-
PDF
- Split View
-
Views
-
Cite
Cite
Dimitrios Tsimogiannis, Maria Stavrakaki, Vassiliki Oreopoulou, Isolation and characterisation of antioxidant components from oregano (Origanum heracleoticum), International Journal of Food Science and Technology, Volume 41, Issue Supplement_1, August 2006, Pages 39–48, https://doi.org/10.1111/j.1365-2621.2006.01259.x
- Share Icon Share
Abstract
In this study, Origanum heracleoticum was examined as potential source of phenolic antioxidants. The components of the plant were extracted in a Soxhlet apparatus sequentially with solvents of increasing polarity, specifically with petroleum ether (P), diethyl ether (D) and ethanol (E); the ethanol extract was further fractionated to obtain a diethyl ether (ED) and an ethyl acetate (EEAc) fraction. The activity of the extracts to scavenge 2′2′-diphenyl-1-picrylhydrazyl followed the order ED > E> D > EEAc >> P. Moreover, the antioxidant activity in refined cottonseed oil at a concentration of 500 ppm was monitored through peroxide value measurements and revealed that ED resulted in the highest decrease in the rate of peroxide formation followed by D. Specific flavonoids and rosmarinic acid were detected as the main components of D, ED through HPLC–DAD and HPLC–MS/MS analyses.
Introduction
The modern trends in nutrition suggest the limitation of synthetic food additives or the substitution with natural ones. Aromatic herbs are probably the most important source of natural antioxidants. Among the most common genera in Greece, oregano, sage, thyme, dittany and marjoram have demonstrated antioxidant activity in lipid substrates. Extracts of these herbs have the ability to retard both the initiation and the rate of lipid oxidation during storage.
Oregano (Origanum vulgare) is widespread in the Mediterranean area, with three subspecies encountered in Greece: vulgare, viridulum and hirtum (Syn. Origanum heracleoticum) (Vokou et al., 1993). The latest is also defined as Greek oregano by several authors (Vokou et al., 1993; Özcan & Akgül, 1995; Bocchini et al., 1998; Exarchou et al., 2002), occurs over most parts of Greece and presents variable morphological characteristics. Analyses of the essential oil of O. vulgare subspecies have been conducted by many researchers. D‘Antuono et al. (2000) identified sixty-four components; among them the most abundant were linalool, γ-terpinene, thymol and carvacrol, while p-cymene, borneol and β-bisabolene were also detected. Taking into account the differences in composition of the essential oil from different O. vulgare populations that they observed, the authors commented on the difficulties encountered in trying to establish clear correspondence between the subspecies of O. vulgare and the chemical composition of their essential oil. However, Vokou et al. (1993) examining essential oils of O. vulgare ssp. hirtum from twenty-three localities scattered all over Greece found that, in spite of the high variability of individual compounds in the essential oil, the sum of carvacrol, thymol, p-cymene and γ-terpinene was stable amounting to more than 80%. Bocchini et al. (1998), on the contrary, identified thymol, p-cymene, γ-terpinene and thymyl methyl ether as the main components of O. heracleoticum essential oil. Carvacrol and thymol appear to be the main components of all O. vulgare ssp., as indicated by the work of D'Antuono et al. (2000), Menaker et al. (2004) and Kulisic et al. (2004), although their content may vary considerably among different populations (Vokou et al., 1993; D'Antuono et al., 2000).
Origanum vulgare extracts have demonstrated antioxidant activity against lipid peroxidation as proved by the work of several researchers. Vekiari et al. (1993) extracted essential oils and phenolic compounds in individual fractions. A fraction obtained by diethyl ether contained several flavonoids and was the most effective in stabilising lard and vegetable oils against oxidation. Abdalla & Roozen (1999) collected the acetone-soluble components and evaluated their antioxidant activity in sunflower oil and 20% oil-in-water emulsion. At a concentration level 600 ppm in bulk oil, the activity was found significant by means of conjugated diene hydroperoxides and volatile compounds measurements, while the extract was less active in emulsion. Lindberg Madsen et al. (1996) studied the 80% ethanol extract, which showed high activity determined by the oxygen depletion method. Oregano has also demonstrated synergism with ascorbyl palmitate on the antirancidity and preservation of α-tocopherol in sunflower oil at 95 °C (Beddows et al., 2001). The activity of oregano extracts against 2′2′-azobis dihydrochloride (Zheng & Wang, 2001) and 2′2′-diphenyl-1-picrylhydrazyl (DPPH) (Exarchou et al., 2002) radicals was also remarkable. Furthermore, Özcan & Akgül (1995) and Milos et al. (2000) studied the essential oil that was found effective in stabilising sunflower oil and lard stored in dark at 70 and 60 °C, respectively. In addition, inhibition of lipid oxidation during long-term frozen storage of chicken meat was observed when oregano essential oil was used as a dietary supplement (Botsoglou et al. (2003). The essential oil activity was verified by the use of various testing methods (Kulisic et al., 2004).
In addition to the above-referred analyses of the essential oil, component analyses of oregano extracts have been performed to identify the antioxidant compounds. According to Vekiari et al. (1993), flavonoid constituents of oregano (O. vulgare) are apigenin, eriodictyol, taxifolin and dihydrokaempferol. The presence of these flavonoids was further supported by the analysis of Bocchini et al. (1998) through a pyrolysis – GC/MS technique. Apigenin and luteolin (in insignificant quantities) were detected in acetone extracts of Greek oregano (O. vulgare ssp. hirtum) by Exarchou et al. (2002). The authors attributed the observed activity against DPPH radical of both ethanol and acetone extracts of the plant to rosmarinic acid, which they detected as the main component. Justesen (2000) and Justesen & Knuthsen (2001) identified rosmarinic acid, luteolin, luteolin glycoside, apigenin, apigenin-acetyl-diglycoside and diosmetin-acetyl-glucuronide, while quercetin, kaempferol, isorhamnetin and hesperetin were not detected (Justesen & Knuthsen, 2001).
In this study, O. heracleoticum collected from the mountains of Ierapetra (Crete) was examined. The plant is used mostly as a condiment and possesses similar flavour to the other O. vulgare subspecies, but pale yellow colour in contrast to the green colour of other O. vulgare populations. Therefore, extracts of the plant could be used as natural antioxidants without bleaching. According to the traditional medicine, O. heracleoticum is diuretic and remedy for psoriasis and epilepsy. Our main objective was the isolation of antioxidant fractions from O. heracleoticum and the determination of their effect against free radicals and lipid oxidation. Fractionation was based on polarity in order to distinguish antioxidants that are readily soluble in lipids, from the rest antioxidant fractions. As the observed activity of a fraction can be attributed to specific components, we focused on identifying the majority of the main antioxidant components of the plant.
Materials and methods
Materials
Origanum heracleoticum was collected from the mountains of Ierapetra (Crete) during July, which is the month of maximal inflorescence of the herb. The extractions were carried out in petroleum ether, diethyl ether and ethanol at 95°C. For the fractionation of the ethanol extract, diethyl ether and ethyl acetate were used. Anhydrous sodium sulphate was used for drying the diethyl ether and ethyl acetate fractions. All the reagents were of analytical grade except ethanol. DPPH radical (purity 95%) was a product of Aldrich, Wisconsin, USA. The standard compounds used for analyses were rosmarinic acid (purum; Fluka, Buchs, Switzerland), apigenin (Fluka 95%), eriodictyol (pure; Extrasynthèse, Genay, France), taxifolin (Sigma) quercetin dihydrate (Fluka, 99%) and luteolin (Sigma, Steinheim, Germany). Refined cottonseed oil (Minerva SA, Inofita, Greece) was used for the autoxidation experiments.
Extraction of antioxidants
The dried plant material was ground in a mill (Retch ZM 1; Haan, Germany), equipped with a 0.5-mm sieve. The ground material (195.5 g) was extracted in a Soxhlet apparatus sequentially with petroleum ether (P), diethyl ether (D) and ethanol (E) until the recovered extract was colourless. The extracts were diluted to approximately 0.9L with the corresponding solvent, filtered and further diluted to the standard volume of 1 L. The amount of the total extracted compounds was finally determined in triplicate.
The ethanol extract (E) was further treated as follows: 50 mL was diluted with 50 mL of deionised water and ethanol was evaporated under vacuum. The aqueous suspension was extracted by turns with diethyl ether (3 × 50 mL) (ED) and ethyl acetate (3 × 50 mL) (EEAc) in a separating funnel. The combined extracts of diethyl ether and ethyl acetate were dried with anhydrous sodium sulphate and condensed under vacuum to the initial volume of the crude extract (50 mL). The whole process is presented in Fig. 1.
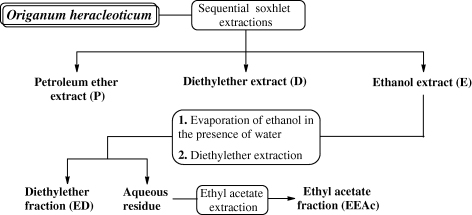
Activity assays
DPPH test
All the DPPH scavenging measurements were held on a digital spectrophotometer (Unicam Helios, Spectronic Unicam EMEA, Cambridge, UK), according to the method proposed by Brand-Williams et al. (1995), under thermostated conditions at 25 °C. 3.9 mL of a daily-prepared DPPH solution of 6.02×10−5m in methanol was placed in a cuvette and 0.1 mL of an extract was added. The cuvette was placed in the spectrophotometer and the absorbance was recorded at 515 nm. In a second cuvette, a blank DPPH solution was screened so as to determine the decomposition rate of the radical and correct the decrease in absorbance during the reaction with the flavonoids, according to Ancerewicz et al. (1998). The procedure was performed for different concentrations of each extract.
Oxidation test
The antioxidant activity of the extracts was tested at a 500 ppm addition level. A specific volume of each extract was added in the corresponding quantity of cottonseed oil, under continuous stirring and purging with nitrogen until total removal of the solvent. An amount of 50.00 g of each sample as well as pure cottonseed oil were transferred into identical 100 mL open beakers and subjected to accelerated oxidation at 70 °C in a ventilated oven (Heraeus Instruments GmbH, Hanau, Germany). The oxidative process was monitored by determination of peroxide value (PV) according to IUPAC, method 2.501 (1987). The experiments were run in duplicate and the presented results are mean values.
Analytical procedures
GC–MS analysis
Petroleum ether extracted the less polar compounds, which comprise the essential oil of the herb. As the components are volatile, GC–MS analysis was applicable for their identification. The analyses were performed using an HP 6890 GC system (plus +) coupled to an HP 5973 mass selective detector (Hewlett Packard, Palo Alto, CA, USA). The constituents of the mixtures were separated using an HP-1 MS column (30 m length, 0.25 i.d., 1.00 μm film thickness, Hewlett Packard, Palo Alto, CA, USA). The oven temperature was kept stable for 2.5 min at 40 °C and then increased to 300 °C at 20 °C min−1 rate. Helium was used as a carrier gas at an average velocity of 28 cm s−1 (0.6 mL min−1 flow). The mass range was 10–400 and compounds were identified by comparison of their mass spectra with the data of NIST and Wiley mass spectral libraries.
HPLC–DAD analysis
The HPLC–DAD method proposed by Mercken & Beecher (2000) was used in order to separate the compounds of the fractions obtained with diethyl ether and ethanol. The HPLC apparatus consisted of an HP 1100 gradient pump and a diode array detector (Hewlett-Packard, Waldbronn, Germany). A Hypersil C18 column ODS 5 μm, 250 × 4.6 mm (MZ Analysentechnik, Mainz, Germany) was used under thermostated conditions at 30 °C. The solvent system consisted of water (A), methanol (B) and acetonitrile (C), each containing 0.2% trifluroacetic acid. The initial composition of the mobile phase was 90% A, 6% B and 4% C. With linear gradients, the composition changed to 85% A, 9% B and 6% C within 5 min, 71% A, 17.4% B and 11.6% C within 30 min, and 0% A, 85% B and 15% C within 60 min. The flow rate was maintained at 1 mL min−1. The elaboration of the chromatographic data was carried out on a ChemStation for LC 3D software (Agilent Technologies, 1999–2000, Waldbrook, Germany). All the major and specific secondary peaks were collected in Eppendorff tubes and analysed further by HPLC–MS/MS.
HPLC–MS/MS analysis
Each of the collected peaks was injected (1 μL) into an UltiMate nano-HPLC (LC-Packings, Amsterdam, the Netherlands) system with an attached C18 HPLC column (75 μm × 15 cm, LC Packings). Acetonitrile–water (1:1), at 200 nL min−1, was used as elution solvent and the samples were analysed on an ion trap mass spectrometer (LCQ-Deca, Thermo-Finnigan, San Jose, CA, USA) with a nanospray source. The capillary temperature was 200 °C and the capillary voltage 31 V. All data were collected as positive-ion spectra while the MS was performing a full mass scan at the range of 50–750; an MS–MS (MS2) of the most abundant ion has obtained using collision and energy of 35 eV for flavanones and dihydroflavonols, and 45 eV for flavonols and flavones.
Statistical analysis
Microsoft Excel was used for linear regression analysis of oil oxidation data.
Results and discussion
The primer component fractionation of the herb was based on the polarity. Petroleum ether was used to extract the less polar compounds such as the constituents of the essential oil. The oil-free plant material was then treated with diethyl ether for the extraction of phenolic aglycones, while the more polar phenolic acids and glycosides were extracted with ethanol. The amount of the total extracted compounds by petroleum ether was 10.0% (±SD 0.9) w/w on dry basis of the plant, while the corresponding values for diethyl ether and ethanol were 2.9% (±SD 0.3) and 11.3% (±SD 0.8), respectively. The ethanol extract was further treated, as mentioned in the Materials and Methods section, as it might probably contain polar compounds insoluble in lipid substrates such as glycosides and free sugars. Furthermore, ethanol is an inappropriate medium for embodying additives in oils; it has low solubility in oils and cannot be removed easily with the purge of nitrogen. Therefore, treatment of ethanol extract aimed to the recovery of lipid-soluble antioxidant substances. Diethyl ether is a low polarity solvent, which can dissolve the potential phenolic acids and flavonoid aglycones of an aqueous suspension. Ethyl acetate extracts medium polarity compounds, while compounds of high polarity such as free sugars remain in aqueous residue.
Activity assays
2′2′-Diphenyl-1-picrylhydrazyl test is a common method applied for the prediction of antioxidant activity of pure compounds or extracts during lipid peroxidation. As autoxidation of oils and fats is based on free radical mechanism, the reaction between a pure radical (DPPH) and hydrogen atom donors, such as polyphenols, can provide important information on the properties of a potential antioxidant. The estimation of antioxidant activity is usually based on the EC50 parameter, which represents the moles of antioxidant necessary to reduce one mole of DPPH radical by 50% (Sanchez-Moreno et al., 1998). In the study of extracts, which contain many components, EC50 is expressed by means of g (dry material) kg−1 DPPH. Obviously, the lower the EC50 the higher the antiradical activity.
The EC50 parameters (g kg−1 DPPH) for the five fractions of origanum are shown in Table 1; the classification of their antiradical activities, according to EC50, is as follows: ED > E > D > EEAc >> P. D extract presented significant activity by contrast to the compounds extracted with petroleum ether. E extract revealed high antiradical activity, and especially ED fraction was the most efficient; these results indicate that a potential efficient antioxidant fraction was isolated, while the remaining phenolic components, recovered to EEAc fraction, also presented high EC50.
Fraction . | Antiradical activity . | Antioxidant activity . | ||
---|---|---|---|---|
![]() | Peroxide formation rate constant . | Protection parameter . | ||
k (meq O2 kg h−1) . | Standard error . | (1 − ki/kc) × 100% . | ||
Control | – | 1.72 | 0.059 | 0 |
P | 9524 | 1.50 | 0.069 | 13 |
D | 719 | 0.88 | 0.013 | 49 |
E | 635 | 1.74 | 0.079 | −1 |
ED | 261 | 0.48 | 0.011 | 72 |
EEAc | 1409 | – | – | – |
Fraction . | Antiradical activity . | Antioxidant activity . | ||
---|---|---|---|---|
![]() | Peroxide formation rate constant . | Protection parameter . | ||
k (meq O2 kg h−1) . | Standard error . | (1 − ki/kc) × 100% . | ||
Control | – | 1.72 | 0.059 | 0 |
P | 9524 | 1.50 | 0.069 | 13 |
D | 719 | 0.88 | 0.013 | 49 |
E | 635 | 1.74 | 0.079 | −1 |
ED | 261 | 0.48 | 0.011 | 72 |
EEAc | 1409 | – | – | – |
Fraction . | Antiradical activity . | Antioxidant activity . | ||
---|---|---|---|---|
![]() | Peroxide formation rate constant . | Protection parameter . | ||
k (meq O2 kg h−1) . | Standard error . | (1 − ki/kc) × 100% . | ||
Control | – | 1.72 | 0.059 | 0 |
P | 9524 | 1.50 | 0.069 | 13 |
D | 719 | 0.88 | 0.013 | 49 |
E | 635 | 1.74 | 0.079 | −1 |
ED | 261 | 0.48 | 0.011 | 72 |
EEAc | 1409 | – | – | – |
Fraction . | Antiradical activity . | Antioxidant activity . | ||
---|---|---|---|---|
![]() | Peroxide formation rate constant . | Protection parameter . | ||
k (meq O2 kg h−1) . | Standard error . | (1 − ki/kc) × 100% . | ||
Control | – | 1.72 | 0.059 | 0 |
P | 9524 | 1.50 | 0.069 | 13 |
D | 719 | 0.88 | 0.013 | 49 |
E | 635 | 1.74 | 0.079 | −1 |
ED | 261 | 0.48 | 0.011 | 72 |
EEAc | 1409 | – | – | – |
The DPPH test suggests that in a more complicated system, like a lipid substrate under oxidation, ED would also scavenge more free radicals than the rest fractions (until the complete exhaust of each compound). Therefore, less oxidation products, such as hydroperoxides, would occur by the end of its action. The truth of this suggestion was confirmed by a series of autoxidation tests.
Using PV as an index for the oxidative process, the activity of a specific concentration of antioxidant can be estimated by the decrease in the rate of peroxide formation. The results of the four additives compared with the control sample are presented in Fig. 2. As can be seen, the control and E, P, and D extracts presented initially a low increase in PV, corresponding to the induction period, followed by a higher rate of oxidation characterised by a linear increase of PV with time of storage. The end of the induction period was estimated through the curves, and the corresponding PV values were significantly lower for the sample with the D extract compared with the others. ED fraction exhibited a very high antioxidant activity and maintained a low rate of peroxide formation throughout the whole oxidation period. EEAc fraction was completely insoluble and the oxidation test was not performed for the relative oil sample. Moreover, it should be pointed out that the low protecting ability of the E extract is attributed to the low solubility in the oil, despite the potent antioxidant components it possesses, as indicated by EC50.
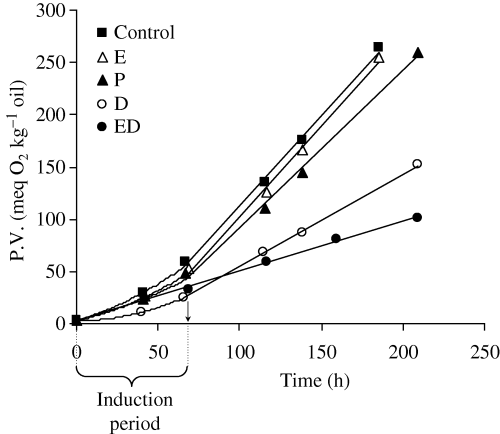
The rate constants of the linear increase of PV after the induction period are presented in Table 1, with the corresponding standard errors. To express the depression of peroxide formation caused by each extract, a protection parameter can be calculated; it represents the decrease in the rate constant of peroxide formation obtained by the addition of antioxidant (ki) compared with that of the blank oil (kc), after the induction period, and is expressed as [1 − (ki/ kc)] × 100%.
The calculated protection parameters are also presented in Table 1. The classification of the additives based on their activity against lipid oxidation is ED > D >> P > E. Regarding the ethanol extract (E), it was partially solubilised in the oil; the insoluble material, which may affect its use, consisted probably of glycosides and free sugars. However, the rest additives had identical classification to the respective of DPPH test.
GC–MS, HPLC–DAD analysis
The petroleum ether extract (P) was analysed by GC–MS and the identified compounds are presented in Table 2. It is apparent that the peak with retention time 10.76 min (Fig. 3) is the main component. MS identified the compound as carvacrol. According to the integration of peaks, the area percentage of carvacrol was 42.53%, as indicated in Table 2, while the rest identified peaks did not exceed 6% each. Especially, thymol is a minor component in O. heracleoticum from Ierapetra (Crete) region, in contrast to other reports for the same subspecies (Bocchini et al., 1998; Kulisic et al., 2004). However, Vokou et al. (1993) after the examination of several samples from locations scattered all over Greece commented that a high thymol content is not typical of all O. vulgare ssp. hirtum (Syn O. heracleoticum) populations.
The (P) extract compounds of Origanum heracleoticum as identified by GC–MS analysis
Retention time (min) . | Compound . | Aia (%) . |
---|---|---|
Petroleum ether extract | ||
7.74 | α-Thujene | 0.13 |
7.86 | α-Pinene | 0.30 |
8.02 | Camphene | 0.17 |
8.26 | Myrcene | 0.67 |
8.50 | α-Phellandrene | 0.10 |
8.62 | p-Cymene | 5.91 |
8.96 | γ-Terpinene | 1.64 |
9.04 | trans-Sabinene hydrate | 0.74 |
9.24 | Terpinolene | 0.17 |
9.31 | cis-Sabinene hydrate | 0.87 |
9.93 | Borneol | 2.51 |
9.99 | Terpinen-4-ol | 1.17 |
10.46 | Linalyl acetate | 2.74 |
10.67 | Thymol | 1.24 |
10.76 | Carvacrol | 42.53 |
11.28 | Carvacryl acetate | 0.33 |
11.66 | α-Cubebene | 0.27 |
12.01 | β-Caryophyllene | 1.44 |
12.14 | Aromadendrene | 0.74 |
12.38 | β-Bisabolene | 4.48 |
12.46 | Leden | 0.43 |
12.49 | β-Sesquiphellandrene | 0.10 |
12.53 | β-Cadinene | 0.70 |
12.96 | (+)-Spathulenol | 0.13 |
13.04 | Caryophyllene oxide | 1.37 |
Retention time (min) . | Compound . | Aia (%) . |
---|---|---|
Petroleum ether extract | ||
7.74 | α-Thujene | 0.13 |
7.86 | α-Pinene | 0.30 |
8.02 | Camphene | 0.17 |
8.26 | Myrcene | 0.67 |
8.50 | α-Phellandrene | 0.10 |
8.62 | p-Cymene | 5.91 |
8.96 | γ-Terpinene | 1.64 |
9.04 | trans-Sabinene hydrate | 0.74 |
9.24 | Terpinolene | 0.17 |
9.31 | cis-Sabinene hydrate | 0.87 |
9.93 | Borneol | 2.51 |
9.99 | Terpinen-4-ol | 1.17 |
10.46 | Linalyl acetate | 2.74 |
10.67 | Thymol | 1.24 |
10.76 | Carvacrol | 42.53 |
11.28 | Carvacryl acetate | 0.33 |
11.66 | α-Cubebene | 0.27 |
12.01 | β-Caryophyllene | 1.44 |
12.14 | Aromadendrene | 0.74 |
12.38 | β-Bisabolene | 4.48 |
12.46 | Leden | 0.43 |
12.49 | β-Sesquiphellandrene | 0.10 |
12.53 | β-Cadinene | 0.70 |
12.96 | (+)-Spathulenol | 0.13 |
13.04 | Caryophyllene oxide | 1.37 |
aAi = The area percentage of each compound as determined by the ChemStation Integrator.
The (P) extract compounds of Origanum heracleoticum as identified by GC–MS analysis
Retention time (min) . | Compound . | Aia (%) . |
---|---|---|
Petroleum ether extract | ||
7.74 | α-Thujene | 0.13 |
7.86 | α-Pinene | 0.30 |
8.02 | Camphene | 0.17 |
8.26 | Myrcene | 0.67 |
8.50 | α-Phellandrene | 0.10 |
8.62 | p-Cymene | 5.91 |
8.96 | γ-Terpinene | 1.64 |
9.04 | trans-Sabinene hydrate | 0.74 |
9.24 | Terpinolene | 0.17 |
9.31 | cis-Sabinene hydrate | 0.87 |
9.93 | Borneol | 2.51 |
9.99 | Terpinen-4-ol | 1.17 |
10.46 | Linalyl acetate | 2.74 |
10.67 | Thymol | 1.24 |
10.76 | Carvacrol | 42.53 |
11.28 | Carvacryl acetate | 0.33 |
11.66 | α-Cubebene | 0.27 |
12.01 | β-Caryophyllene | 1.44 |
12.14 | Aromadendrene | 0.74 |
12.38 | β-Bisabolene | 4.48 |
12.46 | Leden | 0.43 |
12.49 | β-Sesquiphellandrene | 0.10 |
12.53 | β-Cadinene | 0.70 |
12.96 | (+)-Spathulenol | 0.13 |
13.04 | Caryophyllene oxide | 1.37 |
Retention time (min) . | Compound . | Aia (%) . |
---|---|---|
Petroleum ether extract | ||
7.74 | α-Thujene | 0.13 |
7.86 | α-Pinene | 0.30 |
8.02 | Camphene | 0.17 |
8.26 | Myrcene | 0.67 |
8.50 | α-Phellandrene | 0.10 |
8.62 | p-Cymene | 5.91 |
8.96 | γ-Terpinene | 1.64 |
9.04 | trans-Sabinene hydrate | 0.74 |
9.24 | Terpinolene | 0.17 |
9.31 | cis-Sabinene hydrate | 0.87 |
9.93 | Borneol | 2.51 |
9.99 | Terpinen-4-ol | 1.17 |
10.46 | Linalyl acetate | 2.74 |
10.67 | Thymol | 1.24 |
10.76 | Carvacrol | 42.53 |
11.28 | Carvacryl acetate | 0.33 |
11.66 | α-Cubebene | 0.27 |
12.01 | β-Caryophyllene | 1.44 |
12.14 | Aromadendrene | 0.74 |
12.38 | β-Bisabolene | 4.48 |
12.46 | Leden | 0.43 |
12.49 | β-Sesquiphellandrene | 0.10 |
12.53 | β-Cadinene | 0.70 |
12.96 | (+)-Spathulenol | 0.13 |
13.04 | Caryophyllene oxide | 1.37 |
aAi = The area percentage of each compound as determined by the ChemStation Integrator.
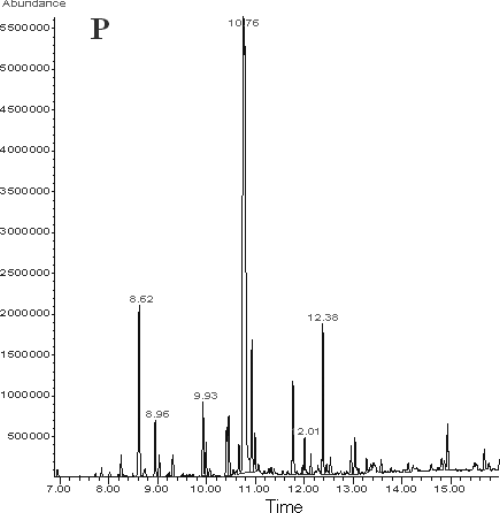
The diethyl ether (D) and ethanol (E) extracts of the plant were analysed by HPLC–DAD and the chromatographic profiles are presented in Fig. 4. D extract analysis revealed six major peaks and several minor ones. The UV–Vis spectra of the major peaks, presented in Fig. 5, were characteristic of flavonoid compounds, with two principal bands of absorbance. Figure. 5 also shows the typical UV–Vis spectra of flavonoids belonging to four basic subgroups. Band A absorbance lies in the range 310–350 nm for flavones, while for flavonols between 350 and 385 nm. Band B is much the same for the aforementioned flavonoid subgroups and it is found in the range 250–280 nm. In flavanones and dihydroflavonols, band A is reduced to little more than a shoulder in 300–330 nm and band B is the main peak in the range 277–295 nm (Bohm, 1998).
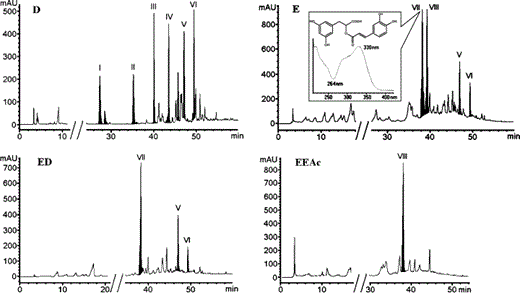
HPLC–DAD chromatograms of diethyl ether (D) and ethanol extract (E), as well as the two fractions of the latter: diethyl ether (ED) and ethyl acetate (EEAc) fraction. The UV–Vis spectrum and the structure of peak VII (rosmarinic acid) are also presented.
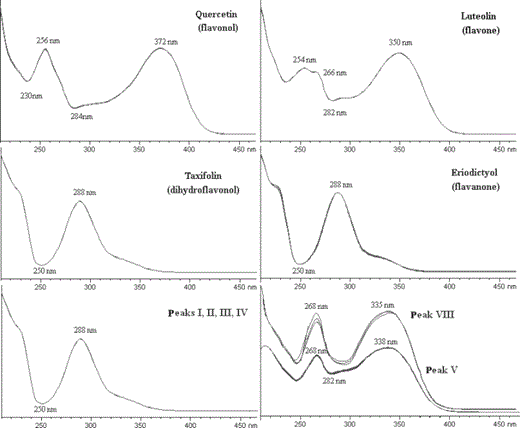
The UV–Vis spectra of selected flavonoids belonging to the major subgroups, as well as the respective spectra of origanum flavonoids.
Peaks I, II, III and IV in D extract of O. heracleoticum had almost identical UV–Vis spectra with absorbance maxima at 288 nm (band A) and reduced band B, clear evidences that they belong either to flavanones or to dihydroflavonols (Fig. 5). Peak VI was identified as carvacrol with the use of internal standard. Carvacrol and compound V with retention time 47.0 min were also detected in ethanol extract, E, which also contained peaks VII and VIII, as main components. V and VIII belong to flavones or flavonols according to their spectra (Fig. 5).
The two fractions, ED and EEAc, respectively, were injected into the HPLC–DAD system; the fractionation procedure led to the separation of VIII from the rest peaks, also the background noise was reduced in each fraction, as indicated in Fig. 4. Compound VII of E extract was recovered in the ED fraction. It was eluted at 38.0 min and revealed a clear UV–Vis spectrum with absorbance maximum at 330 nm (Fig. 4). It was identified as rosmarinic acid with the use of internal standard. According to a reference curve, the amount of rosmarinic acid was determined as 23.5 g L−1 in E extract, 153 g L−1 in ED fraction and 2.71 g kg−1 in origanum (dry basis).
HPLC–MS/MS analysis
Obviously, the obtained UV–Vis spectra of the separated flavonoids have provided rough information only on the substitution of C-ring, which defines the classification of a flavonoid to a subgroup. In order to identify more structural characteristics of each flavonoid compound, the liquid chromatography–nanospray mass spectrometry was applied.
In general, it has been ascertained, according to the literature, that each compound exhibits a characteristic fragmentation behaviour, which includes, as far as the protonated ion is concerned, dehydration, losses of CO and fission of the C-ring. Fission is based on the retro Diels–Alder cleavages of C-ring bonds, while the resulting fragments constitute a diagnostic tool on the substitution of A- and B-rings. According to the nomenclature of Ma et al. (1997), each fragment is denoted by the combined use of i,j A+ or i,j B+. A and B represent the intact ring, while the superscript on the left indicates the broken bonds of the parent ion.
The fragmentation of the compounds analysed by HPLC–MS/MS is presented in Table 3. Peak I, which according to the UV–Vis spectrum was a flavanone or a dihydroflavonol, presented a protonated ion with m/z = 305 and had identical mass spectrum to standard taxifolin. Taxifolin was then added to D as internal standard and the extract was analysed by HPLC–DAD. The increase in the height and area of peak I confirmed the structure of the unknown compound as taxifolin. The concentration, based on reference curve, was determined equal to 36.2 mg L−1 in D extract and 0.46 g kg in origanum (dry basis).
The [M+H]+ ions of Origanum heracleoticum flavonoid aglycones as well as the defying structure fragments produced by MS2
. | Flavonoid aglycone peaks . | ||||
---|---|---|---|---|---|
I . | II . | III . | IV . | V . | |
[M + H]+ | 305 | 289 | 289 | 273 | 271 |
[M + H-H2O]+ | 287 (98)a | 271 (99) | 271 (15.5) | 255 (4) | 253 (20) |
[M + H-H2O-CO]+ | 259 (100) | 243 (100) | – | – | 225 (18) |
[M + H-H2O-2CO]+ | 231 (2) | 215 (3) | – | – | – |
[M + H-C2H2O]+ | – | – | 247 (5) | 231 (6) | – |
0,2 B+ | – | – | – | – | 121 (7) |
0,4 B+ | – | – | 163 (100) | 147 (100) | – |
1,3 A+ | 153 (35) | 153 (33) | 153 (21) | 153 (81) | 153 (97) |
1,3 B+ | – | – | – | – | 119 (18) |
1,4 B+ | 179 (2) | 163 (3) | – | – | – |
[0,2 A-H2O]+ | 149 (3) | 149 (4.5) | – | – | – |
[M + H-B-ring]+ | 195 (16) | 195 (15) | 179 (20) | 179 (5) | – |
. | Flavonoid aglycone peaks . | ||||
---|---|---|---|---|---|
I . | II . | III . | IV . | V . | |
[M + H]+ | 305 | 289 | 289 | 273 | 271 |
[M + H-H2O]+ | 287 (98)a | 271 (99) | 271 (15.5) | 255 (4) | 253 (20) |
[M + H-H2O-CO]+ | 259 (100) | 243 (100) | – | – | 225 (18) |
[M + H-H2O-2CO]+ | 231 (2) | 215 (3) | – | – | – |
[M + H-C2H2O]+ | – | – | 247 (5) | 231 (6) | – |
0,2 B+ | – | – | – | – | 121 (7) |
0,4 B+ | – | – | 163 (100) | 147 (100) | – |
1,3 A+ | 153 (35) | 153 (33) | 153 (21) | 153 (81) | 153 (97) |
1,3 B+ | – | – | – | – | 119 (18) |
1,4 B+ | 179 (2) | 163 (3) | – | – | – |
[0,2 A-H2O]+ | 149 (3) | 149 (4.5) | – | – | – |
[M + H-B-ring]+ | 195 (16) | 195 (15) | 179 (20) | 179 (5) | – |
a% Relative abundance.
The [M+H]+ ions of Origanum heracleoticum flavonoid aglycones as well as the defying structure fragments produced by MS2
. | Flavonoid aglycone peaks . | ||||
---|---|---|---|---|---|
I . | II . | III . | IV . | V . | |
[M + H]+ | 305 | 289 | 289 | 273 | 271 |
[M + H-H2O]+ | 287 (98)a | 271 (99) | 271 (15.5) | 255 (4) | 253 (20) |
[M + H-H2O-CO]+ | 259 (100) | 243 (100) | – | – | 225 (18) |
[M + H-H2O-2CO]+ | 231 (2) | 215 (3) | – | – | – |
[M + H-C2H2O]+ | – | – | 247 (5) | 231 (6) | – |
0,2 B+ | – | – | – | – | 121 (7) |
0,4 B+ | – | – | 163 (100) | 147 (100) | – |
1,3 A+ | 153 (35) | 153 (33) | 153 (21) | 153 (81) | 153 (97) |
1,3 B+ | – | – | – | – | 119 (18) |
1,4 B+ | 179 (2) | 163 (3) | – | – | – |
[0,2 A-H2O]+ | 149 (3) | 149 (4.5) | – | – | – |
[M + H-B-ring]+ | 195 (16) | 195 (15) | 179 (20) | 179 (5) | – |
. | Flavonoid aglycone peaks . | ||||
---|---|---|---|---|---|
I . | II . | III . | IV . | V . | |
[M + H]+ | 305 | 289 | 289 | 273 | 271 |
[M + H-H2O]+ | 287 (98)a | 271 (99) | 271 (15.5) | 255 (4) | 253 (20) |
[M + H-H2O-CO]+ | 259 (100) | 243 (100) | – | – | 225 (18) |
[M + H-H2O-2CO]+ | 231 (2) | 215 (3) | – | – | – |
[M + H-C2H2O]+ | – | – | 247 (5) | 231 (6) | – |
0,2 B+ | – | – | – | – | 121 (7) |
0,4 B+ | – | – | 163 (100) | 147 (100) | – |
1,3 A+ | 153 (35) | 153 (33) | 153 (21) | 153 (81) | 153 (97) |
1,3 B+ | – | – | – | – | 119 (18) |
1,4 B+ | 179 (2) | 163 (3) | – | – | – |
[0,2 A-H2O]+ | 149 (3) | 149 (4.5) | – | – | – |
[M + H-B-ring]+ | 195 (16) | 195 (15) | 179 (20) | 179 (5) | – |
a% Relative abundance.
Compound II (m/z = 289) differed from compound I per 16 mass units and produced similar pattern fragments with taxifolin, i.e. [M+H−H2O]+ (m/z = 271), [M+H−H2O−CO]+ (m/z = 243), [M+H−H2O−2CO]+ (m/z = 215); therefore, the compound could be a dihydroflavonol with lack of one oxygen atom from A- or B-ring. The presence of fragments with m/z = 153, 149 and 195 (Table 3), identical to fragments including A-ring of taxifolin and with the same relative abundance, indicates that A-ring is di-OH-substituted. Fragment with m/z = 163 corresponds to 1,4 B+ of taxifolin minus 16 mass units, indicating further the monohydroxy substitution of B-ring. Consequently, compound II is an A-di-OH, B-mono-OH dihydroflavonol, e.g. the dihydrokaempferol or isomer. This is confirmed by Le Gall et al. (2003) who also detected these fragments analysing dihydrokaempferol.
Component III presented protonated ion with the same m/z as compound II. According to the fragmentation and HPLC–DAD analysis with internal standard, compound III was identified as eriodictyol, and its concentration was calculated as equal to 34.3 mg L−1 in D extract and 0.44 g kg−1 in dried origanum. As far as IV (m/z = 273) is concerned, it corresponded to an A-di-OH, B-mono-OH flavanone, as indicated by the 16 mass units difference with eriodictyol and their similar pattern of fragmentation. The fragment with m/z = 153 corresponded to the 1,3 A+ and was established as the most standard of the mass spectra defying the dihydroxy structure of A-ring.
On the contrary, component V (m/z 271) primarily dehydrated in MS analysis [M+H−H2O]+ (m/z 253), while the product ion further lost one carbonyl group [M+H−H2O−CO]+ (m/z 225). The mass of protonated ion could correspond to a trihydroxyflavone or a dihydroxy-substituted flavonol. The compound was identified as apigenin, as Ma et al. (1997), Avallone et al. (2000) and Bouéet al. (2003) detected identical fragmentation for apigenin. Analysis by HPLC–DAD of D and ED fractions with internal standard confirmed apigenin as component V.
Peak VIII (flavone or flavonol according to UV–Vis spectrum) gave an intense protonated ion [M+H]+ at m/z = 433, the main fragment of which was [M+H−162]+m/z = 271. The loss of 162 mass units is indicative of a hexose sugar; MS2 fragmented the m/z = 271 ion, which matched fragmentation of apigenin. These results identify compound VIII as apigenin glycoside. Bouéet al. (2003) identified a compound with similar UV–Vis and MS spectral properties as compound VIII, as apigenin-7-O-β-d-glucoside. All of the identified compounds by HPLC–DAD or HPLC–MS–MS from the diethyl ether and ethanol extracts are presented in Table 4.
Retention timea (min) . | Compound . |
---|---|
Diethyl ether extract | |
27.5 | I: taxifolin |
35.4 | II: A-di-OH, B-mono-OH dihydroflavonol |
39.5 | III: eriodictyol |
43.6 | IV: A-di-OH, B-mono-OH flavanone |
47.0 | V: apigenin |
49.5 | VI: carvacrol |
Ethanol extract | |
38.3 | VII: rosmarinic acid |
39.4 | VIII: apigenin glycoside |
47.0 | V: apigenin |
49.6 | VI: carvacrol |
Retention timea (min) . | Compound . |
---|---|
Diethyl ether extract | |
27.5 | I: taxifolin |
35.4 | II: A-di-OH, B-mono-OH dihydroflavonol |
39.5 | III: eriodictyol |
43.6 | IV: A-di-OH, B-mono-OH flavanone |
47.0 | V: apigenin |
49.5 | VI: carvacrol |
Ethanol extract | |
38.3 | VII: rosmarinic acid |
39.4 | VIII: apigenin glycoside |
47.0 | V: apigenin |
49.6 | VI: carvacrol |
aThe retention times of the compounds during HPLC–DAD analyses.
Retention timea (min) . | Compound . |
---|---|
Diethyl ether extract | |
27.5 | I: taxifolin |
35.4 | II: A-di-OH, B-mono-OH dihydroflavonol |
39.5 | III: eriodictyol |
43.6 | IV: A-di-OH, B-mono-OH flavanone |
47.0 | V: apigenin |
49.5 | VI: carvacrol |
Ethanol extract | |
38.3 | VII: rosmarinic acid |
39.4 | VIII: apigenin glycoside |
47.0 | V: apigenin |
49.6 | VI: carvacrol |
Retention timea (min) . | Compound . |
---|---|
Diethyl ether extract | |
27.5 | I: taxifolin |
35.4 | II: A-di-OH, B-mono-OH dihydroflavonol |
39.5 | III: eriodictyol |
43.6 | IV: A-di-OH, B-mono-OH flavanone |
47.0 | V: apigenin |
49.5 | VI: carvacrol |
Ethanol extract | |
38.3 | VII: rosmarinic acid |
39.4 | VIII: apigenin glycoside |
47.0 | V: apigenin |
49.6 | VI: carvacrol |
aThe retention times of the compounds during HPLC–DAD analyses.
Correlation of antioxidant activity with composition of Origanum heracleoticum fractions
The ED fraction consists of three major components: rosmarinic acid, apigenin and carvacrol. Rosmarinic acid is a very common cinnamic acid derivative (Fig. 4) in the members of Lamiaceae family (Justesen et al., 2000; Triantaphyllou et al., 2001; Exarchou et al., 2002; Dorman et al., 2004) and possesses significant antioxidant and antiradical activity, as has been presented by several researchers (Hotta et al., 2002). Owing to the structure of the two catecholic groups, the hydroxyl hydrogens abstraction by free radicals is favourable. Therefore, the low EC50 as well as the high protection parameter of ED fraction can be mainly ascribed to rosmarinic acid with much weaker contribution of the other components. Apigenin or its glycosides have been reported as flavonoid constituents in origanum species by other researchers (Vekiari et al., 1993; Justesen, 2000; Justesen & Knuthsen, 2001; Dorman et al., 2004). Apigenin seems to have low activity when tested alone with DPPH (Hotta et al., 2002), although extracts containing apigenin proved effective in oils (Vekiari et al., 1993). Carvacrol is also a low-efficiency antioxidant and has been reported to have an EC50 around 17 000 g kg−1 DPPH (Kulisic et al., 2004). The low activity against DPPH of the EEAc fraction is explained by the existence of apigenin glycoside as main constituent. Flavonoid glycosides are not soluble in oils; therefore this fraction cannot be used for oil stabilisation.
D extract contains two compounds that also possess catecholic moiety and are active against free radicals, taxifolin and eriodictyol. Pure taxifolin has displayed significant activity against DPPH radical and oil oxidation in previous experiments we conducted (Tsimogiannis & Oreopoulou, 2004), while in the same research eriodictyol exhibited weaker activity. The rest flavonoids of this extract have a B-monohydroxy substitution and are expected to attribute moderately to the overall activity of the extract.
P extract consists mainly of the essential oil of the plant. Essential oils of oregano and other herbs have exhibited antioxidant activity in oils and lard (Özcan & Akgül, 1995; Milos et al., 2000; Kulisic et al., 2004); however, Özcan & Akgül (1995) observed that their activity is considerably lower than the activity of the methanol extracts of the same herbs. In particular, thymol and carvacrol, main components of the essential oil of oregano, showed weak (Milos et al., 2000) and moderate (Kulisic et al., 2004) antioxidant activities, respectively.
Conclusions
Conclusively, O. heracleoticum is a member of Lamiaceae family, which presents enough similar components, such as carvacrol, taxifolin, eriodictyol, apigenin and rosmarinic acid, with other O. vulgare subspecies of the Mediterranean region. Thymol was a minor component in O. heracleoticum, while other constituents of the essential oil are presented in Table 2. The high concentrations in taxifolin, eriodictyol and rosmarinic acid classify O. heracleoticum among potent sources of natural antioxidants as proved by our experimental results.