Expanded extrudate | Single screw extruder Moisture: 14% Temperature: 182 °C Screw speed: 150 rpm Die: 3 mm | Extrusion increased IVPD but reduced available lysine | Llopart et al. (2014) |
Decorticated white sorghum α-amylase (thermostable) | Protein concentrate | Twin screw extruder (laboratory scale) Moisture: 12.0%–14.4% Screw speed: 350 rpm Flow rate: 1.48–2.25 kg/h Die: 2 mm circular | Protein content inversely related to moisture level in barrel and quadratically related to enzyme level in barrel (RSM modelling) Protein content enhanced by post-extrusion enzyme treatment when no enzyme in barrel Protein digestibility of concentrate was affected by moisture content in barrel and the interaction of moisture content with enzyme level in barrel (RSM modelling) | de Mesa-Stonestreet et al. (2012) |
Milled sorghum grain | Expanded extrudate | Twin-screw extruder Die: 2 mm Length: diameter ratio of 40:1 Feed rate: 2 kg/h Moisture content: 25%, 30%, and 40% Screw speed: 150, 220, and 300 rpm | Extrusion did not completely disrupt starch–protein interactions Certain extrusion conditions denatured protein bodies | Mahasukhonthachat et al. (2010a) |
Sorghum-containing composites | ||||
Sorghum Mung bean | Extruded snacks | Corotating and intermeshing twin-screw extruder Barrel dia: 25 mm Length: diameter ratio of 16:1 | Elevated IVPD values with increase in barrel temperature owing to the alteration in noncovalent interaction which opens the protein structure | Kaur et al. (2023) |
White, nontannin and red, tannin-containing sorghum flour Corn flour | Ring-shaped RTE cereals | Co-rotating twin screw pilot scale extruder Screw dia: 57 mm Length: diameter ratio of 13.5 | Cereals made from tannin containing sorghum showed low in vitro protein digestibility | Mkandawire et al. (2015) |
Wholegrain red sorghum flour (tannin-free) Maize flour | Extrudate (prototype healthy food) | Co-rotating twin screw extruder Moisture: 21.4%, 25.8% Temperature: 120, 150 °C Screw speed: 250, 450 rpm Flow rate: 2.3, 6.8 kg/h Die: 3 mm cylindrical | In vitro protein digestibility negatively associated with sorghum level and positively with final barrel zone temperature (factorial analysis) | Licata et al. (2014) |
(Continued)
Evidence of the effect of extrusion cooking on protein level, protein digestibility, and essential amino acid content of sorghum- and millet-containing foods.
Formulation . | Product . | Extrusion conditions . | Effect of extrusion . | Reference . |
---|---|---|---|---|
Sorghum only | ||||
White sorghum flour | Extruded flour | Moisture: 10%, 14%, and 18% db Temperature: 110 and 160 °C Die: 4 mm Feed rate: 40 kg/h Screw speed: 150 rpm | Change in sorghum protein conformation Secondary structures of native protein changed from α-helix to random conformation Decrease in protein bodies observed in sorghum flour owing to the shearing force during the extrusion cooking | Jafari et al. (2017) |
Whole grain red sorghum flour | Expanded extrudate | Single screw extruder Moisture: 14% Temperature: 182 °C Screw speed: 150 rpm Die: 3 mm | Extrusion increased IVPD but reduced available lysine | Llopart et al. (2014) |
Decorticated white sorghum α-amylase (thermostable) | Protein concentrate | Twin screw extruder (laboratory scale) Moisture: 12.0%–14.4% Screw speed: 350 rpm Flow rate: 1.48–2.25 kg/h Die: 2 mm circular | Protein content inversely related to moisture level in barrel and quadratically related to enzyme level in barrel (RSM modelling) Protein content enhanced by post-extrusion enzyme treatment when no enzyme in barrel Protein digestibility of concentrate was affected by moisture content in barrel and the interaction of moisture content with enzyme level in barrel (RSM modelling) | de Mesa-Stonestreet et al. (2012) |
Milled sorghum grain | Expanded extrudate | Twin-screw extruder Die: 2 mm Length: diameter ratio of 40:1 Feed rate: 2 kg/h Moisture content: 25%, 30%, and 40% Screw speed: 150, 220, and 300 rpm | Extrusion did not completely disrupt starch–protein interactions Certain extrusion conditions denatured protein bodies | Mahasukhonthachat et al. (2010a) |
Sorghum-containing composites | ||||
Sorghum Mung bean | Extruded snacks | Corotating and intermeshing twin-screw extruder Barrel dia: 25 mm Length: diameter ratio of 16:1 | Elevated IVPD values with increase in barrel temperature owing to the alteration in noncovalent interaction which opens the protein structure | Kaur et al. (2023) |
White, nontannin and red, tannin-containing sorghum flour Corn flour | Ring-shaped RTE cereals | Co-rotating twin screw pilot scale extruder Screw dia: 57 mm Length: diameter ratio of 13.5 | Cereals made from tannin containing sorghum showed low in vitro protein digestibility | Mkandawire et al. (2015) |
Wholegrain red sorghum flour (tannin-free) Maize flour | Extrudate (prototype healthy food) | Co-rotating twin screw extruder Moisture: 21.4%, 25.8% Temperature: 120, 150 °C Screw speed: 250, 450 rpm Flow rate: 2.3, 6.8 kg/h Die: 3 mm cylindrical | In vitro protein digestibility negatively associated with sorghum level and positively with final barrel zone temperature (factorial analysis) | Licata et al. (2014) |
Formulation . | Product . | Extrusion conditions . | Effect of extrusion . | Reference . |
---|---|---|---|---|
Sorghum only | ||||
White sorghum flour | Extruded flour | Moisture: 10%, 14%, and 18% db Temperature: 110 and 160 °C Die: 4 mm Feed rate: 40 kg/h Screw speed: 150 rpm | Change in sorghum protein conformation Secondary structures of native protein changed from α-helix to random conformation Decrease in protein bodies observed in sorghum flour owing to the shearing force during the extrusion cooking | Jafari et al. (2017) |
Whole grain red sorghum flour | Expanded extrudate | Single screw extruder Moisture: 14% Temperature: 182 °C Screw speed: 150 rpm Die: 3 mm | Extrusion increased IVPD but reduced available lysine | Llopart et al. (2014) |
Decorticated white sorghum α-amylase (thermostable) | Protein concentrate | Twin screw extruder (laboratory scale) Moisture: 12.0%–14.4% Screw speed: 350 rpm Flow rate: 1.48–2.25 kg/h Die: 2 mm circular | Protein content inversely related to moisture level in barrel and quadratically related to enzyme level in barrel (RSM modelling) Protein content enhanced by post-extrusion enzyme treatment when no enzyme in barrel Protein digestibility of concentrate was affected by moisture content in barrel and the interaction of moisture content with enzyme level in barrel (RSM modelling) | de Mesa-Stonestreet et al. (2012) |
Milled sorghum grain | Expanded extrudate | Twin-screw extruder Die: 2 mm Length: diameter ratio of 40:1 Feed rate: 2 kg/h Moisture content: 25%, 30%, and 40% Screw speed: 150, 220, and 300 rpm | Extrusion did not completely disrupt starch–protein interactions Certain extrusion conditions denatured protein bodies | Mahasukhonthachat et al. (2010a) |
Sorghum-containing composites | ||||
Sorghum Mung bean | Extruded snacks | Corotating and intermeshing twin-screw extruder Barrel dia: 25 mm Length: diameter ratio of 16:1 | Elevated IVPD values with increase in barrel temperature owing to the alteration in noncovalent interaction which opens the protein structure | Kaur et al. (2023) |
White, nontannin and red, tannin-containing sorghum flour Corn flour | Ring-shaped RTE cereals | Co-rotating twin screw pilot scale extruder Screw dia: 57 mm Length: diameter ratio of 13.5 | Cereals made from tannin containing sorghum showed low in vitro protein digestibility | Mkandawire et al. (2015) |
Wholegrain red sorghum flour (tannin-free) Maize flour | Extrudate (prototype healthy food) | Co-rotating twin screw extruder Moisture: 21.4%, 25.8% Temperature: 120, 150 °C Screw speed: 250, 450 rpm Flow rate: 2.3, 6.8 kg/h Die: 3 mm cylindrical | In vitro protein digestibility negatively associated with sorghum level and positively with final barrel zone temperature (factorial analysis) | Licata et al. (2014) |
(Continued)
Formulation . | Product . | Extrusion conditions . | Effect of extrusion . | Reference . |
---|---|---|---|---|
Sorghum grits black gram grits green gram grits lentil grits pea grits | High energy dense extrudate | Heavy duty low cost collett extruder Moisture: 14% Temperature:? Screw speed: 500 rpm Flow rate: 25 kg/h | Incorporation of legumes resulted in slight increase in protein content | Balasubramanian et al. (2012) |
Sorghum grits Roasted groundnut grits Dehulled cowpea | RTE puffed snack | Single screw extruder Moisture: 12.0%–44.4% Temperature: 165 °C Screw speed: 29 rpm Die: 101 mm | Groundnut and cowpea levels positively associated with protein content (RSM modelling) | Asare et al. (2010a) |
Other millets and their composites | ||||
Pearl millet | Instant beverage powder | Counter rotating twin screw extruder | Heat alters and breaks down high molecular weight proteins and destroys heat labile protease inhibitors, which enhances in vitro protein digestibility Proteins undergo structural unfolding or aggregation when subjected to shear Partial hydrolysis of proteins produces more open configurations and increases the number of exopeptidase-susceptible sites | Obilana et al. (2018) |
Proso millet | Extruded flour | Corotating conical twin screw extruder Screw speed: 210 rpm Moisture content: 17% | Extrusion resulted in an intense decrease in the digestibility of proteins Limited formation or hydrolysis of disulphide linkages during heating or high shear | Gulati et al. (2017) |
Pearl millet Cowpea | Fura extrudates | Single screw extruder Moisture content: 20%, 25%, and 30% Screw speed: 150, 200, and 250 rpm | Decrease in the amount of protein content was observed in the extrudate with increase in the feed moisture and screw speed | Filli et al. (2012) |
Formulation . | Product . | Extrusion conditions . | Effect of extrusion . | Reference . |
---|---|---|---|---|
Sorghum grits black gram grits green gram grits lentil grits pea grits | High energy dense extrudate | Heavy duty low cost collett extruder Moisture: 14% Temperature:? Screw speed: 500 rpm Flow rate: 25 kg/h | Incorporation of legumes resulted in slight increase in protein content | Balasubramanian et al. (2012) |
Sorghum grits Roasted groundnut grits Dehulled cowpea | RTE puffed snack | Single screw extruder Moisture: 12.0%–44.4% Temperature: 165 °C Screw speed: 29 rpm Die: 101 mm | Groundnut and cowpea levels positively associated with protein content (RSM modelling) | Asare et al. (2010a) |
Other millets and their composites | ||||
Pearl millet | Instant beverage powder | Counter rotating twin screw extruder | Heat alters and breaks down high molecular weight proteins and destroys heat labile protease inhibitors, which enhances in vitro protein digestibility Proteins undergo structural unfolding or aggregation when subjected to shear Partial hydrolysis of proteins produces more open configurations and increases the number of exopeptidase-susceptible sites | Obilana et al. (2018) |
Proso millet | Extruded flour | Corotating conical twin screw extruder Screw speed: 210 rpm Moisture content: 17% | Extrusion resulted in an intense decrease in the digestibility of proteins Limited formation or hydrolysis of disulphide linkages during heating or high shear | Gulati et al. (2017) |
Pearl millet Cowpea | Fura extrudates | Single screw extruder Moisture content: 20%, 25%, and 30% Screw speed: 150, 200, and 250 rpm | Decrease in the amount of protein content was observed in the extrudate with increase in the feed moisture and screw speed | Filli et al. (2012) |
Note. IVPD = in vitro protein digestibility; RSM = response surface methodology; RTE = ready to eat.
Formulation . | Product . | Extrusion conditions . | Effect of extrusion . | Reference . |
---|---|---|---|---|
Sorghum grits black gram grits green gram grits lentil grits pea grits | High energy dense extrudate | Heavy duty low cost collett extruder Moisture: 14% Temperature:? Screw speed: 500 rpm Flow rate: 25 kg/h | Incorporation of legumes resulted in slight increase in protein content | Balasubramanian et al. (2012) |
Sorghum grits Roasted groundnut grits Dehulled cowpea | RTE puffed snack | Single screw extruder Moisture: 12.0%–44.4% Temperature: 165 °C Screw speed: 29 rpm Die: 101 mm | Groundnut and cowpea levels positively associated with protein content (RSM modelling) | Asare et al. (2010a) |
Other millets and their composites | ||||
Pearl millet | Instant beverage powder | Counter rotating twin screw extruder | Heat alters and breaks down high molecular weight proteins and destroys heat labile protease inhibitors, which enhances in vitro protein digestibility Proteins undergo structural unfolding or aggregation when subjected to shear Partial hydrolysis of proteins produces more open configurations and increases the number of exopeptidase-susceptible sites | Obilana et al. (2018) |
Proso millet | Extruded flour | Corotating conical twin screw extruder Screw speed: 210 rpm Moisture content: 17% | Extrusion resulted in an intense decrease in the digestibility of proteins Limited formation or hydrolysis of disulphide linkages during heating or high shear | Gulati et al. (2017) |
Pearl millet Cowpea | Fura extrudates | Single screw extruder Moisture content: 20%, 25%, and 30% Screw speed: 150, 200, and 250 rpm | Decrease in the amount of protein content was observed in the extrudate with increase in the feed moisture and screw speed | Filli et al. (2012) |
Formulation . | Product . | Extrusion conditions . | Effect of extrusion . | Reference . |
---|---|---|---|---|
Sorghum grits black gram grits green gram grits lentil grits pea grits | High energy dense extrudate | Heavy duty low cost collett extruder Moisture: 14% Temperature:? Screw speed: 500 rpm Flow rate: 25 kg/h | Incorporation of legumes resulted in slight increase in protein content | Balasubramanian et al. (2012) |
Sorghum grits Roasted groundnut grits Dehulled cowpea | RTE puffed snack | Single screw extruder Moisture: 12.0%–44.4% Temperature: 165 °C Screw speed: 29 rpm Die: 101 mm | Groundnut and cowpea levels positively associated with protein content (RSM modelling) | Asare et al. (2010a) |
Other millets and their composites | ||||
Pearl millet | Instant beverage powder | Counter rotating twin screw extruder | Heat alters and breaks down high molecular weight proteins and destroys heat labile protease inhibitors, which enhances in vitro protein digestibility Proteins undergo structural unfolding or aggregation when subjected to shear Partial hydrolysis of proteins produces more open configurations and increases the number of exopeptidase-susceptible sites | Obilana et al. (2018) |
Proso millet | Extruded flour | Corotating conical twin screw extruder Screw speed: 210 rpm Moisture content: 17% | Extrusion resulted in an intense decrease in the digestibility of proteins Limited formation or hydrolysis of disulphide linkages during heating or high shear | Gulati et al. (2017) |
Pearl millet Cowpea | Fura extrudates | Single screw extruder Moisture content: 20%, 25%, and 30% Screw speed: 150, 200, and 250 rpm | Decrease in the amount of protein content was observed in the extrudate with increase in the feed moisture and screw speed | Filli et al. (2012) |
Note. IVPD = in vitro protein digestibility; RSM = response surface methodology; RTE = ready to eat.
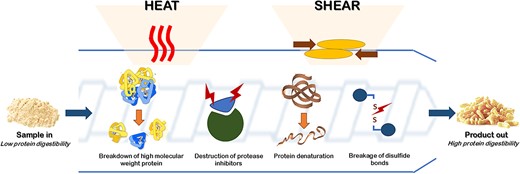
Schematic representation showing the effect of extrusion on protein structure and digestibility in sorghum and millets.
In addition to digestibility, the nutritional quality of protein is also determined by the presence of essential amino acids, with lysine frequently being the most deficient amino acid in cereal-based foods. A recent investigation by Llopart et al. (2014) evaluated the effects of wholegrain red sorghum flour extrusion processing on lysine availability and in vitro protein digestibility. They reported a 25.3% decrease in available lysine and a 31% enhancement in in vitro protein digestibility. Malleshi et al. (1996) reported similar results in their research on the physical and nutritional attributes of weaning foods derived from pearl and finger millet. Importantly, increasing the screw speed during extrusion has been shown to improve lysine retention due to the reduced residence time of the feed material. Licata et al. (2014) investigated the influence of extrusion processing parameters on protein digestibility of sorghum–maize composite flour. Their results indicated that protein digestibility was inversely related to the sorghum content in the dry mix, and positively related to the final barrel zone temperature, while it was not significantly affected by moisture content, feed input rate, or screw speed. Upon evaluating the effect of extrusion processing on protein characteristics of foxtail, finger, and pearl millet, it was seen that extrusion caused cross-linking of protein molecules through disulphide bonds (Yang et al., 2022). High MW subunit proteins were found to polymerise into larger molecules through covalent bonding, which caused a decrease in protein solubility. The rise in temperature can diminish the viscosity of the system, leading to a reduction in shear force and the extent of denaturation. Overall, it can be inferred that shear force is the primary determinant influencing the denaturation extent of proteins soluble in water, salt, and alcohol.
In contrast, the thermal effect is the major factor in alkali-soluble protein. The denaturation of protein can help improve protein digestibility, as Coulibaly et al. (2012) reported upon developing composite blends of foxtail and soybean flour. This can be attributed to the opening up of protein structure upon denaturation, which increases the accessibility of proteolytic enzymes. Significant reduction of antinutritional factors such as tannins, phytic acids, trypsin inhibitors, hemagglutinins, etc. as a result of extrusion, is reported to increase the protein digestibility in sorghum and millet-containing foods. One such example is the study by Onyango et al. (2005), where the digestibility and antinutrient properties of acidified and extruded maize–finger millet blend were studied. They observed a decrease in tannin content and an increase in in vitro protein digestibility following the process. However, the overall amino acid balance was poorer, with a reduction of histidine, lysine, and arginine contents.
Extrusion has been employed to produce food-compatible sorghum protein concentrates, as evidenced by the research conducted by de Mesa Stonestreet et al. (2012). This investigation utilised decorticated white sorghum (average particle size 119 μm) in conjunction with a thermostable α-amylase to establish an extrusion liquefaction methodology, implementing response surface methodology (RSM) to optimise moisture levels within the barrel and enzyme concentrations both during and subsequent to the extrusion process. The primary objective was to enhance both protein concentration and in vitro protein digestibility. The statistical models derived from RSM indicated that a protein concentrate exhibiting a protein content of 75 g/100 g (dry basis) and 65% in vitro digestibility could be attained with 32%-barrel moisture, the absence of enzyme during extrusion, and 2.5% enzyme application post extrusion. The resultant protein concentration exceeded that produced through traditional batch processes; however, the digestibility of the concentrate was found to be inferior to that of raw sorghum flour, a result that contradicts previous research indicating improved protein digestibility associated with low-moisture, high-temperature extrusion of sorghum (Hamaker et al., 1994; Maclean et al., 1983; Mertz et al., 1984).
Pelembe et al. (2002) reported the production of instant porridge from extruded dehulled red sorghum and cowpea flours. As cowpea content increased from 0% to 100%, protein and nitrogen solubility index also rose at both extrusion temperatures (130 and 165 °C) due to cowpea’s higher protein content and greater water solubility than sorghum protein. Asare et al. (2010b) analysed sorghum–cowpea–groundnut composite snacks using RSM. Predictably, increasing cowpea and/or groundnut raised protein content. The study recommended low moisture levels (10%–14%), cowpea level at 18%–20%, and groundnut level at 6%–8% for an optimal nutritious product. Similarly, Balasubramanian et al. (2012) found that adding 15% of legumes like black gram, green gram, lentils, or peas to sorghum-based extrudates increased protein content. Owino et al. (2014) developed a low-cost, locally sourced soybean–maize–sorghum therapeutic food using extrusion cooking, and Devi et al. (2013) created a protein-enriched sorghum snack by adding soy flour, legume flour, and whey protein. However, none of these studies assessed the protein nutritional quality of the products. Mosha et al. (2005) evaluated a sorghum-red kidney bean-sardine extruded food for its protein quality and amino acid profile, comparing it with foods processed by drum drying and conventional cooking. The product showed higher digestibility and protein digestibility-corrected amino acid score than foods produced using other methods, although there was no significant difference in amino acid levels across processing methods. With millets getting a lot of attention lately, a study on the co-extrusion of pearl–millet–whey protein concentrate was attempted to develop expanded snacks. Still, the protein characteristics were not explored (Yadav et al., 2014). More recently, extruded snacks were developed from whole pearl millet-based flour, and an increasing blending ratio of African walnut flour in the snack resulted in the highest crude protein content (Sobowale et al., 2021). Similarly, Akinyemi et al. (2022) attempted to develop extruded breakfast cereal from finger millet flour blends and an in vivo protein quality assessment was performed. A reduction in tannins, phytate, and saponin contents was observed, directly increasing protein digestibility and mineral bioavailability.
In summary, the majority of research indicates that low-moisture, high-temperature extrusion enhances the digestibility of sorghum and millet. However, if these extruded products are rehydrated and then subjected to further cooking, their digestibility may decrease once again. This is an important factor to consider for individuals with protein malnutrition who rely on extruded grains in their diets. Additionally, sorghum and millets lack sufficient levels of the essential amino acid lysine, and extrusion can further reduce lysine content. Therefore, more research is needed to (a) optimise the formulation and processing of millet-based extruded foods, potentially using RSM to minimise lysine loss while improving protein digestibility; and (b) develop affordable and palatable formulations using composite flours to enhance the overall protein quality of these products.
Effect of extrusion on starch digestibility
The rate and degree of starch digestibility in foods have significant implications for nutritional quality and its potential to influence biomarkers of chronic disease risk. Predicting and controlling glucose absorption induced by the consumption of starchy foods are of great interest in global health issues. Slowly digestible starch (SDS), rapidly digestible starch (RDS), and resistant starch (RS) are the three physiologically important starch fractions (Englyst et al., 1992). These starch fractions can be analysed in vitro using tests that mimic human digestion (Sopade & Gidley, 2009). The foods with low RDS or SDS values in vitro will have a low glycemic index (GI) in vivo (Englyst et al., 1999; Englyst et al., 2003). Low GI diets can reduce the risk of diabetes (type 2) by lowering blood sugar and insulin levels (Buyken et al., 2010). Depending on their age and overall dietary intake, foods containing varying levels of SDS, RDS, and RS are required to maintain consumers’ optimal nutrition and health requirements.
On the one hand, foods with a low RS and high RDS content are ideal for quickly obtaining high energy from starchy foods. This is very important in developing countries where malnutrition is still prevalent, particularly during weaning (Shamim et al., 2006), due to the high prices of commercially available weaning products for the target groups. Over nutrition and obesity are now in danger due to rapid urbanisation and changing diets in the developing world (Friel et al., 2007; Popkin, 2011). Therefore, food products with low RDS and/or high SDS with some RS may benefit consumers when overall food consumption is adequate or excessive or when carbohydrate metabolism is disrupted towards a diabetic condition.
Food composition and processing methods significantly impact the amount of physiologically significant starch fractions present. Extrusion cooking, when combined with high temperature and pressure in a hydrated matrix, can substantially improve the digestibility of starchy raw materials by enhancing starch gelatinisation. Table 1 summarises research on the effect of extrusion on in vitro starch digestibility of millet and millet-based products. There has been limited research on the impact of process parameters on the digestibility of extruded sorghum and millet starch. The major changes occurring during the extrusion of millet starch are depicted in Figure 2, and Table 2 summarises some major studies. Most of these studies have concentrated on product formulation and identifying processing conditions that improve sorghum and other millets’ digestibility and energy availability. In extruded tannin-rich sorghum, Dahlin and Lorenz (1993) observed that increasing the process temperature increased digestibility the most. The combination of lower moisture (15%) and temperature (80/100 °C) resulted in lower digestibility.
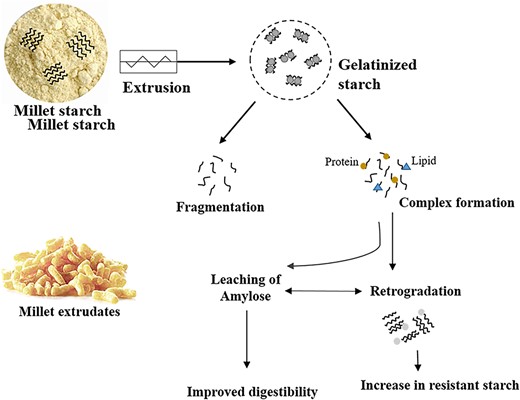
Major changes occurring in the starch during the extrusion of sorghum and millets.
Effect of extrusion on in vitro starch digestibility of sorghum- and millet-based products.
Formulation . | Product . | Extrusion conditions . | Effect of extrusion . | Reference . |
---|---|---|---|---|
Sorghum | ||||
Wholegrain red sorghum flour | Expanded extrudate | Co-rotating intermeshing extruder Moisture: 25%, 30%, 40% Ten temperature zones Screw speed: 150, 220, and 300 rpm Flow rate: 2 kg/h Die: 2 × 2 mm cylindrical | Extrusion increased rate of digestion of starch ~10-fold | Mahasukhonthachat et al. (2010a) |
Sorghum-containing composites | ||||
Wholegrain red sorghum flour (tannin-free) Maize flour | Extrudate (prototype healthy food) | Co-rotating twin screw extruder Moisture: 21.4%, 25.8% Temperature: 120 and 150 °C Screw speed: 250, 450 rpm Flow rate: 2.3, 6.8 kg/h Die: 3 mm cylindrical | Slowly digested starch level is positively associated with sorghum level and negatively with final barrel zone temperature (factorial analysis) | Licata et al. (2014) |
Other millets and their composites | ||||
Pearl millet flour | Extruded flour | Single screw extruder Moisture: 30%–32% Temperature: 120 °C Speed: 700 rpm L/D ratio for extruder: 7.51 Die: 6 mm | Decrease in digestibility due to the formation of amylose–lipid complexes | Onyeoziri et al. (2021) |
Foxtail millet flour | Milled extrudate incorporated into bread and pancake | Twin-screw extruder Moisture: 16% (wb) Temperature: 60, 90, 120, and 175 °C Screw speed: 30 rpm | Higher in vitro starch digestibility Higher moisture content also increased digestibility | Ren et al. (2016) |
Foxtail millet, broken rice, barnyard millet, and quinoa | Extruded rice | Co-rotating twin-screw extruder Moisture: 30% (wb) Temperature: 120 °C Screw speed: 30 rpm | Lower GI and starch digestibility due to starch-lipid and starch–protein complexes formation | Yadav et al. (2022) |
Quinoa, finger millet, red rice | Breakfast cereal | Twin screw extruder Moisture: 10–20 g/100 g Temperature: 120–130 °C Screw speed: 320–350 rpm Die: 4.95 mm | Starch gelatinisation increased digestibility | Sukumar et al. (2022) |
Formulation . | Product . | Extrusion conditions . | Effect of extrusion . | Reference . |
---|---|---|---|---|
Sorghum | ||||
Wholegrain red sorghum flour | Expanded extrudate | Co-rotating intermeshing extruder Moisture: 25%, 30%, 40% Ten temperature zones Screw speed: 150, 220, and 300 rpm Flow rate: 2 kg/h Die: 2 × 2 mm cylindrical | Extrusion increased rate of digestion of starch ~10-fold | Mahasukhonthachat et al. (2010a) |
Sorghum-containing composites | ||||
Wholegrain red sorghum flour (tannin-free) Maize flour | Extrudate (prototype healthy food) | Co-rotating twin screw extruder Moisture: 21.4%, 25.8% Temperature: 120 and 150 °C Screw speed: 250, 450 rpm Flow rate: 2.3, 6.8 kg/h Die: 3 mm cylindrical | Slowly digested starch level is positively associated with sorghum level and negatively with final barrel zone temperature (factorial analysis) | Licata et al. (2014) |
Other millets and their composites | ||||
Pearl millet flour | Extruded flour | Single screw extruder Moisture: 30%–32% Temperature: 120 °C Speed: 700 rpm L/D ratio for extruder: 7.51 Die: 6 mm | Decrease in digestibility due to the formation of amylose–lipid complexes | Onyeoziri et al. (2021) |
Foxtail millet flour | Milled extrudate incorporated into bread and pancake | Twin-screw extruder Moisture: 16% (wb) Temperature: 60, 90, 120, and 175 °C Screw speed: 30 rpm | Higher in vitro starch digestibility Higher moisture content also increased digestibility | Ren et al. (2016) |
Foxtail millet, broken rice, barnyard millet, and quinoa | Extruded rice | Co-rotating twin-screw extruder Moisture: 30% (wb) Temperature: 120 °C Screw speed: 30 rpm | Lower GI and starch digestibility due to starch-lipid and starch–protein complexes formation | Yadav et al. (2022) |
Quinoa, finger millet, red rice | Breakfast cereal | Twin screw extruder Moisture: 10–20 g/100 g Temperature: 120–130 °C Screw speed: 320–350 rpm Die: 4.95 mm | Starch gelatinisation increased digestibility | Sukumar et al. (2022) |
Note. GI = glycemic index.
Effect of extrusion on in vitro starch digestibility of sorghum- and millet-based products.
Formulation . | Product . | Extrusion conditions . | Effect of extrusion . | Reference . |
---|---|---|---|---|
Sorghum | ||||
Wholegrain red sorghum flour | Expanded extrudate | Co-rotating intermeshing extruder Moisture: 25%, 30%, 40% Ten temperature zones Screw speed: 150, 220, and 300 rpm Flow rate: 2 kg/h Die: 2 × 2 mm cylindrical | Extrusion increased rate of digestion of starch ~10-fold | Mahasukhonthachat et al. (2010a) |
Sorghum-containing composites | ||||
Wholegrain red sorghum flour (tannin-free) Maize flour | Extrudate (prototype healthy food) | Co-rotating twin screw extruder Moisture: 21.4%, 25.8% Temperature: 120 and 150 °C Screw speed: 250, 450 rpm Flow rate: 2.3, 6.8 kg/h Die: 3 mm cylindrical | Slowly digested starch level is positively associated with sorghum level and negatively with final barrel zone temperature (factorial analysis) | Licata et al. (2014) |
Other millets and their composites | ||||
Pearl millet flour | Extruded flour | Single screw extruder Moisture: 30%–32% Temperature: 120 °C Speed: 700 rpm L/D ratio for extruder: 7.51 Die: 6 mm | Decrease in digestibility due to the formation of amylose–lipid complexes | Onyeoziri et al. (2021) |
Foxtail millet flour | Milled extrudate incorporated into bread and pancake | Twin-screw extruder Moisture: 16% (wb) Temperature: 60, 90, 120, and 175 °C Screw speed: 30 rpm | Higher in vitro starch digestibility Higher moisture content also increased digestibility | Ren et al. (2016) |
Foxtail millet, broken rice, barnyard millet, and quinoa | Extruded rice | Co-rotating twin-screw extruder Moisture: 30% (wb) Temperature: 120 °C Screw speed: 30 rpm | Lower GI and starch digestibility due to starch-lipid and starch–protein complexes formation | Yadav et al. (2022) |
Quinoa, finger millet, red rice | Breakfast cereal | Twin screw extruder Moisture: 10–20 g/100 g Temperature: 120–130 °C Screw speed: 320–350 rpm Die: 4.95 mm | Starch gelatinisation increased digestibility | Sukumar et al. (2022) |
Formulation . | Product . | Extrusion conditions . | Effect of extrusion . | Reference . |
---|---|---|---|---|
Sorghum | ||||
Wholegrain red sorghum flour | Expanded extrudate | Co-rotating intermeshing extruder Moisture: 25%, 30%, 40% Ten temperature zones Screw speed: 150, 220, and 300 rpm Flow rate: 2 kg/h Die: 2 × 2 mm cylindrical | Extrusion increased rate of digestion of starch ~10-fold | Mahasukhonthachat et al. (2010a) |
Sorghum-containing composites | ||||
Wholegrain red sorghum flour (tannin-free) Maize flour | Extrudate (prototype healthy food) | Co-rotating twin screw extruder Moisture: 21.4%, 25.8% Temperature: 120 and 150 °C Screw speed: 250, 450 rpm Flow rate: 2.3, 6.8 kg/h Die: 3 mm cylindrical | Slowly digested starch level is positively associated with sorghum level and negatively with final barrel zone temperature (factorial analysis) | Licata et al. (2014) |
Other millets and their composites | ||||
Pearl millet flour | Extruded flour | Single screw extruder Moisture: 30%–32% Temperature: 120 °C Speed: 700 rpm L/D ratio for extruder: 7.51 Die: 6 mm | Decrease in digestibility due to the formation of amylose–lipid complexes | Onyeoziri et al. (2021) |
Foxtail millet flour | Milled extrudate incorporated into bread and pancake | Twin-screw extruder Moisture: 16% (wb) Temperature: 60, 90, 120, and 175 °C Screw speed: 30 rpm | Higher in vitro starch digestibility Higher moisture content also increased digestibility | Ren et al. (2016) |
Foxtail millet, broken rice, barnyard millet, and quinoa | Extruded rice | Co-rotating twin-screw extruder Moisture: 30% (wb) Temperature: 120 °C Screw speed: 30 rpm | Lower GI and starch digestibility due to starch-lipid and starch–protein complexes formation | Yadav et al. (2022) |
Quinoa, finger millet, red rice | Breakfast cereal | Twin screw extruder Moisture: 10–20 g/100 g Temperature: 120–130 °C Screw speed: 320–350 rpm Die: 4.95 mm | Starch gelatinisation increased digestibility | Sukumar et al. (2022) |
Note. GI = glycemic index.
Gomez et al. (1988) investigated the impact of moisture content during the extrusion of decorticated sorghum flours with different AM contents. The starch digestibility was estimated using an enzyme-sensitive starch assay. The study reported increased enzyme-sensitive starch values after the extrusion process for all sorghum samples. Reduced digestibility was observed in high moisture samples, possibly due to moisture-induced protein crosslinking in sorghum. The starch digestion kinetics of sorghum flour during extrusion was investigated by Mahasukhonthachat et al. (2010a). The extrusion process was carried out at different moisture contents (25%,30%, and 40%) and screw rotational speeds (150, 220, and 300 rpm). They observed that the rate of starch digestion was not influenced by screw speed. However, increasing humidity above 30% decreased the starch digestion rate, and specific mechanical energy (SME) was related to the digestion rate. In order to maximise the SME of the extrusion process through better starch digestibility, extrusion at 30% moisture content and screw speed of 250 rpm was suggested; on the contrary, 30% moisture content and low screw speed (150 rpm) have been recommended to minimise starch digestibility (Mahasukhonthachat et al., 2010a). These considerations are essential for populations where sorghum is a diet staple, particularly those in developing countries.
Onyango et al. (2005) investigated the starch digestibility and antinutritional composition of extruded maize–finger millet composite and noticed that the starch content was significantly reduced after the extrusion process. Irreversible complex formation between AM and monoglycerides/free fatty acids and AM and proteins is responsible for decreased starch content (Camire, 2011; Tester et al., 2004). In addition, Onyango et al. (2005) revealed that the in vitro starch digestibility of the raw blend increased after extrusion (from 20 to 162–246 mg/g). Extrusion increases starch digestibility by deforming the AP branches, making them more accessible to amylolytic enzymes (Camire, 2011). Increasing starch digestibility and maximising available energy could help decrease malnutrition in vulnerable groups. In contrast, slow starch digestibility and lower available energy may be more pertinent for populations where obesity-related chronic diseases such as diabetes, metabolic disorders, coronary heart diseases, and cancer are major health concerns due to changes in diet and rapid urbanisation in both high- and low-income countries.
The impact of extrusion on metrics related to the starch digestibility of sorghum-legume composite flours has only been reported in a few research studies. According to Pelembe et al. (2002), higher extrusion temperature increased enzyme-susceptible starch content in instant sorghum and cowpea mix porridge. They also observed that increasing the amount of cowpea in the extrudate decreased the enzyme-susceptible starch content. According to Malleshi et al. (1996), extrusion cooking altered the in vitro starch digestibility of the raw mix compared with the finished product when preparing a weaning food comprising sorghum (60%), mung bean (30%), and skimmed milk powder (10%). The starch digestibility of sorghum foods after extrusion cooking is lower than that of other cereal grains subjected to high moisture heat treatments due to forming a matrix of cross-linked starch, protein, and polyphenols (mostly tannins). This reduces starch hydration and gelatinisation and can be a physical barrier to digestive enzyme action (Taylor & Emmambux, 2010).
In a study conducted on two cultivars of milled sorghum grains, Wong et al. (2009) found that the cultivar with higher cross-linked protein content and lower protein digestibility also had reduced starch digestibility. This could be attributed to differences in the structure of the protein–starch matrix. Removing protein from flour and cooking with a reducing agent has been shown to improve starch digestibility (Zhang & Hamaker, 1998). Ezeogu et al. (2005) suggested that reduced starch digestibility in cooked sorghum products is due to increased protein cross-linking and the formation of high-molecular-weight protein complexes. In millet-based products, decortication has been found to influence starch digestibility by reducing dietary fibre and antinutritional factors, which may enhance nutrient digestion in some sorghum cultivars (Rooney & Serna-Saldivar, 2000). However, starch digestibility in extruded sorghum products remains understudied. The ratio of AP to AM impacts the glass transition temperature (Tg) of sorghum starch, which in turn affects the digestibility of starch in extrudates (Taylor & Emmambux, 2010). Gomez et al. (1988) observed that sorghum samples with lower AM content had higher levels of enzymatically soluble starch compared with those with higher AM. In vitro starch digestibility of sorghum extrudates may also be influenced by particle size, though this has not been examined explicitly in millet extrudates. Mahasukhonthachat et al. (2010b) noted a negative correlation between particle size and in vitro starch digestibility in sorghum flour.
Sorghum flour in composite flour foods affects in vitro starch digestion. These foods include sorghum–wheat flour flatbread (Yousif et al., 2012), sorghum–semolina pasta (Licata, 2012; Licata et al., 2014), and sorghum–maize snack-like extrudates (Khan et al., 2013). With the delivery of SDS and RS, which may lower determinant factors of chronic disease risk associated with overnutrition or improve blood glucose control, these studies have identified formulations and methods that produce sorghum foods with desirable quality characteristics and consumer acceptance. Licata et al. (2014) observed that the SDS level of the extrudate made from composite flour of red sorghum and maize was directly correlated with the amount of sorghum and negatively related to barrel temperature. These results show that maximising sorghum addition and minimising extrusion temperature maximise SDS in exudates while maintaining textural quality (expansion ratio and instrumental harness) within consumer acceptability limits. Licata (2012) used RSM to optimise the process formulation for a red sorghum–maize composite extruded snack with the highest in vitro SDS and expansion ratio. This study resulted in an extrudate with an expansion ratio of 2.2 and an SDS level of 22 g/100 g dry starch using 75% composite flour, 22% extruder feed moisture, and 115 °C barrel zone temperature. The influence of varietal differences and the relationship between major compositional elements on the in vitro starch digestibility of extruded millet foods require further investigation. Using low-moisture, high-temperature extrusion cooking to produce millet-based foods with high starch digestibility is promising for increasing energy availability. Due to their elevated risk of chronic health conditions like type 2 diabetes, these consumers are advised to consume foods with higher SDS and RS. Further research is necessary to optimise the process technologies to deliver palatable extruded millet foods with slow starch digestibility and adequate nutrient requirements.
Effect of extrusion on nutritional quality and health
The changes induced by extrusion cooking on nutritional quality and GI are of great interest. Gelatinisation of starch, reduction of lipid oxidation, increase in soluble dietary fibre, and the destruction of antinutritional factors are potential benefits of this process. Maillard reactions between amino groups and sugars, on the other hand, reduce protein nutritional value depending on the type of raw material, composition, and processing conditions. Heat-labile vitamins may lose some of their potency. Changes in protein, amino acid profiles, carbohydrates, vitamins, minerals, dietary fibre, and some nonnutritional healthful food components can all be beneficial or detrimental. Onyango et al. (2005) noticed a substantial reduction in the lipid fraction of an extruded uji made from finger millet and maize. The formation of protein–lipid and amylose–lipid complexes, which resist fat extraction with organic solvents, is attributed to the lower fat content during extrusion. Controlling the temperature during the extrusion is essential for minimising lipid loss, as higher temperatures encourage the oxidation of unsaturated fatty acids (USFA), forming hydroperoxides and lowering the final product’s lipid concentration. Ushakumari et al. (2004) observed a significant increase in USFA in foxtail millet extruded products. This could be due to the release of lipids from cells caused by high temperatures and shear forces (Singh et al., 2007). Nondigestible carbohydrate fractions were reduced from 2.5% to 1.5% in raw finger millet–maize composite extruded products (Onyango et al., 2005). Insoluble dietary fibre may be transformed into soluble dietary fibre during extrusion, reducing the percentage of total nondigestible carbohydrates (Dalbhagat et al., 2019).
The addition of sorghum to foods slows starch digestion and reduces the negative relationship between SDS levels and the in vivo glycemic response of food. Extruded foods made from sorghum have been shown to have a GI that is lower than that of foods made from rice, wheat, and maize. Additionally, extruded snack foods that are ready to eat (RTE) have a high GI. These foods contain low dietary fibre and highly gelatinised starch (Brennan et al., 2013). Therefore, any strategy that can lower the GI of these foods while keeping the same level of consumer acceptability will benefit consumers’ health. Food consumption patterns in urban Africa have changed over the past decades, shifting towards more Western-orientated diets, with the consequent increase in obesity rates and related chronic disorders. Ronquest-Ross et al. (2015) reported that in South Africa, there was a 33% increase in the consumption of extruded snacks between 1999 and 2012. However, the nutritional value of these snack foods was not reported. Sorghum-based extruded products with low GI could be relevant to public health issues in urban areas of the developing world to combat the prevalence of chronic diseases.
However, there is a paucity of published works describing the glycemic response or GI of extruded millet foods. Traditional Indian foods such as upma (thick porridge), missi roti (unleavened flatbread), and dhokla (fermented batter) made with wholegrain sorghum had a lower GI than those made with wheat or rice, according to an in vivo glycemic study in humans with type 2 diabetes. Roasted bread, Sudanese kisra (flat bread), and acida (porridge), foods made from sorghum, had the same glycemic effect (Abdelgadir et al., 2005). Poquette et al. (2014) published a study comparing SDS, RS, and postprandial glycemic response levels in nondiabetic men who consumed muffins made with wholegrain wheat flour and wholegrain sorghum flour. The sorghum muffin had significantly lower RDS levels, higher SDS and RS levels, and significantly lower glycemic and insulinemic responses than the wheat muffin. This result suggests that sorghum muffins may assist in maintaining normal blood sugar levels. Assessing the GI of extruded sorghum-based foods containing SDS requires clinical studies with an appropriate design. These scientific data are essential for deciding whether these extruded sorghum foods can be recommended as part of a diet to reduce the risk and improve type 2 diabetes management.
Conclusion
Extrusion cooking presents promising opportunities for creating millet-based products that improve starch digestibility and boost energy availability, potentially contributing to mitigating malnutrition in at-risk populations. On the other hand, the process can also be adjusted to decrease starch digestibility and reduce energy availability, which may be significant for groups having obesity-related chronic diseases, metabolic disorders, coronary artery diseases, and cancer. Therefore, a comprehensive examination of the extrusion processing of sorghum and millets is essential to meet the diverse needs of different consumer segments. As millets are deficient in the essential amino acid lysine, extrusion cooking can further lower its levels. Therefore, research must be strengthened to refine the processing of millet-based extruded foods to minimise lysine losses and develop cost-effective formulations that enhance protein digestibility, palatability, and consumer acceptance.
Data availability
Data sharing is not applicable to this article as no new data were created or analysed in this review.
Author contributions
Rewati Raman Bhattarai (Conceptualisation [lead], Supervision [lead], Writing—original draft [supporting]), T. Jayasree Joshi (Conceptualisation [supporting], Investigation [supporting], Writing—original draft [lead]), N.U. Sruthi (Conceptualisation [supporting], Investigation [supporting], Writing—original draft [lead]), Pavuluri Srinivasa Rao (Supervision [equal], Writing—review & editing [equal]), and Stuart Johnson (Supervision [equal], Writing—review & editing [equal])
Funding
Not applicable.
Conflicts of interest
The authors declare that there are no conflicts of interest.
Acknowledgements
The authors acknowledge Indian Institute of Technology Kharagpur, India and Curtin University, Australia for providing the oppurtunity for writing this article.
References
Asare, A. T., Gowda, B. S., Galyuon, I. K., Aboagye, L. L., Takrama, J. F., & Timko, M. P. (
Asare, E. K., Sefa-Dedeh, S., Afoakwa, E. O., Sakyi-Dawson, E., & Budu, A. S. (
Balasubramanian, S., Borah, A., Singh, K. K. (
Belton, P. S., Delgadillo, I., Halford, N. G., & Shewry, P. R. (
Belton, P. S., & Taylor, J. R. (
de Mesa Stonestreet, N. J. (
de Mesa-Stonestreet, N. J., Alavi, S., & Gwirtz, J. (
Devi, N. L., Shobha, S., Tang, X., Shaur, S. A., Dogan, H., & Alavi, S. (
El Nour, I. N. A., Peruffo, A. D., & Curioni, A. (
Hamaker, B. R., Mertz, E. T., & Axtell, J. D. (
Llopart, E. E., Drago, S. R., De Greef, D. M., Torres, R. L., & González, R. J. (
MacLean Jr, W. C., de Romaña, G. L., Gastañaduy, A., & Graham, G. G. (
Mertz, E. T., Hassen, M. M., Cairns-Whittern, C., Kirleis, A. W., Tu, L., & Axtell, J. D. (
Mosha, T. C., Bennink, M. R., & Ng, P. K. (
Oria, M. P., Hamaker, B. R., & Schull, J. M. (
Owino, Victor O. (
Shull, J. M., Watterson, J. J., & Kirleis, A. W. (