-
PDF
- Split View
-
Views
-
Cite
Cite
Priyanka Hugar, Prasanna D Belur, Keyur Raval, Fermentative extraction of phenolic compounds from unripe areca nuts by solid-state fermentation using newly isolated Rhizopus orizae MW538932, International Journal of Food Science and Technology, Volume 58, Issue 9, September 2023, Pages 4738–4751, https://doi.org/10.1111/ijfs.16579
- Share Icon Share
Abstract
Areca nut (pericarp of Areca catechu L.) is a rich source of valuable phenolic compounds. Presence of a psychoactive agent, Arecoline, pose a challenge in the use of areca nut extracts. With an aim to maximise the extraction of phenolic compounds, a newly isolated Rhizopus orizae MW538932, was employed for the solid-state fermentation of unripe areca nut (6–7 months' maturity) powder. Supplementary nutrients (carbon and nitrogen sources) for the media and the solvents for the extraction of phenolic compounds from the fermented medium were optimised. The optimised process could produce an extract having a total phenolic content of 186.03 ± 2.50 mg gallic acid equivalent and total flavonoid content of 139.70 ± 2.00 mg catechin equivalent per gram of the sample. UHPLC–MS/MS studies and HPLC analysis showed the presence of plethora of phenolic compounds and the absence of Arecoline and other alkaloids. This flavonoid-rich extract can be a potential source of natural antioxidants for food and pharmaceutical industry.
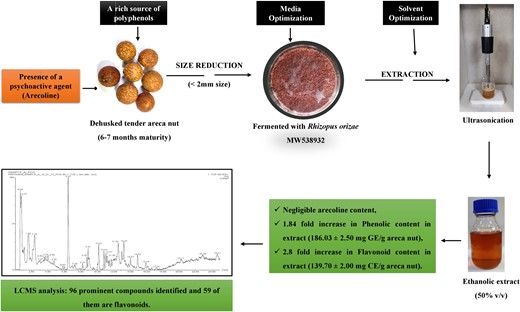
Introduction
Antioxidants have gained immense attention in the research community in the past few years due to lifestyle changes of people and emerging trends in dietary supplements and cosmetic industries. Antioxidants are substances capable of delaying, retarding, or preventing oxidation processes. The lack of adequate quantity of antioxidants in the body is believed to cause many ailments such as cardiovascular diseases, diabetes, cataracts, rheumatoid arthritis, and many more in humans including Alzheimer's and Parkinson's diseases (Mishra & Singh Bisht, 2011). Antioxidants are used as food additives, dietary supplements, and in nutraceutical, pharmaceutical, and cosmetic industries. Increasing consumers' health consciousness and preference for natural ingredients has encouraged the current research to lean toward natural alternatives in these commercial sectors. Phenolic compounds are the most important and widely available natural antioxidants that can replace synthetic antioxidants and consequently help to reduce harmful and carcinogenic effects of free radicals (Selamoglu, 2017). Areca nut seeds (pericarp of Areca catechu L.) are a rich source of natural antioxidants due to the abundance of phenolic acids, tannins, and flavonoids (Wang et al., 2021). Areca nut has been traditionally used in the ancient Indian system of Ayurveda and Chinese medical practices for centuries (Salehi et al., 2020). It has several beneficial physiological effects on the nervous, cardiovascular, and digestive systems (Peng et al., 2015). The WHO has listed as many as twenty-five beneficial properties of the areca nut. Although the isolation, purification, and identification of phytochemicals present in areca nut are extensively studied (Choe & Min, 2009), there are no reports on the degradation of areca nut or fermentative extraction of phenolic antioxidants from areca nut. The plausible reason is their components, such as condensed tannins, which are not readily hydrolysable.
The major chemical constituents found in areca nut are phenolic acids, condensed tannins, flavonoids, sterols, alkaloids, fatty acids, etc. (Peng et al., 2015). The tannin content in tender nuts is higher (38%–41% by weight) than in ripe nuts (16%–22% by weight). In comparison, alkaloids like arecoline are higher in ripe areca nuts (0.2%–0.3% by weight) compared to tender nuts (0.05%–0.1% by weight) (Hore & Anitha, 2015). Condensed tannins (arecatannins and procyanidins) present in areca nut impart astringency and bitterness perception to the consumer (Ma et al., 2014). Procyanidins are found to prevent, manage, or improve several clinical conditions like cancer, osteoporosis, neurodegenerative disorders, cardiovascular diseases, and diabetes mellitus (Satish & Kumaraswamy, 2008; Jaiswal et al., 2011). Among the four prominent alkaloids present in areca nut (arecoline, arecaidine, guavacoline, and guavacine), arecoline is found to be the major psychoactive agent and is believed to possess genotoxic and carcinogenic effects in humans (Dasgupta et al., 2006; Papke et al., 2015).
Several extraction methods including maceration, soxhlet extraction, supercritical fluid extraction, ultrasound, and microwave-assisted extraction often fail to extract the antioxidants efficiently due to the fact that most of the phenolic compounds are bound to the cell wall of the cellulosic matrix of plant material (Ignat et al., 2011). Degradation of extracted phenolics is unavoidable in the case of acid or base hydrolysis, although the complete release of bound phenolics is achieved. Enzymatic extraction could be prohibitively costly due to the requirement of a cocktail of enzymes for each substrate. Hence, the currently used methods for extracting polyphenols are expensive, aggressive, or highly contaminated with meagre yields (Aguilera-Carbo et al., 2009). Under these circumstances, a fermentative extraction is a promising approach for extracting polyphenolic compounds from plant-based sources. Fermentative extraction of valuable phenolics from wheat (Dey & Kuhad, 2014), rice bran (Schmidt et al., 2014), and finger millet (Yadav et al., 2013) using Rhizopus orizae has been reported.
The current study is aimed to extract valuable phenolic compounds from the pericarp of unripe areca nuts through solid-state fermentation. Two cultures isolated from the decomposing areca nut extract (Chogaru) were screened, and the selected one was taken for solid-state fermentation. An effort was made to identify the polyphenolic compounds present primarily in the areca nut and that are being hydrolysed by the isolate. The fermentation process and the subsequent extraction of phenolic compounds using solvents were optimised to maximise their yield.
Materials and methods
Shake flask studies
Previously isolated and characterised isolates, Geotrichum cucujoidarum MW538971 and Rhizopus orizae MW538932, were sub-cultured and maintained on potato dextrose agar (Thangavelu et al., 2022). The inoculum for Rhizopus orizae MW538932 was prepared from freshly prepared PDA culture plates by suspending the fungal spores in sterile distilled water and adjusted to a concentration of 8 × 106 spores mL−1. Erlenmeyer flask (250 mL) with 50 mL medium containing (w/v); 30% areca extract (Chogaru) and 2.4% potato dextrose broth was prepared. The flasks were steam sterilised, inoculated with Geotrichum cucujoidarum MW538971, and Rhizopus orizae MW538932, incubated at 25 °C at 120 rpm for 5–6 days, and the growth was monitored every 12 h.
The mould, Rhizopus orizae MW538932, was checked for its ability to utilise protocatechuic acid (PCA) and commercial procyanidin (Arg 9, Nouveau India). Erlenmeyer flask with 50 mL synthetic medium containing (w/v); PCA – 0.2%, dextrose – 2%, and ammonium nitrate – 2% was prepared. The ability of mould to utilise procyanidin was assessed using a medium having (w/v); 0.15% procyanidin (Arg 9, Nouveau India), and 2.4% potato dextrose broth. A control was included containing (w/v); dextrose – 2%, and ammonium nitrate – 2%. The flasks were sterilised at 121 °C for 15 min. The sterile media was then inoculated with the mould and incubated at 25 °C at 120 rpm for 5–6 days, and the growth was monitored every 12 h. The fermentation broth was first filtered with Whatman No. 1 filter paper and then centrifuged at 8120× g, 4 °C for 15 min, to separate the biomass. The biomass was washed with 0.9% NaCl at 2030× g for 5 min, 2–3 times. The filtrate (supernatant) and the biomass (cell suspension) were stored at 4 °C. The dry weight of the biomass was estimated as per the reported protocol (Leite et al., 2003).
Optimisation of media for solid-state fermentation
The media was optimised to enhance the recovery of total phenolic contents (TPCs) using the full factorial search approach. Three carbon sources (glucose, sucrose, and starch) and three nitrogen sources (ammonium sulfate, yeast extract, and urea) were chosen for the study. Unripe areca nuts of 6–7 months' maturity were collected from the farmers of Shivamogga district of Karnataka state, India, during December 2022 and were stored at −20 ° C deep freezer after removing the outer green husk. Before being taken for experiments, stored areca nut kernels were cut and powdered in a domestic blender. 10 g of crushed areca was sieved through 2 mm mesh size and added to 250 mL Erlenmeyer flasks. Crushed areca nut acts as physical support and nutrient source for the growth of microorganisms. As explained above, carbon (1.0 g) and nitrogen (0.2 g) sources were added to the flasks. A total volume of 4 mL of distilled water was added to each flask. All the contents in the flask were mixed, and the areca nut pieces were settled evenly in the flasks. Later, the flasks were autoclaved, inoculated, and incubated at room temperature. The growth was monitored for 12 days, and sterilised water of 2 mL was added after the 2nd and 7th day to maintain the moisture and avoid drying solid media. Samples were drawn under sterile conditions on 6th, 9th, and 12th day of incubation. Approximately 0.5 g of solid media was drawn under aseptic conditions in 50 mL beakers. For every 0.25 g of solid media, 5 mL of 50% (v/v) ethanol was added for extracting the phenolic compounds (Yadav et al., 2013). The samples were sonicated for 20 min at 65% amplitude with a power output of 130 W and 20 kHz frequency using a probe type ultrasonicator (Sonics Vibra cell, India) and centrifuged at 2862× g for 15 min. Low temperature of the sample was ensured by keeping the sample holder in an ice batch during sonication. The supernatant was collected, transferred to 100 mL beakers, and evaporated in a vacuum oven at 70 °C (Cai et al., 2014). After complete evaporation (15–20 h), the total phenolic and flavonoid contents were analysed.
After a combination was optimised, 1.5× and 2.0× strength of the same combination of the medium was taken in a new set of trials to assess the TPC and total flavonoid content (TFC) values of the extract. All the static flask trials were conducted in triplicates, and the mean values were reported with standard deviation.
Selection of solvent for extraction of phenolic compounds
Many factors affect the extraction of phenolic compounds, such as solvents, aqueous-solvent mixtures, extraction temperature, extraction time, and sample-to-solvent ratio (Dey & Kuhad, 2014). In the current study, the effect of solvents and their concentrations on the TPC and TFC extraction efficiency was studied. For all the experiments, 0.25 g of the test sample was mixed with 5 mL of the desired solvent and sonicated for 20 min at 4 °C before separating the extract. Water, methanol, ethanol, ethyl acetate, and acetone were taken for the study. The optimised medium comprising 10 g of 2 mm mesh size areca nut powder mixed with 1.5 g of sucrose and 0.3 g of ammonium sulfate was taken for the study. Samples drawn on the 6th day of incubation were used for the analysis.
UHPLC–MS/MS studies
The supernatants of fermented areca nut extract and the unfermented areca nut extract were analysed with HPLC–MS/MS facility at Division of Biological Sciences in the Indian Institute of Science, Bengaluru. The LC–MS used was with the Impact HD (Bruker) QTOF (Quadrupole Time-of-flight), equipped with an ESI (Electrospray Ionisation) source mass spectrometer attached to a micro-LC equipped hypersil gold (C18) 4.6 mm inner diameter, 150 mm length and 3 μm particle size. The metabolites were separated using a 40-min LC method with a column temperature of 22 °C. The solvent A was MilliQ water and solvent B was 0.1% formic acid in acetonitrile (Loba, India) and the LC method was as followed; t = 0–3.5 min, 10% B, flowrate 0.5 mL min−1; t = 15, 75% B, flowrate 0.5 mL min; t = 25, 95% B, flowrate 0.5 mL min−1; t = 26–39, 10% B, flowrate 1 mL min−1; t = 40, 10% B, flowrate 0.5 mL min−1. The data acquisition was carried out with the IDA method (Information dependent acquisition) in low mass mode within the m/z range of 50–1500 Da. The IDA method was built using the EMS (enhanced mass spectra) to EPI (enhanced product ion) modes. The data was acquired in negative polarity with capillary voltage −3000 V at 325 nm, and the adduct was [M−H]−. The data was acquired in triplicates. CompassXtract software version 3.2.200 (provided by Bruker Daltonik GmbH) was used to study, analyse, interpret and generate chromatograms of the obtained data. The unknown molecules were identified using an online search engine, MetFrag, based on their fragmentation pattern in the MS/MS data. MetFrag score indicates the similarity between the candidate and the given MS/MS spectrum.
The extract of the unfermented and fermented unripe areca nut extract was analysed using UHPLC–MS/MS available in the Central Research Facility of NITK Surathkal, India. The UPLC-MS/MS data was generated with Xevo G2-XS QToF mass spectrometer, (Waters, USA) was coupled with Acquity UPLC H-Class PLUS Bio System, BEH C18 column (50 mm length, 2.1 mm dia, and 1.7 μm particle size) with an ESI (electron spray ionisation) integrated IDA method. The separation was carried out using a 25 min UPLC method. Solvent A was 0.1% formic acid in MilliQ water, and solvent B was 0.1% formic acid in 90% acetonitrile (Loba India). The LC method was adapted from Song et al. (2022), with some modifications and they were as follows; t = 0–0.41 min, 10% B; t = 3 min, 30% B; t = 5 min, 40% B, t = 6 min, 45% B; t = 7 min, 50% B; t = 8 min, 65% B; t = 9 min, 85% B; t = 10–15 min, 90% B; t = 20–25 min, 95% B with the following conditions: sample volume – 1.5 μL, flowrate – 0.198 mL min−1, column temperature – 30 °C, cell temperature – 15 °C, wavelength – 280 nm, initial condition – 10% B, and m/z range – 100–1000 Da. The mass spectrum data were acquired in positive and negative polarities at 4500 and −4500 V, respectively, with a probe temperature of 120 °C. The compound parameters were set at a capillary voltage (CV) of 3 kV and collision energy (CE) of 6–45 eV. The simultaneous mass correction was done by injecting leucine enkephalin standard. The analysis of the sample was made by studying both the positive and negative ion mode over the range of 100–1000 m/z.
The waters UNIFI software was used to acquire, analyse, and manage experimental data. The accurate mass screening of the metabolites was performed by UNIFI software provided by waters and uses web-based database management (WDM) tool to perform presumptive identification. The raw data files of UPLC-MS/MS were directly uploaded to UNIFI software for analysis. The feature detection of candidate mass was performed using an elucidation toolset as provided by the WDM tool with a tolerance of 0.5 ppm and absolute m/z error of 0.1 Dalton. Adducts that were used for the positive mode was [M+H]+ while, for the negative mode, [M−H]− was used.
Estimation of total phenolic content
A modified Folin–Ciocalteu method was used to estimate the TPC of the samples. The absorbance was measured at 760 nm in a spectrophotometer (Lab India ultraviolet [UV] 3000þ) against blank (Dewanto et al., 2002). A standard curve for gallic acid (Sigma-Aldrich, India) ranging from 10 to 125 μg mL−1 was obtained using different solvents like water, ethanol, acetone, and methanol. The standard regression equation obtained for water was Y = 0.0047X + 0.079 (R2 = 0.9991); for ethanol was Y = 0.0039X + 0.0267 (R2 = 0.9994); for acetone was Y = 0.0037X + 0.3071 (R2 = 0.9953); and for methanol was Y = 0.0046X + 0.0785 (R2 = 0.9983). The results were expressed in mg gallic acid equivalent/gram areca nut (mg GE/g areca nut).
where con. refers to the value obtained from the gallic acid standard curve. All the measurements were made in triplicates, and the mean values were reported with standard deviation.
Estimation of total flavonoid content
The TFC of the samples was determined using a spectrophotometric method (Sari et al., 2020). The absorbance was measured at 510 nm in a spectrophotometer (Lab India ultraviolet [UV] 3000þ) against Millipore water. A standard curve for catechin (Sigma-Aldrich, India) ranging from 10 to 200 μg mL−1 was obtained using different solvents like water, ethanol, acetone, and methanol. The standard regression equation obtained for water was Y = 0.003X + 0.0688 (R2 = 0.9999); for ethanol was Y = 0.0026X + 0.0083 (R2 = 0.9987); for acetone was Y = 0.0035X + 0.034 (R2 = 0.9984); and for methanol was Y = 0.0026X + 0.0557 (R2 = 0.9986). The results were expressed in mg catechin equivalent/gram areca nut (mg CE/g areca nut).
where con. refers to concentration from the catechin standard curve equation. All the measurements were made in triplicates, and the mean values were reported with standard deviation.
Estimation of arecoline content
Arecoline content was estimated with Shimadzu HPLC (Shimadzu Corporation, Japan) with photodiode array (PDA) detector coupled with Shimadzu Reverse-phase C18 column (4.6 inner diameter × 250 mm length, 5 μm particle size). The separation was carried out for 15 min using an isocratic mobile phase containing 50% acetonitrile (Loba India) and 50% 0.01 M disodium hydrogen phosphate in milliQ water of pH 7.8 adjusted using formic acid. The flowrate was 0.8 mL min−1, sample volume was 20 μL, column temperature was 30 °C, cell temperature was 35 °C, and wavelength was 215 nm (Sari et al., 2020). For the preparation of the standard curve, arecoline hydrobromide was used. The standard was prepared in ethanol at various concentrations ranging from 10 to 100 μg mL−1. The ethanolic extracts of fermented and unfermented samples were diluted at appropriate concentrations and analysed to obtain the HPLC chromatograms.
Statistical analysis
All the samples were analysed in triplicates and their means were reported by Origin pro (2022) software. The data of individual variables was tested using analysis of variance (ANOVA) and the means were compared with Tukey's test. A significance level of P < 0.05 was used to measure the results. The statistical significance is specified by appropriate letters within the figures and tables.
Results and discussions
Shake flask studies
Both the isolates (Geotrichum cucujoidarum MW538971 and Rhizopus orizae MW538932) which were being studied here were isolated earlier in our lab from the microbial mat growing on the one-year-old stored extract of areca nut (called Chogaru in Kannada) produced during the production of Kempadike (also known as Kalipak) (Thangavelu et al., 2022). Kempadike is one variety of areca nut produced by boiling unripe areca nut (6–7 months maturity) and repeated coating of chogaru and subsequent sun drying. Chogaru (also known as Kali) is the concentrated extract produced after prolonged boiling of the extract obtained from the previous batches of areca nut processing (Hore & Anitha, 2015). Normally, Chogaru is a deep red coloured liquid, concentrated with phenolic compounds of unripe areca nut. The unripe areca nut contains more than fifty-nine prominent compounds, including alkaloids, polyphenols, phenolic acids, and flavonoids (Peng et al., 2015). Due to the high concentration of phenolic compounds and very low concentration of carbohydrates, microbial growth in Chogaru is limited. Isolation of only two pure cultures (Geotrichum cucujoidarum MW538971 and Rhizopus orizae MW538932) from the air-exposed 1-year-old Chogaru proved the ecological niche of the system. Those two cultures were screened for their ability to utilise the Chogaru in shake flask studies. The mould exhibited higher biomass growth for a prolonged period than the yeast (Data not shown) in the medium-containing Chogaru. After fermentation, the supernatants of fermented medium and the unfermented medium were subjected to LC–MS/MS analysis in negative ion mode. The LC–MS/MS spectra obtained (Figures S1 and S2) were analysed to identify the compounds which were present and were utilised. Most prominent compounds which were missing in the fermented medium with mould were found to be corresponding to the m/z of 577 and m/z of 865, and the former had the highest intensity followed by the latter (Table 1). The parent mass (observed m/z [M−H]−) and the fragmentation mass spectrum (product mass) of m/z 865 and m/z 577 acquired at 46 and 37.3 eV were submitted to MetFrag, applying KEGG as the chosen database with a mass accuracy of 20 ppm for further identification. MetFrag score indicates the similarity between the candidate and the given MS/MS spectrum, and the highest score was one. The [M−H]− ion with m/z 865 and m/z 577 was identified as Arecatannin B1 (Singh et al., 2018) and Procyanidin B4/B5 (Yuzuak et al., 2018), respectively, with a final score of one (Table S1). Arecatannin B1 is a trimeric procyanidin with epicatechin-(4β → 8)-epicatechin-(4β → 6)-catechin (Fig. 1a). Procyanidin B4 (MW-578.52) and Procyanidin B5 (MW-578.52) are dimers of catechin-(4β → 8)-epicatechin and epicatechin-(4β → 6)-epicatechin (Fig. 1b and c), respectively. In the medium fermented by the yeast, the intensity of procyanidin decreased by 71.13% and arecatannin by 54.24%. On the contrary to the yeast, there were no traces of m/z 865 and m/z 577 ions in the LC–MS/MS spectra of medium fermented by the mould, indicating the complete utilisation/degradation of arecatannins and procyanidin B during fermentation (Table 1). This observation is in agreement with the report of Rhizopus oryzae MUCL 28168 metabolising condensed tannins (procyanidin trimer) present in coffee pulp in a 192 h solid-state fermentation (Londoño-Hernandez et al., 2020). Complete utilisation/degradation of arecatannins and procyanidin B by Rhizopus orizae MW538932 in this study proves the fact that it has a remarkable ability to decompose and consume phenolic compounds present in the areca nut extract (Chogaru).
Prominent phenolic compounds found in fermented and unfermented Chogaru in respective negative ion LC–MS/MS spectra
Name . | Calculated data . | Unfermented Chogaru . | Chogaru fermented by yeast . | Chogaru fermented by mould . | ||||
---|---|---|---|---|---|---|---|---|
Molecular weight . | m/z [M−H]− . | Observed m/z [M−H]− . | Intensity . | Observed m/z [M−H]− . | Intensity . | Observed m/z [M−H]− . | Intensity . | |
Procyanidin B | 578.52 | 577.52 | 577.13 | 12880 | 577.13 | 3718 | – | – |
Arecatannin B1 | 866.77 | 865.77 | 865.20 | 8418 | 865.19 | 3852 | – | – |
Name . | Calculated data . | Unfermented Chogaru . | Chogaru fermented by yeast . | Chogaru fermented by mould . | ||||
---|---|---|---|---|---|---|---|---|
Molecular weight . | m/z [M−H]− . | Observed m/z [M−H]− . | Intensity . | Observed m/z [M−H]− . | Intensity . | Observed m/z [M−H]− . | Intensity . | |
Procyanidin B | 578.52 | 577.52 | 577.13 | 12880 | 577.13 | 3718 | – | – |
Arecatannin B1 | 866.77 | 865.77 | 865.20 | 8418 | 865.19 | 3852 | – | – |
Prominent phenolic compounds found in fermented and unfermented Chogaru in respective negative ion LC–MS/MS spectra
Name . | Calculated data . | Unfermented Chogaru . | Chogaru fermented by yeast . | Chogaru fermented by mould . | ||||
---|---|---|---|---|---|---|---|---|
Molecular weight . | m/z [M−H]− . | Observed m/z [M−H]− . | Intensity . | Observed m/z [M−H]− . | Intensity . | Observed m/z [M−H]− . | Intensity . | |
Procyanidin B | 578.52 | 577.52 | 577.13 | 12880 | 577.13 | 3718 | – | – |
Arecatannin B1 | 866.77 | 865.77 | 865.20 | 8418 | 865.19 | 3852 | – | – |
Name . | Calculated data . | Unfermented Chogaru . | Chogaru fermented by yeast . | Chogaru fermented by mould . | ||||
---|---|---|---|---|---|---|---|---|
Molecular weight . | m/z [M−H]− . | Observed m/z [M−H]− . | Intensity . | Observed m/z [M−H]− . | Intensity . | Observed m/z [M−H]− . | Intensity . | |
Procyanidin B | 578.52 | 577.52 | 577.13 | 12880 | 577.13 | 3718 | – | – |
Arecatannin B1 | 866.77 | 865.77 | 865.20 | 8418 | 865.19 | 3852 | – | – |
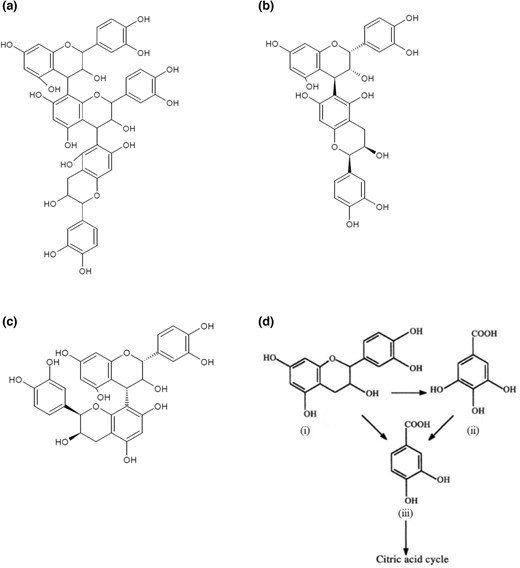
Structures of (a) Arecatannin B1, (b) Procyanidin B5, (c) Procyanidin B4, (d) the degradation of catechin to PCA and PGCA, where (i) catechin, (ii) phloroglucinol carboxylic acid and (iii) protocatechuic acid (adapted from Arunachalam et al., 2003a).
To understand the ability of Rhizopus orizae MW538932 to utilise procyanidin and protocatechuic acid (PCA), shake flask studies were conducted along with a control. Biomass growth was higher over an extended period in the procyanidin medium compared to control and PCA (Table 2). The biomass growth in the flask containing PCA was higher than the control and lower than the procyanidin, indicating its ability to utilise PCA for its growth.
The biomass (dry weight basis) obtained in 50 mL culture in procyanidin, catechin, and control media
Name . | Biomass (dry weight, g) . |
---|---|
Control | 0.18 ± 0.02a |
Procyanidin | 1.69 ± 0.07b |
Catechin | 0.20 ± 0.02a |
PCA | 0.80 ± 0.02c |
Name . | Biomass (dry weight, g) . |
---|---|
Control | 0.18 ± 0.02a |
Procyanidin | 1.69 ± 0.07b |
Catechin | 0.20 ± 0.02a |
PCA | 0.80 ± 0.02c |
a,b,cMeans with different superscript letters are significantly different within a column at P < 0.05.
The biomass (dry weight basis) obtained in 50 mL culture in procyanidin, catechin, and control media
Name . | Biomass (dry weight, g) . |
---|---|
Control | 0.18 ± 0.02a |
Procyanidin | 1.69 ± 0.07b |
Catechin | 0.20 ± 0.02a |
PCA | 0.80 ± 0.02c |
Name . | Biomass (dry weight, g) . |
---|---|
Control | 0.18 ± 0.02a |
Procyanidin | 1.69 ± 0.07b |
Catechin | 0.20 ± 0.02a |
PCA | 0.80 ± 0.02c |
a,b,cMeans with different superscript letters are significantly different within a column at P < 0.05.
From the initial shake flask studies with Chogaru and subsequent studies with procyanidin, and PCA media, it was clear that Rhizopus orizae MW538932 was metabolising procyanidins and arecatannins. In general, it was hypothesised that catechin and or gallic acid could be the intermediate products produced during the utilisation of these complex phenolic substances. The dimers, Procyanidin B4 and Procyanidin B5, are made up of catechin-(4β → 8)-epicatechin (Fig. 1c) and epicatechin-(4β → 6)-epicatechin (Fig. 1b), respectively. Upon hydrolysis, these dimers can be cleaved at their -(4β → 8)- and -(4β → 6)- bond, and as a result, (epi)catechins are released. Further, these molecules are converted to PCA (Protocatechuic acid) or PGCA (Phloroglucinol carboxylic acid) (Fig. 1d) Arecatannin B1 is a trimeric procyanidin with epicatechin-(4β → 8)-epicatechin-(4β → 6)-catechin (Fig. 1a). It can be cleaved at -(4β → 8)- and or -(4β → 6)- to leave behind one (epi)catechin molecule upon hydrolysis. As a result, a dimer is produced, which can be further cleaved into two molecules of (epi)catechin. Further (epi)catechin is converted to PCA (protocatechuic acid) or PGCA (phloroglucinol carboxylic acid). PCA is further consumed to form α-ketoadipate, which finally enters the TCA cycle. Many studies have shown such conversion of procyanidins to PCA and PGCA, which in turn get converted to α-ketoadipate and enter the TCA cycle (Muthukumar et al., 1982; Boominathan & Mahadevan, 1987; Hopper & Mahadevan, 1991; Arunachalam et al., 2003b). 2-(3′,4′-Dihydroxyphenyl) acetic acid and PCA were also found to be the product of (−)- epigallocatechin-3-gallate (EGCG) and (−)-epicatechin-3-gallate (ECG) metabolism in the large intestine of humans. It was proposed that gut microbes release free (−)-epigallocatechin (EGC) and (−)-epicatechin (EC) through esterases assisted cleavage of the gallic acid moiety in EGCG and ECG. Further EGC and EC are converted to 2-(3′,4′-dihydroxyphenyl) acetic acid and PCA through C-ring opening, A-ring fission, phenyl-γ-valerolactones (PVLs), and phenylvaleric acids (PVAs) formation and α- and β-oxidation (Wang et al., 2022).
Quite a few reports are available on the microbial degradation/utilisation of catechin/epicatechin, which are the monomers of procyanidins (Galiotou-Panayotou et al., 1988; Hopper & Mahadevan, 1991; Sambandam & Mahadevan, 1993; Arunachalam et al., 2003; Cruz-Hernández et al., 2005; Matsuda et al., 2008). However, reports on the degradation of dimeric or higher polymeric procyanidins by microorganisms are rather scarce (Nguz et al., 1994; Gamble et al., 1996; Vane et al., 2005). The condensed tannin (procyanidin and arecatannin) utilisation and degradation are poorly understood, and study on them is relatively scarce due to their structural complexity. From the current study, it is evident that Rhizopus orizae MW538932 consumes both. From the subsequent shake flask studies in the PCA medium, it is evident that the culture could utilise PCA for its growth. Thus, it can be hypothesised that the condensed tannins are being decomposed to catechin/epicatechin and subsequently converted to PCA and consumed. This is in concurrence with several reports published elsewhere (Hopper & Mahadevan, 1991; Arunachalam et al., 2003).
Optimisation of media for solid-state fermentation
Areca nut seeds (pericarp of Areca catechu) contain a large amount of phenolic acids, flavonoids, condensed tannins, and minor compounds, including sterols, fatty acids, alkaloids, and starch. All these compounds are packed in the lignocellulosic matrix in the pericarp of the areca nut. As most fungi possess the ability to degrade such lignocellulosic matrix through the release of hydrolytic enzymes and oxidative lignolytic enzymes (Sánchez, 2009), the fermentative extraction of valuable antioxidants from agricultural products using them is a promising strategy. Hence, Rhizopus orizae MW538932, which exhibited the ability to consume procyanidin and arecatannin, was used for fermentative extraction. Initially, 10 g of 2 mm mesh size crushed areca powder (6–7 months matured unripe areca nut) wetted with 4 mL of water was fermented in 250 mL Erlenmeyer flasks. There was limited growth even after a week, perhaps due to the lack of fermentable sugars and other nutrients. Hence supplementary carbon and nitrogen sources were added at a concentration of 1.0 and 0.2 g, respectively. Profuse growth and rapid fermentation were observed when the areca powder was supplemented with glucose and ammonium sulphate (data not shown). Further, three carbon sources (glucose, sucrose, and starch) and three nitrogen sources (ammonium sulphate, yeast extract, and urea) were chosen for the study. A full factorial search was performed to identify the best combination.
The TPC and TFC values of the powdered areca nut extract without any supplementary carbon and nitrogen sources were 101.79 ± 0.48 mg GE/g and 49.68 ± 0.29 CE/g areca nut, respectively. The TPC values were the least (19.40 ± 2.13 mg GE/g areca nut) in trial number 2 on day 12, with urea and glucose as the supplementary nutrients (Table 3). The TPC value for trial number 4 on day 9 was the highest (140.96 ± 0.72 mg GE/g areca nut) among all (Fig. 2). This trial had sucrose and ammonium sulphate as the supplementary carbon and nitrogen sources, respectively. TPC values were generally varying in each trial during the incubation period due to their different degree of release from the lignocellulosic matrix and further decomposition. The TFC values were the least (4.47 ± 1.76 mg CE/g areca nut) in trial number 2 on day 9, having urea and glucose as the supplementary nutrients. The TFC values for trial number 6 and 9 on day 9 was the highest (117.35 ± 1.92 mg CE/g areca nut) among all (Fig. 2). In most trials, TFC values were highest in the extracts on the 9th day compared to the 6th day and 12th day.
Total phenolic content (TPC) and total flavonoid content (TFC) of the extracts obtained in the trials where different supplementary carbon and nitrogen sources were used
Trial name . | Supplementary carbon and nitrogen sources . | 6th day . | 9th day . | 12th day . | |||
---|---|---|---|---|---|---|---|
TPC . | TFC . | TPC . | TFC . | TPC . | TFC . | ||
0 | – | 101.79 ± 0.48 | 49.68 ± 0.29 | 101.79 ± 0.48 | 49.68 ± 0.29 | 101.79 ± 0.48 | 49.68 ± 0.29 |
1 | Glucose – ammonium sulphate | 50.54 ± 1.72 | 28.91 ± 1.92 | 44.81 ± 1.08 | 33.80 ± 1.33 | 77.21 ± 2.95 | 29.12 ± 3.33 |
2 | Glucose – urea | 8.98 ± 0.36 | 7.36 ± 0.38 | 6.73 ± 0.36 | 4.47 ± 1.76 | 19.40 ± 2.13 | 5.13 ± 0 |
3 | Glucose – yeast extract | 74.81 ± 7.10 | 40.91 ± 1.39 | 71.69 ± 1.95 | 59.80 ± 4.81 | 76.90 ± 3.88 | 31.12 ± 1.15 |
4 | Sucrose – ammonium sulphate | 134.92 ± 3.55 | 68.69 ± 1.39 | 140.96 ± 0.72 | 110.46 ± 1.76 | 129.29 ± 3.48 | 40.67 ± 1.54 |
5 | Sucrose – urea | 86.06 ± 0.31 | 48.02 ± 1.54 | 108.88 ± 2.78 | 85.57 ± 1.54 | 79.08 ± 1.26 | 32.90 ± 4.44 |
6 | Sucrose – yeast extract | 57.63 ± 3.55 | 36.93 ± 1.05 | 141.17 ± 4.74 | 117.35 ± 1.92 | 77.21 ± 1.60 | 33.79 ± 2.31 |
7 | Starch – ammonium sulphate | 69.29 ± 0.36 | 28.69 ± 0.77 | 123.04 ± 5.32 | 82.91 ± 0.77 | 97.42 ± 0.79 | 34.23 ± 0.38 |
8 | Starch – urea | 48.46 ± 0.36 | 29.35 ± 0.77 | 56.79 ± 4.51 | 60.91 ± 4.29 | 57.63 ± 4.13 | 24.23 ± 0.38 |
9 | Starch – yeast extract | 74.50 ± 2.48 | 39.58 ± 0.77 | 130.02 ± 2.19 | 115.57 ± 1.02 | 90.85 ± 0.48 | 28.46 ± 0.67 |
10 | 1.5 strength sucrose-ammonium sulphate medium | 151.78 ± 1.61 | 76.83 ± 5.79 | 186.03 ± 2.50 | 139.70 ± 2.00 | n.d. | n.d. |
11 | 2.0 strength sucrose-ammonium sulphate medium | 136.87 ± 0.58 | 74.16 ± 3.67 | 153.03 ± 7.60 | 131.03 ± 4.16 | n.d. | n.d. |
Trial name . | Supplementary carbon and nitrogen sources . | 6th day . | 9th day . | 12th day . | |||
---|---|---|---|---|---|---|---|
TPC . | TFC . | TPC . | TFC . | TPC . | TFC . | ||
0 | – | 101.79 ± 0.48 | 49.68 ± 0.29 | 101.79 ± 0.48 | 49.68 ± 0.29 | 101.79 ± 0.48 | 49.68 ± 0.29 |
1 | Glucose – ammonium sulphate | 50.54 ± 1.72 | 28.91 ± 1.92 | 44.81 ± 1.08 | 33.80 ± 1.33 | 77.21 ± 2.95 | 29.12 ± 3.33 |
2 | Glucose – urea | 8.98 ± 0.36 | 7.36 ± 0.38 | 6.73 ± 0.36 | 4.47 ± 1.76 | 19.40 ± 2.13 | 5.13 ± 0 |
3 | Glucose – yeast extract | 74.81 ± 7.10 | 40.91 ± 1.39 | 71.69 ± 1.95 | 59.80 ± 4.81 | 76.90 ± 3.88 | 31.12 ± 1.15 |
4 | Sucrose – ammonium sulphate | 134.92 ± 3.55 | 68.69 ± 1.39 | 140.96 ± 0.72 | 110.46 ± 1.76 | 129.29 ± 3.48 | 40.67 ± 1.54 |
5 | Sucrose – urea | 86.06 ± 0.31 | 48.02 ± 1.54 | 108.88 ± 2.78 | 85.57 ± 1.54 | 79.08 ± 1.26 | 32.90 ± 4.44 |
6 | Sucrose – yeast extract | 57.63 ± 3.55 | 36.93 ± 1.05 | 141.17 ± 4.74 | 117.35 ± 1.92 | 77.21 ± 1.60 | 33.79 ± 2.31 |
7 | Starch – ammonium sulphate | 69.29 ± 0.36 | 28.69 ± 0.77 | 123.04 ± 5.32 | 82.91 ± 0.77 | 97.42 ± 0.79 | 34.23 ± 0.38 |
8 | Starch – urea | 48.46 ± 0.36 | 29.35 ± 0.77 | 56.79 ± 4.51 | 60.91 ± 4.29 | 57.63 ± 4.13 | 24.23 ± 0.38 |
9 | Starch – yeast extract | 74.50 ± 2.48 | 39.58 ± 0.77 | 130.02 ± 2.19 | 115.57 ± 1.02 | 90.85 ± 0.48 | 28.46 ± 0.67 |
10 | 1.5 strength sucrose-ammonium sulphate medium | 151.78 ± 1.61 | 76.83 ± 5.79 | 186.03 ± 2.50 | 139.70 ± 2.00 | n.d. | n.d. |
11 | 2.0 strength sucrose-ammonium sulphate medium | 136.87 ± 0.58 | 74.16 ± 3.67 | 153.03 ± 7.60 | 131.03 ± 4.16 | n.d. | n.d. |
Total phenolic content (TPC) and total flavonoid content (TFC) of the extracts obtained in the trials where different supplementary carbon and nitrogen sources were used
Trial name . | Supplementary carbon and nitrogen sources . | 6th day . | 9th day . | 12th day . | |||
---|---|---|---|---|---|---|---|
TPC . | TFC . | TPC . | TFC . | TPC . | TFC . | ||
0 | – | 101.79 ± 0.48 | 49.68 ± 0.29 | 101.79 ± 0.48 | 49.68 ± 0.29 | 101.79 ± 0.48 | 49.68 ± 0.29 |
1 | Glucose – ammonium sulphate | 50.54 ± 1.72 | 28.91 ± 1.92 | 44.81 ± 1.08 | 33.80 ± 1.33 | 77.21 ± 2.95 | 29.12 ± 3.33 |
2 | Glucose – urea | 8.98 ± 0.36 | 7.36 ± 0.38 | 6.73 ± 0.36 | 4.47 ± 1.76 | 19.40 ± 2.13 | 5.13 ± 0 |
3 | Glucose – yeast extract | 74.81 ± 7.10 | 40.91 ± 1.39 | 71.69 ± 1.95 | 59.80 ± 4.81 | 76.90 ± 3.88 | 31.12 ± 1.15 |
4 | Sucrose – ammonium sulphate | 134.92 ± 3.55 | 68.69 ± 1.39 | 140.96 ± 0.72 | 110.46 ± 1.76 | 129.29 ± 3.48 | 40.67 ± 1.54 |
5 | Sucrose – urea | 86.06 ± 0.31 | 48.02 ± 1.54 | 108.88 ± 2.78 | 85.57 ± 1.54 | 79.08 ± 1.26 | 32.90 ± 4.44 |
6 | Sucrose – yeast extract | 57.63 ± 3.55 | 36.93 ± 1.05 | 141.17 ± 4.74 | 117.35 ± 1.92 | 77.21 ± 1.60 | 33.79 ± 2.31 |
7 | Starch – ammonium sulphate | 69.29 ± 0.36 | 28.69 ± 0.77 | 123.04 ± 5.32 | 82.91 ± 0.77 | 97.42 ± 0.79 | 34.23 ± 0.38 |
8 | Starch – urea | 48.46 ± 0.36 | 29.35 ± 0.77 | 56.79 ± 4.51 | 60.91 ± 4.29 | 57.63 ± 4.13 | 24.23 ± 0.38 |
9 | Starch – yeast extract | 74.50 ± 2.48 | 39.58 ± 0.77 | 130.02 ± 2.19 | 115.57 ± 1.02 | 90.85 ± 0.48 | 28.46 ± 0.67 |
10 | 1.5 strength sucrose-ammonium sulphate medium | 151.78 ± 1.61 | 76.83 ± 5.79 | 186.03 ± 2.50 | 139.70 ± 2.00 | n.d. | n.d. |
11 | 2.0 strength sucrose-ammonium sulphate medium | 136.87 ± 0.58 | 74.16 ± 3.67 | 153.03 ± 7.60 | 131.03 ± 4.16 | n.d. | n.d. |
Trial name . | Supplementary carbon and nitrogen sources . | 6th day . | 9th day . | 12th day . | |||
---|---|---|---|---|---|---|---|
TPC . | TFC . | TPC . | TFC . | TPC . | TFC . | ||
0 | – | 101.79 ± 0.48 | 49.68 ± 0.29 | 101.79 ± 0.48 | 49.68 ± 0.29 | 101.79 ± 0.48 | 49.68 ± 0.29 |
1 | Glucose – ammonium sulphate | 50.54 ± 1.72 | 28.91 ± 1.92 | 44.81 ± 1.08 | 33.80 ± 1.33 | 77.21 ± 2.95 | 29.12 ± 3.33 |
2 | Glucose – urea | 8.98 ± 0.36 | 7.36 ± 0.38 | 6.73 ± 0.36 | 4.47 ± 1.76 | 19.40 ± 2.13 | 5.13 ± 0 |
3 | Glucose – yeast extract | 74.81 ± 7.10 | 40.91 ± 1.39 | 71.69 ± 1.95 | 59.80 ± 4.81 | 76.90 ± 3.88 | 31.12 ± 1.15 |
4 | Sucrose – ammonium sulphate | 134.92 ± 3.55 | 68.69 ± 1.39 | 140.96 ± 0.72 | 110.46 ± 1.76 | 129.29 ± 3.48 | 40.67 ± 1.54 |
5 | Sucrose – urea | 86.06 ± 0.31 | 48.02 ± 1.54 | 108.88 ± 2.78 | 85.57 ± 1.54 | 79.08 ± 1.26 | 32.90 ± 4.44 |
6 | Sucrose – yeast extract | 57.63 ± 3.55 | 36.93 ± 1.05 | 141.17 ± 4.74 | 117.35 ± 1.92 | 77.21 ± 1.60 | 33.79 ± 2.31 |
7 | Starch – ammonium sulphate | 69.29 ± 0.36 | 28.69 ± 0.77 | 123.04 ± 5.32 | 82.91 ± 0.77 | 97.42 ± 0.79 | 34.23 ± 0.38 |
8 | Starch – urea | 48.46 ± 0.36 | 29.35 ± 0.77 | 56.79 ± 4.51 | 60.91 ± 4.29 | 57.63 ± 4.13 | 24.23 ± 0.38 |
9 | Starch – yeast extract | 74.50 ± 2.48 | 39.58 ± 0.77 | 130.02 ± 2.19 | 115.57 ± 1.02 | 90.85 ± 0.48 | 28.46 ± 0.67 |
10 | 1.5 strength sucrose-ammonium sulphate medium | 151.78 ± 1.61 | 76.83 ± 5.79 | 186.03 ± 2.50 | 139.70 ± 2.00 | n.d. | n.d. |
11 | 2.0 strength sucrose-ammonium sulphate medium | 136.87 ± 0.58 | 74.16 ± 3.67 | 153.03 ± 7.60 | 131.03 ± 4.16 | n.d. | n.d. |
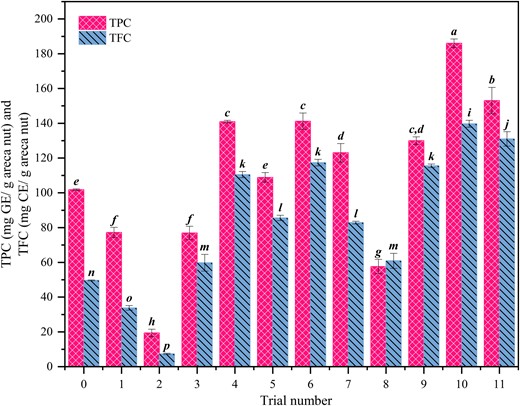
Effect of different supplementary carbon and nitrogen sources and their combinations in the areca nut media on the total phenolics and flavonoids extracted.
As the 9th-day extract of trial number 4 having sucrose and ammonium sulphate was giving the highest TPC values (140.96 ± 0.72 mg GE/g areca nut) and superior TFC values (110.46 ± 1.76 CE/g areca nut), one more set of experiments were conducted with 1.5 times and 2 times higher sucrose and ammonium sulphate in the medium. In both the trials, the TPC and TFC values were higher than the single-strength medium. The highest TPC & TFC values of 186.03 ± 2.50 mg GE/g areca nut and 139.70 ± 2.00 mg CE/g areca nut were recorded on the 9th day of an extract of trial no. 10, respectively. Trial No. 10 had 10 g of 2 mm mesh size areca nut powder supplemented with 1.5 g of sucrose and 0.3 g of ammonium sulfate.
There was a 1.82-fold improvement in the extraction of phenolic compounds due to solid-state fermentation. Similarly, there was a 2.81-fold increase in the extraction of total flavonoids. These results align with numerous reports of enhanced phenolics recovery by solid-state fermentation of agro residues by fungal isolates (Punia et al., 2021; Amaya-Chantaca et al., 2022; Arancibia-Díaz et al., 2023). There are quite a few reports available on the successful use of Rhizopus oryzae for the enhanced extraction of phenolics from rice bran (Schmidt et al., 2014), wheat (Dey & Kuhad, 2014), and finger millet (Yadav et al., 2013).
The unripe areca nut (6–7 months' maturity) contains various phenolic compounds, including condensed tannins (procyanidins and arecatannins), flavonoids (catechin/epicatechin, quercetin, epigallocatechin, luteolin, and isorhamnetin), hydroxybenzoic acids (protocatechuic acid, p-hydroxybenzoic acid, and syringic acid), and hydroxycinnamic acids (sinapic acid, ferulic acid, vanillic acid) (Peng et al., 2015). These compounds are held in a lignocellulosic matrix. During microbial fermentation, they are released due to the enzymatic digestion of this matrix. It is also expected that bigger molecules such as procyanidin and arecatannins are hydrolysed/cleaved to smaller molecules such as catechin/epicatechin and, subsequently, to protocatechuic acid. The release of these compounds and extensive degradation of bigger phenolic compounds starts when there is an exhaustion of one or more essential nutrients such as carbon, nitrogen, or phosphate source (Martins et al., 2011). In our studies, apparently there was a nutrient shortage from the 5th day onwards, due to which there was an increase in TPC & TFC values on the 6th day and onwards. Due to the release and production of smaller molecules, a rapid rise in TPC and TFC values in the 9th day samples was witnessed. However, from the earlier shake flask studies and the pertinent published reports, it is clear that Rhizopus orizae will start consuming the produced molecules (such as protocatechuic acid) due to the prevailing nutrient stress. This could be the reason for the decline in TPC and TFC values in the 12th day samples compared to the 6th day and 9th day samples.
Selection of solvent for extraction
As solvents play a crucial role in extracting the phenolic substances from the fermented medium, it was necessary to find the best solvent and its strength to extract the phenolics from the fermented medium. To begin with, water, methanol, ethanol, ethyl acetate, and acetone of 100% purity were screened for extracting the phenolic compounds from the unripe areca nut. Except ethyl acetate, all the solvents exhibited different degree of extraction. As ethyl acetate is a poor solvent for these phenolic compounds, turbid emulsion formed was creating interference in TPC and TFC analysis and hence it was discontinued for further studies. Further, water, methanol, acetone, and ethanol, having various strengths, were chosen for the study. 10 g of 2 mm mesh size crushed areca powder wetted with 4 mL of water, supplemented with 1.5 g sucrose, and 0.3 g of ammonium sulfate was taken for the study. The samples were taken on the 6th day of incubation for this study. Polar solvents such as water, methanol, acetone, and ethanol and the aqueous solutions of methanol, ethanol, and acetone were chosen for the extraction of TPC, TFC, and arecoline. As the solubility and polarity of solvents are critical parameters in the extraction of plant phenolics (Naczk & Shahidi, 2006), aqueous solutions of methanol, ethanol, and acetone were also tested.
As the profile of phenolics present in the unfermented medium and the fermented medium are different, the effect of solvents was tested in both samples and compared. There was a significant variability in the TPC and TFC values of extracts among the studied solvents (Table S2). In the case of unfermented samples, 70% (v/v) ethanol resulted in the maximum extraction of total phenolics (128.37 ± 0.76 mg GE/g areca nut). The results of 50% and 60% (v/v) ethanol solution were comparable (124.03 ± 1.89 and 121.53 ± 3.82 mg GE/g areca nut, respectively). Compared to 70% (v/v) ethanol, total phenolics extracted 75% for pure acetone, 61% for water, and 35% for pure methanol (45.87 ± 1.74 mg GE/g areca nut). In the case of total flavonoid extraction, 70% (v/v) ethanol fared better (92.83 ± 1.22 mg CE/g areca nut) than all the other solvent systems. Compared to 70% (v/v) ethanol, the extraction of total flavonoids was 75% for pure acetone, 45% for pure methanol, and 19% for water (18.13 ± 1.15 mg CE/g areca nut).
Among all the solvents tested for extraction of fermented samples, absolute alcohol fared better (Fig. 3a). Among the aqueous solutions of ethanol tested, 50% (v/v) ethanol resulted in the maximum extraction of total phenolics (169.38 ± 0.89 mg GE/g areca nut) (Fig. 3b). Among the aqueous solutions of acetone and methanol tested, 70% (v/v) of both found to be the best (Fig. 3c and d). However, compared to 50% (v/v) ethanol, total phenolics extracted was 60% for pure acetone, 31% for pure methanol, and 30% for water. For flavonoids extraction, 50% (v/v) ethanol fared better (113.09 ± 1.67 mg CE/g areca nut) than all the other solvent systems. Compared to 50% (v/v) ethanol, the extraction of total flavonoids was 61% for pure acetone, 16% for pure methanol, and 12% for water. Thus, 50% (v/v) ethanol was found to be the best solvent for the extraction of phenolics and flavonoids from the mould fermented medium.
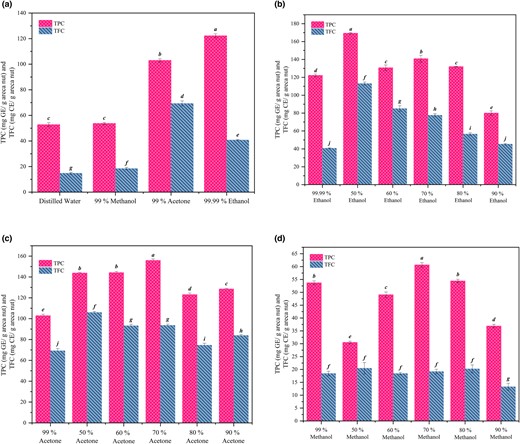
Total phenolics (expressed in terms of TPC & TFC) extracted from the fermented medium using (a) different solvents, (b) ethanol and its aqueous solutions, (c) acetone and its aqueous solutions, and (d) methanol and its aqueous solutions.
HPLC and UHPLC–MS/MS analysis of the extract
The HPLC analysis of the extract from the unfermented areca nut showed it had an arecoline content of 3.1 mg g−1 of areca nut. Whereas the extract obtained from the fermented areca nut (Trial No. 10) using 50% (v/v) ethanol had no traces of arecoline at all (Figure S3). Arecoline is found to be highly hydrophilic, and maximum extraction was achieved with methanol followed by ethanol (Chavan & Singhal, 2013). As the detectable limit of arecoline with this method was 1 μg mL−1, it can be concluded that the residual arecoline content in the extract was below the detectable limit. The reason for not getting arecoline in the extract was not due to the solvent used for extraction, as ethanol is one of the best solvents for arecoline extraction. As the solid media had undergone fermentation, it can be speculated that Rhizopus orizae MW538932 might have consumed/decomposed most of the arecoline during its growth in the solid medium.
The UHPLC–MS/MS analysis showed the presence of numerous phenolic acids, tannins, flavonoids, isoflavonoids, lignans, phenylproponoids, and quassinoid glucosides (Figure S4). The prominent compounds identified through UHPLC–MS/MS are listed in Table 4. Only those compounds having intensity more than 10 000 and those compounds identified with the mass accuracy of 10 mDA or less are listed in the table. Altogether, 73 compounds have been identified. Compounds normally found in areca nut such as tannins (procyanidins, arecatannins, and catechins) were present in the extract even after 9 days of fermentation, yet at low concentrations. Several flavonoids (Kaemferide, Engeletin, Myricetin, Kaempferitrin, Isorhamnetin, Hesperidin, Myricitrin, etc.) commonly found in unripe areca nuts were not detected in the extract (Song et al., 2022). Similarly, alkaloids such as arecoline, homoarecoline arecaidine, guvacine, and guvacoline which are normally found in areca nut were also not detected. Acuminatin (a furan lignan), Picrasinoside G (quassinoid glucosides), Magnolignan E (a lignan), Mulberrofuran P (a benzofuran flavonoid), (+)-Catechin, Procyanidin B4/B5, and 5,3′-Dihydroxy-6,7,4′-trimethoxyflavanone were the prominent compounds identified in the extract. Out of 73 compounds identified, about 33 compounds were flavonoids and their derivatives. It is evident that many of these molecules were produced by Rhizopus orizae MW538932 during the fermentation. Considering the fact that these molecules are present in many medicinal herbs which are proved to confer numerous health benefits, this alternate route of production makes this study interesting.
The major compounds identified from the ethanolic extract obtained by fermentative extraction of unripe areca nut according to UHPLC–MS/MS*
No. . | tR (min) . | Compound name . | Formula . | Calculated mass . | m/z . | Exact mass . | Mass accuracy mDa . | Base peaks (%) . |
---|---|---|---|---|---|---|---|---|
1 | 0.68 | Narcissin | C28H32O16 | 624.17 | 623.16 | 624.17 | 1.50 | 0.03 |
2 | 0.91 | Homoarecoline | C9H15NO2 | 169.11 | 170.12 | 169.11 | 0.10 | 0.33 |
3 | 0.94 | 6″-O-Acetylgenistin | C23H22O11 | 474.12 | 475.12 | 474.11 | −1.90 | 0.03 |
4 | 1.17 | Procyanidin B4/B5 | C30H26O12 | 578.14 | 579.15 | 578.14 | 1.10 | 1.09 |
5 | 1.56 | Arecatannin B1 | C45H38O18 | 866.21 | 867.21 | 866.20 | −1.00 | 0.59 |
6 | 1.73 | (+)-Catechin | C15H14O6 | 290.08 | 291.09 | 290.08 | −0.40 | 1.14 |
7 | 1.73 | 3-Hydroxybenzoic acid | C7H6O3 | 138.03 | 139.04 | 138.03 | 0.10 | 0.08 |
8 | 1.73 | 1-Hydroxy-3,7-dimethoxyxanthone | C15H12O5 | 272.07 | 273.07 | 272.07 | −1.10 | 0.03 |
9 | 1.74 | Plathymenin | C15H12O6 | 288.06 | 289.07 | 288.06 | −0.50 | 0.03 |
10 | 2.28 | Isorhamnetin | C16H12O7 | 316.06 | 315.05 | 316.06 | −1.40 | 0.01 |
11 | 2.94 | Nubigenol | C15H14O6 | 290.08 | 291.09 | 290.08 | 0.10 | 0.34 |
12 | 3.03 | 1,8-Dihydroxy-2,3,4,5-tetramethoxyxanthone | C17H16O8 | 348.08 | 349.09 | 348.08 | −1.10 | 0.01 |
13 | 3.34 | Baicalein | C15H10O5 | 270.05 | 271.06 | 270.05 | −1.00 | 0.01 |
14 | 3.70 | Eriodictyol | C15H12O6 | 288.06 | 287.06 | 288.06 | −0.50 | 0.02 |
15 | 4.46 | Astilbin | C21H22O11 | 450.12 | 451.12 | 450.12 | −0.90 | 0.01 |
16 | 4.59 | Andrographidine C | C23H24O10 | 460.14 | 461.14 | 460.14 | −0.60 | 0.01 |
17 | 4.65 | Complanatuside | C28H32O16 | 624.17 | 625.18 | 624.17 | −0.10 | 0.34 |
18 | 4.65 | Isorhamnetin-3-O-glucoside | C22H22O12 | 478.11 | 479.12 | 478.11 | −0.30 | 0.02 |
19 | 5.76 | Thymonin | C18H16O8 | 360.08 | 359.08 | 360.08 | −0.80 | 0.14 |
20 | 5.79 | Ombuin | C17H14O7 | 330.07 | 331.08 | 330.07 | −0.80 | 0.09 |
21 | 5.79 | Eupatolin | C23H24O12 | 492.13 | 493.13 | 492.13 | −0.40 | 0.02 |
22 | 5.96 | Quercetin | C15H10O7 | 302.04 | 303.05 | 302.04 | −1.10 | 0.03 |
23 | 6.55 | Vittariflavone | C24H26O12 | 506.14 | 505.13 | 506.14 | −2.20 | 0.08 |
24 | 6.64 | 1-Hydroxy-2,3,4,5-tetramethoxyxanthone | C17H16O7 | 332.09 | 333.10 | 332.09 | −0.60 | 0.06 |
25 | 6.85 | Saucernetin | C22H28O5 | 372.19 | 373.20 | 372.19 | 0.10 | 0.01 |
26 | 6.89 | Tenaxin I | C18H16O7 | 344.09 | 343.08 | 344.09 | −0.80 | 0.17 |
27 | 6.93 | 3-O-Methylquercetin | C16H12O7 | 316.06 | 317.07 | 316.06 | 0.00 | 0.20 |
28 | 7.10 | 5,6-Dihydroxy-7,3′,4′-trimethoxyflavone | C18H16O7 | 344.09 | 345.10 | 344.09 | −0.60 | 0.04 |
29 | 7.26 | Reineckiagenin | C27H44O5 | 448.32 | 449.33 | 448.32 | −0.70 | 0.01 |
30 | 7.60 | Kadsulignan F | C29H32O11 | 556.19 | 555.19 | 556.19 | −0.20 | 0.03 |
31 | 7.71 | Coniferyl alcohol | C10H12O3 | 180.08 | 181.09 | 180.08 | 0.70 | 0.05 |
32 | 8.26 | Grandoside | C17H32O11 | 412.19 | 411.19 | 412.19 | −1.60 | 0.01 |
33 | 8.48 | Magnograndiolide | C15H22O4 | 266.15 | 265.15 | 266.15 | 1.10 | 0.01 |
34 | 8.63 | 5,3′-Dihydroxy-6,7,4′-trimethoxyflavanone | C18H18O7 | 346.11 | 347.11 | 346.10 | −0.70 | 1.18 |
35 | 9.54 | Kadsulignan E | C31H30O11 | 578.18 | 579.18 | 578.18 | −2.80 | 0.01 |
36 | 10.18 | Kosamol A | C30H38O8 | 526.26 | 527.26 | 526.25 | −2.20 | 0.20 |
37 | 10.58 | Mulberrofuran P | C34H22O9 | 574.13 | 575.13 | 574.13 | 1.10 | 2.23 |
38 | 10.72 | Genistein | C15H10O5 | 270.05 | 271.06 | 270.05 | −0.50 | 0.14 |
39 | 10.74 | Glycyrrhizol B | C21H18O5 | 350.12 | 349.11 | 350.12 | 0.60 | 0.07 |
40 | 10.75 | Macluraxanthone | C23H22O6 | 394.14 | 393.14 | 394.14 | 0.70 | 0.05 |
41 | 10.76 | Nobiletin | C21H22O8 | 402.13 | 401.12 | 402.13 | −1.20 | 0.02 |
42 | 10.90 | Magnolignan E | C18H18O4 | 298.12 | 297.13 | 298.12 | −0.10 | 2.69 |
43 | 11.03 | Palmitic acid | C16H32O2 | 256.24 | 255.23 | 256.24 | −0.30 | 0.01 |
44 | 11.07 | Quercetin-3-arabinoside | C20H18O11 | 434.08 | 435.09 | 434.08 | −2.20 | 0.04 |
45 | 11.27 | Backuchiol | C18H24O | 256.18 | 257.19 | 256.18 | −0.50 | 0.01 |
46 | 11.31 | Picrasinoside G | C28H44O12 | 572.28 | 573.29 | 572.28 | 0.60 | 8.22 |
47 | 11.34 | Robinin | C33H40O19 | 740.22 | 739.21 | 740.21 | −2.50 | 0.02 |
48 | 11.53 | Tribuloside | C30H26O13 | 594.14 | 595.14 | 594.14 | −2.20 | 0.07 |
49 | 11.53 | Tectorigenin | C16H12O6 | 300.06 | 299.06 | 300.07 | 2.30 | 0.03 |
50 | 11.56 | Tomentolide A | C25H22O5 | 402.15 | 401.14 | 402.15 | 1.40 | 0.04 |
51 | 12.13 | Artemetin | C20H20O8 | 388.12 | 387.11 | 388.12 | 1.50 | 0.03 |
52 | 12.39 | Phanginin F | C21H28O6 | 376.19 | 375.18 | 376.19 | −0.50 | 0.04 |
53 | 12.50 | Stigmast-4-en-3-one | C29H48O | 412.37 | 411.36 | 412.37 | −5.00 | 0.15 |
54 | 12.52 | Picrasin F | C22H30O8 | 422.19 | 423.20 | 422.20 | 1.50 | 0.23 |
55 | 12.52 | Kushenol C | C25H26O7 | 438.17 | 437.16 | 438.17 | 1.40 | 0.07 |
56 | 12.69 | Glisoflavanone | C25H28O6 | 424.19 | 423.18 | 424.19 | 1.50 | 0.06 |
57 | 12.75 | Norkurarinol | C25H30O7 | 442.20 | 443.21 | 442.20 | −1.20 | 0.02 |
58 | 12.78 | Vitexin | C21H20O10 | 432.11 | 433.11 | 432.11 | 0.80 | 0.01 |
59 | 13.18 | Licuraside | C26H30O13 | 550.17 | 551.17 | 550.17 | −1.20 | 0.02 |
60 | 13.73 | Isokurarinone | C26H30O6 | 438.20 | 437.20 | 438.21 | 0.80 | 0.06 |
61 | 14.29 | Campesta-7,22E-dien-3beta-ol | C28H46O | 398.35 | 399.36 | 398.35 | −1.60 | 0.10 |
62 | 14.84 | Inosine | C10H12N4O5 | 268.08 | 267.07 | 268.08 | 0.00 | 0.08 |
63 | 15.14 | Moracin O | C19H18O5 | 326.12 | 325.11 | 326.11 | −0.50 | 0.02 |
64 | 16.20 | Daidzin | C21H20O9 | 416.11 | 417.12 | 416.11 | −1.10 | 0.03 |
65 | 16.28 | Kushenol D | C27H32O6 | 452.22 | 451.21 | 452.22 | 2.10 | 0.47 |
66 | 16.31 | Acuminatin | C21H24O4 | 340.17 | 339.16 | 340.17 | −0.20 | 37.87 |
67 | 16.35 | Neokurarinol | C27H34O7 | 470.23 | 471.24 | 470.23 | −2.20 | 0.03 |
68 | 16.68 | Isoangustone A | C25H26O6 | 422.17 | 421.17 | 422.17 | 0.90 | 0.03 |
69 | 16.70 | Kadsulignan B | C25H30O9 | 474.19 | 473.18 | 474.19 | −0.60 | 0.02 |
70 | 17.04 | Kushenol M | C30H36O7 | 508.25 | 509.25 | 508.25 | −1.10 | 0.01 |
71 | 17.29 | Regaloside A | C18H24O10 | 400.14 | 401.14 | 400.14 | −1.80 | 0.03 |
72 | 18.56 | Magnolignan H | C36H34O6 | 562.24 | 561.23 | 562.24 | 2.40 | 0.02 |
73 | 20.55 | Neoquassin | C22H30O6 | 390.20 | 389.20 | 390.20 | 0.30 | 0.02 |
No. . | tR (min) . | Compound name . | Formula . | Calculated mass . | m/z . | Exact mass . | Mass accuracy mDa . | Base peaks (%) . |
---|---|---|---|---|---|---|---|---|
1 | 0.68 | Narcissin | C28H32O16 | 624.17 | 623.16 | 624.17 | 1.50 | 0.03 |
2 | 0.91 | Homoarecoline | C9H15NO2 | 169.11 | 170.12 | 169.11 | 0.10 | 0.33 |
3 | 0.94 | 6″-O-Acetylgenistin | C23H22O11 | 474.12 | 475.12 | 474.11 | −1.90 | 0.03 |
4 | 1.17 | Procyanidin B4/B5 | C30H26O12 | 578.14 | 579.15 | 578.14 | 1.10 | 1.09 |
5 | 1.56 | Arecatannin B1 | C45H38O18 | 866.21 | 867.21 | 866.20 | −1.00 | 0.59 |
6 | 1.73 | (+)-Catechin | C15H14O6 | 290.08 | 291.09 | 290.08 | −0.40 | 1.14 |
7 | 1.73 | 3-Hydroxybenzoic acid | C7H6O3 | 138.03 | 139.04 | 138.03 | 0.10 | 0.08 |
8 | 1.73 | 1-Hydroxy-3,7-dimethoxyxanthone | C15H12O5 | 272.07 | 273.07 | 272.07 | −1.10 | 0.03 |
9 | 1.74 | Plathymenin | C15H12O6 | 288.06 | 289.07 | 288.06 | −0.50 | 0.03 |
10 | 2.28 | Isorhamnetin | C16H12O7 | 316.06 | 315.05 | 316.06 | −1.40 | 0.01 |
11 | 2.94 | Nubigenol | C15H14O6 | 290.08 | 291.09 | 290.08 | 0.10 | 0.34 |
12 | 3.03 | 1,8-Dihydroxy-2,3,4,5-tetramethoxyxanthone | C17H16O8 | 348.08 | 349.09 | 348.08 | −1.10 | 0.01 |
13 | 3.34 | Baicalein | C15H10O5 | 270.05 | 271.06 | 270.05 | −1.00 | 0.01 |
14 | 3.70 | Eriodictyol | C15H12O6 | 288.06 | 287.06 | 288.06 | −0.50 | 0.02 |
15 | 4.46 | Astilbin | C21H22O11 | 450.12 | 451.12 | 450.12 | −0.90 | 0.01 |
16 | 4.59 | Andrographidine C | C23H24O10 | 460.14 | 461.14 | 460.14 | −0.60 | 0.01 |
17 | 4.65 | Complanatuside | C28H32O16 | 624.17 | 625.18 | 624.17 | −0.10 | 0.34 |
18 | 4.65 | Isorhamnetin-3-O-glucoside | C22H22O12 | 478.11 | 479.12 | 478.11 | −0.30 | 0.02 |
19 | 5.76 | Thymonin | C18H16O8 | 360.08 | 359.08 | 360.08 | −0.80 | 0.14 |
20 | 5.79 | Ombuin | C17H14O7 | 330.07 | 331.08 | 330.07 | −0.80 | 0.09 |
21 | 5.79 | Eupatolin | C23H24O12 | 492.13 | 493.13 | 492.13 | −0.40 | 0.02 |
22 | 5.96 | Quercetin | C15H10O7 | 302.04 | 303.05 | 302.04 | −1.10 | 0.03 |
23 | 6.55 | Vittariflavone | C24H26O12 | 506.14 | 505.13 | 506.14 | −2.20 | 0.08 |
24 | 6.64 | 1-Hydroxy-2,3,4,5-tetramethoxyxanthone | C17H16O7 | 332.09 | 333.10 | 332.09 | −0.60 | 0.06 |
25 | 6.85 | Saucernetin | C22H28O5 | 372.19 | 373.20 | 372.19 | 0.10 | 0.01 |
26 | 6.89 | Tenaxin I | C18H16O7 | 344.09 | 343.08 | 344.09 | −0.80 | 0.17 |
27 | 6.93 | 3-O-Methylquercetin | C16H12O7 | 316.06 | 317.07 | 316.06 | 0.00 | 0.20 |
28 | 7.10 | 5,6-Dihydroxy-7,3′,4′-trimethoxyflavone | C18H16O7 | 344.09 | 345.10 | 344.09 | −0.60 | 0.04 |
29 | 7.26 | Reineckiagenin | C27H44O5 | 448.32 | 449.33 | 448.32 | −0.70 | 0.01 |
30 | 7.60 | Kadsulignan F | C29H32O11 | 556.19 | 555.19 | 556.19 | −0.20 | 0.03 |
31 | 7.71 | Coniferyl alcohol | C10H12O3 | 180.08 | 181.09 | 180.08 | 0.70 | 0.05 |
32 | 8.26 | Grandoside | C17H32O11 | 412.19 | 411.19 | 412.19 | −1.60 | 0.01 |
33 | 8.48 | Magnograndiolide | C15H22O4 | 266.15 | 265.15 | 266.15 | 1.10 | 0.01 |
34 | 8.63 | 5,3′-Dihydroxy-6,7,4′-trimethoxyflavanone | C18H18O7 | 346.11 | 347.11 | 346.10 | −0.70 | 1.18 |
35 | 9.54 | Kadsulignan E | C31H30O11 | 578.18 | 579.18 | 578.18 | −2.80 | 0.01 |
36 | 10.18 | Kosamol A | C30H38O8 | 526.26 | 527.26 | 526.25 | −2.20 | 0.20 |
37 | 10.58 | Mulberrofuran P | C34H22O9 | 574.13 | 575.13 | 574.13 | 1.10 | 2.23 |
38 | 10.72 | Genistein | C15H10O5 | 270.05 | 271.06 | 270.05 | −0.50 | 0.14 |
39 | 10.74 | Glycyrrhizol B | C21H18O5 | 350.12 | 349.11 | 350.12 | 0.60 | 0.07 |
40 | 10.75 | Macluraxanthone | C23H22O6 | 394.14 | 393.14 | 394.14 | 0.70 | 0.05 |
41 | 10.76 | Nobiletin | C21H22O8 | 402.13 | 401.12 | 402.13 | −1.20 | 0.02 |
42 | 10.90 | Magnolignan E | C18H18O4 | 298.12 | 297.13 | 298.12 | −0.10 | 2.69 |
43 | 11.03 | Palmitic acid | C16H32O2 | 256.24 | 255.23 | 256.24 | −0.30 | 0.01 |
44 | 11.07 | Quercetin-3-arabinoside | C20H18O11 | 434.08 | 435.09 | 434.08 | −2.20 | 0.04 |
45 | 11.27 | Backuchiol | C18H24O | 256.18 | 257.19 | 256.18 | −0.50 | 0.01 |
46 | 11.31 | Picrasinoside G | C28H44O12 | 572.28 | 573.29 | 572.28 | 0.60 | 8.22 |
47 | 11.34 | Robinin | C33H40O19 | 740.22 | 739.21 | 740.21 | −2.50 | 0.02 |
48 | 11.53 | Tribuloside | C30H26O13 | 594.14 | 595.14 | 594.14 | −2.20 | 0.07 |
49 | 11.53 | Tectorigenin | C16H12O6 | 300.06 | 299.06 | 300.07 | 2.30 | 0.03 |
50 | 11.56 | Tomentolide A | C25H22O5 | 402.15 | 401.14 | 402.15 | 1.40 | 0.04 |
51 | 12.13 | Artemetin | C20H20O8 | 388.12 | 387.11 | 388.12 | 1.50 | 0.03 |
52 | 12.39 | Phanginin F | C21H28O6 | 376.19 | 375.18 | 376.19 | −0.50 | 0.04 |
53 | 12.50 | Stigmast-4-en-3-one | C29H48O | 412.37 | 411.36 | 412.37 | −5.00 | 0.15 |
54 | 12.52 | Picrasin F | C22H30O8 | 422.19 | 423.20 | 422.20 | 1.50 | 0.23 |
55 | 12.52 | Kushenol C | C25H26O7 | 438.17 | 437.16 | 438.17 | 1.40 | 0.07 |
56 | 12.69 | Glisoflavanone | C25H28O6 | 424.19 | 423.18 | 424.19 | 1.50 | 0.06 |
57 | 12.75 | Norkurarinol | C25H30O7 | 442.20 | 443.21 | 442.20 | −1.20 | 0.02 |
58 | 12.78 | Vitexin | C21H20O10 | 432.11 | 433.11 | 432.11 | 0.80 | 0.01 |
59 | 13.18 | Licuraside | C26H30O13 | 550.17 | 551.17 | 550.17 | −1.20 | 0.02 |
60 | 13.73 | Isokurarinone | C26H30O6 | 438.20 | 437.20 | 438.21 | 0.80 | 0.06 |
61 | 14.29 | Campesta-7,22E-dien-3beta-ol | C28H46O | 398.35 | 399.36 | 398.35 | −1.60 | 0.10 |
62 | 14.84 | Inosine | C10H12N4O5 | 268.08 | 267.07 | 268.08 | 0.00 | 0.08 |
63 | 15.14 | Moracin O | C19H18O5 | 326.12 | 325.11 | 326.11 | −0.50 | 0.02 |
64 | 16.20 | Daidzin | C21H20O9 | 416.11 | 417.12 | 416.11 | −1.10 | 0.03 |
65 | 16.28 | Kushenol D | C27H32O6 | 452.22 | 451.21 | 452.22 | 2.10 | 0.47 |
66 | 16.31 | Acuminatin | C21H24O4 | 340.17 | 339.16 | 340.17 | −0.20 | 37.87 |
67 | 16.35 | Neokurarinol | C27H34O7 | 470.23 | 471.24 | 470.23 | −2.20 | 0.03 |
68 | 16.68 | Isoangustone A | C25H26O6 | 422.17 | 421.17 | 422.17 | 0.90 | 0.03 |
69 | 16.70 | Kadsulignan B | C25H30O9 | 474.19 | 473.18 | 474.19 | −0.60 | 0.02 |
70 | 17.04 | Kushenol M | C30H36O7 | 508.25 | 509.25 | 508.25 | −1.10 | 0.01 |
71 | 17.29 | Regaloside A | C18H24O10 | 400.14 | 401.14 | 400.14 | −1.80 | 0.03 |
72 | 18.56 | Magnolignan H | C36H34O6 | 562.24 | 561.23 | 562.24 | 2.40 | 0.02 |
73 | 20.55 | Neoquassin | C22H30O6 | 390.20 | 389.20 | 390.20 | 0.30 | 0.02 |
The compounds listed in the table have intensity >10 000.
The major compounds identified from the ethanolic extract obtained by fermentative extraction of unripe areca nut according to UHPLC–MS/MS*
No. . | tR (min) . | Compound name . | Formula . | Calculated mass . | m/z . | Exact mass . | Mass accuracy mDa . | Base peaks (%) . |
---|---|---|---|---|---|---|---|---|
1 | 0.68 | Narcissin | C28H32O16 | 624.17 | 623.16 | 624.17 | 1.50 | 0.03 |
2 | 0.91 | Homoarecoline | C9H15NO2 | 169.11 | 170.12 | 169.11 | 0.10 | 0.33 |
3 | 0.94 | 6″-O-Acetylgenistin | C23H22O11 | 474.12 | 475.12 | 474.11 | −1.90 | 0.03 |
4 | 1.17 | Procyanidin B4/B5 | C30H26O12 | 578.14 | 579.15 | 578.14 | 1.10 | 1.09 |
5 | 1.56 | Arecatannin B1 | C45H38O18 | 866.21 | 867.21 | 866.20 | −1.00 | 0.59 |
6 | 1.73 | (+)-Catechin | C15H14O6 | 290.08 | 291.09 | 290.08 | −0.40 | 1.14 |
7 | 1.73 | 3-Hydroxybenzoic acid | C7H6O3 | 138.03 | 139.04 | 138.03 | 0.10 | 0.08 |
8 | 1.73 | 1-Hydroxy-3,7-dimethoxyxanthone | C15H12O5 | 272.07 | 273.07 | 272.07 | −1.10 | 0.03 |
9 | 1.74 | Plathymenin | C15H12O6 | 288.06 | 289.07 | 288.06 | −0.50 | 0.03 |
10 | 2.28 | Isorhamnetin | C16H12O7 | 316.06 | 315.05 | 316.06 | −1.40 | 0.01 |
11 | 2.94 | Nubigenol | C15H14O6 | 290.08 | 291.09 | 290.08 | 0.10 | 0.34 |
12 | 3.03 | 1,8-Dihydroxy-2,3,4,5-tetramethoxyxanthone | C17H16O8 | 348.08 | 349.09 | 348.08 | −1.10 | 0.01 |
13 | 3.34 | Baicalein | C15H10O5 | 270.05 | 271.06 | 270.05 | −1.00 | 0.01 |
14 | 3.70 | Eriodictyol | C15H12O6 | 288.06 | 287.06 | 288.06 | −0.50 | 0.02 |
15 | 4.46 | Astilbin | C21H22O11 | 450.12 | 451.12 | 450.12 | −0.90 | 0.01 |
16 | 4.59 | Andrographidine C | C23H24O10 | 460.14 | 461.14 | 460.14 | −0.60 | 0.01 |
17 | 4.65 | Complanatuside | C28H32O16 | 624.17 | 625.18 | 624.17 | −0.10 | 0.34 |
18 | 4.65 | Isorhamnetin-3-O-glucoside | C22H22O12 | 478.11 | 479.12 | 478.11 | −0.30 | 0.02 |
19 | 5.76 | Thymonin | C18H16O8 | 360.08 | 359.08 | 360.08 | −0.80 | 0.14 |
20 | 5.79 | Ombuin | C17H14O7 | 330.07 | 331.08 | 330.07 | −0.80 | 0.09 |
21 | 5.79 | Eupatolin | C23H24O12 | 492.13 | 493.13 | 492.13 | −0.40 | 0.02 |
22 | 5.96 | Quercetin | C15H10O7 | 302.04 | 303.05 | 302.04 | −1.10 | 0.03 |
23 | 6.55 | Vittariflavone | C24H26O12 | 506.14 | 505.13 | 506.14 | −2.20 | 0.08 |
24 | 6.64 | 1-Hydroxy-2,3,4,5-tetramethoxyxanthone | C17H16O7 | 332.09 | 333.10 | 332.09 | −0.60 | 0.06 |
25 | 6.85 | Saucernetin | C22H28O5 | 372.19 | 373.20 | 372.19 | 0.10 | 0.01 |
26 | 6.89 | Tenaxin I | C18H16O7 | 344.09 | 343.08 | 344.09 | −0.80 | 0.17 |
27 | 6.93 | 3-O-Methylquercetin | C16H12O7 | 316.06 | 317.07 | 316.06 | 0.00 | 0.20 |
28 | 7.10 | 5,6-Dihydroxy-7,3′,4′-trimethoxyflavone | C18H16O7 | 344.09 | 345.10 | 344.09 | −0.60 | 0.04 |
29 | 7.26 | Reineckiagenin | C27H44O5 | 448.32 | 449.33 | 448.32 | −0.70 | 0.01 |
30 | 7.60 | Kadsulignan F | C29H32O11 | 556.19 | 555.19 | 556.19 | −0.20 | 0.03 |
31 | 7.71 | Coniferyl alcohol | C10H12O3 | 180.08 | 181.09 | 180.08 | 0.70 | 0.05 |
32 | 8.26 | Grandoside | C17H32O11 | 412.19 | 411.19 | 412.19 | −1.60 | 0.01 |
33 | 8.48 | Magnograndiolide | C15H22O4 | 266.15 | 265.15 | 266.15 | 1.10 | 0.01 |
34 | 8.63 | 5,3′-Dihydroxy-6,7,4′-trimethoxyflavanone | C18H18O7 | 346.11 | 347.11 | 346.10 | −0.70 | 1.18 |
35 | 9.54 | Kadsulignan E | C31H30O11 | 578.18 | 579.18 | 578.18 | −2.80 | 0.01 |
36 | 10.18 | Kosamol A | C30H38O8 | 526.26 | 527.26 | 526.25 | −2.20 | 0.20 |
37 | 10.58 | Mulberrofuran P | C34H22O9 | 574.13 | 575.13 | 574.13 | 1.10 | 2.23 |
38 | 10.72 | Genistein | C15H10O5 | 270.05 | 271.06 | 270.05 | −0.50 | 0.14 |
39 | 10.74 | Glycyrrhizol B | C21H18O5 | 350.12 | 349.11 | 350.12 | 0.60 | 0.07 |
40 | 10.75 | Macluraxanthone | C23H22O6 | 394.14 | 393.14 | 394.14 | 0.70 | 0.05 |
41 | 10.76 | Nobiletin | C21H22O8 | 402.13 | 401.12 | 402.13 | −1.20 | 0.02 |
42 | 10.90 | Magnolignan E | C18H18O4 | 298.12 | 297.13 | 298.12 | −0.10 | 2.69 |
43 | 11.03 | Palmitic acid | C16H32O2 | 256.24 | 255.23 | 256.24 | −0.30 | 0.01 |
44 | 11.07 | Quercetin-3-arabinoside | C20H18O11 | 434.08 | 435.09 | 434.08 | −2.20 | 0.04 |
45 | 11.27 | Backuchiol | C18H24O | 256.18 | 257.19 | 256.18 | −0.50 | 0.01 |
46 | 11.31 | Picrasinoside G | C28H44O12 | 572.28 | 573.29 | 572.28 | 0.60 | 8.22 |
47 | 11.34 | Robinin | C33H40O19 | 740.22 | 739.21 | 740.21 | −2.50 | 0.02 |
48 | 11.53 | Tribuloside | C30H26O13 | 594.14 | 595.14 | 594.14 | −2.20 | 0.07 |
49 | 11.53 | Tectorigenin | C16H12O6 | 300.06 | 299.06 | 300.07 | 2.30 | 0.03 |
50 | 11.56 | Tomentolide A | C25H22O5 | 402.15 | 401.14 | 402.15 | 1.40 | 0.04 |
51 | 12.13 | Artemetin | C20H20O8 | 388.12 | 387.11 | 388.12 | 1.50 | 0.03 |
52 | 12.39 | Phanginin F | C21H28O6 | 376.19 | 375.18 | 376.19 | −0.50 | 0.04 |
53 | 12.50 | Stigmast-4-en-3-one | C29H48O | 412.37 | 411.36 | 412.37 | −5.00 | 0.15 |
54 | 12.52 | Picrasin F | C22H30O8 | 422.19 | 423.20 | 422.20 | 1.50 | 0.23 |
55 | 12.52 | Kushenol C | C25H26O7 | 438.17 | 437.16 | 438.17 | 1.40 | 0.07 |
56 | 12.69 | Glisoflavanone | C25H28O6 | 424.19 | 423.18 | 424.19 | 1.50 | 0.06 |
57 | 12.75 | Norkurarinol | C25H30O7 | 442.20 | 443.21 | 442.20 | −1.20 | 0.02 |
58 | 12.78 | Vitexin | C21H20O10 | 432.11 | 433.11 | 432.11 | 0.80 | 0.01 |
59 | 13.18 | Licuraside | C26H30O13 | 550.17 | 551.17 | 550.17 | −1.20 | 0.02 |
60 | 13.73 | Isokurarinone | C26H30O6 | 438.20 | 437.20 | 438.21 | 0.80 | 0.06 |
61 | 14.29 | Campesta-7,22E-dien-3beta-ol | C28H46O | 398.35 | 399.36 | 398.35 | −1.60 | 0.10 |
62 | 14.84 | Inosine | C10H12N4O5 | 268.08 | 267.07 | 268.08 | 0.00 | 0.08 |
63 | 15.14 | Moracin O | C19H18O5 | 326.12 | 325.11 | 326.11 | −0.50 | 0.02 |
64 | 16.20 | Daidzin | C21H20O9 | 416.11 | 417.12 | 416.11 | −1.10 | 0.03 |
65 | 16.28 | Kushenol D | C27H32O6 | 452.22 | 451.21 | 452.22 | 2.10 | 0.47 |
66 | 16.31 | Acuminatin | C21H24O4 | 340.17 | 339.16 | 340.17 | −0.20 | 37.87 |
67 | 16.35 | Neokurarinol | C27H34O7 | 470.23 | 471.24 | 470.23 | −2.20 | 0.03 |
68 | 16.68 | Isoangustone A | C25H26O6 | 422.17 | 421.17 | 422.17 | 0.90 | 0.03 |
69 | 16.70 | Kadsulignan B | C25H30O9 | 474.19 | 473.18 | 474.19 | −0.60 | 0.02 |
70 | 17.04 | Kushenol M | C30H36O7 | 508.25 | 509.25 | 508.25 | −1.10 | 0.01 |
71 | 17.29 | Regaloside A | C18H24O10 | 400.14 | 401.14 | 400.14 | −1.80 | 0.03 |
72 | 18.56 | Magnolignan H | C36H34O6 | 562.24 | 561.23 | 562.24 | 2.40 | 0.02 |
73 | 20.55 | Neoquassin | C22H30O6 | 390.20 | 389.20 | 390.20 | 0.30 | 0.02 |
No. . | tR (min) . | Compound name . | Formula . | Calculated mass . | m/z . | Exact mass . | Mass accuracy mDa . | Base peaks (%) . |
---|---|---|---|---|---|---|---|---|
1 | 0.68 | Narcissin | C28H32O16 | 624.17 | 623.16 | 624.17 | 1.50 | 0.03 |
2 | 0.91 | Homoarecoline | C9H15NO2 | 169.11 | 170.12 | 169.11 | 0.10 | 0.33 |
3 | 0.94 | 6″-O-Acetylgenistin | C23H22O11 | 474.12 | 475.12 | 474.11 | −1.90 | 0.03 |
4 | 1.17 | Procyanidin B4/B5 | C30H26O12 | 578.14 | 579.15 | 578.14 | 1.10 | 1.09 |
5 | 1.56 | Arecatannin B1 | C45H38O18 | 866.21 | 867.21 | 866.20 | −1.00 | 0.59 |
6 | 1.73 | (+)-Catechin | C15H14O6 | 290.08 | 291.09 | 290.08 | −0.40 | 1.14 |
7 | 1.73 | 3-Hydroxybenzoic acid | C7H6O3 | 138.03 | 139.04 | 138.03 | 0.10 | 0.08 |
8 | 1.73 | 1-Hydroxy-3,7-dimethoxyxanthone | C15H12O5 | 272.07 | 273.07 | 272.07 | −1.10 | 0.03 |
9 | 1.74 | Plathymenin | C15H12O6 | 288.06 | 289.07 | 288.06 | −0.50 | 0.03 |
10 | 2.28 | Isorhamnetin | C16H12O7 | 316.06 | 315.05 | 316.06 | −1.40 | 0.01 |
11 | 2.94 | Nubigenol | C15H14O6 | 290.08 | 291.09 | 290.08 | 0.10 | 0.34 |
12 | 3.03 | 1,8-Dihydroxy-2,3,4,5-tetramethoxyxanthone | C17H16O8 | 348.08 | 349.09 | 348.08 | −1.10 | 0.01 |
13 | 3.34 | Baicalein | C15H10O5 | 270.05 | 271.06 | 270.05 | −1.00 | 0.01 |
14 | 3.70 | Eriodictyol | C15H12O6 | 288.06 | 287.06 | 288.06 | −0.50 | 0.02 |
15 | 4.46 | Astilbin | C21H22O11 | 450.12 | 451.12 | 450.12 | −0.90 | 0.01 |
16 | 4.59 | Andrographidine C | C23H24O10 | 460.14 | 461.14 | 460.14 | −0.60 | 0.01 |
17 | 4.65 | Complanatuside | C28H32O16 | 624.17 | 625.18 | 624.17 | −0.10 | 0.34 |
18 | 4.65 | Isorhamnetin-3-O-glucoside | C22H22O12 | 478.11 | 479.12 | 478.11 | −0.30 | 0.02 |
19 | 5.76 | Thymonin | C18H16O8 | 360.08 | 359.08 | 360.08 | −0.80 | 0.14 |
20 | 5.79 | Ombuin | C17H14O7 | 330.07 | 331.08 | 330.07 | −0.80 | 0.09 |
21 | 5.79 | Eupatolin | C23H24O12 | 492.13 | 493.13 | 492.13 | −0.40 | 0.02 |
22 | 5.96 | Quercetin | C15H10O7 | 302.04 | 303.05 | 302.04 | −1.10 | 0.03 |
23 | 6.55 | Vittariflavone | C24H26O12 | 506.14 | 505.13 | 506.14 | −2.20 | 0.08 |
24 | 6.64 | 1-Hydroxy-2,3,4,5-tetramethoxyxanthone | C17H16O7 | 332.09 | 333.10 | 332.09 | −0.60 | 0.06 |
25 | 6.85 | Saucernetin | C22H28O5 | 372.19 | 373.20 | 372.19 | 0.10 | 0.01 |
26 | 6.89 | Tenaxin I | C18H16O7 | 344.09 | 343.08 | 344.09 | −0.80 | 0.17 |
27 | 6.93 | 3-O-Methylquercetin | C16H12O7 | 316.06 | 317.07 | 316.06 | 0.00 | 0.20 |
28 | 7.10 | 5,6-Dihydroxy-7,3′,4′-trimethoxyflavone | C18H16O7 | 344.09 | 345.10 | 344.09 | −0.60 | 0.04 |
29 | 7.26 | Reineckiagenin | C27H44O5 | 448.32 | 449.33 | 448.32 | −0.70 | 0.01 |
30 | 7.60 | Kadsulignan F | C29H32O11 | 556.19 | 555.19 | 556.19 | −0.20 | 0.03 |
31 | 7.71 | Coniferyl alcohol | C10H12O3 | 180.08 | 181.09 | 180.08 | 0.70 | 0.05 |
32 | 8.26 | Grandoside | C17H32O11 | 412.19 | 411.19 | 412.19 | −1.60 | 0.01 |
33 | 8.48 | Magnograndiolide | C15H22O4 | 266.15 | 265.15 | 266.15 | 1.10 | 0.01 |
34 | 8.63 | 5,3′-Dihydroxy-6,7,4′-trimethoxyflavanone | C18H18O7 | 346.11 | 347.11 | 346.10 | −0.70 | 1.18 |
35 | 9.54 | Kadsulignan E | C31H30O11 | 578.18 | 579.18 | 578.18 | −2.80 | 0.01 |
36 | 10.18 | Kosamol A | C30H38O8 | 526.26 | 527.26 | 526.25 | −2.20 | 0.20 |
37 | 10.58 | Mulberrofuran P | C34H22O9 | 574.13 | 575.13 | 574.13 | 1.10 | 2.23 |
38 | 10.72 | Genistein | C15H10O5 | 270.05 | 271.06 | 270.05 | −0.50 | 0.14 |
39 | 10.74 | Glycyrrhizol B | C21H18O5 | 350.12 | 349.11 | 350.12 | 0.60 | 0.07 |
40 | 10.75 | Macluraxanthone | C23H22O6 | 394.14 | 393.14 | 394.14 | 0.70 | 0.05 |
41 | 10.76 | Nobiletin | C21H22O8 | 402.13 | 401.12 | 402.13 | −1.20 | 0.02 |
42 | 10.90 | Magnolignan E | C18H18O4 | 298.12 | 297.13 | 298.12 | −0.10 | 2.69 |
43 | 11.03 | Palmitic acid | C16H32O2 | 256.24 | 255.23 | 256.24 | −0.30 | 0.01 |
44 | 11.07 | Quercetin-3-arabinoside | C20H18O11 | 434.08 | 435.09 | 434.08 | −2.20 | 0.04 |
45 | 11.27 | Backuchiol | C18H24O | 256.18 | 257.19 | 256.18 | −0.50 | 0.01 |
46 | 11.31 | Picrasinoside G | C28H44O12 | 572.28 | 573.29 | 572.28 | 0.60 | 8.22 |
47 | 11.34 | Robinin | C33H40O19 | 740.22 | 739.21 | 740.21 | −2.50 | 0.02 |
48 | 11.53 | Tribuloside | C30H26O13 | 594.14 | 595.14 | 594.14 | −2.20 | 0.07 |
49 | 11.53 | Tectorigenin | C16H12O6 | 300.06 | 299.06 | 300.07 | 2.30 | 0.03 |
50 | 11.56 | Tomentolide A | C25H22O5 | 402.15 | 401.14 | 402.15 | 1.40 | 0.04 |
51 | 12.13 | Artemetin | C20H20O8 | 388.12 | 387.11 | 388.12 | 1.50 | 0.03 |
52 | 12.39 | Phanginin F | C21H28O6 | 376.19 | 375.18 | 376.19 | −0.50 | 0.04 |
53 | 12.50 | Stigmast-4-en-3-one | C29H48O | 412.37 | 411.36 | 412.37 | −5.00 | 0.15 |
54 | 12.52 | Picrasin F | C22H30O8 | 422.19 | 423.20 | 422.20 | 1.50 | 0.23 |
55 | 12.52 | Kushenol C | C25H26O7 | 438.17 | 437.16 | 438.17 | 1.40 | 0.07 |
56 | 12.69 | Glisoflavanone | C25H28O6 | 424.19 | 423.18 | 424.19 | 1.50 | 0.06 |
57 | 12.75 | Norkurarinol | C25H30O7 | 442.20 | 443.21 | 442.20 | −1.20 | 0.02 |
58 | 12.78 | Vitexin | C21H20O10 | 432.11 | 433.11 | 432.11 | 0.80 | 0.01 |
59 | 13.18 | Licuraside | C26H30O13 | 550.17 | 551.17 | 550.17 | −1.20 | 0.02 |
60 | 13.73 | Isokurarinone | C26H30O6 | 438.20 | 437.20 | 438.21 | 0.80 | 0.06 |
61 | 14.29 | Campesta-7,22E-dien-3beta-ol | C28H46O | 398.35 | 399.36 | 398.35 | −1.60 | 0.10 |
62 | 14.84 | Inosine | C10H12N4O5 | 268.08 | 267.07 | 268.08 | 0.00 | 0.08 |
63 | 15.14 | Moracin O | C19H18O5 | 326.12 | 325.11 | 326.11 | −0.50 | 0.02 |
64 | 16.20 | Daidzin | C21H20O9 | 416.11 | 417.12 | 416.11 | −1.10 | 0.03 |
65 | 16.28 | Kushenol D | C27H32O6 | 452.22 | 451.21 | 452.22 | 2.10 | 0.47 |
66 | 16.31 | Acuminatin | C21H24O4 | 340.17 | 339.16 | 340.17 | −0.20 | 37.87 |
67 | 16.35 | Neokurarinol | C27H34O7 | 470.23 | 471.24 | 470.23 | −2.20 | 0.03 |
68 | 16.68 | Isoangustone A | C25H26O6 | 422.17 | 421.17 | 422.17 | 0.90 | 0.03 |
69 | 16.70 | Kadsulignan B | C25H30O9 | 474.19 | 473.18 | 474.19 | −0.60 | 0.02 |
70 | 17.04 | Kushenol M | C30H36O7 | 508.25 | 509.25 | 508.25 | −1.10 | 0.01 |
71 | 17.29 | Regaloside A | C18H24O10 | 400.14 | 401.14 | 400.14 | −1.80 | 0.03 |
72 | 18.56 | Magnolignan H | C36H34O6 | 562.24 | 561.23 | 562.24 | 2.40 | 0.02 |
73 | 20.55 | Neoquassin | C22H30O6 | 390.20 | 389.20 | 390.20 | 0.30 | 0.02 |
The compounds listed in the table have intensity >10 000.
Conclusions
In conclusion, Rhizopus orizae MW538932 isolated from the microbial mat growing on the stored Chogaru exhibited an ability to consume procyanidins and arecatannins. It is speculated that procyanidins and arecatannins are converted to PCA and PGCA, which in turn get converted to α-ketoadipate and enter the TCA cycle. This remarkable ability of Rhizopus orizae MW538932 to consume condensed tannins present in the unripe areca nut was exploited to produce various phenolic compounds by fermentative extraction. As the areca nut itself is lean on fermentable sugars and proteins, supplementary carbon and nitrogen sources were added, and the fermentation was monitored. The carbon and nitrogen sources were optimised initially by full factorial search, and then the best performing carbon-nitrogen source combination was tried at 1.5× strength and 2× strength. Considering the fact that the phenolics profile is variable during the fermentation, an attempt was made to find a suitable solvent to extract the maximum phenolics. Among all the solvents and their aqueous solutions studied (water, methanol, ethanol, and acetone), 50% (v/v), ethanol was found to be the best. Areca nut powder (10 g wet weight) supplemented with sucrose (1.5 g) and ammonium sulphate (0.3 g) fermented for 9 days and extracted with 50% (v/v) ethanol resulted in the extract having a total phenolics of 186.03 ± 2.50 mg GE/g areca nut and total flavonoids of 139.70 ± 2.00 mg CE/g areca nut. In this study, areca nut which is believed to contain valuable antioxidants and confer several health benefits as per the Indian system of Ayurveda and Chinese medical practices, has been subjected to fermentative extraction using a GRAS organism and produced an extract that is free from arecoline. This is the first report of fermentative extraction of phenolics from unripe areca nut. Quantification of prominent flavonoids, phenolic acids and polyphenols present in this extract can pave a way for its application in food and pharmaceutical industry.
Acknowledgment
Priyanka Hugar was supported by scholarship for doctoral course by the National Institute of Technology Karnataka (N.I.T.K.), Surathkal, India.
Author contributions
Priyanka Hugar: Data curation (lead); formal analysis (lead); investigation (lead); methodology (lead); writing – original draft (lead). Prasanna D. Belur: Conceptualization (lead); formal analysis (lead); methodology (lead); project administration (lead); supervision (lead); writing – review and editing (lead). Keyur Raval: Data curation (lead); formal analysis (lead); methodology (supporting); visualization (lead); writing – review and editing (supporting).
Conflict of interest statement
The authors have no conflict of interest to declare.
Ethical statement
Ethics approval was not required for this research.
Peer review
The peer review history for this article is available at https://www.webofscience.com/api/gateway/wos/peer-review/10.1111/ijfs.16579.
Data availability statement
Research data is not shared.
References
This research paper was extensively refered in this paper while elucidating the mechanism of catechin degradation and its disappearenec in the ferementation broth during the growth of Rhizopus orizae MW538932.
This research paper describe a solid-state fermentaion process for removing toxic molecules from the nutritious waste using fungal fermentation. This paper helped us to conceptualise the current work wherein we used Rhizopus orizae MW538932 to eliminate arocoline from the unripe areca nut powder, rich in phenolic substances.
This review paper became a basis for conceptualising the current research work on unripe areca nuts. Further, this paper was extensively refered in this research paper.
This research paper was the basis for developing our own UHPLC-MS/MS protocal for analysing the constituents of the unfermented and fermented areca nut samples.