-
PDF
- Split View
-
Views
-
Cite
Cite
Mehrajfatema Z Mulla, Jasim Ahmed, Duygu Baskaya-Sezer, Noor Al-Ruwaih, Effect of high-pressure treatment and cellulase-mediate hydrolysis on functional, rheological and microstructural properties of garden cress seed residual fibre, International Journal of Food Science and Technology, Volume 58, Issue 10, October 2023, Pages e1–e11, https://doi.org/10.1111/ijfs.15464
- Share Icon Share
Abstract
Garden cress (Lepidium sativum) is valued in Asia and some part of Africa for its seed oil and other parts of plant that retain curative activities and act as a tonic in traditional medicine. The residue left over after the oil and protein extraction is a rich source of bioactive compounds and dietary fibres. The garden cress seed residual fibre (GCRF) contained 99.82% total dietary fibre (TDF), 99.4% insoluble dietary fibre (IDF) and 0.38% soluble dietary fibre (SDF). To improve the industrial applications of the GCRF, an approach was taken to break down the IDF into the SDF following a treatment of enzymatic hydrolysis [cellulase at 2% or 5% (w/w)] and high-pressure processing (600 MPa/15 min) or a combination of both. The combined treatment had significantly improved the SDF concentration by loosening the surface structure of the GCRF and enhanced the hydration properties. The structural changes were elucidated using the Fourier transform infrared spectroscopy, rheometry and scanning electron microscopy. The Fourier transform infrared spectrum revealed a major shift in the cellulose band because of the combined effect of high-pressure processing and enzymatic hydrolysis. High-pressure-assisted enzymatic hydrolysis was determined the best combination to be ensured almost 18-fold increase in SDF ratio and to be improved radical scavenging activity more than two times in addition to well-documented change in viscoelastic properties of the GCRF.
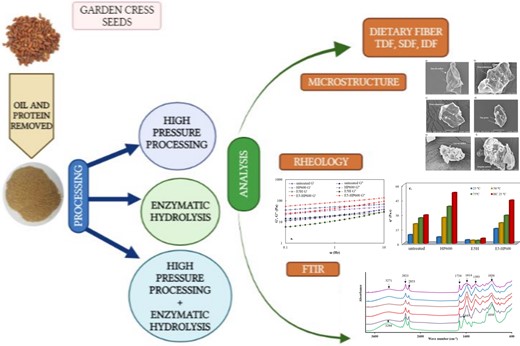
Introduction
Agricultural waste may create environmental and economic burdens to the society. The waste can be switched into resources using improved conversion processes, such as the green process that may yield sustainable potential bioproducts. After extraction of seed oil, the remaining residues are discarded by the processing industries. A planned execution of such waste conversion into value-added products as dietary fibre (DF) could bring additional revenue to the industry as a new food ingredient as well as it could contribute to the circular economy (Kavitha et al., 2020). Consumption of DF has become very common recently due to its proven health benefits. Epidemiological studies confirmed that there is a correlation between the consumption of DF and human health (Anderson et al., 2009, Chen et al., 2016). DF intake in daily meals stimulates gastrointestinal motility, cutting blood glucose, improves faecal bulk, and cholesterol levels (Kurek et al., 2018). Based on the water solubility, the DF can be divided into insoluble dietary fibre (IDF) and soluble dietary fibre (SDF) (Gan et al., 2021). IDF has a compact polymeric structure that is formed by stiff cellulose and flexible hemicellulose components, which makes it a highly tough structure that is difficult to disintegrate (Xie et al., 2019). Both IDF and SDF exhibited health benefits; however, SDF had superior functional and physicochemical properties than the IDF, which resulted in its wider applications in food industries as stabilising, emulsifying and thickening agents (Zhao et al., 2018; Gan et al., 2020). Additionally, SDF could reduce the rate of nutrient absorption in the small intestine because of their high viscosity and gel-forming capability, which assist to ferment by gut microbiota in the colon (Liu et al., 2020). Thus, a planned conversion strategy of the IDF obtained from the discarded waste into SDF could serve the purpose of generating a value-added product.
Transformation of lignocellulosic structure of IDF to soluble form requires a structural modification. The most common method used for the purpose is the enzymatic hydrolysis because of their superior efficiency, high specificity and mild treatment condition (Zhao, & Dong, 2016). Conversely, physical processing [e.g. high-pressure treatment and size reduction] has an advantage in performance and sustainability of structural modification and functional properties of DF (Yan, et al., 2015). It has been reported that the HP treatment (>500 MPa) increases the SDF content of mango pulp and seaweeds by 22% and 35% respectively (Elizondo-Montemayor et al., 2020) (Tapia-Salazar et al., 2019). Additionally, a significant increase in SDF content was observed for HP processed mango, orange and prickly pear peels containing DF content in the range of 40–50% at 600 MPa for 10 min at two temperatures 22 and 55 °C (Tejada-Ortigoza et al. 2017). Therefore, the HP treatment of 300 and 600 MPa at 15 min was decided on the basis of the DF content of garden cress residue to obtain maximum SDF. Moreover, it is also expected that a combined treatment of enzymatic hydrolysis and high-pressure treatment could effectively transform the IDF to SDF by the size reduction and cleaving the IDF glycosidic linkages resulting in increase in the yield, so the process could be successful commercially.
Garden cress (Lepidium sativum L.) is a perennial herb that belongs to the Cruciferae family; the seeds are enriched with edible oil and mucilaginous substances and the seeds contain 33–54% carbohydrates, 22%–25% proteins, 14%–27% lipids and 8% of crude fibre (Gokavi et al., 2004). The seed bran has high DF contents, which comprise >74% IDF and a marginal amount of SDF (<1%) (Gokavi, et al., 2004). Extracted cress seeds mucilage/gum has excellent gelling, emulsifying, stabilising and thickening properties because of its high-molecular weight and semi-rigid chain conformation, resulting in improvement in the rheological and textural properties of food products (Karazhiyan et al., 2011; Shams-abadi, & Razavi, 2021). Garden cress seeds are effective against many diseases, including cancer, hypertensive, spasmodic, bronchitis, diarrheal, asthma, coughs and many others (Maghrani et al., 2005; Patel et al., 2009; Rehman et al., 2012).
In this study, the influence of high-pressure and enzymatic hydrolysis individually and in a combination on the yield, functional, rheological and structural properties of garden cress seed residual fibre (GCRF) was studied. The findings of this study may provide a basis for increasing the utilisation of the garden cress seed residue or other oil seed residue for DF recovery and its fractionation, and also for the future application of recovered DFs, i.e., SDF and IDFs in food industries.
Materials and methods
Materials
Garden cress seeds (Indian produce) were procured from the Kuwait market. The garden cress residue was obtained after oil and protein recovery. Details of oil and protein recovery methods are available elsewhere (Mulla & Ahmed, 2019). The lyophilised cellulase enzyme (with 6000 U/g standard activity) produced by Trichoderma reesei fungus was obtained from Orba Biochemistry Corporation (İstanbul, Turkey). All the other chemicals were obtained from Sigma-Aldrich (Saint-Louis, MO, USA).
GCRF sample preparation
Garden cress seed residual fibre samples obtained after the protein and oil extraction from garden cress seed were washed with deionised water (six repeated cycles) and dried in a hot air tray dryer (Harvest Saver, Model #R-5A, Serial #13-355, USA) at 60 °C for 48 h. The air velocity and the relative humidity of the hot air oven were maintained at 2.9 m/sec and 30% respectively. The dried sample (20 g) was ground in a coffee grinder for 30 s (Siemens, MC23200GB, Slovenia) and sieved through 200-mesh sieve (<74 μm) (Endecotts, London, UK). The samples were sealed, packed in a food grade plastic jar and stored in a cold room (4–6 °C) until further use.
Preparation of buffer solution
A quantity of 50 mm citrate buffer solution was prepared by dissolving 10.5 g citric acid monohydrate (SD Fine-Chem Limited, India) in 750-mL distilled water. Sodium hydroxide pellet (Panreac Quimica, Spain) was added, and the pH adjusted to 5. Finally, the volume was made to 1 L using the distilled water.
Enzymatic hydrolysis of GCRF
The enzymatic hydrolysis (EH) used in our laboratory for sour cherry pomace was followed in this work with a minor modification (Sezer et al., 2021). The dried GCRF samples (25 g) were dissolved in 200 mL of 50 mm citrate buffer solution (1:10, w/v) at pH 5.0, and put in a refrigerator overnight for a complete hydration. The hydrated samples were digested with two selected concentrations of cellulase enzyme (2% and 5%, w/w) at 60 °C with continuous shaking for 60 min followed by inactivating the enzyme in boiling water. The enzymatically hydrolysed samples with 2% and 5% enzyme concentrations are termed as E2H and E5H respectively.
High-pressure-treated samples
For the high-pressure (HP) treatment, two sets of aqueous samples were made as discussed in the earlier section: (i) hydrated samples without cellulase and (ii) hydrated samples with the addition 5% cellulase (w/w). Samples were packed in low-density polyethylene (LDPE) pouches (Whirl- Pak, USA) and pressure treated. The first set of samples were treated at 300 (HP300) and 600 MPa (HP600) for 15 min, whereas the second set treated at 600 MPa for 15 min only (E5-HP600). Details of the HP process are available elsewhere (Ahmed et al., 2019). In brief, QFP 2 L-700 – a laboratory scale equipment (Avure Technologies, Ohio, USA) was employed for the pressurisation. The vessel diameter and height were 100 mm and 254 mm respectively. The initial temperature was 24 °C, which increased to 36 °C during the pressurisation from 0.101 to 600 MPa. After the HP treatment, samples were freeze-dried and ground to powder for further studies. Prior to freeze-drying, the cellulase of the second set of samples was inactivated in boiling water. A control or untreated sample without pressure treatment (0.01 MPa) was also freeze-dried for a comparison purpose with the HP-treated samples.
Dietary fibre assay
Automated IDF/SDF procedure (AOAC 991.43; Lee et al., 1992) was followed in the DF analyser (ANKOM, New York, USA). Results were corrected by considering ash (Method 923.03, AOAC 2005) and protein contents (Method 991.20, AOAC 2000). The process measured water and alcohol insoluble and water-soluble DF in the GCRF. Water soluble but alcohol-insoluble form of DF was reported as SDF contents.
Functional and structural properties
Measurement of colour and water-holding capacity (WHC)
The colour parameters [L (lightness), a (redness and greenness) and b (yellowness and blueness)] were measured using a colorimeter (LabScan XE; Hunter Associates Laboratory, Reston, VA). The colour values of white tile were taken as a reference (Lo = 93.77, ao = −1.02 and bo = −0.42). Three specimens for each sample were measured. Colour difference (∆E*) was calculated using the following Equation (1):
Water-holding capacity of the samples was measured at 25 °C using the centrifugal technique (Ahmed et al., 2016).
Oscillatory rheology
Oscillatory rheological measurements of the untreated and the treated GCRF samples [powdered sample (particle size of 297 μm) to water ratio = 1–4] were performed on a Discovery HR-3 (TA Instruments, New Castle, DE) with a 20-mm parallel plate geometry at 500-μm gap. Prior to the measurement, an oscillatory strain sweep test (0.001 and 10%) was conducted at 1 Hz in order to identify the linear viscoelastic range of the samples. The frequency sweep tests were carried out from 0.1 to 10 Hz at a constant strain of 0.1% at selected temperatures (25, 50 and 75 °C). To avoid the moisture evaporation from the sample, silicone oil was put in the periphery of the sample. Additionally, the heat-treated sample at 75 °C was cooled down to 25 °C using chiller within a few seconds and rerun the frequency sweep to explore the possible change during heating above the starch gelation temperature and back. All measurements were performed in duplicate and oscillatory moduli were acquired directly from the instrument software (TRIOS, TA Instruments, New Castle, DE, USA).
Fourier transform infrared (FTIR) spectroscopy
The Infrared spectra of the GCRF were recorded using a Nicolet iS5 FTIR spectrometer (Thermo Scientific, Madison, WI, USA). Spectrum in the range of 4000 to 550 cm−1 was recorded (average of 32 scans with a resolution of 4 cm−1).
Scanning electron microscopy (SEM) and particle size distribution
The microstructure and particle dimensions of the lyophilised GCRF samples were scanned over an SEM at 500× magnifications (JEOL, JCM-6000 Plus, Tokyo, Japan). Randomly 10 particles were selected for the particle size measurement through instrument software. Particle size distribution (PSD) of the treated and the untreated GCRF was measured by a Laser Diffraction Particle Size Analyser (Mastersizer 3000, Malvern Instruments, Worcestershire, UK) (Mulla and Ahmed, 2019).
Determination of 2, 2-diphenyl-1-picryhydrazyl (DPPH) radical-scavenging activity (DRSA)
The scavenging activities of the GCRF against the DPPH radical were determined following the method of Mulla and Ahmed (2019) with slight modifications. DPPH (100 μm) was dissolved in methanol and sample solutions were dissolved in 0.1 m phosphate buffer (pH 7.0) containing 1% (w/v) Triton X-100. An equal amount of DPPH solution and sample solution (0.3 mL) or buffer (control) were dynamically mixed followed by overtaxing and stored in the black room for 30 min. The absorbance of stored samples was measured at 517 nm using a spectrophotometer, and the scavenging activity was measured following the Equation (2):
where Ac is absorbance of control and As is absorbance of the sample.
Statistical analysis
The experimental data were subjected to one-way analysis of variance (ANOVA), followed by an appropriate comparison of mean values (JMP Pro 15, SAS Institute Inc., Cary, NC, USA). Tukey’s test was performed for multiple comparisons of the treatments.
Results and discussion
TDF, IDF and SDF contents
The GCRF samples mainly consist of TDF, IDF and a minor amount of SDF as shown in Table 1. The TDF content decreased significantly (P < 0.05) with high-pressure, enzymatic hydrolysis or in a combined treatment. Among treatments, the E5H was found to be the effective one that decreased the TDF to the maximum followed by the E5-HP600. A reduction of TDF from 40% to 24% using HP and EH was reported for okra (Pérez-López et al., 2016). It happened because of the reduction in particle size during the treatments, in particular, the degradation of hemicellulose, cellulose and lignin, which are turned into some finer particles (Xie et al., 2019, Zhu et al., 2010). The adopted method (AOAC 991.43) in the work could not be able to detect the alcohol-soluble fractions resulting in a major decrease in the TDF ratio as compared to the untreated sample.
The dietary fibre compositions, water-holding capacity, colour and scavenging activity of the GCRF samples
Samples . | IDF% . | SDF% . | TDF% . | WHC (g/g) . | L* . | a* . | b* . | ∆E* . | DPPH scavenging% . |
---|---|---|---|---|---|---|---|---|---|
Untreated | 99.44 ± 0.04a | 0.38 ± 0.04e | 99.82 ± 0.02a | 24.05 ± 0.32a | 60.11 ± 0.03e | 5.85 ± 0.02d | 22.26 ± 0.01c | 40.76 ± 0.02d | 12.92 ± 0.05f |
HP300 | 54.83 ± 0.03c | 1.98 ± 0.02d | 56.80 ± 0.05b | 19.40 ± 0.40d | 75.78 ± 0.09a | 4.06 ± 0.02g | 16.93 ± 0.01f | 25.11 ± 0.06f | 14.50 ± 0.10e |
HP600 | 56.69 ± 0.01b | 7.19 ± 0.02a | 63.82 ± 0.21c | 19.71 ± 0.54d | 75.15 ± 0.38b | 4.31 ± 0.11f | 16.64 ± 0.22g | 25.43 ± 0.44f | 20.32 ± 0.84d |
E2H | 36.12 ± 0.02d | 5.27 ± 0.02c | 41.40 ± 0.05e | 21.20 ± 0.19c | 59.06 ± 0.02f | 10.24 ± 0.01a | 28.65 ± 0.02b | 46.28 ± 0.02b | 24.31 ± 0.54c |
E5H | 28.51 ± 0.01f | 5.98 ± 0.02b | 34.48 ± 0.02g | 21.62 ± 0.05bc | 60.96 ± 0.08d | 9.69 ± 0.03b | 29.13 ± 0.06a | 45.06 ± 0.10c | 44.30 ± 0.57b |
E5-HP600 | 31.13 ± 0.14e | 7.17 ± 0.04a | 38.30 ± 0.16f | 22.21 ± 0.11b | 52.33 ± 0.01g | 9.44 ± 0.01c | 21.37 ± 0.00d | 47.59 ± 0.01a | 48.82 ± 0.02a |
Samples . | IDF% . | SDF% . | TDF% . | WHC (g/g) . | L* . | a* . | b* . | ∆E* . | DPPH scavenging% . |
---|---|---|---|---|---|---|---|---|---|
Untreated | 99.44 ± 0.04a | 0.38 ± 0.04e | 99.82 ± 0.02a | 24.05 ± 0.32a | 60.11 ± 0.03e | 5.85 ± 0.02d | 22.26 ± 0.01c | 40.76 ± 0.02d | 12.92 ± 0.05f |
HP300 | 54.83 ± 0.03c | 1.98 ± 0.02d | 56.80 ± 0.05b | 19.40 ± 0.40d | 75.78 ± 0.09a | 4.06 ± 0.02g | 16.93 ± 0.01f | 25.11 ± 0.06f | 14.50 ± 0.10e |
HP600 | 56.69 ± 0.01b | 7.19 ± 0.02a | 63.82 ± 0.21c | 19.71 ± 0.54d | 75.15 ± 0.38b | 4.31 ± 0.11f | 16.64 ± 0.22g | 25.43 ± 0.44f | 20.32 ± 0.84d |
E2H | 36.12 ± 0.02d | 5.27 ± 0.02c | 41.40 ± 0.05e | 21.20 ± 0.19c | 59.06 ± 0.02f | 10.24 ± 0.01a | 28.65 ± 0.02b | 46.28 ± 0.02b | 24.31 ± 0.54c |
E5H | 28.51 ± 0.01f | 5.98 ± 0.02b | 34.48 ± 0.02g | 21.62 ± 0.05bc | 60.96 ± 0.08d | 9.69 ± 0.03b | 29.13 ± 0.06a | 45.06 ± 0.10c | 44.30 ± 0.57b |
E5-HP600 | 31.13 ± 0.14e | 7.17 ± 0.04a | 38.30 ± 0.16f | 22.21 ± 0.11b | 52.33 ± 0.01g | 9.44 ± 0.01c | 21.37 ± 0.00d | 47.59 ± 0.01a | 48.82 ± 0.02a |
Samples are HP300: Treated with high pressure (HP) at 300 MPa for 15 min; HP600: Treated at 600 MPa for 15 min; E2H: After 2% (w/w) cellulase addition, enzymatic hydrolysis assisted by conventional heating at 60 °C for 60 min; E5H: After 5% (w/w) cellulase addition, enzymatic hydrolysis assisted by conventional heating at 60 °C for 60 min; E5-HP600: After 5% (w/w) cellulase addition, enzymatic hydrolysis assisted by HP at 600 MPa for 15 min. All values are means ± SD, n = 3; values in the same column with different letters are significantly different (P < 0.05).
The dietary fibre compositions, water-holding capacity, colour and scavenging activity of the GCRF samples
Samples . | IDF% . | SDF% . | TDF% . | WHC (g/g) . | L* . | a* . | b* . | ∆E* . | DPPH scavenging% . |
---|---|---|---|---|---|---|---|---|---|
Untreated | 99.44 ± 0.04a | 0.38 ± 0.04e | 99.82 ± 0.02a | 24.05 ± 0.32a | 60.11 ± 0.03e | 5.85 ± 0.02d | 22.26 ± 0.01c | 40.76 ± 0.02d | 12.92 ± 0.05f |
HP300 | 54.83 ± 0.03c | 1.98 ± 0.02d | 56.80 ± 0.05b | 19.40 ± 0.40d | 75.78 ± 0.09a | 4.06 ± 0.02g | 16.93 ± 0.01f | 25.11 ± 0.06f | 14.50 ± 0.10e |
HP600 | 56.69 ± 0.01b | 7.19 ± 0.02a | 63.82 ± 0.21c | 19.71 ± 0.54d | 75.15 ± 0.38b | 4.31 ± 0.11f | 16.64 ± 0.22g | 25.43 ± 0.44f | 20.32 ± 0.84d |
E2H | 36.12 ± 0.02d | 5.27 ± 0.02c | 41.40 ± 0.05e | 21.20 ± 0.19c | 59.06 ± 0.02f | 10.24 ± 0.01a | 28.65 ± 0.02b | 46.28 ± 0.02b | 24.31 ± 0.54c |
E5H | 28.51 ± 0.01f | 5.98 ± 0.02b | 34.48 ± 0.02g | 21.62 ± 0.05bc | 60.96 ± 0.08d | 9.69 ± 0.03b | 29.13 ± 0.06a | 45.06 ± 0.10c | 44.30 ± 0.57b |
E5-HP600 | 31.13 ± 0.14e | 7.17 ± 0.04a | 38.30 ± 0.16f | 22.21 ± 0.11b | 52.33 ± 0.01g | 9.44 ± 0.01c | 21.37 ± 0.00d | 47.59 ± 0.01a | 48.82 ± 0.02a |
Samples . | IDF% . | SDF% . | TDF% . | WHC (g/g) . | L* . | a* . | b* . | ∆E* . | DPPH scavenging% . |
---|---|---|---|---|---|---|---|---|---|
Untreated | 99.44 ± 0.04a | 0.38 ± 0.04e | 99.82 ± 0.02a | 24.05 ± 0.32a | 60.11 ± 0.03e | 5.85 ± 0.02d | 22.26 ± 0.01c | 40.76 ± 0.02d | 12.92 ± 0.05f |
HP300 | 54.83 ± 0.03c | 1.98 ± 0.02d | 56.80 ± 0.05b | 19.40 ± 0.40d | 75.78 ± 0.09a | 4.06 ± 0.02g | 16.93 ± 0.01f | 25.11 ± 0.06f | 14.50 ± 0.10e |
HP600 | 56.69 ± 0.01b | 7.19 ± 0.02a | 63.82 ± 0.21c | 19.71 ± 0.54d | 75.15 ± 0.38b | 4.31 ± 0.11f | 16.64 ± 0.22g | 25.43 ± 0.44f | 20.32 ± 0.84d |
E2H | 36.12 ± 0.02d | 5.27 ± 0.02c | 41.40 ± 0.05e | 21.20 ± 0.19c | 59.06 ± 0.02f | 10.24 ± 0.01a | 28.65 ± 0.02b | 46.28 ± 0.02b | 24.31 ± 0.54c |
E5H | 28.51 ± 0.01f | 5.98 ± 0.02b | 34.48 ± 0.02g | 21.62 ± 0.05bc | 60.96 ± 0.08d | 9.69 ± 0.03b | 29.13 ± 0.06a | 45.06 ± 0.10c | 44.30 ± 0.57b |
E5-HP600 | 31.13 ± 0.14e | 7.17 ± 0.04a | 38.30 ± 0.16f | 22.21 ± 0.11b | 52.33 ± 0.01g | 9.44 ± 0.01c | 21.37 ± 0.00d | 47.59 ± 0.01a | 48.82 ± 0.02a |
Samples are HP300: Treated with high pressure (HP) at 300 MPa for 15 min; HP600: Treated at 600 MPa for 15 min; E2H: After 2% (w/w) cellulase addition, enzymatic hydrolysis assisted by conventional heating at 60 °C for 60 min; E5H: After 5% (w/w) cellulase addition, enzymatic hydrolysis assisted by conventional heating at 60 °C for 60 min; E5-HP600: After 5% (w/w) cellulase addition, enzymatic hydrolysis assisted by HP at 600 MPa for 15 min. All values are means ± SD, n = 3; values in the same column with different letters are significantly different (P < 0.05).
The IDF content of the samples decreased with the application of pressure and enzyme treatments. The application of the highest pressure (600 MPa) reduced the IDF from 99.44 to 56.69%, whereas the maximum drop was achieved after 5% cellulase hydrolysis to 28.51%. Cellulose converts into hemicellulose with the modification of cellulase (Horn et al., 2012). Conversely, HP treatment induces reduction of particle size and also the conversion of IDF (Pérez-López et al., 2016). The reduction of the IDF from 83% to 70% and 75% to 67% was reported for carrot pomace and rice bran, respectively, after the pressurisation (Yu et al., 2018; Wen et al., 2017). The yield of the SDF was increased with treatment as can be observed in the Table 1. Apparently, the HP600 resulted in the highest SDF of 7.19% followed by the E5-HP600 with a marginally lower value of 7.17% (P > 0.05). The HP300-treated sample had lower SDF content than found in HP600. It was probably due to the fact that cell conformation and permeability might have been modified due to the change in cell wall structure without detrimental effect above 400 MPa (Tapia-Salazar et al., 2019). Interestingly, the decrease in IDF and yield of the SDF did not follow a trend. For example, the decrease in the IDF was the maximum (28.51%) for E5H, whereas the yield of the SDF was 5.98%. It infers that the conversion of IDF to SDF is a complex phenomenon, and it depends upon many factors, including the ease of separation of hemicellulose and cellulose, particle size, reactivity and breaking down of the bonds, etc. The drop in the IDF could be initiated by the depolymerisation of macromolecules which results in rupture of cellulosic structure which improves the superficial area and promotes particle size reduction (Xie et al., 2019). Additionally, cellulase randomly attacked the internal sites of the amorphous region of the polysaccharide chains and generally employed to solubilise cell wall, thereby accelerating the release of intracellular biomolecules and breaking the weak bonds (Hari Krishna and Karanth, 2002). Therefore, the particles were exposed to more water which improved the solubility of the material (Elleuch et al., 2011) and small oligosaccharides were formed with varied lengths (Fernandes and Carvalho, 2017).
Water-holding capacity
The WHC of the GCRF subjected to selected treatments is shown in Table 1. The WHC decreased with the treatment of the GCRF. The maximum WHC of the untreated sample might arise due to the presence of the highest amount of IDF content. The relation between IDF and WHC was believed to be associated with the cell wall structures and polarity of the functional groups (i.e. OH, COOH and C = O). The functional groups are attached to the sugar residues in cell wall polysaccharides that could allow the formation of hydrogen bonding with water molecules (Tejada-Ortigoza et al., 2016). The drop in the WHC for the treated sample is related to the DF content and decrease in particle size after the HP and EH treatments (Parra et al., 2019, Mancebo et al., 2018). Similar results were reported by particle size reduction in Qingke bran IDF (Zhu et al., 2015) and in rice bran IDF (Zhao et al., 2018).
Colour
The lightness (L*) of the GCRF increased after the HP treatment, although there was no significant difference between the pressurisation at 300 and 600 MPa, which is consistent with the earlier report (Sánchez-Moreno et al. (2006). Conversely, EH did not improve the lightness significantly (P > 0.05). The decline in L* value of the combined treatment might occur because of the removal or separation of the top layer of the surface. Similar decreasing trend of L* values was reported by Kuan and Liong (2008) for the rice husk. Both colour a* and b* values dropped after the pressurisation, whereas the EH and the combined treatment improved those values, indicating increase in the redness and yellowness. It has been reported that the HP treatment did not affect the pigments, so a marginal decrease in both a and b values after pressurisation is expected and matched with the published literature (Ahmed et al. (2005), McInerney et al. (2007). A significant increase of a* and b* could be influenced by the enzymatic extraction of carotenoid pigments in the garden cress seed (Rahman et al., 2017). The total colour difference (∆E*) followed the trend of a* and b* resulting in decrease after HP treatment and increased significantly after EH and combined treatment.
Oscillatory rheology
Mechanical spectra of the untreated and the treated GCRF samples measured at 25 °C are illustrated in Fig. 1a, b. Apparently both the evolved elastic (Gʹ) and viscous modulus (Gʺ) are frequency dependent and the values increased with increasing the frequency from 0.1 to 10 Hz. The predominating Gʹ over Gʺ (Gʹ > Gʺ) indicates a solid-like behaviour for the DF samples irrespective of the treatment. This observation is consistent with the work reported by Liu et al. (2020) for various quinoa SDF samples, which was obtained through ultrasound-assisted enzymatic extraction from quinoa brans. The Gʹ-ω and Gʹ- η* rheograms were lowered by about 30% and 60% for the sample treated at 600 MPa for 15 min and 5% cellulase treatment, respectively, as compared to the untreated sample. The solid-like property reduced with the treatment as indicated by the increase of the slope of the equation ln ω vs. ln G′ from 0.14 to 0.20. It indicates that the high-pressure treatment lowered the rigidity with simultaneous increment of liquid-like property; however, the enzymatic hydrolysis lowered the rigidity dramatically to its lowest level. Such changes in the viscoelastic properties of the treated DF are believed to be contributed to the compositional change as reported in Table 1. The obtained results showed that the HP and EH markedly altered all three major components, namely, TDF, IDF and SDF. The reduction of IDF content might be due, in part, to the conversion from insoluble cellulose and hemicellulose to SDF after pressure treatment (Kokorevics & Gravitis, 1997). The enzymatic hydrolysis caused depolymerisation of DFs resulting in a drastic change in the rheological behaviour. A significant change of the rheological behaviour of guar gum solution was reported after the enzymatic hydrolysis and the resulted solution transformed from a non-Newtonian to Newtonian behaviour (Mudgil et al., 2012). Conversely, a combination of cellulase hydrolysis followed by the HP treatment improved the dynamic moduli significantly (>60%) (Fig. 1a, b). We believe that such an improvement in the rheological properties could be associated with the reassociation of the depolymerised finer particles of water-soluble polymer.
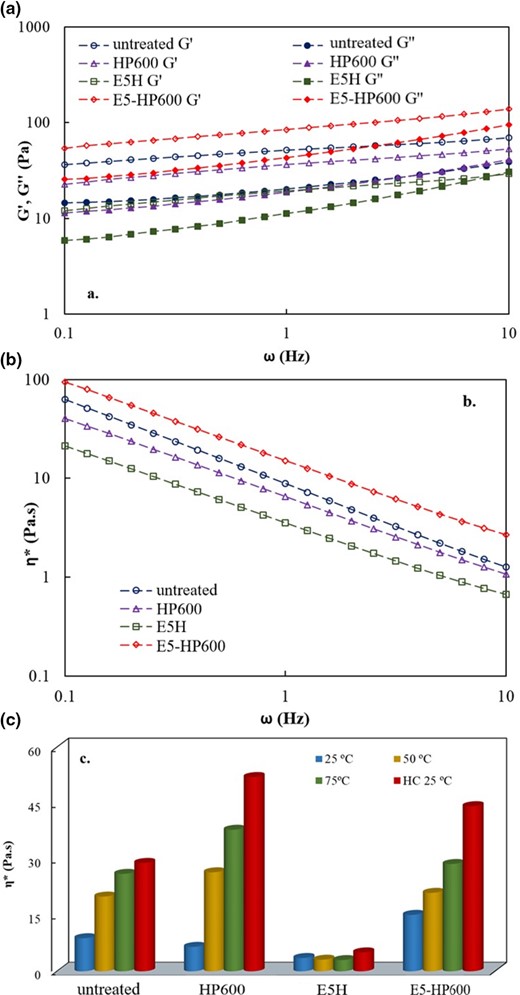
Oscillatory rheology of garden cress residue fibres after selected treatments (a). the elastic and viscous modulus as a function of frequency at 25 °C (b). complex viscosity as a function of frequency at 25 °C and (c). influence of heat and heat–cool (HC) treatments on the complex viscosity measured at 1 Hz.
The influence of temperature on the complex viscosity (η*) of DF dispersions with and without treatment at 1 Hz is shown in Fig. 1c. It can be seen that the η* increased linearly with increasing temperature from 25 to 75 °C. The increment might be attributed to the gelatinisation and denaturation of residual starch and protein present in the DF respectively (Schmidt et al., 2018). The HP-treated samples at 600 MPa/15 min attained the highest η* because of the synergic pressure and temperature effect on the DF with the presence of the SDF at the highest level. The enzyme-hydrolysed samples disrupted major chemical bonding, and therefore did not exhibit much influence on the temperature. Conversely, the combined treatment (E5-HP600) showed higher η* values as compared to DF; however, the range was lower than that of the HP-treated one. This result indicated that E5-HP600 treatment proved to be more effective in converting the DF fractions from insoluble into a soluble one, which showed a change in the η* lower than the HP alone. All heat-cool (HC) samples followed a similar trend as observed for the temperature effect. The significantly higher values of η* were obtained after the heating–cooling cycle because of the strong three-dimensional gel network formation after gelatinisation/denaturation followed by the cooling (P < 0.05).
Molecular structure
The influence of the selected treatments on the polymer structures of the GCRF was elucidated by the FTIR spectroscopy (Fig. 2). Overall, the treatment did not have a significant influence on the spectral profile (P < 0.05). GCRF depicted absorption peaks located at 3271, 2923, 1393, 1235, 1147, 1036 and 894. Several studies reported the presence of the cellulose polysaccharide compounds with many absorbance peaks located at 3294, 2923, 1393, 1234, 1146, 1039 and 894 cm−1 (Zhang et al., 2015). However, HP treatment did not alter the structure and reorganisation of GCRF resulted in the peaks located at 3271, 2923, 1393, 1235, 1147, 1036 and 894 remaining almost unchanged. Nonetheless, E5H and E5-HP600 samples exhibited a major shift of cellulose bands from 3294 to 3271 cm−1 and from 1039 to 1036 cm−1. It indicates the breakage of the intra-molecular hydrogen bonding in cellulose and hemicellulose and dissociation of the non-crystalline region in DF as assisted by the EH (Wen et al., 2017; Xie et al., 2019). The band at 1645 cm−1 was observed only in the untreated sample that is associated with the characteristic absorption of benzene ring in lignin (Lin et al., 2019).
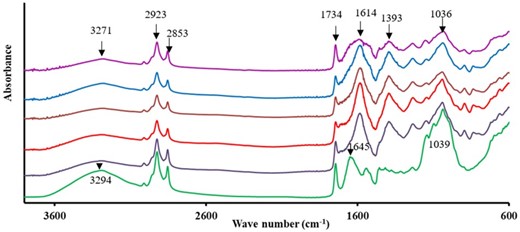
FTIR spectra of the GCRF samples (untreated;
HP300;
HP600;
E2H;
E5H and
E5-HP600).
Scanning electron microscopy (SEM) and particle size analysis
The particle SEM morphology of the untreated GCRF portrayed a smooth surface; however, deep indentations were evident after the HP treatments (Fig. 3). The GCRF hydrolysed with 2% cellulase resulted in a partial porous structure with dent, while 5% cellulase treatment produced irregular shapes with uneven surface. The E5-HP600 treatment produced a compact structure with an enlargement of the particle. Earlier, it has been reported that the EH produced rough and porous structure in the DF (Yu et al. 2018), whereas a combination of EH and HP treatment resulted in irregular particles (Yu et al., 2018; Xie et al., 2019).
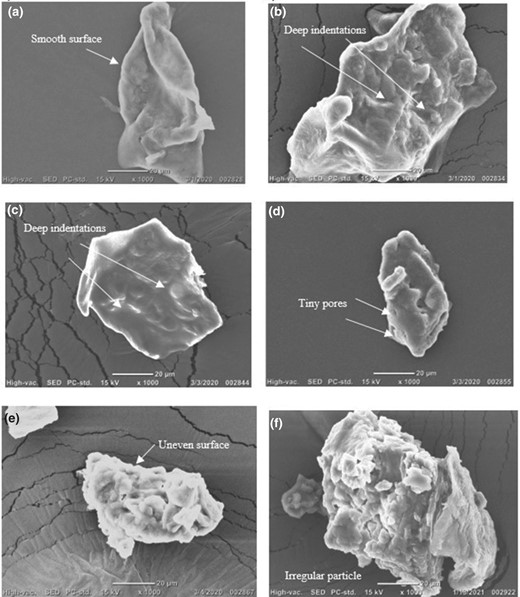
SEM of the GCRF samples (a) untreated, (b) HP300, (c) HP600, (d) E2H, (e) E5H and (f) E5-HP600.
Particle size distribution (Dv10, Dv50 and Dv90) of the untreated and the treated GCRF samples is presented in Table 2. The Dv50 (median particle size) of the GCRF before the treatment was 435 μm, which was significantly (P < 0.05) reduced the size after the treatments; the HP-treated samples reduced in the range of 349–356 μm; EH produced the size between 230 and 250 μm, and the combined treatment resulted in the size of 316 μm. Similarly, the Dv90 of the untreated samples reduced significantly (P < 0.05) from 991 μm to 753 μm, 646 μm and 676 μm for HP-600, E5H and E5-HP600 treatments respectively. These data clearly show that the treatments reduced the particle size significantly; however, the final size depends upon the mode of treatment applied to the GCRF. Among treatments, the E2H reduced the size at the maximum (47% and 36% for Dv50 and Dv90 respectively). The combination treatment showed a value between the HP and EH treatments, confirming that both treatments have a contribution in the degradation of GCRF. Degradation of the polymers is a critical change which contributes to the transformation of IDF into soluble form. Additionally, it can also improve antioxidative activity of the material (Zheng et al., 2021, Qiu et al., 2019). Since the compact arrangements of the matrix are disintegrated using physical, chemical and biological methods, particle size of the material decreased, and also the hydrophilics surrounded by hydrophobic structures could liberate and bind water molecules (Gu et al., 2020, Wen et al., 2017). A similar reduction in particle size was reported for the HP treated and EH deoiled cumin seed DF (Ma & Mu 2016).
Particle sizes . | Untreated . | HP300 . | HP600 . | E2H . | E5H . | E5-HP600 . |
---|---|---|---|---|---|---|
Dv(10) | 98 ± 40a | 88 ± 30b | 109 ± 20c | 36 ± 40d | 25 ± 10e | 64 ± 11f |
Dv(50) | 435 ± 19a | 356 ± 90b | 349 ± 13b | 230 ± 90d | 250 ± 50d | 316 ± 15c |
Dv(90) | 991 ± 76a | 816 ± 61b | 753 ± 60c | 637 ± 26d | 646 ± 24d | 676 ± 31d |
Particle sizes . | Untreated . | HP300 . | HP600 . | E2H . | E5H . | E5-HP600 . |
---|---|---|---|---|---|---|
Dv(10) | 98 ± 40a | 88 ± 30b | 109 ± 20c | 36 ± 40d | 25 ± 10e | 64 ± 11f |
Dv(50) | 435 ± 19a | 356 ± 90b | 349 ± 13b | 230 ± 90d | 250 ± 50d | 316 ± 15c |
Dv(90) | 991 ± 76a | 816 ± 61b | 753 ± 60c | 637 ± 26d | 646 ± 24d | 676 ± 31d |
All values are means ± SD, n = 3; values in the same row with different letters are significantly different (P < 0.05).
Particle sizes . | Untreated . | HP300 . | HP600 . | E2H . | E5H . | E5-HP600 . |
---|---|---|---|---|---|---|
Dv(10) | 98 ± 40a | 88 ± 30b | 109 ± 20c | 36 ± 40d | 25 ± 10e | 64 ± 11f |
Dv(50) | 435 ± 19a | 356 ± 90b | 349 ± 13b | 230 ± 90d | 250 ± 50d | 316 ± 15c |
Dv(90) | 991 ± 76a | 816 ± 61b | 753 ± 60c | 637 ± 26d | 646 ± 24d | 676 ± 31d |
Particle sizes . | Untreated . | HP300 . | HP600 . | E2H . | E5H . | E5-HP600 . |
---|---|---|---|---|---|---|
Dv(10) | 98 ± 40a | 88 ± 30b | 109 ± 20c | 36 ± 40d | 25 ± 10e | 64 ± 11f |
Dv(50) | 435 ± 19a | 356 ± 90b | 349 ± 13b | 230 ± 90d | 250 ± 50d | 316 ± 15c |
Dv(90) | 991 ± 76a | 816 ± 61b | 753 ± 60c | 637 ± 26d | 646 ± 24d | 676 ± 31d |
All values are means ± SD, n = 3; values in the same row with different letters are significantly different (P < 0.05).
Effect of EH on antioxidant activity of the GCRF
DPPH radical-scavenging activity (DRSA)
The effect of HP and cellulase addition on the antioxidant properties of the GCRF were assessed on their DPPH radical-scavenging activity (DRSA) (Table 1). DRSA values of the untreated GCRF samples increased with the treatments from 12.92% to 20.32%, 44.30 and 48.82% for HP600, E5H and E5-HP600 treatments respectively. Such an increase could be attributed to the enhanced concentration of soluble polysaccharides or SDF on account of HP and cellulase treatment. Similar findings were obtained for wheat bran polysaccharides (Yan et al., 2015) and cumin seed DF (Ma & Mu 2016) due to the increase in water-soluble polysaccharides and SDF content of enzyme-treated samples. It has been reported that the HP processed, and enzyme-treated DFs form phenol–polysaccharide bonds and elevated antioxidant activity (Yan et al., 2015; Malunga, & Beta, 2015). Interestingly, glycosidic linkages and the proportion of monosaccharides are also accountable for elevated antioxidant activity (Malunga, & Beta, 2015).
Conclusions
In this work, the residue of garden cress seed was treated using high-pressure and enzymatic hydrolysis in order to modify the IDF to a soluble one. Among selected treatments, the results suggested that a combination of enzymatic treatment with 5% cellulase and a pressure level of 600 MPa for 15 min to GCRF resulted in reducing the IDF significantly and increased the yield of the SDF. Enzymatic hydrolysis of GCRF imparted porous network structure, whereas the combined treatment showed crystals with porous interconnected particles. All GCRF samples in aqueous solution showed a solid-like property (G′>G″) and the viscoelasticity increased with the treatments. The particle size of the DFs was reduced significantly by the treatments and EH was found the most effective in the size reduction (P < 0.05). FTIR spectroscopy of these different DFs also showed varied molecular characteristics. Moreover, the highest DRSA value and SDF ratio were measured in the E5-HP600 sample. Garden cress SDF might possess the potential for functional ingredients, such as prebiotics in the food and pharma industry for improving the human gut health and immune function via promoting healthy microbiome and reducing the risk of developing cardiovascular disease and type 2 diabetes by decreasing postprandial blood glucose, insulin, triglycerides and LDL cholesterol levels. Therefore, the associated physiological function and fermentative properties of SDF will be explored in future study. In addition, due to the nature of GCRF, the investigation of the odour profile of the material might be of greater importance for its exploitation as a functional ingredient in food products and prebiotics for future studies.
Conflict of interest
The authors declare no conflicts of interest.
Acknowledgment
The authors would like to thank Orba Biochemistry Corp. (İstanbul, Turkey) for their generosity for providing enzymes for the work.
Author contribution
Mehrajfatema Zafar Mulla: Conceptualization (lead); Project administration (lead); Writing – original draft (lead). jasim ahmed: Writing – review & editing (lead). Duygu Baskaya-Sezer: Formal analysis (equal). Noor Al-Ruwaih: Formal analysis (equal).
Ethical guidelines
Ethics approval was not required for this research.
Peer review
The peer review history for this article is available at https://publons.com/publon/10.1111/ijfs.15464.
Data availability statement
The data that support the findings of this study are available on request from the corresponding author. The data are not publicly available due to privacy or ethical restrictions.
References
The paper investigated the effect of shear deformation and high pressure on the cellulose depolymerisation. In particular, SDHP provides better transformations in a short period of time.
The paper described the impact of alcalase enzyme on garden cress protein extracted from garden cress pomace. While the enzyme treatment slightly improved the antioxidant properties and the total phenolic compounds, the garden cress protein considerably enhances the rheological and structural properties.
The manuscript reported the impact of enzymatic treatment under high hydrostatic pressure on okara and release of low molecular weight carbohydrates. The outcome showed that enzyme treatment improved the effect of high hydrostatic pressure treatment.
The paper described the effects of partial flour replacement by blackcurrant pomace in savoury crackers. Pomace addition significantly affected both systems. A restrained protein development was observed in pomace containing dough, leading to a lower extensibility, and to reduced volume increase and lower breaking force after baking.