-
PDF
- Split View
-
Views
-
Cite
Cite
Pochanart Kanjan, Phat Sakpetch, Effect of antifungal compounds secreted by Lactiplantibacillus plantarum 124 against Aspergillus flavus and Penicillium sp. and its application in Kaeng-Tai-Pla-Haeng to extend the shelf life, International Journal of Food Science and Technology, Volume 58, Issue 10, October 2023, Pages 5376–5387, https://doi.org/10.1111/ijfs.16656
- Share Icon Share
Abstract
Aspergillus flavus and Penicillium spp. are the most common spoilage fungi in the ready-to-eat, intermediate-moisture Thai curry of Kaeng-Tai-Pla-Haeng, which causes serious economic loss and public health concerns. The use of lactic acid bacteria (LAB) with antifungal activity has been a growing interest in food bio-preservation. In this study, the antifungal efficacy of Lactiplantibacillus plantarum 124 (L. plantarum 124) against the spoilage fungi Aspergillus flavus and Penicillium sp. was evaluated. The cell-free supernatants (CFSs) of L. plantarum 124 showed strong inhibition against A. flavus and Penicillium sp., with MIC ranging from 11.25 to 22.5 mg mL−1. The significant loss of antifungal activity was observed by pH neutralisation, whereas either heat or proteinase K treatment showed no influence. The major compounds present in CFSs were lactic acid (49.57%) and acetic acid (25.85%). Others, such as 2,3-dimethyl-5-ethylpyrazine (6.07%), 2,5-dimethyl-3-propylpyrazine (2.10%), Cyclo(D-Leu-L-Pro) (2.30%), Ethyl 5-oxopyrrolidine-3-carboxylate (1.84%), and palmitic acid (1.80%), were detected in low relative amounts. As observed by electron microscopy, the CFSs secreted by L. plantarum 124 caused severe damage to cell membranes and organelles of A. flavus and Penicillium sp. In addition, L. plantarum 124 supernatant demonstrated no cytotoxicity on Vero cells, and it could extend the food product's shelf life from 7 days to 21 days without any commercial preservatives. These results demonstrate that antifungal metabolites secreted by L. plantarum 124 are candidates for the development of bio-preservative agents.
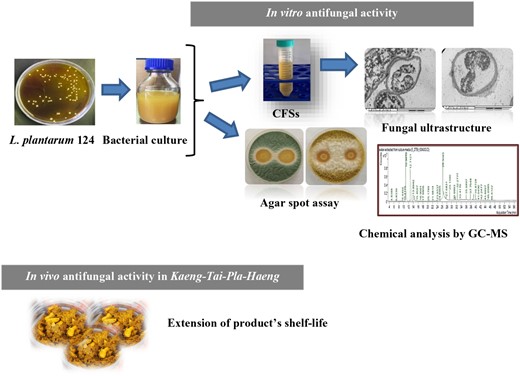
Introduction
The highly spicy cuisine, Kaeng-Tai-Pla, is a typical Thai curry widely consumed in the southern region of Thailand. This traditional curry is commonly processed and preserved into ready-to-eat form as an intermediate moisture product called Kaeng-Tai-Pla-Haeng. The main ingredients are fresh curry paste, grilled fish meat, and Tai-Pla sauce (salty, fermented fish entrails). Kaeng-tai-pla-haeng is cooked and processed by heating the mixture of all curry ingredients under continuous stirring until water activity (Aw) has reached approximately 0.85 or lower (Choopan et al., 2021). However, this product is highly susceptible to contamination by fungi of various genera, among which Penicillium and Aspergillus are the most commonly found fungi (Cheenkaew et al., 2020). Fungal spoilage can cause the deterioration of the odour, flavour, or texture of food ingredients, and strongly negative health impacts are possible from mycotoxins (Guimarães et al., 2018).
The common methods of food preservation to control fungal spoilage in food products are based on the use of chemical additives and physical treatments (Schnürer & Magnusson, 2005). However, physical methods such as irradiation or thermal treatments often cause a loss in nutrition, and can change the sensory characteristics of the food (Choopan et al., 2021). Meanwhile, chemical preservatives, such as sorbic acid, benzoic acid, and calcium propionate, are highly concerned regarding their negative impacts on human health at high concentrations (Adebayo & Aderiye, 2011). Therefore, the need to prevent fungal spoilage of foods has challenged scientists to develop efficient and safe bio-preservatives for humans as alternative treatments.
The application of bio-preservative methods has received significant interest in the last decade due to consumer demands. Among the most suitable candidates for bio-protective cultures in food, lactic acid bacteria (LAB), widely used as probiotic strains, are of great interest for suppressing spoilage fungi (Luz et al., 2017; Chen et al., 2021). The mechanism of LAB antifungal action may result from competitive growth, low pH and acidity, and the secretion of antifungal compounds. L. fermentum YML014 showed strong antifungal activity against the spoilage fungi of A. flavus and P. expansum (Adedokun et al., 2015). L. plantarum AR524 strongly inhibited the fungal pathogen Fusarium graminearum (Cao et al., 2021). Moreover, L. plantarum UM55 could produce multiple organic compounds to inhibit the growth of A. flavus MUM 17.14 (Guimarães et al., 2018). So far, the application of antifungal metabolites derived from LAB to control spoilage fungi in Thai food products has not yet been reported.
Hence, this research was intended to evaluate the antifungal activity of L. plantarum 124, isolated from a fermented rice noodle routinely reactivated in MRS broth (Himedia, India) at 37 °C for 18 h before use, which can inhibit the growth of spoilage fungi like A. flavus and Penicillium sp., and to test its application as a bio-preservative for Kaeng-Tai-Pla-Haeng during processing. Additionally, changes in the characteristics of Kaeng-Tai-Pla-Haeng during storage were also determined.
Materials and methods
Growth media and condition of microorganisms
L. plantarum 124, previously isolated from a fermented rice noodle, was routinely reactivated in MRS broth (Himedia, India) at 37 °C for 18 h before use. The fungal strains used in this study were Aspergillus flavus TP12 and Penicillium sp. TP7, previously isolated from the spoilage of Thai curry paste products. These strains were cultured on Potato Dextrose Agar slants (PDA; Himedia, India) at 35 °C for 7 days. After adding sterile 0.1% (v/v) Tween 80, the fungal spores were harvested by robustly shaking slants, and the spore suspension was adjusted to 106 spores mL−1 using a hemacytometer (BOECO, Hamburg, Germany).
Antifungal efficacy of L. plantarum 124 using an agar spot assay
The antifungal efficacy of L. plantarum 124 against Penicillium sp. TP7 and Aspergillus flavus TP12 was assayed (Magnusson et al., 2003). Briefly, 10 μL of culture broth was dropped onto the surface of MRS agar and incubated at 37 °C. After incubation for 48 h, the agar plates were overlaid with PDA soft agar (0.8% agar) with a final mould spore concentration of 106 spores mL−1. The measurement was conducted after 48 h by assessing the zone of inhibition around the LAB spots. The inhibition tests were performed in triplicate over two separate runs.
Antifungal properties of L. plantarum 124 supernatant
Lactiplantibacillus plantarum 124 was grown in MRS broth at 37 °C for 48 h. To obtain CFSs, the cells were centrifuged at 4 °C and then sterilised using a sterile membrane with a 0.22 mm pore size. Subsequently, the sterile supernatant was concentrated 10-fold by freeze-drying using a freeze-dryer (FTS System, Waltham, MA, USA) for 15–18 h to produce a supernatant powder. The antifungal activity of sterile liquid or freeze-dried supernatant of L. plantarum 124 was assayed using a broth microdilution assay as described by Sangmanee & Hongpattarakere (2014).
Assaying of the antifungal activities of L. plantarum 124
To investigate pH effects, the pH of the LAB supernatant was adjusted to 4.0, 5.0, 6.0, and 7.0 using 1 M NaOH. For heat treatment, the LAB supernatant was then heated for 30 min at 63, 100, and 121 °C for and immediately cooled to room temperature. Finally, at a final concentration of 0.1 mg mL−1, proteinase K, pepsin, and catalase were added to the neutralised freeze-dried supernatant (pH 7.0) and incubated at 37 °C for 2 h before heating at 80 °C for 2 min. The antifungal activities of all samples were evaluated using a broth microdilution assay. The untreated LAB supernatant was used as a control (Sangmanee & Hongpattarakere, 2014). All the assays were conducted in triplicate over two separate runs.
Cytotoxicity assay of L. plantarum 124 supernatant
The cytotoxicity of L. plantarum 124 supernatant was tested in the Monkey Kidney Epithelial (Vero) cell line. The cells were grown in Dulbecco's modified Eagle's medium (DMEM) as described by Go et al. (2021). Vero cells were seeded into a 96-well microplate (4 × 104 cells/well) and cultured overnight. Subsequently, the cell culture medium was replaced with serum-free medium and maintained for 24 h. After removing the medium, the Vero cells were co-cultured with medium containing L. plantarum 124 its MIC, or fresh MRS, and then incubated for another 24 h. The blank control wells were prepared using the serum-free medium with the same volume. After incubation, the cell viability was assessed using the MTT assay (Chuah et al., 2019). MTT solution (100 μL mL−1) was added to each well. After 2 h of incubation at 37 °C, 100 μL of dimethyl sulfoxide (DMSO) were added to dissolve the blue crystals, and absorbance was read. The optical density was measured at 570 nm using a microplate reader. The assay was repeated at least five times.
Identification of antifungal metabolites by GC-MS
The antifungal substances produced by L. plantarum 124 grown in MRS broth were assessed using GC-MS analysis. After 48 h of incubation, the cells of L. plantarum 124 were separated by centrifuging at 11 200 g for 10 min at 4 °C in order to obtain the clear supernatant. Subsequently, the sample was ethylated as previously described by Sangmanee & Hongpattarakere (2014) and then subjected to GC-MS analysis. The analysis was carried out using a TRACE GC Ultra Gas Chromatograph in conjunction with an ISQ Single Quadrupole Mass Spectrometer (Thermo Scientific Inc., Waltham, MA, USA). The retention periods and fragmentation patterns of mass spectra were compared to those documented in the literature as well as the GC/MS system's Wiley 275.L data library.
TEM analysis
The fungal spore suspensions were transferred to the PDB medium containing the freeze-dried supernatant at its MIC, or 0.3% lactic acid. After incubation at 30 °C for 3 days, the fungal materials were processed for TEM according to Tian et al. (2012). Briefly, the treated fungal mycelia were pre-fixed in 2.5% glutaraldehyde containing 0.1 M phosphate buffer (pH 7.2) at room temperature for 3 h before TEM analysis. The samples were washed three times with 0.1 M phosphate buffer and then post-fixed in 1% osmium tetroxide (OsO4). The post-fixed cells were dehydrated in a graded ethanol series, then processed in graded propylene oxide with araldite, and finally embedded in araldite. The polymerisation was carried out to generate a specimen block at 70–80 °C overnight. The specimen blocks were subsequently processed in accordance with Rammanee & Hongpattarakere (2011). The fungal morphology was observed under TEM (JEM-2010; JEOL Ltd., Tokyo, Japan).
In vivo study of antifungal activity of L. plantarum 124 supernatant
Kaeng-Tai-Pla-Haeng was prepared by the red curry paste mixed with shrimp paste and mackeral meat, then heated with water together with Tai pla sauce until it boiled and let the water evaporate until the water activity was approximately 0.85. (Choopan et al., 2021), and then divided into four groups. A freeze-dried supernatant of L. plantarum 124 at its MIC was supplemented in Kaeng-Tai-Pla-Haeng, which was expected to inhibit fungal spoilage growth. The control groups were treated with sterile distilled water and commercial preservatives (sodium benzoate and lactic acid). All products were placed in a plastic cup, which was covered with a lid, and then analysed for quality according to Thai Community Product Standards (TCPS, 2018). All treatments were determined in triplicate.
Water activity, Aw, and pH of Kaeng-Tai-Pla-Haeng
The products of Kaeng-Tai-Pla-Haeng were measured for pH levels using a digital pH meter (Sartorius, Goettingen, Germany) by inserting the probe at three random sites in the sample, in accordance with AOAC (2000). Aw was measured using a water activity meter (Aqua Lab 4 TE, Washington, USA) according to the manufacturer's instructions. Moisture content was measured following standard procedures (AOAC, 2000).
Microbiological counts
The products of Kaeng-Tai-Pla-Haeng were determined for total viable counts (TVCs), yeast, and mould, as well as the pathogenic bacteria recommended by Thai Community Product Standards (TCPS, 2018). Ten grams of each sample were homogenised in 90 mL of 0.85% NaCl in a stomacher bag using a stomacher machine for 2 min at medium speed. Aliquots of 100 μL of appropriate dilutions were spread on plate count agar and incubated at 37 °C for 1 day for the TVC, on PDA with pH 3.5, and incubated at 30 °C for 3 days for yeast and mould. Eosin methylene blue agar was used for determining the Escherichia coli count after being incubated at 37 °C for 24 h. Perfringens agar base was used for the enumeration of Clostridium perfringens after being incubated anaerobically at 35 °C for 3 days. Mannitol Egg Yolk Polymyxin (MYP) agar, Xylose Lysine Deoxycholate (XLD) agar, and Baird Parker agar containing EY Tellurite enrichment were used for determining Bacillus cereus, Salmonella spp., and Staphylococcus aureus, respectively, after incubation at 37 °C for 24 h (BAM, 2001). The experiments were run in three replications.
Assessment of product shelf life during storage
The products of Kaeng-Tai-Pla-Heang were sampled every 7 days to determine the microbiological counts of total bacteria, yeast, and mould and water activity (Aw), during storage at room temperature (25 °C–28 °C).
Statistical analysis
For statistical analysis, the SPSS software package for Windows (SPSS ver. 18; SPSS Inc., Chicago, USA) was used. The data was subjected to Anova, and the means were tested for significant differences using Duncan's multiple range test. The 95% confidence level (P < 0.05) was used to determine statistical significance.
Results and discussion
Antifungal activity of L. plantarum 124
The antifungal activity results are shown in Fig. 1. According to the findings, both spoilage from A. flavus TP12 and Penicillium sp. TP7 covered the entire plate in the absence of L. plantarum 124 (data not shown). The potential L. plantarum 124 antifungal levels measured by agar spot assay against A. flavus TP12 and Penicillium sp. TP7 were 19.8 and 38.6 mm, respectively. The current study's findings correlated with those of several researchers who tested Lactobacillus species comparable to those employed in this study. Lactobacillus strains may inhibit fungal growth rates by producing secondary metabolites such as organic acids, bacteriocins, and hydrogen peroxide (Sangmanee & Hongpattarakere, 2014). Luz et al. (2017) reported that Aspergillus parasiticus and Penicillium expansum were inhibited by L. plantarum CECT 749 through the production of organic acids and other low-molecular-weight compounds. L. plantarum HD1 isolated from kimchi was similarly effective against the bread microbial contaminants A. flavus and A. fumigatus (Ryu et al., 2014). Many strains of L. plantarum isolated from different food matrices have exerted strong inhibition on Fusarium culmorum CECT 2148, Penicillium expansum CECT 2278, and Penicillium chrysogenum CECT 2669 (Russo et al., 2017).
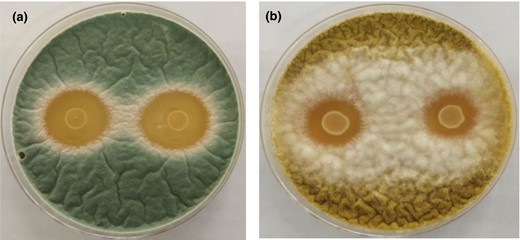
Antifungal activity of Lactobacillus plantarum 124 against Penicillium sp. TP7 (a) and Aspergillus flavus TP12 (b) in an agar spot assay.
The inhibitory activity of freeze-dried CFS from L. plantarum 124 against these fungi was also tested in a broth microdilution assay. At concentrations of 5.62, 11.25, and 22.52 mg mL−1, Penicillium sp. was more sensitive than A. flavus after 12 h of exposure (Fig. 2). Complete inhibition was observed with a concentration of the supernatant at 22.52 mg mL−1 after 24 h and 36 h exposures. Therefore, L. plantarum 124 supernatant at more than 23 mg mL−1 could effectively inhibit the growth of these two spoilage fungi. Similarly, inhibition of spoilage fungi by L. plantarum cell-free supernatant has been reported earlier (Russo et al., 2017; Guimarães et al., 2018). Quattrini et al. (2018) observed that CFS from L. plantarum ITEM 17215 significantly reduced the mycelial growth of Penicillium roqueforti, Mucor circinelloides, A. flavus, and Fusarium verticillioides.
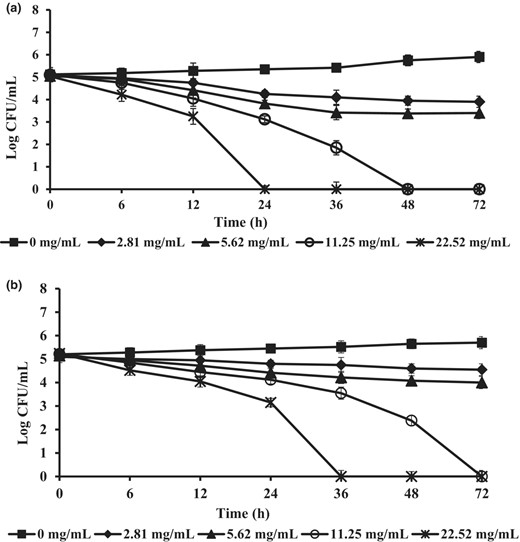
Antifungal activity of freeze-dried cell-free culture supernatant (CFS) from L. plantarum 124 at concentrations 0, 2.81, 5.62, 11.25, and 22.52 mg mL−1 on growth of Penicillium sp., (a) and A. flavus (b) tested in potato dextrose broth at 35 °C for 72 h. The data shown are the mean values of triplicate determinations ± standard deviation.
Characteristics of antifungal substances secreted by L. plantarum 124
The antifungal activity of the L. plantarum 124 supernatant against spoilage fungi was characterised as shown in Fig. 3. The findings show that pH treatment had a significant impact on the supernatant's antifungal activity. The inhibitory action against Penicillium sp. TP7 and A. flavus TP12 was dramatically reduced when the pH of the supernatant was more than 4, with MIC values of 11.25 mg mL−1 and 22.5 mg mL−1 increasing to 90 mg mL−1 and 180 mg mL−1, respectively (Fig. 3a). However, the control treatments, whose pH was adjusted by 1 M HCl, at the same pH did not show inhibition of the growth of Penicillium sp. TP7 and A. flavus TP12, even at a relatively low pH (data not shown). Sathe et al. (2007) reported the loss of inhibitory activity after neutralisation of CFS by highly antifungal strains of L. plantarum CUK501, which is consistent with our findings. The antifungal activity of the L. plantarum 124 supernatant remained unaltered after treatment at temperatures of 63 °C, 100 °C, and 121 °C for 30 min (P > 0.05) (Fig. 3b), showing that the active compounds in the supernatant were not heat sensitive. The temperature stability of antifungal substances in LAB fermentation supernatants is dependent on the specific types of substances. Crowley et al. (2012) noted that organic acids and other small-molecule antifungal substances usually exhibit high temperature stability and even extreme heat resistance. Finally, enzymatic action had no effect on the neutralised supernatant, indicating that no proteinaceous or hydrogen peroxide molecules were involved in the antifungal activity of L. plantarum 124 (Fig. 3c).

Effects of pH (a), temperature (b), and enzymatic digestion (c) on the antifungal activity of freeze-dried CFS from L. plantarum 124 against A. flavus and Penicillium sp. The MIC value (mg mL−1) is used to show antifungal effects. The data shown are the means ± SD (n = 3). Different letters indicate significant differences (P < 0.05).
Cytotoxic activity
The cytotoxicity of the supernatant produced by L. plantarum 124 at MIC values was investigated on Vero cells viability using an MTT assay. The MTT assay is regarded as a highly sensitive assay for evaluating cellular respiration, cell viability, and cytotoxicity because only living cells are able to create formazan products during this reaction. As shown in Fig. 4, the isolates did not affect the Vero cells viability after 24 h of incubation when compared with the control treatment. This suggests that the supernatant produced by L. plantarum 124 has been proven safe for application in food products as a bio-preservative. The result of the current findings was also consistent with the results of Chuah et al. (2019), who evaluated the postbiotic metabolites produced by Lactobacillus plantarum and revealed no cytotoxicity of probiotics. Fu et al. (2022) reported similar results: Enterococcus lactis JDM1 co-cultured with a human embryonic intestinal cell line (INT-407) did not affect cell viability within 24 h.
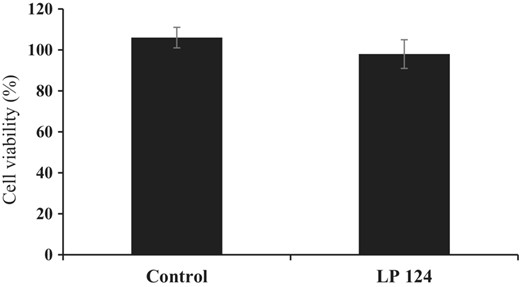
Cytotoxicity of freeze-dried CFS from L. plantarum 124 on African Monkey Kidney Epithelial cells. The cell viability was determined using an MTT assay. The values are shown as the mean ± standard deviation of three replicates.
Identification of antifungal compounds from L. plantarum 124
The antifungal substances of L. plantarum 124 supernatants were analysed by GC-MS, and the results are summarised in Table 1. The compounds detected at the highest concentrations in the CFS extract from L. plantarum 124 were lactic acid (49.57%), acetic acid (25.85%), 2,3-Dimethyl-5-ethylpyrazine (6.07%), 2,5-Dimethyl-3-propylpyrazine (2.10%), Cyclo (D-Leu-L-Pro) (2.30%), Ethyl 5-oxopyrrolidine-3-carboxylate (1.85%), and palmitic acid (1.80%). Among these, organic acids, including lactic acid and acetic acid, were clearly dominant. Thus, the inhibition by L. plantarum 124 of the growth of Penicillium sp. and A. flavus was mainly due to the production of lactic and acetic acids that act synergistically to limit cell growth in an acidic environment. In the study by Chen et al. (2021), multiple antifungal metabolites of weak acids such as lactic acid, acetic acid, propanoic acid, formic acid, tartaric acid, and succinic acid were produced by L. plantarum SL778. However, lactic acid may not be considered a major contributor to the antifungal activity of L. plantarum 124 at pH 4 and above. Only non-dissociated molecules of weak organic acids can easily flow through fungal cell membranes, resulting in low intracellular pH and abolishing protonmotive force (Guimarães et al., 2018). Because the pKa value of lactic acid is 3.8, the adjustment of the LAB supernatant to a pH above 4.0 can remove its inhibitory activity. Contrary to lactic acid, acetic acid, with a higher pKa of 4.76, may contribute to the antifungal effect of L. plantarum 124.
Identification of potential antifungal compounds in cell-free supernatant of L. plantarum 124
Retention time . | Compound . | Area (%) . | Formula . | Mw . |
---|---|---|---|---|
10.95 | Lactic acid | 49.57 | C3H6O3 | 90.08 |
12.62 | Trimethyl pyrazine | 0.20 | C7H10N2 | 122.17 |
12.71 | Acetic acid | 25.85 | C2H4O2 | 60.05 |
13.74 | 2,3-Dimethyl-5-ethylpyrazine | 6.07 | C8H12N2 | 136.19 |
15.47 | Leucic acid | 0.23 | C6H12O3 | 132.16 |
16.90 | Butyric acid | 0.19 | C4H8O2 | 88.11 |
23.93 | 3-Methylheptan-4-one | 0.17 | C8H16O | 128.21 |
26.68 | 2,5-Dimethyl-3-propylpyrazine | 2.10 | C9H14N2 | 150.22 |
31.47 | 4-Hydroxy-4-methyltetrahydro-2H-pyran-2-one | 0.53 | C6H10O3 | 130.14 |
32.28 | Ethyl 5-oxopyrrolidine-3-carboxylate | 1.85 | C7H11NO3 | 157.17 |
33.42 | 1,2-Benzenedicarboxylic acid | 0.13 | C8H6O4 | 166 |
34.12 | 3-Hydroxypyridine-2-carboxaldehyde | 0.23 | C6H5NO2 | 123.11 |
35.99 | Palmitic acid | 1.80 | C16H32O2 | 256.43 |
37.75 | Cyclo(D-Leu-L-Pro) | 2.30 | C11H18N2O2 | 210.27 |
38.58 | Stearic acid | 0.22 | C18H36O2 | 284.48 |
45.10 | Cyclo (Gly-L-Pro) | 0.52 | C7H10N2O2 | 154.17 |
Retention time . | Compound . | Area (%) . | Formula . | Mw . |
---|---|---|---|---|
10.95 | Lactic acid | 49.57 | C3H6O3 | 90.08 |
12.62 | Trimethyl pyrazine | 0.20 | C7H10N2 | 122.17 |
12.71 | Acetic acid | 25.85 | C2H4O2 | 60.05 |
13.74 | 2,3-Dimethyl-5-ethylpyrazine | 6.07 | C8H12N2 | 136.19 |
15.47 | Leucic acid | 0.23 | C6H12O3 | 132.16 |
16.90 | Butyric acid | 0.19 | C4H8O2 | 88.11 |
23.93 | 3-Methylheptan-4-one | 0.17 | C8H16O | 128.21 |
26.68 | 2,5-Dimethyl-3-propylpyrazine | 2.10 | C9H14N2 | 150.22 |
31.47 | 4-Hydroxy-4-methyltetrahydro-2H-pyran-2-one | 0.53 | C6H10O3 | 130.14 |
32.28 | Ethyl 5-oxopyrrolidine-3-carboxylate | 1.85 | C7H11NO3 | 157.17 |
33.42 | 1,2-Benzenedicarboxylic acid | 0.13 | C8H6O4 | 166 |
34.12 | 3-Hydroxypyridine-2-carboxaldehyde | 0.23 | C6H5NO2 | 123.11 |
35.99 | Palmitic acid | 1.80 | C16H32O2 | 256.43 |
37.75 | Cyclo(D-Leu-L-Pro) | 2.30 | C11H18N2O2 | 210.27 |
38.58 | Stearic acid | 0.22 | C18H36O2 | 284.48 |
45.10 | Cyclo (Gly-L-Pro) | 0.52 | C7H10N2O2 | 154.17 |
Identification of potential antifungal compounds in cell-free supernatant of L. plantarum 124
Retention time . | Compound . | Area (%) . | Formula . | Mw . |
---|---|---|---|---|
10.95 | Lactic acid | 49.57 | C3H6O3 | 90.08 |
12.62 | Trimethyl pyrazine | 0.20 | C7H10N2 | 122.17 |
12.71 | Acetic acid | 25.85 | C2H4O2 | 60.05 |
13.74 | 2,3-Dimethyl-5-ethylpyrazine | 6.07 | C8H12N2 | 136.19 |
15.47 | Leucic acid | 0.23 | C6H12O3 | 132.16 |
16.90 | Butyric acid | 0.19 | C4H8O2 | 88.11 |
23.93 | 3-Methylheptan-4-one | 0.17 | C8H16O | 128.21 |
26.68 | 2,5-Dimethyl-3-propylpyrazine | 2.10 | C9H14N2 | 150.22 |
31.47 | 4-Hydroxy-4-methyltetrahydro-2H-pyran-2-one | 0.53 | C6H10O3 | 130.14 |
32.28 | Ethyl 5-oxopyrrolidine-3-carboxylate | 1.85 | C7H11NO3 | 157.17 |
33.42 | 1,2-Benzenedicarboxylic acid | 0.13 | C8H6O4 | 166 |
34.12 | 3-Hydroxypyridine-2-carboxaldehyde | 0.23 | C6H5NO2 | 123.11 |
35.99 | Palmitic acid | 1.80 | C16H32O2 | 256.43 |
37.75 | Cyclo(D-Leu-L-Pro) | 2.30 | C11H18N2O2 | 210.27 |
38.58 | Stearic acid | 0.22 | C18H36O2 | 284.48 |
45.10 | Cyclo (Gly-L-Pro) | 0.52 | C7H10N2O2 | 154.17 |
Retention time . | Compound . | Area (%) . | Formula . | Mw . |
---|---|---|---|---|
10.95 | Lactic acid | 49.57 | C3H6O3 | 90.08 |
12.62 | Trimethyl pyrazine | 0.20 | C7H10N2 | 122.17 |
12.71 | Acetic acid | 25.85 | C2H4O2 | 60.05 |
13.74 | 2,3-Dimethyl-5-ethylpyrazine | 6.07 | C8H12N2 | 136.19 |
15.47 | Leucic acid | 0.23 | C6H12O3 | 132.16 |
16.90 | Butyric acid | 0.19 | C4H8O2 | 88.11 |
23.93 | 3-Methylheptan-4-one | 0.17 | C8H16O | 128.21 |
26.68 | 2,5-Dimethyl-3-propylpyrazine | 2.10 | C9H14N2 | 150.22 |
31.47 | 4-Hydroxy-4-methyltetrahydro-2H-pyran-2-one | 0.53 | C6H10O3 | 130.14 |
32.28 | Ethyl 5-oxopyrrolidine-3-carboxylate | 1.85 | C7H11NO3 | 157.17 |
33.42 | 1,2-Benzenedicarboxylic acid | 0.13 | C8H6O4 | 166 |
34.12 | 3-Hydroxypyridine-2-carboxaldehyde | 0.23 | C6H5NO2 | 123.11 |
35.99 | Palmitic acid | 1.80 | C16H32O2 | 256.43 |
37.75 | Cyclo(D-Leu-L-Pro) | 2.30 | C11H18N2O2 | 210.27 |
38.58 | Stearic acid | 0.22 | C18H36O2 | 284.48 |
45.10 | Cyclo (Gly-L-Pro) | 0.52 | C7H10N2O2 | 154.17 |
In addition to weak organic acids, the compound pyrazine (2,3-dimethyl and 2,5-dimethyl) is found in abundance in L. plantarum for the first time. This compound was identified for its broad-spectrum antifungal activity against endophytic bacteria (Gao et al., 2017). However, the antifungal activity of pyrazine (2,5-dimethyl) is rarely reported, especially among inhibitors of spoilage fungi such as Penicillium sp. and A. flavus. Several cyclic dipeptides, such as cyclo (Gly-L-Leu), cyclo (L-Phe-L-Pro), and cyclo (L-Phe-trans-4-OHL-Pro) were identified as peptides related to antifungal activity (Magnusson et al., 2003). Ström et al. (2002) reported that L. plantarum MiLAB 393 produced various antifungal substances such as cyclo (L-phe-L-pro), cyclo (L-phe-trans-4-OH-L-pro), and phenyllactic acid. Long chain fatty acids, particularly palmitic acid and stearic acid, were also present in the fermentation broth of L. plantarum 124. Palmitic acid has more antifungal action than oleic acid (Walters et al., 2004). According to Liu et al. (2008), fatty acids cause fungal membrane rupture and, in some cases, suppress mycelial development.
The identified antifungal compounds from L. plantarum 124 support previous reports indicating that multiple components function synergistically, leading to effective antifungal activity. It is noteworthy that L. plantarum 124 did not release 3-phenyllactic acid (PLA), a well-known LAB antifungal component. Our results indicate that the antifungal components of L. plantarum 124 are different from those previously reported. The active antifungal compound from L. plantarum was identified as 3-phenyllactic acid (Cao et al., 2021). Sangmanee & Hongpattarakere (2014) found lactic acid, 2-butyl-4-hexyloctahydro-1H-indene, 3-phenyllactic acid, oleic acid, and palmitic acid as the major metabolites of L. plantarum K35 by GC-MS. Gerez et al. (2013) also showed that the secretion of acetic acid, phenyllactic acid, and lactic acid by L. plantarum inhibited the growth of six filamentous fungi, including Aspergillus niger. L. plantarum's antifungal profile is strain-specific as well as dependent on nutrient availability and growth conditions.
Effects of L. plantarum 124 supernatants on the fungal ultrastructure
The effects of the L. plantarum 124 supernatant on the ultrastructure of the target fungi were revealed by TEM imaging, as shown in Fig. 5. The control A. flavus (Fig. 5a) and Penicillium sp. (Fig. 5b) showed regular shapes, and the cell wall, cell membrane, and organelles were clearly visible. The main organelles, such as mitochondria, vacuoles, and the nucleus, have normal and uniform structures. In contrast, the severe destruction of the cell membrane and organelle structures of A. flavus (Fig. 5c) and Penicillium sp. (Fig. 5d) significantly changed when the cells were treated with the L. plantarum 124 supernatant. The plasma membranes were completely detached from the cell wall, leading to a marked depletion of the cytoplasmic content with the formation of small lomasomes. The destruction and degeneration of various fungal organelles, such as the loss of mitochondrial cristae and fusion of the nucleus, are clearly seen. The lactic acid-treated cells displayed that the plasma membranes of Penicillium sp. (Fig. 5e) and A. flavus (Fig. 5f) remained intact with a smooth surface, and the cytoplasmic matrix was abundant. This result supports the hypothesis that many antifungal compounds produced by L. plantarum 124 are involved in the antifungal action, as evidenced by the numerous target sites detected by TEM on A. flavus and Penicillium sp.
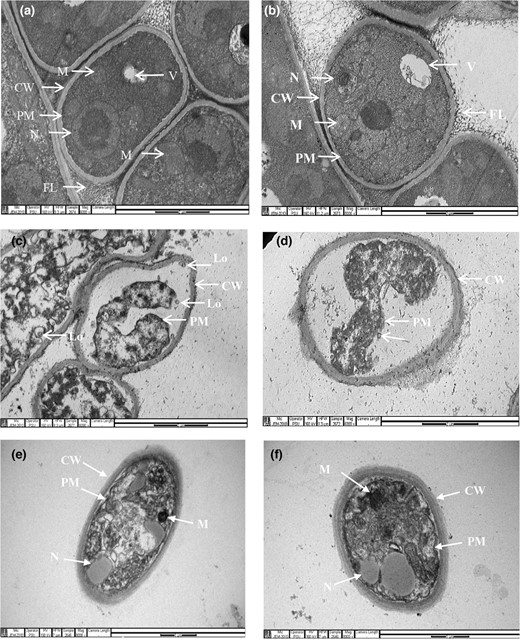
Transmission electron micrographs (TEM) of Penicillium sp. (a, c) and A. flavus (b, d) with or without treatment with freeze-dried CFS from L. plantarum 124 at MIC (mg mL−1). Non-treated Penicillium sp. (a) and A. flavus (b) with normal fibrillar layer (FL), cell wall (CW), plasma membrane (PM), nucleus (N), vacuole (V), and mitochondria (M). The CFS-treated mycelia show a thickened fibrillar layer (c, d) and complete detachment and disruption of plasma membranes (thick arrows in c and d), leading to a loss of cytoplasmic content with the formation of small lomasomes (Lo). The plasma membranes of Penicillium sp. and A. flavus exposed to 0.3% lactic acid at pH 4.0 (e, f) show uniformity with a smooth surface. The cytoplasmic matrix was abundant.
As previously reported, the antifungal mechanism of weak organic acids is hypothesised to focus on the plasma membrane (Freese et al., 1973; Cao et al., 2021; Li et al., 2021). This study corroborates the concept that the low pH and the multiple acids produced by L. plantarum 124 could destroy the plasma membrane, resulting in notable morphological changes in fungal mycelia and the formation of membrane-bound vesicles inside the cells. Fatty acids are well-known as membrane-targeting antimicrobials. Palmitic acid is inserted into the lipid bilayer through ATP-induced sequestration of calcium, resulting in a decrease in phospholipid content (Herbette et al., 1984; Poornachandra Rao et al., 2019). Cyclic dipeptides' antifungal action was thought to be linked to cell wall destruction (Zhao et al., 2019; Garnier et al., 2020).
The effects of antifungal compounds from L. plantarum 124 on the quality characteristics of Kaeng-Tai-Pla-Haeng
All products (before storage) had pH, water activity (Aw), and moisture content as shown in Table 2. Our study showed that Kaeng-Tai-Pla-Haeng had an Aw below 0.85, which is in the range required by TCPS (2018). However, with its Aw, this sample may easily be spoiled by mould; it is in a suitable condition for mould growth (Cheenkaew et al., 2020). The inorganic contaminants, including Pb, As, Cd, and Hg, were also analysed in this study to confirm that this product is acceptable according to the standard TCPS (2018). As indicated in Table 2, the results confirmed that all Kaeng-Tai-Pla-Haeng products contained acceptable levels of inorganic contaminants.
Chemical composition, physical properties, and microbial population of Kaeng-Tai-Pla-Haeng
Parameter . | Values . | |||
---|---|---|---|---|
Control . | Tai Pla + lactic acid . | Tai Pla + sodium benzoate . | Tai Pla + CFSs . | |
Chemical composition | ||||
Moisture (%) | 49.27 ± 0.00 | 49.22 ± 0.00 | 49.25 ± 0.01 | 49.29 ± 0.01 |
pH | 5.88 ± 0.00 | 5.86 ± 0.00 | 5.87 ± 0.00 | 5.87 ± 0.00 |
Water activity (Aw) | 0.80 ± 0.00 | 0.81 ± 0.00 | 0.82 ± 0.00 | 0.81 ± 0.00 |
Aflatoxin (μg kg−1) | ND | ND | ND | ND |
Inorganic contaminants | ||||
Pb (mg kg−1) | 0.056 ± 0.02 | 0.055 ± 0.02 | 0.056 ± 0.01 | 0.056 ± 0.01 |
As (mgkg−1) | 0.130 ± 0.01 | 0.131 ± 0.01 | 0.131 ± 0.01 | 0.132 ± 0.02 |
Cd (mgkg−1) | 0.012 ± 0.01 | 0.012 ± 0.01 | 0.013 ± 0.01 | 0.013 ± 0.01 |
Hg (mgkg−1) | ND | ND | ND | ND |
Microbial populations | ||||
Total viable count (TVC) | 1.62 × 102 CFU g−1 | 1.57 × 102 CFU g−1 | 1.48 × 102 CFU g−1 | 1.5 × 102 CFU g−1 |
Yeast and Mould | 10 CFU g−1 | 10 CFU g−1 | 7 CFU g−1 | 5 CFU g−1 |
Salmonella spp. | ND | ND | ND | ND |
S. aureus | <10 CFU g−1 | <10 CFU g−1 | <10 CFU g−1 | <10 CFU g−1 |
B. cereus | <10 × 103 CFU g−1 | <10 × 103 CFU g−1 | <10 × 103 CFU g−1 | <10 × 103 CFU g−1 |
C. perfringens | <10 CFU g−1 | <10 CFU g−1 | <10 CFU g−1 | <10 CFU g−1 |
E. coli | <3 CFU g−1 | <3 CFU g−1 | <3 CFU g−1 | <3 CFU g−1 |
Parameter . | Values . | |||
---|---|---|---|---|
Control . | Tai Pla + lactic acid . | Tai Pla + sodium benzoate . | Tai Pla + CFSs . | |
Chemical composition | ||||
Moisture (%) | 49.27 ± 0.00 | 49.22 ± 0.00 | 49.25 ± 0.01 | 49.29 ± 0.01 |
pH | 5.88 ± 0.00 | 5.86 ± 0.00 | 5.87 ± 0.00 | 5.87 ± 0.00 |
Water activity (Aw) | 0.80 ± 0.00 | 0.81 ± 0.00 | 0.82 ± 0.00 | 0.81 ± 0.00 |
Aflatoxin (μg kg−1) | ND | ND | ND | ND |
Inorganic contaminants | ||||
Pb (mg kg−1) | 0.056 ± 0.02 | 0.055 ± 0.02 | 0.056 ± 0.01 | 0.056 ± 0.01 |
As (mgkg−1) | 0.130 ± 0.01 | 0.131 ± 0.01 | 0.131 ± 0.01 | 0.132 ± 0.02 |
Cd (mgkg−1) | 0.012 ± 0.01 | 0.012 ± 0.01 | 0.013 ± 0.01 | 0.013 ± 0.01 |
Hg (mgkg−1) | ND | ND | ND | ND |
Microbial populations | ||||
Total viable count (TVC) | 1.62 × 102 CFU g−1 | 1.57 × 102 CFU g−1 | 1.48 × 102 CFU g−1 | 1.5 × 102 CFU g−1 |
Yeast and Mould | 10 CFU g−1 | 10 CFU g−1 | 7 CFU g−1 | 5 CFU g−1 |
Salmonella spp. | ND | ND | ND | ND |
S. aureus | <10 CFU g−1 | <10 CFU g−1 | <10 CFU g−1 | <10 CFU g−1 |
B. cereus | <10 × 103 CFU g−1 | <10 × 103 CFU g−1 | <10 × 103 CFU g−1 | <10 × 103 CFU g−1 |
C. perfringens | <10 CFU g−1 | <10 CFU g−1 | <10 CFU g−1 | <10 CFU g−1 |
E. coli | <3 CFU g−1 | <3 CFU g−1 | <3 CFU g−1 | <3 CFU g−1 |
Mean ± SD from triplicate determinations.
ND, not detectable.
Chemical composition, physical properties, and microbial population of Kaeng-Tai-Pla-Haeng
Parameter . | Values . | |||
---|---|---|---|---|
Control . | Tai Pla + lactic acid . | Tai Pla + sodium benzoate . | Tai Pla + CFSs . | |
Chemical composition | ||||
Moisture (%) | 49.27 ± 0.00 | 49.22 ± 0.00 | 49.25 ± 0.01 | 49.29 ± 0.01 |
pH | 5.88 ± 0.00 | 5.86 ± 0.00 | 5.87 ± 0.00 | 5.87 ± 0.00 |
Water activity (Aw) | 0.80 ± 0.00 | 0.81 ± 0.00 | 0.82 ± 0.00 | 0.81 ± 0.00 |
Aflatoxin (μg kg−1) | ND | ND | ND | ND |
Inorganic contaminants | ||||
Pb (mg kg−1) | 0.056 ± 0.02 | 0.055 ± 0.02 | 0.056 ± 0.01 | 0.056 ± 0.01 |
As (mgkg−1) | 0.130 ± 0.01 | 0.131 ± 0.01 | 0.131 ± 0.01 | 0.132 ± 0.02 |
Cd (mgkg−1) | 0.012 ± 0.01 | 0.012 ± 0.01 | 0.013 ± 0.01 | 0.013 ± 0.01 |
Hg (mgkg−1) | ND | ND | ND | ND |
Microbial populations | ||||
Total viable count (TVC) | 1.62 × 102 CFU g−1 | 1.57 × 102 CFU g−1 | 1.48 × 102 CFU g−1 | 1.5 × 102 CFU g−1 |
Yeast and Mould | 10 CFU g−1 | 10 CFU g−1 | 7 CFU g−1 | 5 CFU g−1 |
Salmonella spp. | ND | ND | ND | ND |
S. aureus | <10 CFU g−1 | <10 CFU g−1 | <10 CFU g−1 | <10 CFU g−1 |
B. cereus | <10 × 103 CFU g−1 | <10 × 103 CFU g−1 | <10 × 103 CFU g−1 | <10 × 103 CFU g−1 |
C. perfringens | <10 CFU g−1 | <10 CFU g−1 | <10 CFU g−1 | <10 CFU g−1 |
E. coli | <3 CFU g−1 | <3 CFU g−1 | <3 CFU g−1 | <3 CFU g−1 |
Parameter . | Values . | |||
---|---|---|---|---|
Control . | Tai Pla + lactic acid . | Tai Pla + sodium benzoate . | Tai Pla + CFSs . | |
Chemical composition | ||||
Moisture (%) | 49.27 ± 0.00 | 49.22 ± 0.00 | 49.25 ± 0.01 | 49.29 ± 0.01 |
pH | 5.88 ± 0.00 | 5.86 ± 0.00 | 5.87 ± 0.00 | 5.87 ± 0.00 |
Water activity (Aw) | 0.80 ± 0.00 | 0.81 ± 0.00 | 0.82 ± 0.00 | 0.81 ± 0.00 |
Aflatoxin (μg kg−1) | ND | ND | ND | ND |
Inorganic contaminants | ||||
Pb (mg kg−1) | 0.056 ± 0.02 | 0.055 ± 0.02 | 0.056 ± 0.01 | 0.056 ± 0.01 |
As (mgkg−1) | 0.130 ± 0.01 | 0.131 ± 0.01 | 0.131 ± 0.01 | 0.132 ± 0.02 |
Cd (mgkg−1) | 0.012 ± 0.01 | 0.012 ± 0.01 | 0.013 ± 0.01 | 0.013 ± 0.01 |
Hg (mgkg−1) | ND | ND | ND | ND |
Microbial populations | ||||
Total viable count (TVC) | 1.62 × 102 CFU g−1 | 1.57 × 102 CFU g−1 | 1.48 × 102 CFU g−1 | 1.5 × 102 CFU g−1 |
Yeast and Mould | 10 CFU g−1 | 10 CFU g−1 | 7 CFU g−1 | 5 CFU g−1 |
Salmonella spp. | ND | ND | ND | ND |
S. aureus | <10 CFU g−1 | <10 CFU g−1 | <10 CFU g−1 | <10 CFU g−1 |
B. cereus | <10 × 103 CFU g−1 | <10 × 103 CFU g−1 | <10 × 103 CFU g−1 | <10 × 103 CFU g−1 |
C. perfringens | <10 CFU g−1 | <10 CFU g−1 | <10 CFU g−1 | <10 CFU g−1 |
E. coli | <3 CFU g−1 | <3 CFU g−1 | <3 CFU g−1 | <3 CFU g−1 |
Mean ± SD from triplicate determinations.
ND, not detectable.
The microbial counts and some pathogens of Kaeng-Tai-Pla-Haeng were determined based on regulations in TCPS (2018). Each sample had a total viable count (TVC) in the range of 1.48 to1.62 × 102 CFU g−1 sample (Table 2). Normally, microorganisms in food containing no more than <105 CFU g−1 sample are considered safe (BAM, 2001). Food microbial development is linked to Aw (Frazier & Westhoff, 1988). Most microorganisms can grow with a minimum Aw of 0.90. Moreover, the yeast and mould counts of all products (5–7 CFU g−1 sample), as well as the amounts of all pathogens (Table 2), are in the acceptable ranges as regulated by TCPS (2018). This indicates that our product had quality characteristics, especially as regards microbiological standards, adequate for consumer safety. However, the numbers of microorganisms, especially yeast and mould, can increase during storage. This can have a direct impact on consumer safety as well as the shelf life of Kaeng-Tai-Pla-Haeng.
The effects of antifungal compounds from L. plantarum 124 on Aw and microbial population of Kaeng-Tai-Pla-Haeng during storage at room temperature
Kaeng-Tai-Pla-Haeng at day 0 of storage has a water activity of Aw = 0.81 (Table 2). There were no changes in Aw in any of the samples during the 21 days of storage (P > 0.05), as shown in Fig. 6a. As regulated by TCPS (2018), the Aw of Kaeng-Tai-Pla-Haeng should not exceed 0.85. The initial total viable count (TVC) of Kaeng-Tai-Pla-Haeng at day 0 was 1.5 × 102 CFU g−1, as shown in Table 2. TVC in the control treatment (without any preservative) continuously increased with storage time, especially during the first 3 days of storage (Fig. 6b). On day 7 of storage, the TVC of this sample was changed to the acceptable range regulated by TCPS (2018). A similar trend was observed in Kaeng-Tai-Pla-Haeng, which contains 0.3% lactic acid. TVC in cases supplemented with antifungal compounds from L. plantarum 124% and 0.3% sodium benzoate slightly increased until 21 days of storage (Fig. 6b). This means that the shelf life of Kaeng-Tai-Pla-Haeng supplemented with antifungal compounds was extended from 7 to 21 days compared to the control treatment. Choopan et al. (2021) reported that free amino acids and other soluble non-nitrogenous substances derived from Tai-Pla (fermented fish viscera) sauce and fermented shrimp paste, as sources of protein, could serve as nutrients supporting microorganism growth until 1 × 104 CFU g−1 sample.
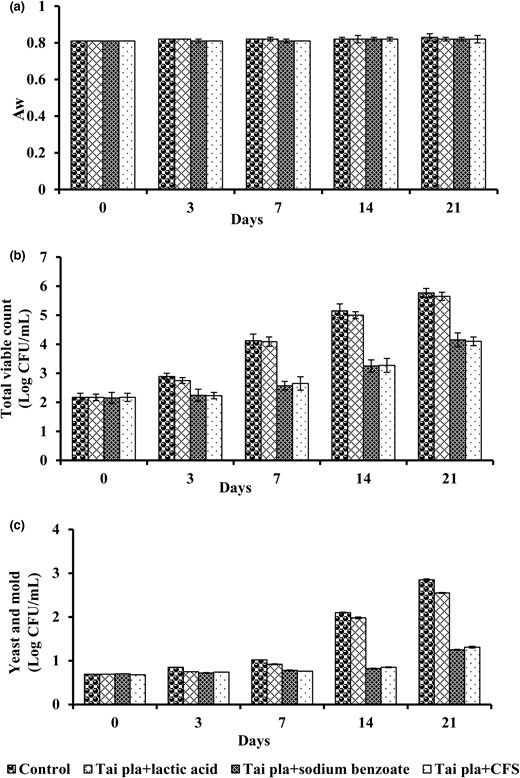
Time profiles of Aw (a), total viable count (b), and yeast and mould (c) in Kaeng-Tai-Pla-Haeng during storage at room temperature (25 °C). Data are given as means ± SD (n = 3). Columns with different lowercase letters within the same panel are significantly different (P < 0.05) according to Duncan's multiple range test.
The yeast and mould counts are a good indicator of Kaeng-Tai-Pla-Haeng's shelf life. Based on the standard regulations in TCPS (2018), the yeast and mould count should not exceed 100 CFU g−1 sample. Increased yeast and mould counts in the control and 0.3% lactic acid treatments were assessed every 3 days during the storage, and they exceeded the acceptable limit on day 7 (Fig. 6c). Therefore, Kaeng-Tai-Pla-Haeng stored in plastic cups with lids, which is the traditional packaging, spoils within 7 days in ambient storage. No yeast or mould colonies were found in the other treatments until 14 days of storage, and the count exceeded the acceptable limit on day 21 (Fig. 6c). The extended shelf life of
Kaeng-Tai-Pla-Haeng may be attributed to the inhibitory metabolites produced by L. plantarum 124. These findings agree with the report of Abouloifa et al. (2021), who found that CFS from L. plantarum S61 delayed the growth of P. digitatum in tomato puree for 15 days. Luz et al. (2019) found that bread fermented with L. reuteri and L. brevis extended shelf life by 2 and 4 days, respectively, when compared to control bread without additions.
Conclusion
L. plantarum 124, isolated from fermented food, displayed strong antifungal activity against the spoilage fungi Penicillium sp. and A. flavus. Multiple antifungal compounds were analysed and identified from the supernatant of L. plantarum 124. The antifungal mechanisms of these components were associated with overall morphological changes in these fungal strains, destroyed plasma membranes, and formed membrane bound vesicles inside the cells. The application of antifungal metabolites produced by L. plantarum 124 extended the shelf life of Kaeng-Tai-Pla-Haeng. Therefore, CFS from L. plantarum 124, for replacing traditional chemical preservatives, is a promising candidate bio-preservative for human food or animal feed.
Acknowledgement
Our great appreciation is extended to Assoc. Prof.Dr. Tipparat Hongpattarakere for editing the manuscript.
Author contributions
Pochanart Kanjan: Conceptualization (lead); data curation (equal); formal analysis (equal); methodology (equal); supervision (lead); writing – original draft (lead); writing – review and editing (lead). Phat Sakpetch: Data curation (equal); formal analysis (lead); methodology (equal); software (equal).
Funding
The authors are grateful to the Halal Institute of Prince of Songkla University (Grant No. SAT6201056S) for their financial support.
Conflict of interest
The authors declare no competing interests.
Ethical approval
Ethics approval was not required for this research.
Peer review
The peer review history for this article is available at https://www.webofscience.com/api/gateway/wos/peer-review/10.1111/ijfs.16656.
Data availability statement
Data will be available upon request to the corresponding author.
References
This paper reported that Lactobacillus plantarum (L. plantarum) AR524 exhibited strong inhibitory activity against F. graminearum growth in a pH-dependent manner. It helped to support our statement that the inhibitory action against Penicillium sp. TP7 and A. flavus TP12 was dramatically reduced when the pH of the supernatant was more than 4 (freeze-dried CFS).
This article studied the effect of Postbiotic metabolites produced by Lactobacillus plantarum strains exert selective cytotoxicity effects on cancer cells. It was cited for providing support for the parameter determination and result analyses in the cytotoxicity.
This article is important for this manuscript. Because the method and result analysis of ultrastructure alterations in fungal strains were done according to this article.
This article is important for this manuscript. Because the method of identification of antifungal metabolites by GC-MS was done according to this article.