-
PDF
- Split View
-
Views
-
Cite
Cite
Fengjun Wang, Ling Zhu, Hadiatullah Hadiatullah, Zhaofei Li, Jing He, Yunping Yao, Guozhong Zhao, Enhancing flavour with non-Saccharomyces during vinegar alcoholisation: a mechanism study, International Journal of Food Science and Technology, Volume 58, Issue 10, October 2023, Pages 5001–5008, https://doi.org/10.1111/ijfs.16584
- Share Icon Share
Abstract
The effects of mixed fermentation of non-Saccharomyces (Pichia fabianii, Kluyveromyces thermotolerans, and Saccharomycopsis fibuligera) with Saccharomyces cerevisiae on the physicochemical indexes and flavour of rice vinegar fermentation were studied. The mixed fermentation increased the activity of β-glucosidase and esterification enzyme. The β-glucosidase activity of P. fabianii was 1.6 times higher than that of S. cerevisiae alone, and the esterification enzyme activity was increased by about four times. Compared with the single fermentation of S. cerevisiae, the mixed fermentation of P. fabianii and S. cerevisiae increased the type and content of aromatic esters and produced ethyl acetate and phenethyl acetate. The mixed fermentation of K. thermotolerans and S. cerevisiae made an outstanding contribution to produce higher alcohols. Among them, the content of isobutanol and phenylethanol increased by nearly ten times; the content of isoamylol increased by more than eight times. S. fibuligera provides a unique flavour of phenols because of 2-methoxy-4-ethylphenol and 2-methoxy-4-ethylphenol.
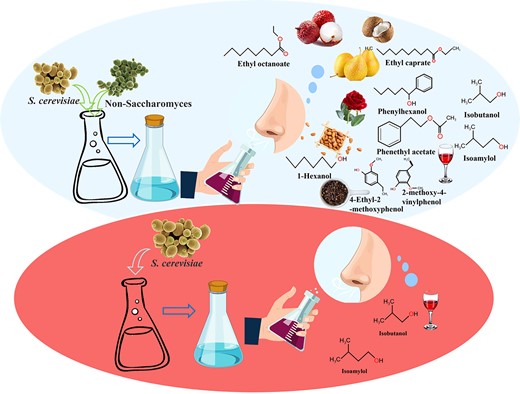
Introduction
Vinegar production is an extensively studied process due to its significant role as a condiment. In the traditional vinegar-making process, there are several key stages, including saccharification, alcoholisation, acidification, and ageing. During the alcoholisation stage, S. cerevisiae plays a crucial role as the primary microorganism. It converts the available reducing sugar derived from the starch present in the grains into ethanol and carbon dioxide through fermentation. Subsequently, in the following acidification stage, acetic acid bacteria are introduced to convert the produced ethanol into acetic acid. In addition to ethanol, S. cerevisiae produces a small number of higher alcohols in the alcoholisation stage.
Non-Saccharomyces was previously considered as spoilage yeast and was not preferred due to its production of undesirable flavours, which affects the quality of the final product (Padilla et al., 2016). However, recent research has shown that non-Saccharomyces can produce more abundant flavour substances in red wine, such as fruity and floral aromas from Pichia spp (Tufariello et al., 2021) and higher alcohols and sulphur-containing compounds from Hanseniaspora spp (Capozzi et al., 2015). A recent study found that the use of H. uvarum in grape juice fermentation led to high concentrations of isoamyl acetate and isobutyl acetate, which enhanced the aroma of banana and strawberry (Hall et al., 2017). Pichia fabianii is a high ester-producing yeast which enhances floral and fruity flavour (Nyanga et al., 2013), while Kluyveromyces thermotolerans produced higher concentrations and types of higher alcohols and had strong alcohol resistance in wine. Saccharomycopsis fibuligera efficiently converted substrates into sugars while generating special phenolic compounds that provided a unique aroma. If these characteristic yeasts are fixedly introduced into the vinegar fermentation process or added to Daqu, it has great potential to weaken the irritating taste caused by acetic acid and improve the quality of vinegar. However, it is important to consider the interactions and competition between different strains during fermentation (Vendramini et al., 2017).
This work uses three non-Saccharomyces, namely P. fabianii, K. thermotolerans and S. fibuligera, for mixed fermentation with S. cerevisiae respectively. The growth competition relationship between them and their influence on the flavour of vinegar alcoholisation process was studied. The mechanism of the influence of non-Saccharomyces on vinegar flavour was expounded from the aspects of sugar metabolism, ethanol and glycerol production and enzyme activity. This work provides a theoretical basis and technical support for the improvement of vinegar flavour.
Materials and methods
Materials and fermentation conditions
The brown rice for fermentation was purchased from Perxian Flagship Store (China Huaian). Strains and enzyme preparations were obtained from Angel Yeast Co., Ltd. (Yichang, China).
The simulated fermentation experiment was carried out in rice medium with a solid–liquid ratio of 1:6. The weighed rice was steamed and sterilised, and UV-sterilised sterile enzyme preparations (prepared by 0.2% glucoamylase, 0.1% amylase and 0.05% protease) were added. Inoculated yeast for fermentation, single-strain fermentation to obtain the initial cell concentration of 106 cells/mL as the inoculation amount, mixed fermentation of non-Saccharomyces and S. cerevisiae inoculation ratio of 1:4. Single S. cerevisiae was used as the control.
Monitoring of strain growth
The cell concentration was tracked every 24 h and counted using Wallerstein Laboratory (WL) and Lysine Agar (LYS) medium (Polizzotto et al., 2016). After culturing at 28°C for 48 h, they were distinguished according to their different morphological characteristics in WL medium (S. cerevisiae showed dark green and large colonies; P. fabianii showed light blue and small colonies; K. thermotolerans showed milky white and S. fibuligera's surface was dry and shrunk.) and the characteristics that S. cerevisiae could not grow on LYS medium.
Measurement of physico-chemical values
The reducing sugar (RS) was determined by 3,5-dinitrosalicylic acid (DNS) method.
Determination of glycerol concentration using Glycerol enzyme-linked immunosorbent assay (ELISA) kit (Wuhan Adanti Biotechnology Co., Ltd.; Wuhan China). The second method of Chinese national standard (GB 5009.225-2016) was used to measure the alcohol content.
The activity of β-glucosidase was determined using 4-Nitrophenyl-β-d-glucopyranoside as substrate (Romero et al., 2012).
Esterase activities towards p-nitrophenyl esters were measured at 30°C by the method of Toshimitsu (Toshimitsu et al., 1986).
Sensory test
Thirty members (20–30 years old) were recruited and participated in a 1-month (60 h) sensory training under expert guidance to evaluate and learn about the sensory characteristics of vinegar. The sensory characteristics of the simulated fermentation broth samples were analysed by quantitative descriptive analysis (QDA). Ten qualified panellists defined and identified the sensory attributes of simulated fermentation broth samples based on their consensus, discriminability and reproducibility. Four kinds of reagents were used to characterise the sensory properties. The concentration was greater than the aroma threshold: 1000 mg/L ethanol (alcohols), 0.01 mg/L ethyl acetate (ester aroma), 0.6 mg/L phenylethanol (floral aroma) and 0.02 mg/L 4-ethylguaiacol (spice aroma). Score according to the softness of each sample. 20 mL samples of fermentation broth from different fermentation days were taken in different paper cups. Each sample is assigned a unique code consisting of three random numbers, with a 5-min interval between each two samples. The scoring criteria were 0 (no intensity), 1–3 (weak), 4–6 (medium), 7–9 (strong) and 10 (very strong).
Measurement of volatile flavour compounds
The volatile compounds of simulated fermentation broth were extracted by headspace solid-phase microextraction (HS-SPME). HS-SPME was performed in a water bath equipped with 50/30 μm DVB/CAR/PDMS extraction at 60°C for 30 min (Supelco, Inc., Bellefonte, PA). Quantitative analysis was performed with 2-octanol as the internal standard. Key flavour substances, such as ethyl acetate, phenethyl acetate, phenylethanol, etc., were quantified by standard addition method.
Statistical analysis
The data analysis was conducted based on three parallel experiments, and the results were presented as mean ± standard deviation. One-way analysis of variance (Anova) was performed. Duncan's multiple range test was applied with a significance level set at P < 0.05. The statistical analysis was conducted using SPSS Software (version 27.0, SPSS Inc., Chicago, IL, USA) with Tukey's test.
Results and discussion
Physico-chemical monitoring
Measurement of reducing sugar and alcohol
Reducing sugars are necessary for the growth of the strain and the production of metabolites. Monitoring the concentration of reducing sugars in the fermentation medium can be used to determine the growth of the strain. Additionally, reducing sugar is an important substrate for alcohol fermentation, which is the main function of S. cerevisiae. However, the addition of non-Saccharomyces to improve flavour may use up some of the reducing sugars, which could have a direct impact on the production of ethanol by S. cerevisiae. Therefore, it is important to consider whether adding non-Saccharomyces to improve flavour will decrease the content of ethanol. Based on Fig. S2, it can be concluded that mixed fermentation has a similar maximum alcohol content to single fermentation. It is worth noting that P. fabianii accelerated the production of ethanol and increased the maximum production. Co-fermentation of S. fibuligera and S. cerevisiae did not significantly affect ethanol production but led to early ethanol consumption. After 5 days, the reduction of ethanol in the co-culture group was accelerated, possibly due to ethanol reacting with other substances promoted by P. fabianii or produced by it. K. thermotolerans yeast slowed down ethanol production initially, with faster-reducing sugar consumption than other groups, indicating that K. thermotolerans consumed reducing sugar required by S. cerevisiae to produce ethanol. The ethanol in this group briefly peaked and then decreased rapidly, suggesting conversion to other substances. S. fibuligera accelerated alcohol production, but limiting S. cerevisiae's capacity to produce more ethanol.
The addition of non-Saccharomyces accelerated the consumption of sugar but P. fabianii was not obvious. The main sugar after the fermentation medium is decomposed is glucose, which is the first sugar used by yeast and has the highest utilisation efficiency. The transport of glucose in yeast is a complex process. The efficiency of transport is related to the transporters on the plasma membrane of yeast. The interaction between strains affects the activity of these transporters, resulting in changes in the absorption of sugar by strains.
However, in this experiment, a direct correlation between alcohol production and sugar consumption was not observed. This is because the consumption of reducing sugar is not solely dedicated to alcohol production; it can also be utilised for the growth and reproduction of the yeast strain. The introduction of non-Saccharomyces yeast strains can result in excessive glucose consumption, and the enzymes produced by these strains can increase the availability of monosaccharides. As a result, the direct relationship between alcohol production and sugar consumption was not discussed in this study.
Generally, alcohol fermentation is carried out under anaerobic conditions, but it is not that S. cerevisiae does not produce alcohol in the presence of oxygen. This is triggered by factors such as the way sugar is transported and the activity of enzymes involved in alcohol fermentation—known as the ‘Krabtree effect’, which occurs when sugar is excessive (Dashko et al., 2014). The production process of alcohol is complex, which is produced in different ways under different conditions, which is closely related to the content of sugar. The presence or absence of oxygen is also a key factor. When oxygen is deficient, it leads to the loss of the final electron acceptor in the missing respiratory chain, which will reduce the tricarboxylic acid cycle and mitochondrial ATP production (Dashko et al., 2014). Moreover, the production of alcohol is bound to consume a large amount of sugar, which will inevitably lead to a decrease in sugar used to increase biomass and produce energy (Gonzalez et al., 2013). It was reported that non-Saccharomyces reduced alcohol production, but this experiment did not confirm this (Gonzalez et al., 2013; Quirós et al., 2014). The increase in ethanol concentration changes the solubility of some flavour compounds (Saha et al., 2018), thus changing the overall sensory.
Glycerol production
Glycerol is an important by-product of some yeast fermentation. A certain concentration of glycerol will play a role in protecting cells. It can maintain osmotic pressure, protect cells from hyperosmotic environment and also manage the balance of phosphate in solution and maintain redox balance. Glycerin affects viscosity, enhances sweetness and gives different tastes, increasing the complexity of the product (Comitini et al., 2011).
Based on Fig. 1, glycerol content increased initially and then decreased with fermentation time. Mixed fermentation of non-Saccharomyces and S. cerevisiae increased glycerol content to varying degrees. However, the co-fermentation group of S. fibuligera and S. cerevisiae showed a different trend, with a significant increase in the first 1–2 days, and a slight upward trend but no peak, remaining above the amount of glycerol produced by S. cerevisiae alone. The common conclusion is that the addition of non-Saccharomyces promotes the production of more glycerol (Ivit et al., 2020). This experiment also showed that non-Saccharomyces slowed down the rate of glycerol reduction.
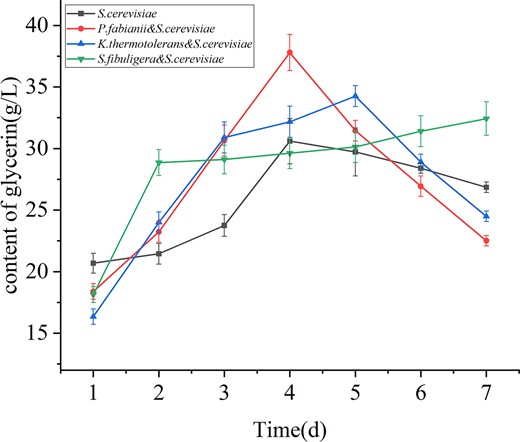
The production of glycerol and ethanol was negatively correlated. (Fairbairn et al., 2021). Glycerol formation is catalysed by glycerol-3-phosphate dehydrogenase (GPDH) and specific phosphatase (Mbuyane et al., 2018). The formation of glycerol changes the redox balance, and the carbon flow is shifted from ethanol to glycerol.
This study has shown that glycerol production increases rapidly when ethanol content reaches its highest point, which typically occurs on the third day of fermentation. This suggests that glycerol production is a mechanism that helps yeast cells maintain redox balance under stress conditions. The glycerol formed by GPDH can be re-generated into NAD+ (Kutyna et al., 2010), which is an important co-factor in yeast metabolism. This provides a mechanism for cells to maintain redox balance using glycerol formation as a way to consume excess NADH. Overall, glycerol production is an important mechanism for yeast cells to adapt to stress conditions and maintain redox balance during fermentation.
The activity of key enzymes in the fermentation process
Activity of β-glucosidase
As a hydrolytic enzyme, β-glucosidase acts on the β (1–4) glycosidic bond of disaccharides, oligosaccharides and glucose-substituted molecules (Ahmed et al., 2017). The β-glucosidase hydrolyzes the non-reducing β-D-glucose bonding at the end and releases β-D-glucose and the corresponding ligands (López et al., 2015). β-glucosidase decomposes macromolecular sugar and releases substances, which not only help to produce more polysaccharides, but also provide a greater possibility for the formation of aromatic flavours. Non-Saccharomyces typically exhibit high β-glucosidase activity during fermentation (López et al., 2015), which can help produce higher concentrations of flavour substances in mixed fermentation.
Fig. 2a showed that non-Saccharomyces greatly increased the activity of β-glucosidase in the early stage, especially the enzyme activity of P. fabianii was 1.6 times higher than that of S. cerevisiae alone. K. thermotolerans and S. fibuligera improved relatively little. The activity of β-glucosidase showed a downward trend as a whole, which was most obvious in the first 48 h, which may be related to the increase of ethanol, and finally, the enzyme activity of all experimental groups was inhibited to the same level. In particular, co-fermentation of P. fabianii and S. cerevisiae significantly increased β-glucosidase activity during the early stages of fermentation, which could be beneficial for aroma substance formation. Pichia spp has great potential to produce β-glucosidase, and shows high β-glucosidase activity at suitable pH and ethanol concentration. In the sugar metabolism experiment, the reducing sugar content of the strain in the first 48 h was higher than that of the other two fermentation methods, which may be the result of β-glucosidase assisted decomposition of brown rice fibre to produce single molecule glucose. However, K. thermotolerans and S. fibuligera did not increase β-glucosidase activity when co-fermented with S. cerevisiae and, in fact, K. thermotolerans inhibited the activity of the enzyme. These findings suggest that different yeast strains can have varying effects on β-glucosidase activity during co-fermentation, which could impact the overall aroma profile of the fermented product.
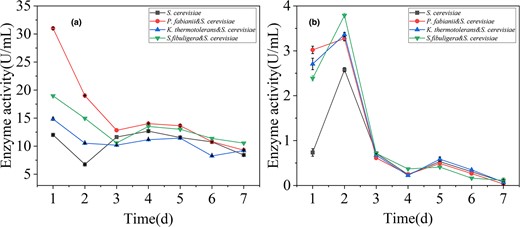
Activity of β-glucosidase during fermentation (a) Esterase activity during fermentation (b).
Esterase activity
Esterase is an enzyme that catalyses the formation of esters, including important aromatic esters such as acetate and isoamyl acetate. Mixed fermentation with non-Saccharomyces increased esterase activity to varying degrees (Fig. 2b), especially in the first 3 days of fermentation, which helped generate more aromatic esters before the production of large amounts of ethanol, enhancing the product's aroma characteristics. The enzyme activity of non-Saccharomyces increased almost threefold during initial fermentation but was severely inhibited by the growth of S. cerevisiae. Enzyme activity decreased sharply after reaching the peak on the second day, which may be due to the formation of ethanol. These results suggest that the presence of different yeast strains during fermentation can impact esterase activity and, consequently, the aroma profile of the fermented product.
Fig. 2 demonstrated that P. fabianii increased the activity of both β-glucosidase and esterase enzymes, providing a catalyst for the production of aromatic compounds (Azzolini et al., 2012). These enzymes not only catalyse the synthesis of their own compounds but may also promote the combination of substances produced by S. cerevisiae to generate other compounds or catalyse the reaction of substances produced by both bacteria. These findings suggest that the presence of P. fabianii during fermentation can impact the activity of these enzymes, ultimately affecting the overall aroma profile of the fermented product.
Flavour evaluation of fermentation broth
When fermented solely by S. cerevisiae, the resulting product has a limited aroma and lower levels of ester content. However, the presence of non-Saccharomyces in natural brewing processes enhances the aromatic complexity and creates a more layered product (Styger et al., 2011). Additionally, the use of non-Saccharomyces can help decrease the alcohol concentration and regulate acidity, which positively affects the brewing process (Padilla et al., 2016).
S. cerevisiae fermentation alone produced fewer types and lower levels of substances that contribute to aromatic odours (Table 1). Typical esters such as phenethyl acetate with rose and honey aromas and isoamyl acetate with apple aromas were not detected. P. fabianii is a proficient producer of aromatic esters, and co-fermentation with S. cerevisiae results in increased content of certain esters, such as ethyl caprylate, which imparts fruity aromas like pear and lychee. Additionally, P. fabianii produces many new esters that S. cerevisiae cannot produce alone, including ethyl acetate and phenylethyl acetate, which help to enhance the unique aroma and alleviate the sharp taste associated with S. cerevisiae fermentation. Among them, similar to the detected Ethyl caprylate belongs to ethyl esters. The formation of ethyl ester first requires the acid to be activated by acetyl coenzyme A (CoA) and then react with alcohol. This type of ester is related to ethanol, and a large amount of ethanol produced by S. cerevisiae provides a substrate for its production. During the formation process, esterase plays a crucial catalytic role and non-Saccharomyces yeast strains are responsible for providing the necessary esters. These esters, particularly ethyl acetate, are essential for the production of aromatic esters. This is consistent with the experimental results of esterase activity, which catalyses the formation of more aromatic esters. At the same time, these esters are the source of floral and fruity aromas in the sensory process, and it is precisely because of their presence that the sharp taste is masked. Another ester is acetate, such as isoamyl acetate and phenylethyl acetate, which is produced by the reaction of acetyl-CoA with alcohol. The source of alcohol here is different from ethanol, which is produced by the degradation of amino acids, carbohydrates or lipids catalysed by alcohol acetyltransferase (AAT) (Russo et al., 2020). The ligands released through the action of β-glucosidase on glycosides can serve as substrates for the synthesis of aromatic esters containing complex groups, such as phenylethyl acetate.
The content of main flavour substances on the third day of fermentation Unit: mg/L
. | S. cerevisiae . | P. fabianii & S. cerevisiae . | K. thermotolerans & S. cerevisiae . | S. fibuligera & S. cerevisiae . |
---|---|---|---|---|
Ethyl acetate | nd | 1.625 ± 0.043a | 0.116 ± 0.009b | 0.200 ± 0.010b |
Phenethyl acetate | nd | 3.662 ± 0.254a | nd | 0.224 ± 0.024b |
Isoamyl formate | 0.006 ± 0.000b | 0.077 ± 0.002a | nd | nd |
Ethyl octanoate | 0.043 ± 0.019c | 0.676 ± 0.017a | 0.081 ± 0.002b | 0.512 ± 0.056a |
Methyl decanoate | nd | nd | nd | 0.053 ± 0.004 |
Ethyl caprate | 0.149 ± 0.004b | 0.306 ± 0.009b | 0.121 ± 0.006b | 4.723 ± 0.073a |
Isobutanol | 0.069 ± 0.006c | 0.126 ± 0.006c | 0.633 ± 0.024b | 1.259 ± 0.144a |
2-Methyl-1-butanol | 1.287 ± 0.074a | 1.301 ± 0.051c | 2.792 ± 0.088b | 2.621 ± 0.092b |
Isoamylol | 0.301 ± 0.004c | 1.365 ± 0.087bc | 2.650 ± 0.034b | 3.623 ± 0.094a |
1-Hexanol | 0.018 ± 0.008ab | 0.024 ± 0.004a | 0.007 ± 0.002b | 0.016 ± 0.003ab |
Phenylethanol | 0.601 ± 0.064c | 0.838 ± 0.023c | 5.712 ± 0.721a | 3.025 ± 0.257b |
Phenylhexanol | nd | 0.165 ± 0.023ab | nd | 0.246 ± 0.072a |
Decanal | 0.015 ± 0.004b | nd | 0.037 ± 0.002ab | 0.057 ± 0.009ab |
Benzeneacetaldehyde | 0.098 ± 0.005a | 0.108 ± 0.005a | nd | nd |
2-methoxy-4-ethylphenol | nd | nd | nd | 0.415 ± 0.088 |
2-methoxy-4-vinylphenol | nd | nd | nd | 0.947 ± 0.099 |
. | S. cerevisiae . | P. fabianii & S. cerevisiae . | K. thermotolerans & S. cerevisiae . | S. fibuligera & S. cerevisiae . |
---|---|---|---|---|
Ethyl acetate | nd | 1.625 ± 0.043a | 0.116 ± 0.009b | 0.200 ± 0.010b |
Phenethyl acetate | nd | 3.662 ± 0.254a | nd | 0.224 ± 0.024b |
Isoamyl formate | 0.006 ± 0.000b | 0.077 ± 0.002a | nd | nd |
Ethyl octanoate | 0.043 ± 0.019c | 0.676 ± 0.017a | 0.081 ± 0.002b | 0.512 ± 0.056a |
Methyl decanoate | nd | nd | nd | 0.053 ± 0.004 |
Ethyl caprate | 0.149 ± 0.004b | 0.306 ± 0.009b | 0.121 ± 0.006b | 4.723 ± 0.073a |
Isobutanol | 0.069 ± 0.006c | 0.126 ± 0.006c | 0.633 ± 0.024b | 1.259 ± 0.144a |
2-Methyl-1-butanol | 1.287 ± 0.074a | 1.301 ± 0.051c | 2.792 ± 0.088b | 2.621 ± 0.092b |
Isoamylol | 0.301 ± 0.004c | 1.365 ± 0.087bc | 2.650 ± 0.034b | 3.623 ± 0.094a |
1-Hexanol | 0.018 ± 0.008ab | 0.024 ± 0.004a | 0.007 ± 0.002b | 0.016 ± 0.003ab |
Phenylethanol | 0.601 ± 0.064c | 0.838 ± 0.023c | 5.712 ± 0.721a | 3.025 ± 0.257b |
Phenylhexanol | nd | 0.165 ± 0.023ab | nd | 0.246 ± 0.072a |
Decanal | 0.015 ± 0.004b | nd | 0.037 ± 0.002ab | 0.057 ± 0.009ab |
Benzeneacetaldehyde | 0.098 ± 0.005a | 0.108 ± 0.005a | nd | nd |
2-methoxy-4-ethylphenol | nd | nd | nd | 0.415 ± 0.088 |
2-methoxy-4-vinylphenol | nd | nd | nd | 0.947 ± 0.099 |
Note: All the substances listed in this table are quantified by standard curve method. Each value is expressed as mean ± standard deviation (n = 3). Different letters (a–d) obtained by Anova indicate significant differences at P < 0.05.
The content of main flavour substances on the third day of fermentation Unit: mg/L
. | S. cerevisiae . | P. fabianii & S. cerevisiae . | K. thermotolerans & S. cerevisiae . | S. fibuligera & S. cerevisiae . |
---|---|---|---|---|
Ethyl acetate | nd | 1.625 ± 0.043a | 0.116 ± 0.009b | 0.200 ± 0.010b |
Phenethyl acetate | nd | 3.662 ± 0.254a | nd | 0.224 ± 0.024b |
Isoamyl formate | 0.006 ± 0.000b | 0.077 ± 0.002a | nd | nd |
Ethyl octanoate | 0.043 ± 0.019c | 0.676 ± 0.017a | 0.081 ± 0.002b | 0.512 ± 0.056a |
Methyl decanoate | nd | nd | nd | 0.053 ± 0.004 |
Ethyl caprate | 0.149 ± 0.004b | 0.306 ± 0.009b | 0.121 ± 0.006b | 4.723 ± 0.073a |
Isobutanol | 0.069 ± 0.006c | 0.126 ± 0.006c | 0.633 ± 0.024b | 1.259 ± 0.144a |
2-Methyl-1-butanol | 1.287 ± 0.074a | 1.301 ± 0.051c | 2.792 ± 0.088b | 2.621 ± 0.092b |
Isoamylol | 0.301 ± 0.004c | 1.365 ± 0.087bc | 2.650 ± 0.034b | 3.623 ± 0.094a |
1-Hexanol | 0.018 ± 0.008ab | 0.024 ± 0.004a | 0.007 ± 0.002b | 0.016 ± 0.003ab |
Phenylethanol | 0.601 ± 0.064c | 0.838 ± 0.023c | 5.712 ± 0.721a | 3.025 ± 0.257b |
Phenylhexanol | nd | 0.165 ± 0.023ab | nd | 0.246 ± 0.072a |
Decanal | 0.015 ± 0.004b | nd | 0.037 ± 0.002ab | 0.057 ± 0.009ab |
Benzeneacetaldehyde | 0.098 ± 0.005a | 0.108 ± 0.005a | nd | nd |
2-methoxy-4-ethylphenol | nd | nd | nd | 0.415 ± 0.088 |
2-methoxy-4-vinylphenol | nd | nd | nd | 0.947 ± 0.099 |
. | S. cerevisiae . | P. fabianii & S. cerevisiae . | K. thermotolerans & S. cerevisiae . | S. fibuligera & S. cerevisiae . |
---|---|---|---|---|
Ethyl acetate | nd | 1.625 ± 0.043a | 0.116 ± 0.009b | 0.200 ± 0.010b |
Phenethyl acetate | nd | 3.662 ± 0.254a | nd | 0.224 ± 0.024b |
Isoamyl formate | 0.006 ± 0.000b | 0.077 ± 0.002a | nd | nd |
Ethyl octanoate | 0.043 ± 0.019c | 0.676 ± 0.017a | 0.081 ± 0.002b | 0.512 ± 0.056a |
Methyl decanoate | nd | nd | nd | 0.053 ± 0.004 |
Ethyl caprate | 0.149 ± 0.004b | 0.306 ± 0.009b | 0.121 ± 0.006b | 4.723 ± 0.073a |
Isobutanol | 0.069 ± 0.006c | 0.126 ± 0.006c | 0.633 ± 0.024b | 1.259 ± 0.144a |
2-Methyl-1-butanol | 1.287 ± 0.074a | 1.301 ± 0.051c | 2.792 ± 0.088b | 2.621 ± 0.092b |
Isoamylol | 0.301 ± 0.004c | 1.365 ± 0.087bc | 2.650 ± 0.034b | 3.623 ± 0.094a |
1-Hexanol | 0.018 ± 0.008ab | 0.024 ± 0.004a | 0.007 ± 0.002b | 0.016 ± 0.003ab |
Phenylethanol | 0.601 ± 0.064c | 0.838 ± 0.023c | 5.712 ± 0.721a | 3.025 ± 0.257b |
Phenylhexanol | nd | 0.165 ± 0.023ab | nd | 0.246 ± 0.072a |
Decanal | 0.015 ± 0.004b | nd | 0.037 ± 0.002ab | 0.057 ± 0.009ab |
Benzeneacetaldehyde | 0.098 ± 0.005a | 0.108 ± 0.005a | nd | nd |
2-methoxy-4-ethylphenol | nd | nd | nd | 0.415 ± 0.088 |
2-methoxy-4-vinylphenol | nd | nd | nd | 0.947 ± 0.099 |
Note: All the substances listed in this table are quantified by standard curve method. Each value is expressed as mean ± standard deviation (n = 3). Different letters (a–d) obtained by Anova indicate significant differences at P < 0.05.
On the contrary, the addition of K. thermotolerans does not produce many esters, but increases the type and content of higher alcohols, including isobutanol and isoamylol, which contribute to make the taste more mellow, and the reason why the mellow sense was stronger than that of S. cerevisiae alone. Furthermore, this yeast significantly increases the content of Phenylethanol, which imparts a special fruit and floral aroma. Despite producing fewer substances, this yeast does not introduce excess odour. It is noteworthy that K. thermotolerans can tolerate the metabolites produced by S. cerevisiae, particularly ethanol, which is an important advantage for co-fermentation. Furthermore, the yeast can produce L-Lactic acid, which adds complexity to the product flavour without introducing any unwanted odours.
The addition of S. fibuligera in the fermentation process brings about a notable improvement in the flavour of the end product. This particular yeast strain has the capacity to generate various aromatic esters, including phenethyl acetate and ethyl octanoate, contributing fruity and floral notes to the aroma. Moreover, S. fibuligera stimulates S. cerevisiae to produce higher levels of higher alcohol, further enriching the flavour profile. While S. fibuligera is known for its amylase production and starch decomposition into trehalose (Wang et al., 2011), these characteristics were not observed in this experiment, possibly due to the inhibitory effect of S. cerevisiae on S. fibuligera's functionality. Additionally, S. fibuligera produces two distinct compounds, namely 2-methoxy-4-ethylphenol and 2-methoxy-4-vinylphenol, imparting a unique spice and nutty flavour to the fermentation broth. Overall, incorporating S. fibuligera in the fermentation process substantially enhances the flavour complexity of the final product, and its ability to produce distinctive substances like 2-methoxy-4-ethylphenol and 2-methoxy-4-vinylphenol imparts a characteristic flavour profile that sets it apart. The increased variety and content of flavour substances can be attributed to the enhanced activity of β-glucosidase, leading to the more thorough breakdown of glycosides.
According to Fig. 3, in the left panel, the relationship between sensory perception and volatile flavour substances in the fermentation broth is displayed. While the right panel depicts the distribution and content of various flavour substances in different experimental groups involving mixed fermentation and single fermentation of S. cerevisiae. By analysing these panels, we can analyse the odour characteristics of the four groups of fermentation broth. In the fermentation broth of S. cerevisiae fermented alone, the main flavour substances associated with a rose fragrance, such as ethyl acetate, phenethyl acetate and phenethyl alcohol, were not detected. Additionally, there was a lower detection of ethyl octanoate, which contributes to a fruit aroma, in this experimental group. However, in the mixed fermentation of P. fabianii and S. cerevisiae, benzeneacetaldehyde, isoamyl formate and phenylhexanol were found in higher quantities compared to other experimental groups. When K. thermotolerans and S. cerevisiae were co-fermented, the flavour substances associated with a wine-like flavour, such as isobutanol, isoamylol and 2-methyl-1-butanol, were predominant, while aromatic ester compounds were rarely detected. This finding explains why the mixed fermentation method resulted in a mellow taste perception. The co-fermentation of S. fibuligera and S. cerevisiae exhibited the most abundant variety of flavour substances, with special attention given to 2-methoxy-4-ethylphenol and 2-methoxy-4-vinylphenol, which impart a spice flavour.
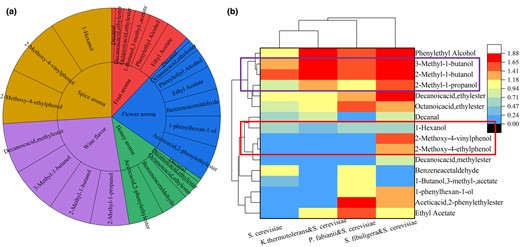
The diagram chart of main flavour substances produced by fermentation broth (a) and heat map of main volatile flavour substances (b). (a) shows the relationship between sensory flavour and compounds. The inner ring is the sensory flavour and the outer ring is the compound showing the corresponding flavour. The representative compounds of mellow flavour are in the purple box and the compounds of spice flavour are in the red box in (b).
Conclusions
This study shows that the addition of non-Saccharomyces into the fermentation process enhanced the overall flavour and enzyme activity, resulting in a positive impact on the fermentation outcome. The simultaneous inoculation method increased the content and type of volatile flavour substances and enhanced the sense of hierarchy, weakened the sharp irritation. The enzyme activity of esterase and β-glucosidase in mixed fermentation of non-Saccharomyces and S. cerevisiae was enhanced. The addition of non-Saccharomyces increases the concentration of glycerol while ensuring the normal production of alcohol, which will improve the taste and texture of vinegar. By comparison, it was found that P. fabianii and S. fibuligera had poor tolerance to ethanol, resulting in less growth when they were inoculated with S. cerevisiae. In the next study, we should pay attention to whether sequential inoculation can improve this.
Acknowledgments
This work was supported by the National Natural Science Foundation of China (No. 31972194 and 32272458).
Author contributions
Fengjun Wang: methodology (lead); writing – original draft (lead). Ling Zhu: Methodology (lead). Hadiatullah Hadiatullah: Writing – review and editing (lead). Zhaofei Li: Resources (equal). Jing He: Resources (equal). Guozhong Zhao: Conceptualization (lead). Yunping Yao: Project administration (equal); supervision (equal).
Conflict of interest statement
No potential conflict of interest was reported by the authors.
Ethical approval
Ethics approval was not required for this research.
Peer review
The peer review history for this article is available at https://www.webofscience.com/api/gateway/wos/peer-review/10.1111/ijfs.16584.
Data availability statement
The data is available by request to the corresponding author.