-
PDF
- Split View
-
Views
-
Cite
Cite
Sanjaya Karki, Susma Bhattarai, Ranjit Singh, Anil Kumar Anal, Protein extraction technologies for foxtail, pearl, and proso millets along with their structural and techno-functional properties: a review, International Journal of Food Science and Technology, Volume 60, Issue 1, January 2025, vvae045, https://doi.org/10.1093/ijfood/vvae045
- Share Icon Share
Abstract
As the world’s population grows, so does the demand for protein, and the growing population is actively seeking alternative protein sources that are affordable and available locally. Millet, a resilient crop with a remarkable ability to adapt to unfavourable conditions, has emerged as a potential alternative in recent years. Millet contains protein levels ranging from 7% to 21%, depending on the variety, growing condition, maturity of seeds, and species. This review looks closely into the current protein extraction technologies used on three popular millets: pearl, foxtail, and proso millet. Aside from the extraction technologies, this review provides a thorough critical analysis of structural and techno-functional properties of millet proteins, namely, solubility, emulsifying activity, water and oil holding capacity, and foaming ability, which are important attributes impacting the potential use of millet proteins in food and beverages. Thus, the insights gained from this review provide a strong foundation for future research and development on pearl, foxtail, and proso millet as an ingredient in a wide range of gluten-free food products, meat analogue formulations, and nutraceuticals.
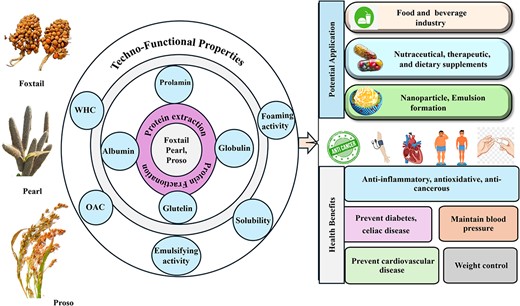
Introduction
Animal-based products contribute to approximately 57% of greenhouse gas emissions from the entire food system, raising significant sustainability concerns. Consequently, there has been a growing shift among consumers towards plant-based proteins, which offer potential health and environmental benefits (Malek & Umberger, 2023). Additionally, food supply chain disruptions due to pandemics like COVID-19, natural disasters, and wars have highlighted the importance of alternatives like millet.
Millet, a versatile and nutritious cereal grain from the Poaceae family, includes several species like foxtail millet, pearl millet, and proso millet. Cultivated for over 10,000 years, millet thrives in arid and semi-arid conditions (Jindal & Nikhanj, 2023). Millet holds cultural significance in Africa and Asia, where it is a staple ingredient in traditional dishes like porridge, bread, pasta, and fermented beverages, reflecting local populations’ cultural diversity and dietary preferences.
Asia and Africa produce 97% of the world’s millet, with India contributing about 27% of global production (Meena et al., 2021). Millets are rich in carbohydrates (56%–73%), protein (7%–21%), fat (1%–5%), and crude fibre (2%–13%) (Kumar et al., 2023). Millet proteins offer numerous health benefits including improved cardiovascular health, weight control, and better glycemic control (Jacob et al., 2024). They are also used in plant-based foods and supplements and have the potential to encapsulate lipophilic compounds like curcumin (Wang et al., 2018). Additionally, foxtail millet bran, a by-product of milling, generates 480,000 tons annually in China, presenting environmental and cost challenges. Rich in protein (8.1%–19.6%) with a balanced amino acid profile, foxtail millet bran is easily digestible, hypoallergenic (Peng et al., 2024), and suitable for protein-rich food formulations. This review explores the proteins of pearl, foxtail, and proso millet, including amino acid profiles, protein extraction methodologies, and the structural and techno-functional properties, highlighting their potential application in the food industry.
Overview of protein in pearl, foxtail, and proso millet
This overview details the protein content and fractions in three millet varieties: pearl millet (Pennisetum glaucum), foxtail millet (Setaria italica), and proso millet (Panicum miliaceum). Pearl millet, foxtail millet, and proso millet contain approximately 11.6%, 12.3%, and 11% (wt/wt) with variable fractions (albumin, globulin, prolamins, and glutelins,) varying by species and cultivar (Kaur et al., 2023). Foxtail millet, cultivated in over 26 countries and second in production to pearl millet, has a dominant prolamin fraction (60%–65%) (Suri et al., 2024a). Proso millet primarily consists of prolamins, followed by globulin, albumin, and glutelin. In contrast, glutenin is the major protein fraction in wheat (28.92%–49.97%), while albumin and globulin account for 22.92%–36.60%. Gliadin contributes the least, comprising 18.55%–34.29% of total crude protein (Siddiqi et al., 2021). Rice exhibits significant glutelin content (75%–81%), but its overall protein profile shows lower concentrations of albumin and globulin (Jayaprakash et al., 2022). This highlights millet’s unique profile compared with wheat and rice, with prolamins predominating, enhancing their potential as alternative protein sources for gluten-intolerant consumers. Supplementary Table 1 summarizes the protein fractions of pearl, proso, and foxtail millet.
The quality of cereal proteins is determined by their essential amino acid composition and in millet, particularly in flour and protein concentrate, amino acids play a key role as a biocatalyst and a nutrient carrier (Rao & Poonia ,2023). Factors such as variety, climate, harvest time, and seed maturity affect amino acid profiles. Table 1 compares the amino acid composition of millet with other cereals.
Amino acid . | Pearl milleta . | Foxtail milletb . | Proso milletc . | Wheatd . | Maized . | Riced . |
---|---|---|---|---|---|---|
Lysine | 26.67 | 24.90 | 19.00–21.30 | 31.30 | 26.40 | 37.00 |
Histidine | 17.80 | 22.80 | 12.20–16.30 | 26.50 | 27.00 | 24.50 |
Arginine | 35.18 | 63.50 | 31.80–48.30 | 51.30 | 42.00 | 77.20 |
Aspartic acid | 57.27 | 52.20 | 21.40–23.20 | 54.40 | 65.50 | 87.30 |
Threonine | 29.93 | 35.70 | 12.40–14.80 | 30.10 | 32.30 | 32.80 |
Serine | 35.70 | 56.70 | 16.30–20.30 | 48.00 | 45.80 | 49.50 |
Glutamate | – | 214.20 | 25.80–34.20 | 270.60 | 193.90 | 189.20 |
Proline | 47.19 | 72.90 | 19.80–25.00 | 102.50 | 78.80 | 43.10 |
Glycine | 24.59 | 37.30 | 21.70–27.00 | 41.90 | 32.70 | 41.80 |
Alanine | 55.63 | 87.90 | 19.50–22.70 | 36.40 | 77.30 | 55.10 |
Cystine | 5.87 | 20.20 | – | 23.50 | 15.50 | 18.40 |
Valine | 44.08 | 50.60 | 19.90–23.30 | 51.10 | 54.10 | 60.60 |
Methionine | 43.91 | 43.60 | 6.50–8.40 | 17.50 | 21.00 | 26.00 |
Isoleucine | 33.38 | 46.50 | 12.70–14.20 | 38.30 | 36.70 | 42.90 |
Leucine | 76.02 | 68.80 | 26.00–30.30 | 68.10 | 122.40 | 80.90 |
Tyrosine | 28.90 | 44.00 | 13.10–17.40 | 31.20 | 37.10 | 43.60 |
Phenylalanine | 42.80 | 59.60 | 20.60–24.90 | 47.50 | 51.40 | 53.60 |
Tryptophan | – | 20.50 | 0.10 | 14.00 | 5.70 | 12.70 |
Amino acid . | Pearl milleta . | Foxtail milletb . | Proso milletc . | Wheatd . | Maized . | Riced . |
---|---|---|---|---|---|---|
Lysine | 26.67 | 24.90 | 19.00–21.30 | 31.30 | 26.40 | 37.00 |
Histidine | 17.80 | 22.80 | 12.20–16.30 | 26.50 | 27.00 | 24.50 |
Arginine | 35.18 | 63.50 | 31.80–48.30 | 51.30 | 42.00 | 77.20 |
Aspartic acid | 57.27 | 52.20 | 21.40–23.20 | 54.40 | 65.50 | 87.30 |
Threonine | 29.93 | 35.70 | 12.40–14.80 | 30.10 | 32.30 | 32.80 |
Serine | 35.70 | 56.70 | 16.30–20.30 | 48.00 | 45.80 | 49.50 |
Glutamate | – | 214.20 | 25.80–34.20 | 270.60 | 193.90 | 189.20 |
Proline | 47.19 | 72.90 | 19.80–25.00 | 102.50 | 78.80 | 43.10 |
Glycine | 24.59 | 37.30 | 21.70–27.00 | 41.90 | 32.70 | 41.80 |
Alanine | 55.63 | 87.90 | 19.50–22.70 | 36.40 | 77.30 | 55.10 |
Cystine | 5.87 | 20.20 | – | 23.50 | 15.50 | 18.40 |
Valine | 44.08 | 50.60 | 19.90–23.30 | 51.10 | 54.10 | 60.60 |
Methionine | 43.91 | 43.60 | 6.50–8.40 | 17.50 | 21.00 | 26.00 |
Isoleucine | 33.38 | 46.50 | 12.70–14.20 | 38.30 | 36.70 | 42.90 |
Leucine | 76.02 | 68.80 | 26.00–30.30 | 68.10 | 122.40 | 80.90 |
Tyrosine | 28.90 | 44.00 | 13.10–17.40 | 31.20 | 37.10 | 43.60 |
Phenylalanine | 42.80 | 59.60 | 20.60–24.90 | 47.50 | 51.40 | 53.60 |
Tryptophan | – | 20.50 | 0.10 | 14.00 | 5.70 | 12.70 |
Amino acid . | Pearl milleta . | Foxtail milletb . | Proso milletc . | Wheatd . | Maized . | Riced . |
---|---|---|---|---|---|---|
Lysine | 26.67 | 24.90 | 19.00–21.30 | 31.30 | 26.40 | 37.00 |
Histidine | 17.80 | 22.80 | 12.20–16.30 | 26.50 | 27.00 | 24.50 |
Arginine | 35.18 | 63.50 | 31.80–48.30 | 51.30 | 42.00 | 77.20 |
Aspartic acid | 57.27 | 52.20 | 21.40–23.20 | 54.40 | 65.50 | 87.30 |
Threonine | 29.93 | 35.70 | 12.40–14.80 | 30.10 | 32.30 | 32.80 |
Serine | 35.70 | 56.70 | 16.30–20.30 | 48.00 | 45.80 | 49.50 |
Glutamate | – | 214.20 | 25.80–34.20 | 270.60 | 193.90 | 189.20 |
Proline | 47.19 | 72.90 | 19.80–25.00 | 102.50 | 78.80 | 43.10 |
Glycine | 24.59 | 37.30 | 21.70–27.00 | 41.90 | 32.70 | 41.80 |
Alanine | 55.63 | 87.90 | 19.50–22.70 | 36.40 | 77.30 | 55.10 |
Cystine | 5.87 | 20.20 | – | 23.50 | 15.50 | 18.40 |
Valine | 44.08 | 50.60 | 19.90–23.30 | 51.10 | 54.10 | 60.60 |
Methionine | 43.91 | 43.60 | 6.50–8.40 | 17.50 | 21.00 | 26.00 |
Isoleucine | 33.38 | 46.50 | 12.70–14.20 | 38.30 | 36.70 | 42.90 |
Leucine | 76.02 | 68.80 | 26.00–30.30 | 68.10 | 122.40 | 80.90 |
Tyrosine | 28.90 | 44.00 | 13.10–17.40 | 31.20 | 37.10 | 43.60 |
Phenylalanine | 42.80 | 59.60 | 20.60–24.90 | 47.50 | 51.40 | 53.60 |
Tryptophan | – | 20.50 | 0.10 | 14.00 | 5.70 | 12.70 |
Amino acid . | Pearl milleta . | Foxtail milletb . | Proso milletc . | Wheatd . | Maized . | Riced . |
---|---|---|---|---|---|---|
Lysine | 26.67 | 24.90 | 19.00–21.30 | 31.30 | 26.40 | 37.00 |
Histidine | 17.80 | 22.80 | 12.20–16.30 | 26.50 | 27.00 | 24.50 |
Arginine | 35.18 | 63.50 | 31.80–48.30 | 51.30 | 42.00 | 77.20 |
Aspartic acid | 57.27 | 52.20 | 21.40–23.20 | 54.40 | 65.50 | 87.30 |
Threonine | 29.93 | 35.70 | 12.40–14.80 | 30.10 | 32.30 | 32.80 |
Serine | 35.70 | 56.70 | 16.30–20.30 | 48.00 | 45.80 | 49.50 |
Glutamate | – | 214.20 | 25.80–34.20 | 270.60 | 193.90 | 189.20 |
Proline | 47.19 | 72.90 | 19.80–25.00 | 102.50 | 78.80 | 43.10 |
Glycine | 24.59 | 37.30 | 21.70–27.00 | 41.90 | 32.70 | 41.80 |
Alanine | 55.63 | 87.90 | 19.50–22.70 | 36.40 | 77.30 | 55.10 |
Cystine | 5.87 | 20.20 | – | 23.50 | 15.50 | 18.40 |
Valine | 44.08 | 50.60 | 19.90–23.30 | 51.10 | 54.10 | 60.60 |
Methionine | 43.91 | 43.60 | 6.50–8.40 | 17.50 | 21.00 | 26.00 |
Isoleucine | 33.38 | 46.50 | 12.70–14.20 | 38.30 | 36.70 | 42.90 |
Leucine | 76.02 | 68.80 | 26.00–30.30 | 68.10 | 122.40 | 80.90 |
Tyrosine | 28.90 | 44.00 | 13.10–17.40 | 31.20 | 37.10 | 43.60 |
Phenylalanine | 42.80 | 59.60 | 20.60–24.90 | 47.50 | 51.40 | 53.60 |
Tryptophan | – | 20.50 | 0.10 | 14.00 | 5.70 | 12.70 |
Millet processing and utilization
In 2022, global millet production was approximately 30.85 million MT. Processing this grain involves cleaning, de-husking, grading, and grinding (Supplementary Figure 1), resulting in by-products like husk, bran, and broken grains, which make up to 35% of the grain’s weight (Suri et al., 2024b). Cleaning removes physical impurities like soil, dust, and stones, while de-husking, using central dehullers, removes the husks. The hulled grains are then graded and milled for distribution and sale. By-products like bran and husk are rich in carbohydrates, fibre, and polyphenols, with bran containing 13.13%–16.23% protein and husk 7.45%–10.40% protein (Li et al., 2022). Millet husks and bran can be used in animal feed, functional foods, gluten-free bread, brewing, and biomass energy, while broken grains are suitable for flour or other food products (Suri et al., 2024b). However, these by-products are often underutilized, indicating a need for greater incorporation into food products to enhance protein content and health benefits. Millet is currently used in food and beverages, including pancakes, kichadi mix, porridge, sweet beer, and vermicelli. Researchers are also exploring its potential in nutraceutical products, extruded items, and 3D-printed food products (Anal et al., 2024).
Extraction of pearl, foxtail, and proso millet protein
Protein extraction from plant sources involves two categories: conventional techniques, like maceration using water, salt, solvents, detergents, and alkali, and non-conventional methods including microwave (MW)-assisted extraction, pulsed electric field, enzyme-assisted, high-pressure processing, and ultrasound techniques (Chandran et al., 2023). These approaches enhance the commercial value of plant-derived proteins.
Alkaline extraction/pH shifting method
Protein extraction from millet using alkaline methods typically involves defatting the flour with non-polar solvents, suspending it in an alkaline solution (pH 9–11), centrifuging, and precipitating the protein-rich supernatant at pH 4–5 and drying (Ji et al., 2019; Sachdev et al., 2023). The protein extraction process from millet is presented in Figure 1.
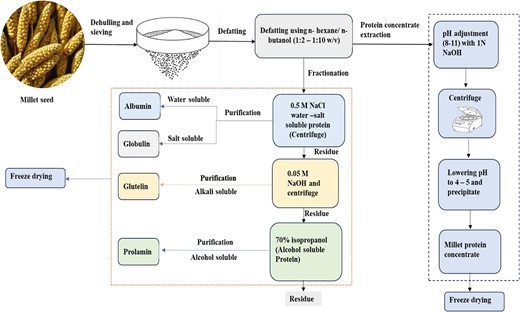
Foxtail millet protein (FMP) concentrate was recovered with a protein content of 70.79% by dispersing millet flour in distilled water (1:4 wt/vol), adjusting the pH to 9.5, centrifuging, and precipitating at pH 4 with 1 N HCl (Sharma et al., 2022). In comparison, Sachdev et al. (2023) optimized a similar extraction method with a flour-to-water ratio of 1:5 (wt/vol) and pH 10. Their method, however, resulted in a lower protein yield (44.64%) than that of Sharma et al. (2022). This difference may be attributed to the absence of a defatting step in Sachdev et al.,’s method. Additionally, Ji et al. (2019) extracted foxtail millet prolamin with 80% ethanol (1:8 wt/vol), yielding a protein content of 90%. Overall, the extraction conditions affect the protein content, particularly pH and defatting of the raw millets.
Protein recoveries of 54.02% and 60.70% from the Dawn and Plateau varieties of proso millet, respectively, demonstrate the varietal influence on protein yield (Akharume et al., 2020). Wet milling with 60% ethanol yielded higher protein contents (74.5% and 94.6%), suggesting predominant prolamin recovery (Wang et al., 2018). Alkaline extraction yielded 61.80%–76.57% protein for proso millet and 80.3% for pearl millet (Wang et al., 2021; Jhan et al., 2021), while in vitro hydrolysis of pearl millet resulted in 71% protein content (Agrawal et al., 2020). Variations in protein yields indicate that ethanol-based methods effectively isolate prolamins, while alkaline extraction provides high overall protein content. Combining these with hydrolysis may enhance functionality in millet for food applications.
Alkaline extraction is a common method for plant protein extraction, but it is time-consuming, energy-intensive, and may cause undesirable reactions at high pH. Techniques like ultrasound, MW, enzymatic treatments, and high-pressure homogenization effectively disrupt plant cell walls and increase protein yield. Several studies show that combining these approaches with alkaline extraction improves efficiency, reduces time and energy, and enhances protein functionality (Behere et al., 2021; Naik et al., 2022). Therefore, combining the alkaline extraction method with non-conventional techniques shows promise for millet protein isolation. Table 2 summarizes protein extraction methods using various solvents, including alkali, alcohol, and alcohol combined with NaCl.
Millet . | Processing method . | Treatment . | Protein content . | Recovery and properties of protein . | Reference . |
---|---|---|---|---|---|
Pearl millet | Successive fractionation | 70% aq. isopropyl–OH, | 81.40% | Ethanol and alkaline extraction recovered 48.5% and 24.2% of the original protein, respectively, with ethanol-extracted protein yielding higher amino acid recovery (76% vs. 62%). | Bailey and Sumrell (1980) |
Solid centrifuge with Aq. NaOH extract, pH 11 to pH 4 | 70.60% | ||||
Mineral acid extract, pH 4 to pH 2 | 19.80% | ||||
Residual meal | 4.00% | ||||
Alkaline extraction | Defatting at 1:10 (flour: hexane), 1:15 (wt/vol) flour: water, pH 8.5, precipitated at pH 4.5 | 85.19% | WHC (82.45 ml/g) and least gelation concentration (21%) were lower than other millet studied, with comparatively stable solubility across pH 2–10. | Agrawal et al. (2020) | |
1:4 (wt/vol) flour: water, pH 9.5, centrifugation 4,000 g for 30 min, precipitated at pH 4 | 80.30% | Maximum solubility (90.98%) and FS (42.09%) at pH 10 while nano-reduced protein had solubility 95.98% and FS 48.76%. | Jhan et al. (2021) | ||
Enzymatic | In vitro hydrolysis using pepsin and pancreatin | 71.00% | Hydrophobic amino acid, absolute zeta potential, solubility, WHC, and least gelation concentration significantly improved. OAC decreased significantly compared with protein isolate. | Agrawal et al. (2020) | |
Foxtail millet | Alkaline extraction | pH 9.5, 30 °C, centrifugation at 4,000 g for 30 min precipitate at pH 4 | 75.00%–80.00% | Compared with soy protein concentrate, higher WAC, emulsifying capacity, foam capacity, and stability. | Mohamed et al. (2009) |
1:4(wt/vol) (flour: distilled water), pH 9.5, centrifugation at 5,000 rpm for 30 min, and precipitation at pH 4 | 70.79% | Protein yield (12.13 g/100 g flour), and the highest solubility in the pH range of 7–9. EAI, ESI, FC, and FS were significantly lower than the ultrasound-treated samples. | Sharma et al. (2022) | ||
1:4 (wt/vol) flour: water, pH 9.5, centrifugation 4,000 g for 30 min, precipitated at pH 4 | 73.00% | Nano reduction of protein using ultrasound had better functionality including less turbidity and higher solubility. Foaming and emulsifying properties were better at low pH. | Jhan et al. (2021) | ||
1:5 (wt/vol) flour: distilled water, pH 10, centrifuge at 10,000 rpm for 30 min, precipitated at pH 5 | 44.64% | Mass yield and protein recovery were 3.4% and 9.91%, respectively. Higher content of lysine (2.13 g/100 g), the digestibility of soluble proteins in foxtail millet flour was reduced to 25.50%. | Sachdev et al. (2023) | ||
Ethanol combined with NaCl | 1: 8 (wt/vol) 80% ethanol–water ratio, centrifuge at 4,500 g for 15 min, 3 M NaCl (0.3%) for precipitation followed by dialysis to separate NaCl | 93.00% | The ethanol-extracted protein initially had less than 20% solubility, which increased to around 60% after 15 min of ultrasonic and heat treatment. | Ji et al. (2019) | |
Proso millet | Successive fractionation | Defatting by 1:6 flour: hexane, 40:60 chloroform: methanol to remove carotenes and xanthophylls, and successive fractionation | 54.02%–60.70% | Protein yield was 7.41–8.11 g/100 g flour. Dawn and Plateau variety protein fractions had poor solubility because of the dominant prolamin and glutenin fraction (75% of the total protein). | Akharume et al. (2020) |
Wet milling (MPW) | Millet grains were steeped for 3 hr at 40 °C, followed by overnight soaking at 4 °C, then blended, filtered, and centrifuged to isolate the protein layer before undergoing hydrolysis. | 74.50% | Solubility was evaluated as a function of ethanol concentration, with MPE being more soluble than MPW at all ethanol levels. Maximum solubility was observed at 60% ethanol. | Wang et al. (2018) | |
Ethanol extraction (MPE) | Dehulled millet seeds were milled, treated with hexane and chloroform-methanol for lipid and carotene removal, and then re-dispersed in 60% alcohol with 0.5% 2-mercaptoethanol at 60 °C before evaporating excess ethanol | 94.60% | Solubility was assessed across ethanol concentrations, with MPE showing higher solubility than MPW at all levels, peaking at 60% ethanol. | Wang et al. (2018) | |
Alkaline extraction | Defatting by n-hexane (1:10 wt/vol), Alkaline extraction pH 8, 0.5 N NaOH, precipitation at pH 3.8 | 61.80%–76.75% | WHC and OAC significantly increased, while FC and FS decreased after nitrogen fertilizer application. | Wang et al. (2021) | |
Wet milling | Millet grains were steeped (3 hr, 40 °C), followed by overnight steeping at 4 °C, blended, filtered, centrifuged to separate the protein layer, and subjected to hydrolysis | 80.00% | an average 50% reduction in digestibility (from 83.4% to 39.1%) after heat processing (100 °C), indicating the formation of hydrophobic protein aggregates during cooking. | Gulati et al. (2017) |
Millet . | Processing method . | Treatment . | Protein content . | Recovery and properties of protein . | Reference . |
---|---|---|---|---|---|
Pearl millet | Successive fractionation | 70% aq. isopropyl–OH, | 81.40% | Ethanol and alkaline extraction recovered 48.5% and 24.2% of the original protein, respectively, with ethanol-extracted protein yielding higher amino acid recovery (76% vs. 62%). | Bailey and Sumrell (1980) |
Solid centrifuge with Aq. NaOH extract, pH 11 to pH 4 | 70.60% | ||||
Mineral acid extract, pH 4 to pH 2 | 19.80% | ||||
Residual meal | 4.00% | ||||
Alkaline extraction | Defatting at 1:10 (flour: hexane), 1:15 (wt/vol) flour: water, pH 8.5, precipitated at pH 4.5 | 85.19% | WHC (82.45 ml/g) and least gelation concentration (21%) were lower than other millet studied, with comparatively stable solubility across pH 2–10. | Agrawal et al. (2020) | |
1:4 (wt/vol) flour: water, pH 9.5, centrifugation 4,000 g for 30 min, precipitated at pH 4 | 80.30% | Maximum solubility (90.98%) and FS (42.09%) at pH 10 while nano-reduced protein had solubility 95.98% and FS 48.76%. | Jhan et al. (2021) | ||
Enzymatic | In vitro hydrolysis using pepsin and pancreatin | 71.00% | Hydrophobic amino acid, absolute zeta potential, solubility, WHC, and least gelation concentration significantly improved. OAC decreased significantly compared with protein isolate. | Agrawal et al. (2020) | |
Foxtail millet | Alkaline extraction | pH 9.5, 30 °C, centrifugation at 4,000 g for 30 min precipitate at pH 4 | 75.00%–80.00% | Compared with soy protein concentrate, higher WAC, emulsifying capacity, foam capacity, and stability. | Mohamed et al. (2009) |
1:4(wt/vol) (flour: distilled water), pH 9.5, centrifugation at 5,000 rpm for 30 min, and precipitation at pH 4 | 70.79% | Protein yield (12.13 g/100 g flour), and the highest solubility in the pH range of 7–9. EAI, ESI, FC, and FS were significantly lower than the ultrasound-treated samples. | Sharma et al. (2022) | ||
1:4 (wt/vol) flour: water, pH 9.5, centrifugation 4,000 g for 30 min, precipitated at pH 4 | 73.00% | Nano reduction of protein using ultrasound had better functionality including less turbidity and higher solubility. Foaming and emulsifying properties were better at low pH. | Jhan et al. (2021) | ||
1:5 (wt/vol) flour: distilled water, pH 10, centrifuge at 10,000 rpm for 30 min, precipitated at pH 5 | 44.64% | Mass yield and protein recovery were 3.4% and 9.91%, respectively. Higher content of lysine (2.13 g/100 g), the digestibility of soluble proteins in foxtail millet flour was reduced to 25.50%. | Sachdev et al. (2023) | ||
Ethanol combined with NaCl | 1: 8 (wt/vol) 80% ethanol–water ratio, centrifuge at 4,500 g for 15 min, 3 M NaCl (0.3%) for precipitation followed by dialysis to separate NaCl | 93.00% | The ethanol-extracted protein initially had less than 20% solubility, which increased to around 60% after 15 min of ultrasonic and heat treatment. | Ji et al. (2019) | |
Proso millet | Successive fractionation | Defatting by 1:6 flour: hexane, 40:60 chloroform: methanol to remove carotenes and xanthophylls, and successive fractionation | 54.02%–60.70% | Protein yield was 7.41–8.11 g/100 g flour. Dawn and Plateau variety protein fractions had poor solubility because of the dominant prolamin and glutenin fraction (75% of the total protein). | Akharume et al. (2020) |
Wet milling (MPW) | Millet grains were steeped for 3 hr at 40 °C, followed by overnight soaking at 4 °C, then blended, filtered, and centrifuged to isolate the protein layer before undergoing hydrolysis. | 74.50% | Solubility was evaluated as a function of ethanol concentration, with MPE being more soluble than MPW at all ethanol levels. Maximum solubility was observed at 60% ethanol. | Wang et al. (2018) | |
Ethanol extraction (MPE) | Dehulled millet seeds were milled, treated with hexane and chloroform-methanol for lipid and carotene removal, and then re-dispersed in 60% alcohol with 0.5% 2-mercaptoethanol at 60 °C before evaporating excess ethanol | 94.60% | Solubility was assessed across ethanol concentrations, with MPE showing higher solubility than MPW at all levels, peaking at 60% ethanol. | Wang et al. (2018) | |
Alkaline extraction | Defatting by n-hexane (1:10 wt/vol), Alkaline extraction pH 8, 0.5 N NaOH, precipitation at pH 3.8 | 61.80%–76.75% | WHC and OAC significantly increased, while FC and FS decreased after nitrogen fertilizer application. | Wang et al. (2021) | |
Wet milling | Millet grains were steeped (3 hr, 40 °C), followed by overnight steeping at 4 °C, blended, filtered, centrifuged to separate the protein layer, and subjected to hydrolysis | 80.00% | an average 50% reduction in digestibility (from 83.4% to 39.1%) after heat processing (100 °C), indicating the formation of hydrophobic protein aggregates during cooking. | Gulati et al. (2017) |
Note. WHC = water-holding capacity; FS = foaming stability; OAC = oil-absorption capacity; EAI = emulsifying activity index; ESI = emulsion stability index; MPE = millet protein extract (ethanol); MPW = millet protein by wet milling.
Millet . | Processing method . | Treatment . | Protein content . | Recovery and properties of protein . | Reference . |
---|---|---|---|---|---|
Pearl millet | Successive fractionation | 70% aq. isopropyl–OH, | 81.40% | Ethanol and alkaline extraction recovered 48.5% and 24.2% of the original protein, respectively, with ethanol-extracted protein yielding higher amino acid recovery (76% vs. 62%). | Bailey and Sumrell (1980) |
Solid centrifuge with Aq. NaOH extract, pH 11 to pH 4 | 70.60% | ||||
Mineral acid extract, pH 4 to pH 2 | 19.80% | ||||
Residual meal | 4.00% | ||||
Alkaline extraction | Defatting at 1:10 (flour: hexane), 1:15 (wt/vol) flour: water, pH 8.5, precipitated at pH 4.5 | 85.19% | WHC (82.45 ml/g) and least gelation concentration (21%) were lower than other millet studied, with comparatively stable solubility across pH 2–10. | Agrawal et al. (2020) | |
1:4 (wt/vol) flour: water, pH 9.5, centrifugation 4,000 g for 30 min, precipitated at pH 4 | 80.30% | Maximum solubility (90.98%) and FS (42.09%) at pH 10 while nano-reduced protein had solubility 95.98% and FS 48.76%. | Jhan et al. (2021) | ||
Enzymatic | In vitro hydrolysis using pepsin and pancreatin | 71.00% | Hydrophobic amino acid, absolute zeta potential, solubility, WHC, and least gelation concentration significantly improved. OAC decreased significantly compared with protein isolate. | Agrawal et al. (2020) | |
Foxtail millet | Alkaline extraction | pH 9.5, 30 °C, centrifugation at 4,000 g for 30 min precipitate at pH 4 | 75.00%–80.00% | Compared with soy protein concentrate, higher WAC, emulsifying capacity, foam capacity, and stability. | Mohamed et al. (2009) |
1:4(wt/vol) (flour: distilled water), pH 9.5, centrifugation at 5,000 rpm for 30 min, and precipitation at pH 4 | 70.79% | Protein yield (12.13 g/100 g flour), and the highest solubility in the pH range of 7–9. EAI, ESI, FC, and FS were significantly lower than the ultrasound-treated samples. | Sharma et al. (2022) | ||
1:4 (wt/vol) flour: water, pH 9.5, centrifugation 4,000 g for 30 min, precipitated at pH 4 | 73.00% | Nano reduction of protein using ultrasound had better functionality including less turbidity and higher solubility. Foaming and emulsifying properties were better at low pH. | Jhan et al. (2021) | ||
1:5 (wt/vol) flour: distilled water, pH 10, centrifuge at 10,000 rpm for 30 min, precipitated at pH 5 | 44.64% | Mass yield and protein recovery were 3.4% and 9.91%, respectively. Higher content of lysine (2.13 g/100 g), the digestibility of soluble proteins in foxtail millet flour was reduced to 25.50%. | Sachdev et al. (2023) | ||
Ethanol combined with NaCl | 1: 8 (wt/vol) 80% ethanol–water ratio, centrifuge at 4,500 g for 15 min, 3 M NaCl (0.3%) for precipitation followed by dialysis to separate NaCl | 93.00% | The ethanol-extracted protein initially had less than 20% solubility, which increased to around 60% after 15 min of ultrasonic and heat treatment. | Ji et al. (2019) | |
Proso millet | Successive fractionation | Defatting by 1:6 flour: hexane, 40:60 chloroform: methanol to remove carotenes and xanthophylls, and successive fractionation | 54.02%–60.70% | Protein yield was 7.41–8.11 g/100 g flour. Dawn and Plateau variety protein fractions had poor solubility because of the dominant prolamin and glutenin fraction (75% of the total protein). | Akharume et al. (2020) |
Wet milling (MPW) | Millet grains were steeped for 3 hr at 40 °C, followed by overnight soaking at 4 °C, then blended, filtered, and centrifuged to isolate the protein layer before undergoing hydrolysis. | 74.50% | Solubility was evaluated as a function of ethanol concentration, with MPE being more soluble than MPW at all ethanol levels. Maximum solubility was observed at 60% ethanol. | Wang et al. (2018) | |
Ethanol extraction (MPE) | Dehulled millet seeds were milled, treated with hexane and chloroform-methanol for lipid and carotene removal, and then re-dispersed in 60% alcohol with 0.5% 2-mercaptoethanol at 60 °C before evaporating excess ethanol | 94.60% | Solubility was assessed across ethanol concentrations, with MPE showing higher solubility than MPW at all levels, peaking at 60% ethanol. | Wang et al. (2018) | |
Alkaline extraction | Defatting by n-hexane (1:10 wt/vol), Alkaline extraction pH 8, 0.5 N NaOH, precipitation at pH 3.8 | 61.80%–76.75% | WHC and OAC significantly increased, while FC and FS decreased after nitrogen fertilizer application. | Wang et al. (2021) | |
Wet milling | Millet grains were steeped (3 hr, 40 °C), followed by overnight steeping at 4 °C, blended, filtered, centrifuged to separate the protein layer, and subjected to hydrolysis | 80.00% | an average 50% reduction in digestibility (from 83.4% to 39.1%) after heat processing (100 °C), indicating the formation of hydrophobic protein aggregates during cooking. | Gulati et al. (2017) |
Millet . | Processing method . | Treatment . | Protein content . | Recovery and properties of protein . | Reference . |
---|---|---|---|---|---|
Pearl millet | Successive fractionation | 70% aq. isopropyl–OH, | 81.40% | Ethanol and alkaline extraction recovered 48.5% and 24.2% of the original protein, respectively, with ethanol-extracted protein yielding higher amino acid recovery (76% vs. 62%). | Bailey and Sumrell (1980) |
Solid centrifuge with Aq. NaOH extract, pH 11 to pH 4 | 70.60% | ||||
Mineral acid extract, pH 4 to pH 2 | 19.80% | ||||
Residual meal | 4.00% | ||||
Alkaline extraction | Defatting at 1:10 (flour: hexane), 1:15 (wt/vol) flour: water, pH 8.5, precipitated at pH 4.5 | 85.19% | WHC (82.45 ml/g) and least gelation concentration (21%) were lower than other millet studied, with comparatively stable solubility across pH 2–10. | Agrawal et al. (2020) | |
1:4 (wt/vol) flour: water, pH 9.5, centrifugation 4,000 g for 30 min, precipitated at pH 4 | 80.30% | Maximum solubility (90.98%) and FS (42.09%) at pH 10 while nano-reduced protein had solubility 95.98% and FS 48.76%. | Jhan et al. (2021) | ||
Enzymatic | In vitro hydrolysis using pepsin and pancreatin | 71.00% | Hydrophobic amino acid, absolute zeta potential, solubility, WHC, and least gelation concentration significantly improved. OAC decreased significantly compared with protein isolate. | Agrawal et al. (2020) | |
Foxtail millet | Alkaline extraction | pH 9.5, 30 °C, centrifugation at 4,000 g for 30 min precipitate at pH 4 | 75.00%–80.00% | Compared with soy protein concentrate, higher WAC, emulsifying capacity, foam capacity, and stability. | Mohamed et al. (2009) |
1:4(wt/vol) (flour: distilled water), pH 9.5, centrifugation at 5,000 rpm for 30 min, and precipitation at pH 4 | 70.79% | Protein yield (12.13 g/100 g flour), and the highest solubility in the pH range of 7–9. EAI, ESI, FC, and FS were significantly lower than the ultrasound-treated samples. | Sharma et al. (2022) | ||
1:4 (wt/vol) flour: water, pH 9.5, centrifugation 4,000 g for 30 min, precipitated at pH 4 | 73.00% | Nano reduction of protein using ultrasound had better functionality including less turbidity and higher solubility. Foaming and emulsifying properties were better at low pH. | Jhan et al. (2021) | ||
1:5 (wt/vol) flour: distilled water, pH 10, centrifuge at 10,000 rpm for 30 min, precipitated at pH 5 | 44.64% | Mass yield and protein recovery were 3.4% and 9.91%, respectively. Higher content of lysine (2.13 g/100 g), the digestibility of soluble proteins in foxtail millet flour was reduced to 25.50%. | Sachdev et al. (2023) | ||
Ethanol combined with NaCl | 1: 8 (wt/vol) 80% ethanol–water ratio, centrifuge at 4,500 g for 15 min, 3 M NaCl (0.3%) for precipitation followed by dialysis to separate NaCl | 93.00% | The ethanol-extracted protein initially had less than 20% solubility, which increased to around 60% after 15 min of ultrasonic and heat treatment. | Ji et al. (2019) | |
Proso millet | Successive fractionation | Defatting by 1:6 flour: hexane, 40:60 chloroform: methanol to remove carotenes and xanthophylls, and successive fractionation | 54.02%–60.70% | Protein yield was 7.41–8.11 g/100 g flour. Dawn and Plateau variety protein fractions had poor solubility because of the dominant prolamin and glutenin fraction (75% of the total protein). | Akharume et al. (2020) |
Wet milling (MPW) | Millet grains were steeped for 3 hr at 40 °C, followed by overnight soaking at 4 °C, then blended, filtered, and centrifuged to isolate the protein layer before undergoing hydrolysis. | 74.50% | Solubility was evaluated as a function of ethanol concentration, with MPE being more soluble than MPW at all ethanol levels. Maximum solubility was observed at 60% ethanol. | Wang et al. (2018) | |
Ethanol extraction (MPE) | Dehulled millet seeds were milled, treated with hexane and chloroform-methanol for lipid and carotene removal, and then re-dispersed in 60% alcohol with 0.5% 2-mercaptoethanol at 60 °C before evaporating excess ethanol | 94.60% | Solubility was assessed across ethanol concentrations, with MPE showing higher solubility than MPW at all levels, peaking at 60% ethanol. | Wang et al. (2018) | |
Alkaline extraction | Defatting by n-hexane (1:10 wt/vol), Alkaline extraction pH 8, 0.5 N NaOH, precipitation at pH 3.8 | 61.80%–76.75% | WHC and OAC significantly increased, while FC and FS decreased after nitrogen fertilizer application. | Wang et al. (2021) | |
Wet milling | Millet grains were steeped (3 hr, 40 °C), followed by overnight steeping at 4 °C, blended, filtered, centrifuged to separate the protein layer, and subjected to hydrolysis | 80.00% | an average 50% reduction in digestibility (from 83.4% to 39.1%) after heat processing (100 °C), indicating the formation of hydrophobic protein aggregates during cooking. | Gulati et al. (2017) |
Note. WHC = water-holding capacity; FS = foaming stability; OAC = oil-absorption capacity; EAI = emulsifying activity index; ESI = emulsion stability index; MPE = millet protein extract (ethanol); MPW = millet protein by wet milling.
Enzymatic-assisted extraction
Enzymatic-assisted extraction, an eco-friendly method, uses enzymes to degrade plant cell walls and release proteins while hydrolyzing them into smaller, more soluble components. Pepsin and pancreatin hydrolysis of pearl millet improved essential amino acid content, increasing leucine from 92.17 to 128.04 mg/g and threonine from 25.62 to 33.19 mg/g, though protein content dropped from 85.19% to 71% due to denaturation (Agrawal et al., 2020). Likewise, various enzymes—protamex, papain, Alcalase, flavourzyme, and neutrase—were applied to investigate their effects on the functional properties of FMPs (Kamara et al., 2010). These studies revealed improved functionality, suggesting potential use in food systems. Despite the advantages of enhanced functionality from enzyme treatment, the high cost and energy demands associated with enzymes limit their widespread adoption.
MW-assisted extraction
Microwave-assisted extraction (MAE) utilizes dipole rotation and ionic polarization through electromagnetic radiation (300 MHz—300 GHz) to rapidly heat polar cell components, increasing pressure and causing cell rupture for protein extraction. This method effectively isolates proteins from various plant sources, including watermelon seed and mustard meal, improving yield and techno-functional properties (Behere et al., 2021; Jahan et al., 2023). For instance, MAE of watermelon seed protein (50 W, 2 min, pH 10, and 1:30 solid-to-solvent ratio) resulted in a 25% increase in protein recovery compared with conventional batch extraction (Behere et al., 2021). Microwave treatment (600 W, 30 min, 1:7 grain: water) of proso millet flour improved water-holding capacity (WHC) (by 64.80%) and oil-absorption capacity (OAC) (by 42.86%) due to partial protein denaturation. However, protein content (4.48%) was lower compared with other millets (Kumar et al., 2020).
Despite its advantages—such as efficiency, user-friendliness, and cost-effectiveness—research on MW-assisted protein extraction from millet remains limited. The rapid heating can denature proteins, affecting yield and functionality. For instance, MW-treated foxtail millet (784.30 W, 4 min) had a lower yield (70.5%) than untreated millet (80.5%) due to protein denaturation (Rao et al., 2023). Therefore, further studies should focus on optimizing MW parameters to maximize yield while preserving protein quality and functionality.
Ultrasound-assisted extraction
Ultrasound-assisted extraction is an efficient, eco-friendly method that enhances protein yield and functionality, as demonstrated in bitter-melon seeds and eggshell membranes (Jain & Anal, 2016; Naik et al., 2022), but its application to millet protein is still limited. Akharume and Adedeji (2023) found that high-power ultrasound (50%–100% amplitude, 29.29–52.72 W, 5–10 min) enhanced prolamin and glutelin solubility, foaming, and digestibility in proso millet, with pepsin digestibility increasing by 33.09% for Dawn prolamin and 48.57% for Plateau glutelin after a 52.72 W, 10 min treatment. This improvement is attributed to cavitation, which enhances shear rate, micro-streaming, and enzyme accessibility. Similarly, Sharma et al. (2022) demonstrated improvement in solubility, emulsification, and foaming after high-intensity ultrasound (5%–10% amplitude, 5–20 min) treatment on FMP, achieving a 70.79% protein recovery. Additionally, ultrasound at 73.95 W/cm2 for 12.5 min improved proso millet’s functional properties (Nazari et al., 2018). Despite promising results showing improved solubility, digestibility, and functional properties from ultrasound treatment on millet proteins, the limited research and variability in ultrasound parameters highlight the need for more standardized and comprehensive studies.
Structural properties
Fourier transform infrared spectroscopy (FTIR) analysis of foxtail millet treated with MW and hot air radio frequency (HARF) heating shows significant changes in protein structure, with MW reducing β-sheet and α-helix content more than HARF due to hydrogen bond disruption and thermal effects (Rao et al., 2023). FTIR spectra of foxtail millet’s prolamin fraction reveal structural modifications after cooking, with reduced O-H and N-H vibrations, higher β-sheet content, and lower α-helix and random coil proportions (Zhang et al., 2021). High intensity ultrasound (HIU) treatments (24–37 W/cm2, 5–20 min,) of foxtail millets cause shifts in α-helix, β-sheet, β-turn, and random coil configurations, affecting protein conformation (Sharma et al., 2022).
X-ray diffraction (XRD) analysis of MW and HARF-treated FMPs showed an amorphous halo at 2θ = 20° indicating structural alterations, with relative crystallinity decreasing from 40.86% (untreated) to 33.67% and 32.35%, respectively (Rao et al., 2023). This reduction in crystallinity results from mild protein denaturation and the interconnection of denatured protein molecules through thermal and non–thermal processes, including carbon-centred free radicals (Malik & Saini, 2019).
Techno–functional properties
Solubility
The solubility of grain proteins (albumins, globulins, glutelins, and prolamins) varies with media and pH. Solubility studies on FMP (glutelin, prolamin) show the lowest solubility near the isoelectric point (pH 5), with increased solubility at acidic, neutral, and alkaline pH levels (Sharma et al., 2022). However, prolamin and glutelin fractions are less soluble due to their higher hydrophobic amino acid residues (Akharume et al., 2020). HIU treatment improved the solubility of FMP by breaking amino acid bonds and enhancing water–protein interactions (Jhan et al., 2021; Ji et al., 2019). This indicates that non-conventional extraction methods may enhance protein functionality, as solubility is crucial for emulsification and foaming applications. Further research is needed to explore the specific impact on the different protein fractions before recommending their application as a functional ingredient.
Pearl millet protein isolate extracted via an alkaline method showed minimal solubility at pH 4 but higher solubility between pH 5 and 9 (Agrawal et al., 2020), with hydrolysates showing maximum solubility at pH 9, likely due to reduced surface hydrophobicity and molecular weight. Bailey and Sumrell (1980) found that pearl millet protein exhibited maximum solubility at pH 11, suggesting that most pearl millet proteins exhibit solubility in alkaline pH conditions.
Proso millet’s albumin fraction had lower solubility at pH 3 (<20%), while the prolamin and glutelin showed increased solubility at pH 7–9 (Akharume et al., 2020). Similarly, the average solubility of proso millet was reported to be 55.78% and 59.68% for non-waxy and waxy varieties, respectively (Ren et al., 2023). In summary, protein structure, hydrophobicity, and particle size influence solubility, and are crucial factors for developing suitable ingredients for beverages and food industry applications.
WHC and OAC
Water-holding capacity and OAC are essential for food processing, influenced by protein structure and composition. Pearl millet protein hydrolysate exhibited a higher WHC (82.45 ml/g) than the isolate (59.16 ml/g) due to increased polar groups like NH2 and –COOH, enhancing water–protein interaction (Agrawal et al., 2020). Water-holding capacity in proso millet ranged from 2.35 to 2.93 g/g, with non-waxy proso millet protein showing significantly higher values than waxy variety (Ren et al., 2023). Nitrogen fertilizer improved WHC of proso millet, increasing values from 3.81–4.07 to 4.35–4.66 g/g (Wang et al., 2021). This improvement suggests a significant enhancement in the protein’s hydrophilic amino group ratio, potentially improving the grain’s texture and nutritional profile.
OAC increases with a higher composition of non-polar amino acids. Pearl millet hydrolysates had lower OAC (2.96 ml/g) than isolates (5.01 ml/g) due to reduced molecular weight (Agrawal et al., 2020). Wang et al. (2021) reported improvement in OAC from 239.92% to 256.52% for waxy and 218.64% to 268.42% for non-waxy proso millet after the application of nitrogen fertilizer. The fertilizer application led to a lighter, more porous structure, improving fat absorption. Kumar et al. (2020) observed an increase in the oil OAC of proso millet flour after MW cooking rising from 0.63 to 0.9 g/g, likely due to changes in non-polar protein subunits that enhance oil binding.
MW and HARF treatments significantly increase WHC and OAC in FMPs (WHC: 5.50% and 5.23%; OAC: 3.90% and 4.18%, respectively) due to better exposure of polar and non-polar side chains that facilitate water or oil binding (Rao et al., 2023). HARF treatment produced greater OAC and WHC than MW treatment, likely due to enhanced capillary and interfacial action from exposed hydrated carboxyl and hydroxyl groups in HARF-treated FMPs. Thus, the significant improvements in WHC and OAC of millet proteins through various treatments highlight the influence of amino acid composition, and processing methods, indicating the potential for enhanced functionality in food applications despite variations among millet types.
Emulsifying properties
Two key parameters, namely, emulsifying activity index (EAI) and emulsion stability index (ESI), assess protein emulsifying properties. Agrawal et al. (2020) found that pearl millet isolates had the lowest EAI (<20 m2/g) at pH 4, attributed to decreased solubility, while millet protein hydrolysate reached nearly 35 m2/g. The highest EAI was at pH 10, with 75 m2/g for pearl protein isolate and 91.88 m2/g for hydrolysates, indicating that enzyme modification enhances EAI.
Akharume et al. (2020) reported higher EAI for proso millet’s albumin and globulin fractions, with prolamin showing the lowest EAI (0.02–0.03 m2/g) due to low surface hydrophobicity (Lam & Nickerson, 2013). Prolamin had an ESI of 64 and 35 min for Dawn and Plateau varieties, respectively, indicating a stable emulsion, while globulin had the least stable emulsion (15 min ESI). Ren et al. (2023) showed that non-waxy proso millet protein had a better ESI (19.71 min), and waxy proso millet protein had a better EAI (2.21 m2/g). These results indicate that a careful selection of protein fractions is essential for specific applications, as there is a trade-off between EAI and ESI.
Foxtail millet’s emulsifying capacity was 74% and 65% for white and yellow varieties, respectively, attributed to greater hydrophobic exposure in the white variety (Mohamed et al., 2009). Compared with untreated samples (ES–22.67%; EAI–35.67%) and the MW-treated sample (ES–31.00%; EAI–42.67%), the protein extracted from foxtail millet treated with HARF showed higher ES at 22.67% and EAI at 46.33%. This improvement is due to partial protein unfolding, increased exposure to hydrophobic groups, and structural changes at higher temperatures (Rao et al., 2023). Moreover, HIU treatment significantly improves the EAI and ESI of FMPs. Protein concentrates outperformed prolamin (EAI: 5.5–14.22 m2/g; ESI: 27.96–46.02 min) and glutelin (EAI: 12.54–35.05 m2/g; ESI: 21.54–41.32 min) fractions, with values ranging from 40.41 to 75.23 m2/g for EAI and 14.88 to 42.85 min for ESI. HIU treatment caused the partial unfolding of globular proteins, enhancing their ability to form stable oil–water complexes, thereby improving both EAI and ESI (Sharma et al., 2022). Overall, emulsifying activity depends on protein characteristics, and both foxtail and proso millet show promising potential as emulsifiers in the food industries.
Foaming capacity and foaming stability
Certain food products, like cakes and bread, rely on proteins to create stable foams, which are influenced by pH and processing. The glutelin fraction of proso millet exhibited the highest foaming capacity (FC) at 87% for the Dawn cultivar and 60% for the Plateau cultivar, while the prolamin fraction showed the lowest FC at 18% and 14%, respectively. The glutelin fraction also had the highest foaming stability (FS), approximately 97% and 88% for Dawn and Plateau cultivars, respectively, compared with the prolamin fraction’s lowest FS of 56% and 58% for Dawn and Plateau varieties (Akharume et al., 2020). The better FC and FS of glutelin suggest its potential in baked goods and beverages.
Foaming capacity was reported to be 137 and 124 g/ml for white and yellow varieties of foxtail millet, higher than that of soy protein concentrate, suggesting that the white variety of foxtail millet can be used as a foaming agent in protein-rich food (Mohamed et al., 2009). Variations in the foaming properties of FMP were observed across different treatments (Rao et al., 2023). Untreated samples showed higher FC (39.17%) and FS (47.83%) than MW-treated (FC: 36.00%, FS: 44.33%) and HARF-treated (FC: 33.50%, FS: 41.27%) samples, with thermal denaturation leading to insoluble protein clumps that hinder foaming (Ling et al., 2019). Furthermore, ultrasound treatment significantly enhanced FC for protein concentrates (21.36% to 89.14%), prolamin (7.32% to 27.59%), and glutelin (15.96% to 53.14%). All fractions exhibited notable FS, with protein concentrate at 67.85% and glutelin at 50.23%. Foaming stability improved as well, ranging from 67.85% to 95.64% for concentrates, 57.89% to 97.36% for prolamin, and 50.23% to 89.89% for glutelin, underscoring their potential as functional food ingredients (Sharma et al., 2022).
Conclusion and future perspectives
The growing global population underscores the need for affordable, nutritious, and sustainable protein sources. Plant-based proteins, including millet, have gained attention for their efficient production and lower environmental impact compared with animal-based proteins. Millet, with its favourable essential amino acid profile particularly methionine, which is scarce in most cereals, shows promise for diverse food applications. Improved extraction technologies using ultrasound, high-pressure, MW, or enzymatic modification can further improve millet protein’s functional properties. However, limited research on the proteomics of millets hinders their broader application. Advanced characterization of pearl, foxtail, and proso millet proteins using techniques like matrix-assisted laser desorption ionization-time of flight (MALDI–TOF), liquid chromatography-mass spectrometry (LC–MS), and nuclear magnetic resonance (NMR) could significantly enhance their potential in diverse food products and nutraceuticals.
Data availability
The data supporting this study’s findings are available in the article.
Author contributions
Sanjaya Karki (Conceptualization, Investigation, Writing—original draft [Equal], Writing—review & editing, Visualization), Susma Bhattarai (Conceptualization, Investigation, Writing—original draft, Writing—review & editing [Equal]), Ranjit Singh (Investigation, Writing—original draft [Equal], Writing—review & editing), and Anil Kumar Anal (Conceptualization, Supervision [Equal], Validation, Writing—review & editing)
Funding
The authors did not receive any funding for this article.
Conflicts of interest
The authors declare no competing interests.
Acknowledgements
The authors wish to acknowledge the Department of Food Agriculture and Bioresources, Asian Institute of Technology (AIT), for providing constructive suggestions and technical support during the preparation of this manuscript.