-
PDF
- Split View
-
Views
-
Cite
Cite
Kuang Canyan, Zulema Rosalia Arias, Kazuhiro Omori, Tadashi Yamamoto, Yuki Shinoda-Ito, Shogo Takashiba, The effects of soybeans and its derivatives on oral diseases: a narrative review, International Journal of Food Science and Technology, Volume 60, Issue 1, January 2025, vvae044, https://doi.org/10.1093/ijfood/vvae044
- Share Icon Share
Abstract
Soybean is a widely utilized protein source that originated in China and has been associated with preventing and treating various diseases. Soy protein has been found to inhibit fat accumulation effectively, and soybeans contain isoflavones, saponins, phytic acid, and other substances with preventive and therapeutic effects on heart disease, cancer, and diabetes. Furthermore, processed soybean products, such as Avocado-Soybean unsaponifiable, Tempeh, and Bowman-Birk inhibitor, have demonstrated health benefits. These products have been shown to have antibacterial, antioxidant, and anticancer effects in oral diseases. Numerous experiments have provided evidence of the antibacterial, antioxidant, and anticancer effects of polyunsaturated fatty acids, isoflavones, and soybean polypeptides. This comprehensive review assesses the relationship and mechanism of soybeans and their derivatives on oral diseases, providing valuable insights into their prevention and treatment.
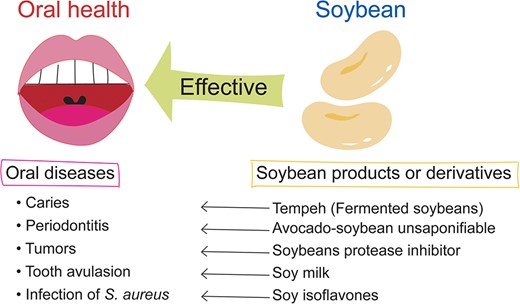
Introduction
Soybeans (Glycine max L) are legumes that serve as a high-quality protein source with significant biological value. Originating in China, soybeans have a 5,000-year history of cultivation and are now widely grown worldwide (do Prado et al., 2022; Rizzo, 2020). There are over 12,000 types of foods containing soy ingredients globally, with common products including miso, soy cheese, soy mayonnaise, soy milk, soy sauce, soy yoghurt, corn flour, tempeh, and tofu (Cai et al., 2021).
Soybeans contain 40% protein, 35% carbohydrates, 20% lipids, and 5% other substances (do Prado et al., 2022; Kim et al., 2021a, 2021b). The protein content of soybeans is more than double that of grains such as wheat and rice (Kudełka et al., 2021) and is equivalent to the protein content of meat, dairy products, and eggs while being cholesterol-free (Kim et al., 2021a, 2021b; Kudełka et al., 2021). Soy protein is a complete protein containing nine essential amino acids the human body requires. It primarily consists of stored protein, with other proteins like β-amylase, cytochrome c, lipoxygenase, and Bowman-Birk inhibitor (BBI) also present. Soybean protein helps lower the risk of cardiovascular disease and has a cholesterol-lowering effect, positively linked to the body’s initial cholesterol content (Kudełka et al., 2021). Additionally, soy protein can increase the concentration of gastrointestinal kinin, contributing to a feeling of fullness, and regulate the expression of genes related to appetite suppressors to inhibit fat accumulation and enhance fat metabolism, thereby aiding in weight loss (Kim et al., 2021a, 2021b; Weigle et al., 2005).
Soybeans also contain several phytochemicals, including carotenoids, coumarins, isoflavones, saponins, phytic acid, which play a role in preventing and treating chronic diseases such as heart disease, cancer, diabetes, and hypertension, as well as suppressing HIV and preventing gallstone formation, Alzheimer’s disease, and hyperlipidemia (Chatterjee et al., 2018; Kim et al., 2021a, 2021b). Furthermore, soybeans are rich in dietary fiber (Kim et al., 2021a; Mateos-Aparicio et al., 2008). The consumption of soybeans and their derivatives is associated with a low incidence of chronic diseases. Studies indicate that in China, Singapore, and Hawaii, soybeans are inversely linked to the risk of type 2 diabetes, prostate cancer risk, and cancer mortality (Nachvak et al., 2019; Rizzo 2020).
Studies have shown that fermented soy products effectively reduce the incidence of diabetes, hypertension, heart disease, and cancer (Jayachandran & Xu, 2019). Furthermore, soy fermentation by Bacillus subtilis or Bacillus licheniformis can reduce the harmful impact of intestinal bacteria and deter pathogenic bacteria by absorbing toxic substances (Kudełka et al., 2021). Fermented soybeans with Bacillus species include Tempeh, natto, soy sauce, and soy paste (Lee et al., 2018). Additionally, soy products like BBI and soy protein extract (SPE), obtained through extraction, have been found to have anti-tumor effects on oral, breast, colon, and prostate cancers (Fan et al., 2022; Fereidunian et al., 2014; Kennedy, 1998; Kingsley et al., 2011). Therefore, soy-related products considerably contribute to preserving health.
Oral diseases are among the most prevalent health issues worldwide and significantly impact patients’ quality of life, as well as pose substantial health and economic burdens. Prevention is crucial since most oral diseases are chronic and asymptomatic. The most common oral diseases include periodontal disease, dental caries, tooth loss, and oral cancer (Peres et al., 2019). Some reviews have suggested that soybean derivatives such as isoflavone promote oral health (Valizadeh et al., 2021); however, there are few comprehensive reports on oral diseases and soybeans. This review was focused on those main oral diseases and other oral infection-related diseases often literarily mentioned in connection with soy products, such as oral infection with Staphylococcus aureus (S. aureus) and tooth avulsion, to explore the impact of soybeans and their derivatives on oral diseases.
Main content
Soybeans and periodontal disease
Periodontal disease definition
Periodontal disease, a commonly occurring oral condition in the periodontal tissue, is a response to tissue destruction caused by microorganisms. It progresses from gingivitis, an excessive inflammatory response that destroys the supporting tissues of the teeth (Aral et al., 2020). The pathophysiological process of periodontal disease is regulated by various inflammatory cytokines, such as interleukin (IL)-1β and IL-6 (Kinane, 2001; Naruishi & Nagata, 2018). During the inflammatory response between immune cells and bacteria, the up-regulation of pro-inflammatory cytokines exacerbates the response, leading to increased activity of osteoclasts and subsequent destruction of alveolar bone (Murakami et al., 2020). Overactivation of these pro-inflammatory cytokines can contribute to systemic inflammation and interact with chronic diseases like cardiovascular diseases, diabetes, cancers, and chronic respiratory diseases. Therefore, inhibiting inflammatory cytokines is vital for preventing and treating periodontal disease (Cardoso et al., 2018).
Avocado-soybean unsaponifiables
The saponification reaction is a process in which lipids are hydrolyzed into glycerol and salt under alkaline conditions. During this reaction, the substances obtained that are insoluble in water but soluble in organic solvents are called unsaponifiables. Some examples of unsaponifiables include sterols, polymer alcohols, hydrocarbons, pigments, and fat-soluble vitamins. Avocado-soybean unsaponifiables (ASU), a mixture of avocado and soybean oil, can increase the content of soluble collagen (Basiri et al., 2020; Christiansen et al., 2015; Lamaud et al., 1982; Pourentezari et al., 2021). ASU is a natural derivative originally produced in France, most often configured in a ratio of 1:2, and contains chemical ingredients such as sterols and tocopherols (Christiansen et al., 2015; Salehi et al., 2020).
Sterols play a crucial role in the biological activity of articular chondrocytes. Additionally, ASU reduces the degradation of cartilage mediated by inflammatory factors by inhibiting inflammatory cytokines such as IL-1, IL-6, tumor necrosis factor, and more. It also promotes the synthesis of collagen and glycan, contributing to cartilage repair (Christiansen et al., 2015). ASU is primarily used to treat osteoarthritis (OA) (Christiansen et al., 2015; Oryan et al., 2015). Furthermore, ASU has beneficial effects in early scleroderma, characterized by upper limb oedema, erythema, low skin temperature, reduced sweating, and small cracks on the skin surface, as it can interfere with limb malformation, fibrous tissue shrinkage, and atrophy caused by this disease (Jablonska, 1998).
Research has confirmed that ASU, used for wound healing, can stimulate collagen production. Its anti-inflammatory properties also contribute to faster and smaller scar tissue formation, with denser and better-organized collagen fibers (Oryan et al., 2015).
Benefits of ASU on periodontal treatment
IL-1β serves as the primary pro-inflammatory cytokine in the development of periodontal disease. This cytokine is produced by various immune cells and can increase the number of eosinophils adhering to the blood vessel wall by activating endothelial cells, thereby enhancing the inflammatory response (Aral et al., 2020; Mize et al., 2015). On the other hand, higher levels of transforming growth factor-β1 (TGF-β1) are typically present in actively differentiated cells such as osteoblasts and platelets at sites of inflammation. TGF-β1 can promote tissue remodeling and wound healing (Mize et al., 2015).
An experiment revealed that in human periodontal ligament (HPL) fibroblasts treated with IL-1β, the mRNA level of the TGF-β family was lower than that of the control group. This suggests that IL-1β can decrease the expression of members of the TGF-β family, thereby worsening the erosion of supporting tissues in periodontal disease (Andriamanalijaona et al., 2006). However, after ASU addition, the downward trend in the number of TGF-β family members, which was originally decreased due to IL-1β stimulation, was suppressed. This indicates that ASU can enhance the expression of TGF-β family members, especially TGF-β1 and TGF-β2, and can counteract the inhibitory effect of IL-1β, thus promoting the healing of periodontal disease (Altinel et al., 2007; Andriamanalijaona et al., 2006; Basiri et al., 2020; Oliveira et al., 2017).
Interestingly, although the expression of TGF-β2 mRNA was suppressed in HPL cells treated with IL-1β, in alveolar bone, IL-1β appears to have a synergistic effect with ASU in up-regulating the expression of TGF-β2 protein. This may be related to the regulatory sequences of the promoter of TGF-β2 (Andriamanalijaona et al., 2006). Another study using a ligature-induced periodontitis rat model showed that the ratio of bone filling in the furcation area of the groups treated with ASU was higher than that of the control group. This effect was suggested to be due to ASU's anti-inflammatory and proliferative effects on connective tissue (de Oliveira et al., 2024; Oliveira et al., 2017; Tsurumaki et al., 2019).
Overall, ASU is characterized by its anti-inflammatory potential and works as a supplemental treatment for OA (Salehi et al., 2020; Yang et al., 2024), which would be the prospective candidate for periodontal treatment.
Benefits of functional peptides on periodontal bacteria
Soy-derived bioactive peptides are produced from soy proteins by hydrolysis, and their functions, such as anti-inflammatory, anti-tumor, and antioxidative effects, have been reported (Chatterjee et al., 2018; Lule et al., 2015). Porphyromonas gingivalis (P. gingivalis) and Fusobacterium nucleatum (F. nucleatum) have been recognized as common gram-negative anaerobic bacteria strongly associated with periodontal disease (Brennan & Garrett, 2019; Mysak et al., 2014). Cationic and amphiphilic peptides called Glycinin-17, BCAS-16, and BCBS-11, derived from soybean proteins, have been reported to exhibit antibacterial and antibiofilm activity due to their membrane-disrupting ability in periodontopathic P. gingivalis and other several oral bacteria (Lwin et al., 2022; Taniguchi et al., 2017). Thus, Soybean derivatives could help control periodontal disease from both immunological and antibacterial aspects.
Soybeans and dental caries
Caries definition
Dental caries and periodontal disease are considered to be the most prevalent oral non-communicable diseases globally. These diseases not only impact oral function, causing pain and infection, but can also contribute to an increased risk of systemic conditions such as cardiovascular disease and respiratory tract infections (Cianetti et al., 2021; Simon, 2007). Streptococcus mutans (S. mutans) is identified as a significant cariogenic bacterium responsible for tooth decay. It is known for its acid resistance, acid production, and involvement in forming biofilms and caries on teeth in combination with other oral bacteria (Lin et al., 2021). The activity of S. mutans is intensified in an environment with decreased pH after sugar consumption, and its binding to teeth is strengthened by generating water-insoluble glucan (Simon, 2007).
A multitude of bacteria attaches to dental enamel using the extracellular polymeric substances (EPS) produced by S. mutans, which serve as a framework for the further development of biofilms (Kriswandini et al., 2020; Lin et al., 2021). Mature biofilms form a three-dimensional tower structure, which can harbor bacteria, supply them with necessary nutrients, and protect them from antibiotics, immune cell attacks, and environmental stress (Lin et al., 2021). The ability of S. mutans to create and sustain an acidic environment in the oral cavity makes it unsuitable for other co-existing bacteria, ultimately leading them to become caries-causing pathogens. Subsequently, tooth demineralization and cavitation occur, exacerbating the progression of dental caries (Simon, 2007).
Tempeh
Tempeh is a soy food that is sodium-free and high in protein. It was originally fermented by the people of Central Java in Indonesia around the 18th century. It is primarily made from soybeans but can also be fermented with other beans and seeds, such as sword beans, velvet beans, and pigeon beans. The production of tempeh involves four main steps: soaking, boiling, inoculating with microbes, and then incubating at room temperature. The Rhizopus species used in Indonesia for fermentation include R. oligosporus, R. oryzae, R. arhizus, R. stolonifer, and R. microsporus. They use hibiscus or teak leaf as an inoculant and cooked rice to prepare the powder. However, tempeh has no absolute standard production process (Ahnan-Winarno et al., 2021; Astuti et al., 2000; Kameda et al., 2018; Rizzo, 2024).
Tempeh is not just a delicious addition to the diet; it also offers many health benefits. It can protect human and animal intestinal cells from infection and has a significant role in maintaining intestinal and bone health and preventing cardiovascular diseases and certain types of cancer (Afifah et al., 2020; Bintari & Nugraheni, 2017; Kiers et al., 2007; Kuligowski et al., 2013; Nurkolis et al., 2022; Wresdiyati et al., 2021; Yang et al., 2018; Yuliani et al., 2016). Additionally, it has anti-hypertensive, anti-diabetic, antioxidant, antibacterial, and antidiarrheal effects, making it a powerhouse of health benefits (Ahmad et al., 2015; Aoki et al., 2003; Bintari et al., 2015; Lim & Tay, 2011; Muawanah et al., 2022; Tamam et al., 2019; Teoh et al., 2024; Watanabe et al., 2006).
During the fermentation process, the protein in tempeh breaks down into free amino acids, peptides, or simple proteins, releasing iron from the ferritin complex and increasing the soluble iron content. (Tamam et al., 2019; Wronkowska et al., 2015) Furthermore, Rhizopus produces phytase, which reduces the phytic acid content and enhances the mineral bioavailability (Eklund-Jonsson et al., 2006). Tempeh also contains increased levels of various tocopherols, enhancing its natural antioxidant activity (Hubert et al., 2008).
Tempeh stands out in the world of soy products due to its unique nutritional value. Isoflavones in tempeh have anti-tumor, antioxidant, and anti-diabetic effects, making it a nutritional powerhouse (Romulo & Surya, 2021).
Antibacterial effect of tempeh against pathogens of dental caries
In the process of growing and fermenting Rhizopus in heat-processed soybean, lipase (produced by Rhizopus) breaks down triacylglycerol into free long-chain polyunsaturated fatty acids (such as Linoleic acid and α-linolenic acid) and monolinolenins (Astuti et al., 2000; Ito et al., 2020; Kusumah et al., 2020). Studies have shown that the level of free fatty acids in soybeans fermented by R. oryzae at 37 °C increased from 0.3 to 0.8 g/100 g in dry tempeh (Derue et al., 1994). These fatty acids exhibit strong antibacterial effects on gram-positive bacteria (Casillas-Vargas et al., 2021). S. mutans, the main pathogen of dental caries, is a gram-positive anaerobe. Research has demonstrated that tempeh has an inhibitory effect against S. mutans, with tempeh extract fermented by Rhizopus stolonifer showing the strongest inhibitory effect against S. mutans at a concentration of 1 mg/ml (Ito et al., 2020).
The antibacterial effects of polyunsaturated fatty acids (PUFA) target bacterial membranes, increasing their permeability. This causes the bacteria's contents to leak through the holes created in the cell membrane, ultimately inhibiting bacterial growth. If the concentration of PUFA continues to increase, the fluidity of the cell membrane will increase excessively, ultimately leading to the bacteria's dissolution (Desbois & Smith, 2010; Kusumah et al., 2020).
The biological oxidation process of the electron transfer chain on the bacterial cell membrane plays a crucial role in synthesizing adenosine triphosphate (ATP). PUFAs can hinder this process by combining with electron transfer chain carriers, disrupting electron transfer. Furthermore, due to cis bonds, PUFAs can be inserted into the membrane to increase its fluidity and permeability. PUFAs can also directly bind to ATP synthase, preventing the enzyme from functioning normally. Additionally, the amphiphilic structure of PUFA enables it to penetrate the lipid bilayer, affecting the concentration of protons inside the bacteria and reducing the proton gradient, ultimately inhibiting ATP synthesis. As a result, bacteria tend to die under the combined influence of PUFA on cell membranes and ATP (Desbois & Smith, 2010). It was summarized that PUFA showed an antimicrobial effect on various bacteria by stimulating NADPH-dependent superoxide production and an inflammatory impact by enhancing the immune system (Casillas-Vargas et al., 2021; Das, 2018) (Figure 1).
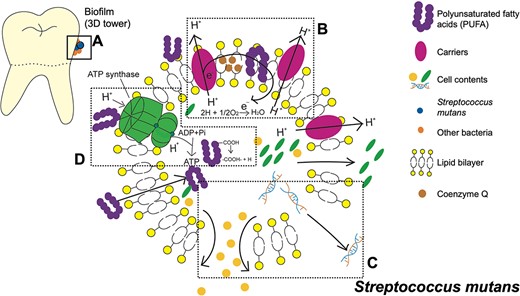
Three-dimensional (3D) tower structure biofilm on the dental enamel surface and the antibacterial mechanism of polyunsaturated fatty acids (PUFA) against Streptococcus mutans (S. mutans). (A) Biofilm 3D tower structure includes S. mutans and other bacteria. (B) PUFA combines with the carriers or is inserted within the lipid bilayer, preventing the transportation of electrons or protons. (C) Due to the addition of PUFAs, the fluidity and permeability of cell membranes are over-enhanced. Pores appear on the membrane's surface, and the bacteria's nutrients are lost. (D) PUFA also binds with adenosine triphosphate (ATP) synthase to directly affect the synthesis of ATP. In addition, it penetrates the cell membrane to separate the protons in the carboxyl group, thereby reducing the proton gradient to hinder the synthesis of ATP.
Soybeans and oral infections caused by S. aureus
Oral infections caused by S. aureus
S. aureus is a gram-positive opportunistic pathogen closely associated with infection. It is frequently found in the oral cavity and can be transmitted from person to person. S. aureus is known to cause various oral infectious diseases, such as angular cheilitis, parotitis, osteomyelitis of the jaw, and staphylococcal mucositis. Common clinical symptoms of S. aureus infection in the oral cavity include oral discomfort and oral erythroplakia (Passariello et al., 2012; Smith et al., 2001; Smith et al., 2003).
S. aureus can adhere to oral tissues, dental prostheses, and implants, possibly leading to implant failure. The surface proteins of S. aureus facilitate the attachment of host proteins and the formation of biofilms after implantation. Once S. aureus colonizes an implant, it can only be eliminated through thermal disinfection or implant replacement (Lister & Horswill, 2014; Rokadiya & Malden, 2008; Smith et al., 2001). The biofilm protects S. aureus from the host's immune response and can hinder the effects of antibiotics. As the biofilm matures, individual S. aureus can detach from the biofilm, creating new avenues for infection or causing acute infections such as sepsis. S. aureus also produces a toxin, neutral sphingomyelinase, which can cause the lysis of erythrocytes and lymphocytes (Lister & Horswill, 2014).
Although S. aureus is not typically considered part of the normal oral microbiota (Smith et al., 2001), it has been reported to be present in the oral environment regardless of the periodontal status of the host (Koukos et al., 2015).
Antibacterial effect of soybeans against S. aureus
Soy isoflavones are phenolic compounds produced during soybean growth and metabolism, with antioxidant and anti-tumor effects. S. aureus are aerobic or facultative anaerobes. When ingested sugars, such as glucose, are oxidized aerobically, they are mainly decomposed into carbon dioxide and water. In this process, hydrogen ions released participate in the biological oxidation process through the electron transfer chain, and S. aureus uses these operations to obtain energy. Malate dehydrogenase (MDH) is a nucleotide-binding protein crucial in the tricarboxylic acid (TCA) cycle. In the TCA cycle, MDH dehydrogenates malate to oxaloacetate. Soy isoflavones are believed to bind to MDH and inhibit its activity, impacting the entire cycle. As a result, S. aureus cannot produce the necessary ATP to survive and eventually dies (Minárik et al., 2002). It has been reported that soybean isoflavones can also inhibit nucleic acid synthesis in S. aureus, possibly due to the bacteria's inability to obtain enough energy for synthesis (Wang et al., 2010).
Polyunsaturated fatty acids in tempeh also have antibacterial effects against gram-positive bacteria, including S. aureus (Kusumah et al., 2020; Das 2018; Yuyama et al., 2020). During fungal growth and fermentation after soybean thermal processing, lipolytic enzymes produced by fermentative bacteria convert triglycerides in soybean oil into long-chain unsaturated fatty acids, mainly linoleic acid. These polyunsaturated fatty acids act on the cell membrane of S. aureus as the main antibacterial substance. High concentrations of unsaturated fatty acids lead to peroxidation of unsaturated double bonds, greatly increasing cell membrane permeability and inhibiting the cell's respiratory metabolism (Li, 2009). The antibacterial activity of unsaturated fatty acids, such as linoleic acid, against S. aureus was demonstrated by inhibiting fatty acid synthesis (Zheng et al., 2005) (Figure 2).
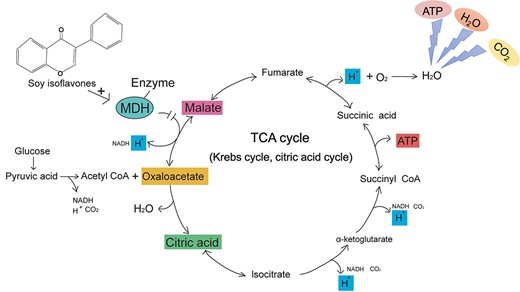
Aerobic oxidation of sugars in Staphylococcus aureus (S. aureus). Glucose generates pyruvic acid through glycolysis. Acetyl CoA generated by oxidative decarboxylation of pyruvic acid is condensed with oxaloacetate to form citric acid to start the first step of the tricarboxylic acid cycle (TCA cycle). During the entire oxidation process, the glucose finally resolves into carbon dioxide and water, providing energy to S. aureus. The hydrogen ions released in the cycle participate in the biological oxidation process through the electron transport chain, combine with oxygen to generate water, and provide energy for the synthesis of adenosine triphosphate (ATP). Malate dehydrogenase (MDH) catalyzes the dehydrogenation of malate to oxaloacetate, a crucial step in the TCA cycle. Soy isoflavones bind to MDH, inhibiting its activity and disrupting the operation of the TCA cycle. Eventually, S. aureus cannot synthesize ATP and dies.
Soybeans and oral cancer
Oral cancer definition
Oral cancer and oropharyngeal cancer rank as the sixth most common malignant tumors worldwide. Over 90% of oral malignant tumors are oral squamous cell carcinomas (OSCCs). Other tumors of the oral cavity include small salivary glands and lymphomas (Abati et al., 2020; Warnakulasuriya, 2009; Warnakulasuriya & Kerr, 2021). The prognosis of oral cancer worsens as the disease progresses. Even in cases where oral cancer has been successfully treated, patients often experience changes in facial appearance and difficulties with functions such as chewing, swallowing, and speaking. The incidence of oral cancer is higher in South and Southeast Asia, and the primary causes are tobacco and alcohol consumption (Tranby et al., 2022; Warnakulasuriya, 2009). Most lip and mouth cancers are preventable, with daily consumption of fruits and vegetables associated with a lower risk of oral cancer. Additionally, for every 25 g/day increase in soy intake, the overall risk of cancer incidence decreases by 4% (Fan et al., 2022; Warnakulasuriya, 2009). Research has shown that substances present in soybeans, such as isoflavones, saponins, and phytic acid, have anti-cancer effects, leading to ongoing studies on soybean-derived cancer prevention drugs (Kennedy, 1998; Nurkolis et al., 2022).
Relationship between SPE and oral cancer
Research has shown that soy-derived isoflavones can inhibit the growth of oral cancer cells in laboratory studies. At concentrations higher than 20 mmol/L, isoflavones reduced the viability of OSCC cells in a manner that depended on the concentration (Johnson et al., 2010). Furthermore, at the same physiological concentration, soybean extract induced a higher apoptosis rate in cancer cells than single isoflavones. It was also observed that single isoflavones induced apoptosis in benign prostatic hyperplasia-1 (BPH-1) cells, indicating potential cytotoxic effects on non-cancer cells. However, this effect was not observed with isoflavones in whole soybean extracts. As such, combinations of active compounds in natural foods were considered safer and more effective chemo-preventive agents than individual compounds (Hsu et al., 2010; Kingsley et al., 2011).
Furthermore, an analysis of cells treated with soybean protein extract at a concentration of 50 μg/ml for 48 hr indicated that the extract may have anti-proliferative effects on certain cancer cells. The examination of cell cycle genes (c-myc, ODC, and p53) and apoptosis genes (caspase-2, caspase-8, and bax) of oral adenosquamous carcinoma cells (CAL27), tongue squamous cell carcinoma cells (SCC25), and human gingival fibroblast cells (HGF-1) showed that the soybean protein extract potentially inhibits the cell cycle gene ODC in CAL27 and SCC25 cells. Moreover, the extract significantly up-regulates the expression of apoptosis genes, caspase-2, caspase-8, and bax, in the cancer cells, further highlighting its potential in cancer treatment. Notably, this experiment further supported the idea that the whole soybean protein extract has no harmful effects on normal cells (HGF-1) (Kingsley et al., 2011).
The relationship between BBI and oral cancer
The BBI is a protease inhibitor extracted from soybeans with a molecular weight of 8,000. This protein has 71 amino acids and seven disulfide bonds. It possesses both chymotrypsin and trypsin inhibition sites and can bind 1:1 with either chymotrypsin, trypsin, or both enzymes. The anticancer activity of BBI is attributed to its chymotrypsin inhibitor activity (Kennedy, 1998; Meyskens, 2001). As a general non-toxic cancer preventative, BBI's ability to inhibit cancer in animals is more effective than other anti-cancer substances. BBI can be toxic to precancerous cells and some malignant cells in vitro. The trypsin-inhibitory ability of soybean protease inhibitors was found to promote atypical growth of the rat pancreas, but this negative effect was not seen in humans. The effects of these two protease inhibitor sites for trypsin and chymotrypsin differ in BBI (Hsieh et al., 2010; Kennedy, 1998).
The mechanism by which protease inhibitors inhibit carcinogenesis is unclear; however, it may be related to preventing polymorphonuclear leukocytes and other types of cells from releasing superoxide anion radical (O2•-) and hydrogen peroxide. BBI prevents the production of free radicals in cells, although it does not act as a free radical scavenger, thereby reducing oxidative damage (Kennedy, 1998; Ware et al., 1999; Clemente & Arques Mdel, 2014; Kennedy, 2021). Additionally, it has been found that BBI has dose-related activity against oral leukoplakia, a precancerous lesion. The yield of tumors and precancerous lesions was significantly reduced in BBI-treated animals, suggesting that BBI treatment destroys precancerous lesions. BBI can block the cleavage of the extracellular domain of Neu protein, thereby preventing the Neu protein from transitioning to the active conformation. (Armstrong et al., 2003; Kennedy, 1998; Kennedy, 2021; Meyskens, 2001) (Figure 3).
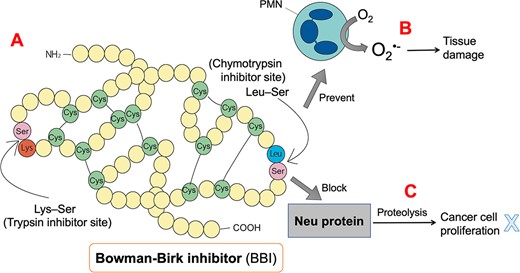
Structure of Bowman-Birk inhibitor (BBI) and its possible anticancer mechanism. BBI consists of 71 amino acids and seven disulfide bonds and possesses a trypsin inhibitory site (Lys-Ser) and a chymotrypsin inhibitory site (Leu-Ser). BBI can prevent polymorphonuclear cells (PMN) from producing a large amount of superoxide anion free radicals (O2•-) after consuming oxygen, thereby reducing tissue damage. In addition, BBI prevents the Neu protein from being converted into the active conformation by proteolysis.
Since BBI's purification is costly, BBIC (BBI Concentrate) was developed as a low-cost and dietary-friendly concentrated extract with the same carcinogenic ability as BBI. BBIC is stable and retains chymotrypsin inhibitory activity for over two years in vitro (Armstrong et al., 2003; Meyskens, 2001). BBIC is the same as BBI without any toxicity, even at 1066 CIU (chymotrypsin inhibitor units). BBIC removed most trypsin inhibitor activity, greatly enhancing chymotrypsin inhibitor activity. The dose of BBIC required to prevent cancer development was much lower than that required for the activity of a single BBI (Kennedy, 1998; Meyskens, 2001) (Figure 3).
Lunasin is another major bioactive component of BBIC, along with BBI. It is a 43-amino-acid-long polypeptide extracted from soybean seeds (Galvez et al., 2001; Hsieh et al., 2010; Lam et al., 2003). The tumor suppressor gene Rb recruits histone deacetylase (HDAC) to restore histone deacetylation, leading to tightly arranged chromatin and preventing the activation of gene transcription by the E2F transcription factor. The oncogene E1A can inactivate Rb, exposing the acetylation modification sites of histones. Lunasin acts similarly to HDAC, competing with histone acetylase (HAT) to bind to histones and prevent their acetylation, thus arresting mitosis and inducing cell death, which is also the chemopreventive effect of lunasin on cancer. Notably, lunasin only affects transfected cells, not interfering with immortalization (non-tumorigenicity) or the growth of established cancer cell lines (Galvez et al., 2001; Hsieh et al., 2010; Lam et al., 2003; Pabona et al., 2013; Seber et al., 2012). While single lunasin is readily digested by trypsin in the human body, when received as part of BBIC, it receives protection from BBI, greatly improving its bioavailability (Galvez et al., 2001) (Figure 4).
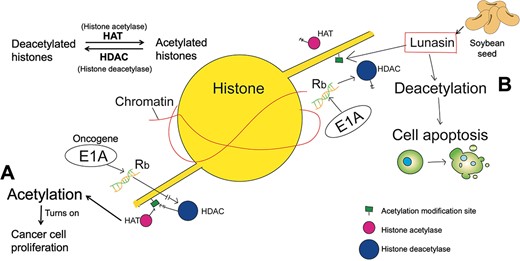
Lunasin prevents histone acetylation. Graphics are histones in transfected cells. Without lunasin, the oncogene E1A inactivates the tumor suppressor gene Rb, which recruits histone deacetylases (HDACs). At this time, histone acetylase (HAT) acetylates histones and turns on oncogene transcription (A). When lunasin is present, it competes with HAT for binding to deacetylated histones, disrupting cell division and leading to apoptosis (B).
Soybeans and tooth avulsion
Tooth avulsion definition
Tooth avulsion refers to the complete displacement of a tooth from its socket due to external forces. In such a situation, the periodontal support tissue becomes separated from the dental tissue, causing pulp necrosis as the pulp tissue loses its blood supply (Adnan et al., 2018; Côvre et al., 2021; Moazami et al., 2012; Poi et al., 2013; Trope, 2011). This complete avulsion occurs in approximately 1%–16% of all cases of permanent tooth traumas, with the maxillary central incisors being the most commonly affected teeth (Adnan et al., 2018; Moazzami et al., 2017; Trope, 2011).
Successful replantation depends on the tooth's dryness period and the survival of periodontal ligament (PDL) cells (Poi et al., 2013; Sigalas et al., 2004). PDL cells can become necrotic after 20 min of drying, and after 2 hr, their activity cannot be detected (Moazami et al., 2012; Moura et al., 2014; Reis et al., 2017; Saluja & Anegundi, 2016).
Replantation within the first 5 min of avulsion is the ideal recommendation for complete healing (Moura et al., 2012; Reis et al., 2017; Sigalas et al., 2004). However, tooth avulsion often occurs during outdoor sports, so immediate replantation is only sometimes possible. In such cases, choosing a suitable storage medium becomes critical (Moazzami et al., 2017; Reis et al., 2017). Avulsed teeth can be stored for 1–3 hr under proper conditions before replantation without complications (Chamorro et al., 2008).
Soymilk as a storage medium for avulsed teeth
Cells must grow under an osmotic pressure of 230–400 mOsmol/kg and a pH of 6.6–7.8. Thus, the storage medium must provide the necessary environment for cell survival and maintain cell function (Adnan et al., 2018; Silva et al., 2013). Hank's Balanced Salt Solution (HBSS) is the preferred storage medium recommended by the American Association of Endodontics (AAE guideline, 2013). It is non-toxic, has an osmotic pressure of 270–290 mOsmol/kg, a pH of 7.2, and contains essential nutrients (Adnan et al., 2018; Moazzami et al., 2017; Silva et al., 2013). After 24 hr of culture in HBSS, 94% of the cells remained viable. However, HBSS is primarily used in laboratories and is expensive and not easily accessible for everyday use (Poi et al., 2013).
Apart from HBSS, milk is a commonly recommended storage medium due to its low cost, wide availability, and abundance of essential nutrients and growth factors (Adnan et al., 2018; Harkacz et al., 1997; Saluja & Anegundi, 2016; Sigalas et al., 2004; Silva et al., 2013). However, some people have allergies to milk proteins and lactose intolerance. In this case, soy milk is a better alternative (Moazami et al., 2012; Sethi et al., 2016). Soy milk, an aqueous extract of soybeans rich in proteins, amino acids, vitamins, and minerals, has been found to provide a suitable environment for cell growth and maintenance of cellular activity (Moura et al., 2012; Onuegbu et al., 2011; Sharma et al., 2023; Silva et al., 2013). The osmotic pressure of soy milk is 261 mOsmol/kg, and its pH is 7.2 (Côvre et al., 2021). Unlike milk, soy milk is cholesterol- and lactose-free and has a very low saturated fatty acid content (Moazami et al., 2012; Silva et al., 2013). Studies evaluating the effectiveness of replanting avulsed teeth showed no statistical difference between soy milk, HBSS, and whole milk. All three resulted in mild or moderate inflammatory cell presence, limited to the lamina propria inside the epithelium (Côvre et al., 2021; Moura et al., 2012; Silva et al., 2013).
In addition to providing suitable osmotic pressure and an adequate pH environment, soy milk is rich in antioxidants, such as isoflavones. These antioxidants appear to positively affect cell growth and proliferation (Moazami et al., 2012; Reis et al., 2017). Research has shown that storing avulsed teeth in a medium containing one or more antioxidants can improve the success rate of replantation (Buttke & Trope, 2003). Oxidative damage can adversely affect PDL cells and promote root surface resorption after replantation. In the electron transport chain process, oxygen may combine with an electron to produce superoxide anion (O2•-). O2•- can then generate hydrogen peroxide (H2O2) under the action of superoxide dismutase (SOD), which is then reduced to water and oxygen by catalase (CAT). When an avulsed tooth is exposed to air, a large amount of oxygen competes for the electrons, leading to increased production of O2•- and an excess of H2O2, causing oxidative damage. However, when the avulsed tooth is placed in soy milk, the isoflavones can directly bind to O2•-, reducing the generation of H2O2 and minimizing oxidative damage (Buttke & Trope, 2003; Kim, 2021; Reis et al., 2017) (Figure 5).
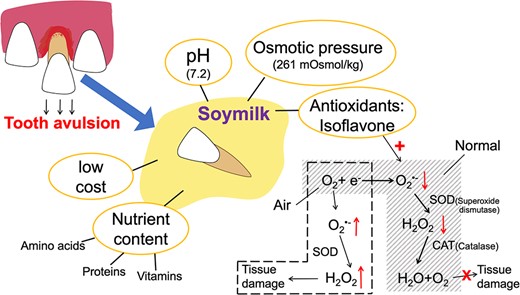
Benefits of soy milk as a storage medium for avulsed teeth. Soymilk, as a storage medium, can provide a good living environment for cells. Isoflavones, which act as antioxidants, bind directly to superoxide anions radical (O2•-), reducing tissue damage caused by hydrogen peroxide (H2O2). SOD = superoxide dismutase; CAT = catalase.
Conclusion
This review provides a summary of the effects and mechanisms of soybean and its derivatives on oral diseases. Soybeans, being a common source of protein, have been found to have a positive effect on the treatment of oral diseases. The review demonstrates that soybeans and their derivatives have numerous positive effects on the prevention and treatment of various oral diseases. For instance, soybean products such as ASU and tempeh and soybean derivatives represented by functional peptides, isoflavone, and BBI have been proven to have antibacterial, antioxidant, and anticancer effects in oral diseases. In other words, soybeans, as a functional food, could be applied to the human body safely and efficiently and contribute to maintaining health nutritionally, physiologically, and biochemically. They might be an ideal food for preventing infectious oral major diseases such as dental caries, periodontal disease, and the onset of oral cancer. Specifically, soybeans-related items could be safe alternatives, such as mouthwash and tooth storage solutions. Moreover, they might be the key to solving the problem of antibiotic-resistant bacteria due to the overuse of antibiotics.
However, further research is necessary to determine the specific concentrations and effective doses of this natural product in order to fully leverage its beneficial properties.
Data availability
The data that support the findings of this study are available from the corresponding author upon reasonable request.
Author contributions
Canyan Kuang (Visualization [equal], Writing—original draft [equal]), Zulema Arias (Conceptualization [equal], Methodology [equal]), Kazuhiro Omori (Supervision [equal], Writing—review & editing [equal]), Tadashi Yamamoto (Supervision [equal]), Yuki Shinoda-Ito (Visualization [equal], Writing—review & editing [equal]), and Shogo Takashiba (Project administration [equal], Supervision [equal], Writing—review & editing [equal]).
Funding
No funding sources are associated with this paper.
Conflicts of interest
There are no conflicts to declare.
Acknowledgements
The authors thank Tasuku Ito for the illustration of the figures.