-
PDF
- Split View
-
Views
-
Cite
Cite
Adela Herraiz-Martínez, Anna Llach, Carmen Tarifa, Jorge Gandía, Verónica Jiménez-Sabado, Estefanía Lozano-Velasco, Selma A Serra, Alexander Vallmitjana, Eduardo Vázquez Ruiz de Castroviejo, Raúl Benítez, Amelia Aranega, Christian Muñoz-Guijosa, Diego Franco, Juan Cinca, Leif Hove-Madsen, The 4q25 variant rs13143308T links risk of atrial fibrillation to defective calcium homoeostasis, Cardiovascular Research, Volume 115, Issue 3, 1 March 2019, Pages 578–589, https://doi.org/10.1093/cvr/cvy215
- Share Icon Share
Abstract
Single nucleotide polymorphisms on chromosome 4q25 have been associated with risk of atrial fibrillation (AF) but the exiguous knowledge of the mechanistic links between these risk variants and underlying electrophysiological alterations hampers their clinical utility. Here, we tested the hypothesis that 4q25 risk variants cause alterations in the intracellular calcium homoeostasis that predispose to spontaneous electrical activity.
Western blotting, confocal calcium imaging, and patch-clamp techniques were used to identify mechanisms linking the 4q25 risk variants rs2200733T and rs13143308T to defects in the calcium homoeostasis in human atrial myocytes. Our findings revealed that the rs13143308T variant was more frequent in patients with AF and that myocytes from carriers of this variant had a significantly higher density of calcium sparks (14.1 ± 4.5 vs. 3.1 ± 1.3 events/min, P = 0.02), frequency of transient inward currents (ITI) (1.33 ± 0.24 vs. 0.26 ± 0.09 events/min, P < 0.001) and incidence of spontaneous membrane depolarizations (1.22 ± 0.26 vs. 0.56 ± 0.17 events/min, P = 0.001) than myocytes from patients with the normal rs13143308G variant. These alterations were linked to higher sarcoplasmic reticulum calcium loading (10.2 ± 1.4 vs. 7.3 ± 0.5 amol/pF, P = 0.01), SERCA2 expression (1.37 ± 0.13 fold, P = 0.03), and RyR2 phosphorylation at ser2808 (0.67 ± 0.08 vs. 0.47 ± 0.03, P = 0.01) but not at ser2814 (0.28 ± 0.14 vs. 0.31 ± 0.14, P = 0.61) in patients carrying the rs13143308T risk variant. Furthermore, the presence of a risk variant or AF independently increased the ITI frequency and the increase in the ITI frequency observed in carriers of the risk variants was exacerbated in those with AF. By contrast, the presence of a risk variant did not affect the amplitude or properties of the L-type calcium current in patients with or without AF.
Here, we identify the 4q25 variant rs13143308T as a genetic risk marker for AF, specifically associated with excessive calcium release and spontaneous electrical activity linked to increased SERCA2 expression and RyR2 phosphorylation.
1. Introduction
Atrial fibrillation (AF) is the most common cardiac arrhythmia and a major contributory factor to increase mortality and risk of cerebrovascular embolism.1 Even though AF has been associated with a number of electrophysiological, molecular, and structural alterations that favour the maintenance and self-perpetuation of the arrhythmia,2,3 current treatments of AF are often deficient or ineffective.
Among the electrophysiological alterations, several studies have reported disturbances in the calcium homoeostasis4–7 and malfunctioning of the sarcoplasmic reticulum (SR).4,5,7–10 Indeed, myocytes from patients with AF depict a high rate of spontaneous SR calcium release,4 which has been linked to deficient phosphodiesterase activity,11 reduced phosphatase activity,12 or excessive protein kinase A5,9 or calmodulin kinase type II-dependent8,10,13 phosphorylation of the cardiac ryanodine receptor (RyR2). Importantly, spontaneous calcium waves can induce arrhythmogenic membrane depolarizations in atrial myocytes from patients with AF,10 and occur both in resting and electrically stimulated human atrial myocytes.14,15 Moreover, transgenic mouse models with high rates of spontaneous calcium release are more prone to present arrhythmia10,16–18; supporting the notion that spontaneous SR calcium release plays a central role in these arrhythmogenic processes.
Thus, electrophysiological mechanistic studies have been narrowing down the molecular mechanisms that underlie defective calcium homoeostasis in AF. Still, specific molecular triggers of AF are not well defined, except from mutations in ion channels associated to familial AF19–21 that only account for a minority of the patients with AF. However, genome-wide association studies have identified several single nucleotide polymorphisms (SNPs) on chromosomes 4q25, 1q21, and 16q22 that are associated with increased risk of AF,22,23 and recent meta-analyses have further expanded the number of risk variants.24 The most striking arrhythmogenic variants remain those located at 4q2525 and some of them have been reported to modify the risk of AF recurrence after catheter ablation26 and the response to antiarrhythmic drug treatment.27 Furthermore, the bicoid-related homeodomain transcription factor Pitx2, which is located in the vicinity of the 4q25 variants has been postulated as a molecular link between risk variants at 4q25 and AF.22 However, studies on Pitx2 expression in patients with 4q25 risk variants28,29 and in patients with AF29–31 have not been able to link AF to changes in Pitx2 expression or function caused by 4q25 risk variants. On the other hand, no studies have so far investigated if the 4q25 risk variants entail electrophysiological disturbances observed in atrial myocytes from patients with AF that have been associated to alterations in the intracellular calcium homoeostasis. Therefore, here, we tested the hypothesis that risk variants on chromosome 4q25 cause alterations in the intracellular calcium homoeostasis that predispose to spontaneous electrical activity.
2. Methods
2.1 Human biological samples and SNP genotyping
A total of 605 blood samples were genotyped for the presence of the normal 4q25 variants rs2200733C and rs13143308G or for the corresponding AF risk variants rs2200733T and rs13143308T. Polymerase chain reaction amplification of the SNPs rs2200733 and rs13143308 were carried out using flanking oligonucleotides (Supplementary material online, Methods section) followed by direct sequencing. For all atrial samples used for electrophysiological and/or molecular biological studies, genotyping was done for batches of samples after experiments had been performed and analysed. Right atrial myocardial samples were collected from 342 genotyped patients undergoing cardiac surgery with extracorporeal circulation. Each patient gave written consent to obtain blood and tissue samples. The latter would otherwise have been discarded during the surgical intervention. The study was approved by the Ethics Committees of the Spanish National DNA Bank (BNADN, Salamanca), Hospital de la Santa Creu i Sant Pau (Barcelona), and of the University of Jaén; and the investigation conforms to the principles outlined in the Declaration of Helsinki.
2.2 Clinical characterization
Clinical information relevant to this study and included in Table 1 was collected from the clinical records by a clinician. Medication refers to the patient medication prescribed before the surgical intervention. Patients without any incidents of AF in the clinical record were considered free of the arrhythmia. We cannot rule out that some patients with paroxysmal AF may not have been detected, but we assume that this error is similar for patients with and without risk variants. Patients were classified as having paroxysmal or chronic AF according to the information available in the clinical record. Chronic AF included patients having persistent or long-term persistent AF. To reduce fractioning of the data, patients with paroxysmal and chronic AF were pooled into a single group of AF patients. This grouping was done because unpublished data from our laboratory and published data7 show that spontaneous calcium release is already elevated in patients with paroxysmal AF.
Clinical characterization of the patients included in electrophysiological and molecular biological analyses
. | Normal (n = 214) . | Risk (n = 128) . | P-value . |
---|---|---|---|
Age (years) | 68.3 ± 0.7 | 68.0 ± 1 | 0.8 |
Height (cm) | 164.4 ± 0.7 | 164.4 ± 0.8 | 1 |
Weight (kg) | 75.7 ± 0.9 | 75.3 ± 1.2 | 0.8 |
Cardiovascular disease, % (n) | |||
Arterial hypertension | 65 (139) | 72 (92) | 0.2 |
Diabetes | 41 (88) | 27 (34) | 0.01 |
Aortic valve disease | 49 (104) | 57 (73) | 0.15 |
Mitral valve disease | 24 (51) | 24 (31) | 1 |
Tricuspid valve disease | 12 (26) | 13 (16) | 1 |
Ischaemic heart disease | 64 (136) | 50 (64) | 0.02 |
Echocardiography | |||
Left atrial diameter (mm) | 43.2 ± 0.6 | 43.0 ± 0.8 | 0.8 |
Left atrial diameter index | 2.40 ± 0.05 | 2.38 ± 0.05 | 0.8 |
LVEF (%) | 55.7 ± 1 | 57.6 ± 1.2 | 0.2 |
Pharmacological treatment, % (n) | |||
ACE-inhibitors | 37 (79) | 35 (45) | 0.8 |
ARB | 18 (39) | 21 (27) | 0.6 |
β-blockers | 49 (105) | 52 (67) | 0.6 |
Calcium antagonists | 22 (47) | 25 (32) | 0.6 |
Nitrates | 25 (54) | 23 (30) | 0.8 |
Dicoumarin | 25 (54) | 30 (38) | 0.4 |
Acetyl salicylic acid | 45 (96) | 46 (59) | 0.9 |
Statins | 61 (130) | 66 (85) | 0.3 |
. | Normal (n = 214) . | Risk (n = 128) . | P-value . |
---|---|---|---|
Age (years) | 68.3 ± 0.7 | 68.0 ± 1 | 0.8 |
Height (cm) | 164.4 ± 0.7 | 164.4 ± 0.8 | 1 |
Weight (kg) | 75.7 ± 0.9 | 75.3 ± 1.2 | 0.8 |
Cardiovascular disease, % (n) | |||
Arterial hypertension | 65 (139) | 72 (92) | 0.2 |
Diabetes | 41 (88) | 27 (34) | 0.01 |
Aortic valve disease | 49 (104) | 57 (73) | 0.15 |
Mitral valve disease | 24 (51) | 24 (31) | 1 |
Tricuspid valve disease | 12 (26) | 13 (16) | 1 |
Ischaemic heart disease | 64 (136) | 50 (64) | 0.02 |
Echocardiography | |||
Left atrial diameter (mm) | 43.2 ± 0.6 | 43.0 ± 0.8 | 0.8 |
Left atrial diameter index | 2.40 ± 0.05 | 2.38 ± 0.05 | 0.8 |
LVEF (%) | 55.7 ± 1 | 57.6 ± 1.2 | 0.2 |
Pharmacological treatment, % (n) | |||
ACE-inhibitors | 37 (79) | 35 (45) | 0.8 |
ARB | 18 (39) | 21 (27) | 0.6 |
β-blockers | 49 (105) | 52 (67) | 0.6 |
Calcium antagonists | 22 (47) | 25 (32) | 0.6 |
Nitrates | 25 (54) | 23 (30) | 0.8 |
Dicoumarin | 25 (54) | 30 (38) | 0.4 |
Acetyl salicylic acid | 45 (96) | 46 (59) | 0.9 |
Statins | 61 (130) | 66 (85) | 0.3 |
Values are represented as mean ± standard error or percentage and number (n) of patients with the condition. P-values for differences between the normal and risk groups are given on the right.
ACE-inhibitors, angiotensin converting enzyme inhibitors; ARB, angiotensin receptor blockers; LVEF, left ventricular ejection fraction.
Clinical characterization of the patients included in electrophysiological and molecular biological analyses
. | Normal (n = 214) . | Risk (n = 128) . | P-value . |
---|---|---|---|
Age (years) | 68.3 ± 0.7 | 68.0 ± 1 | 0.8 |
Height (cm) | 164.4 ± 0.7 | 164.4 ± 0.8 | 1 |
Weight (kg) | 75.7 ± 0.9 | 75.3 ± 1.2 | 0.8 |
Cardiovascular disease, % (n) | |||
Arterial hypertension | 65 (139) | 72 (92) | 0.2 |
Diabetes | 41 (88) | 27 (34) | 0.01 |
Aortic valve disease | 49 (104) | 57 (73) | 0.15 |
Mitral valve disease | 24 (51) | 24 (31) | 1 |
Tricuspid valve disease | 12 (26) | 13 (16) | 1 |
Ischaemic heart disease | 64 (136) | 50 (64) | 0.02 |
Echocardiography | |||
Left atrial diameter (mm) | 43.2 ± 0.6 | 43.0 ± 0.8 | 0.8 |
Left atrial diameter index | 2.40 ± 0.05 | 2.38 ± 0.05 | 0.8 |
LVEF (%) | 55.7 ± 1 | 57.6 ± 1.2 | 0.2 |
Pharmacological treatment, % (n) | |||
ACE-inhibitors | 37 (79) | 35 (45) | 0.8 |
ARB | 18 (39) | 21 (27) | 0.6 |
β-blockers | 49 (105) | 52 (67) | 0.6 |
Calcium antagonists | 22 (47) | 25 (32) | 0.6 |
Nitrates | 25 (54) | 23 (30) | 0.8 |
Dicoumarin | 25 (54) | 30 (38) | 0.4 |
Acetyl salicylic acid | 45 (96) | 46 (59) | 0.9 |
Statins | 61 (130) | 66 (85) | 0.3 |
. | Normal (n = 214) . | Risk (n = 128) . | P-value . |
---|---|---|---|
Age (years) | 68.3 ± 0.7 | 68.0 ± 1 | 0.8 |
Height (cm) | 164.4 ± 0.7 | 164.4 ± 0.8 | 1 |
Weight (kg) | 75.7 ± 0.9 | 75.3 ± 1.2 | 0.8 |
Cardiovascular disease, % (n) | |||
Arterial hypertension | 65 (139) | 72 (92) | 0.2 |
Diabetes | 41 (88) | 27 (34) | 0.01 |
Aortic valve disease | 49 (104) | 57 (73) | 0.15 |
Mitral valve disease | 24 (51) | 24 (31) | 1 |
Tricuspid valve disease | 12 (26) | 13 (16) | 1 |
Ischaemic heart disease | 64 (136) | 50 (64) | 0.02 |
Echocardiography | |||
Left atrial diameter (mm) | 43.2 ± 0.6 | 43.0 ± 0.8 | 0.8 |
Left atrial diameter index | 2.40 ± 0.05 | 2.38 ± 0.05 | 0.8 |
LVEF (%) | 55.7 ± 1 | 57.6 ± 1.2 | 0.2 |
Pharmacological treatment, % (n) | |||
ACE-inhibitors | 37 (79) | 35 (45) | 0.8 |
ARB | 18 (39) | 21 (27) | 0.6 |
β-blockers | 49 (105) | 52 (67) | 0.6 |
Calcium antagonists | 22 (47) | 25 (32) | 0.6 |
Nitrates | 25 (54) | 23 (30) | 0.8 |
Dicoumarin | 25 (54) | 30 (38) | 0.4 |
Acetyl salicylic acid | 45 (96) | 46 (59) | 0.9 |
Statins | 61 (130) | 66 (85) | 0.3 |
Values are represented as mean ± standard error or percentage and number (n) of patients with the condition. P-values for differences between the normal and risk groups are given on the right.
ACE-inhibitors, angiotensin converting enzyme inhibitors; ARB, angiotensin receptor blockers; LVEF, left ventricular ejection fraction.
2.3 Human atrial tissue and myocyte isolation
Samples were excised from the right atrial appendage of patients undergoing cardiac surgery with extracorporeal circulation, stored immediately in cold oxygenated Tyrode solution and snap-frozen or used for myocyte isolation within 5–10 min after excision. For myocyte isolation, the sample was cut into small fragments and digested in a calcium-free Tyrode solution with collagenase, proteinase, and fatty acid-free albumin as described in the Supplementary material online, Methods section. Only elongated cells with clear cross striations and without abnormal granulation were used.
2.4 Western blot and immunofluorescent labelling
Proteins were separated by SDS-PAGE and electrotransferred onto polyvinylidene diflouride membranes. Membranes were incubated with primary antibodies against SERCA2a, calsequestrin-2(CSQ-2), NCX-1, phospholamban (PLB), PLB-s16, or PLB-t17 (Supplementary material online, Methods section for details). Detection was performed using horseradish peroxidase-labelled IgG and the Supersignal detection system.
Isolated myocytes were fixed with paraformaldehyde for 5 min at room temperature. Subsequently, cells were inmunofluorescently labelled with the primary antibodies mouse anti-RyR2 and rabbit anti-ser2808-P or anti-ser2814P. After labelling, cells were washed again and stored at 4°C until visualization (Supplementary material online, Methods section for further details).
2.5 Patch-clamp technique
Calcium current (ICa) was elicited by a 200 ms depolarization to 0 mV, and spontaneous transient inward currents (ITI) were measured at holding potentials of −80 or −50 mV using whole cell voltage-clamp in the perforated patch configuration with a HEKA EPC-10 amplifier (Supplementary material online, Methods section for further details). Experiments began when the series resistance did not decrease further, and cells were discarded if it was larger than 6 times the pipette resistance.
The SR calcium load was determined from the charge carried by the Na+-Ca2+ exchange (NCX) current elicited by rapid exposure to 10 mM caffeine (for 5 s) that releases the calcium stored in the SR into the cytosol. The charge was converted to amoles (10−18 mol) of calcium released from the SR, assuming a stoichiometry of 3Na+:1Ca2+ for the NCX and normalized to the cell capacitance. The remaining calcium available for extrusion by the NCX at a given time point during the caffeine exposure was determined as the SR calcium load minus the NCX time integral at that time point (Supplementary material online, Figure S1). Experimental protocols were linked and executed sequentially. Erroneous measurement(s) were eliminated, giving rise to variations in the total number of experiments for different currents. Most of these cases occurred because exposure of myocytes to caffeine produced sudden increases in the leakage current or irreversible cell contracture in some myocytes.
Membrane potentials were measured in the current-clamp configuration using K+-containing intra- and extracellular media. The holding current was varied in order to achieve a resting membrane potential near −80 mV and hence compare the effect of normal and risk variants on the amplitude and frequency of spontaneous membrane depolarizations without interference of concurrent alterations in the membrane potential. We did not attempt to measure the native resting membrane potential because the cholinergic tone is washed out in isolated human atrial myocytes where acetyl choline activated potassium channels play a key role in setting the resting membrane potential32 and because their expression and activation is altered in AF.32,33
2.6 Confocal calcium imaging
Confocal calcium images (512 × 140 pixels) were recorded at 90 Hz. To detect calcium sparks, a wavelet-based detection method was applied to the normalized time-dependent fluorescence signal zi(t) at every pixel in order to detect candidates for Ca2+ release events (Supplementary material online, Methods section for details on signal processing). After supervised validation and merging, sparks that coincided spatially (within a radius of 2 µm) were pooled into a common spark site and calcium traces were generated for each spark site. Each spark was characterized by its amplitude, its duration characterized as the full duration at half maximum and its width characterized as the full width at half maximum. The spark frequency was calculated as the number of sparks/cell/s or normalized to the cell area within the confocal plane and expressed as sparks/µm2/s. Additionally, the number of spark sites were expressed as sites/cell or normalized to the cell area and given as sites/µm2. The average spark frequency per site was given as sparks/site/s.
2.7 Statistical analysis
Electrophysiological and molecular biological analyses of human atrial samples were performed without knowledge about clinical data or genotypes and clinicians gathering the clinical data did not know the experimental results. Data sets were analysed with SPSS statistical software. Unless otherwise stated, values were averaged for each patient. Mean values are indicated with black horizontal bars and 95% confidence intervals with grey horizontal bars in dot plots or given as mean ± SEM, when indicated. Statistical significance was evaluated using the Fisher’s exact test for categorical data. The Student’s t-test was used for paired or unpaired comparisons and a Mann–Whitney test was used for data that did not have a normal distribution. ANOVA analysis or a linear regression model was used for comparison of multiple effects as indicated. For the statistical analysis of the effects of the different genotypes containing different SNPs, we used a linear regression model taking into account the effects of the genotype, the presence of AF, and whether the patients were undergoing mitral valve replacement. Based on the linear regression model, the effect of AF and the most common genotypes was estimated and given as mean ± SEM. Statistically significant effects are indicated with P-values or *P < 0.05, **P < 0.01, and ***P < 0.001.
3. Results
3.1 4q25 risk variants in the study population
Genotyping was done in 605 patients for the 4q25 risk variants rs2200733T and rs13143308T that are highly associated with AF risk.22,34 The rs13143308T risk variant was more frequent in patients with AF (P = 0.004) while the rs2200733T risk variant depicted a non-significant trend (Figure 1A). Furthermore, the rs2200733T risk variant co-segregated with rs13143308T (P < 0.001; Figure 1B).
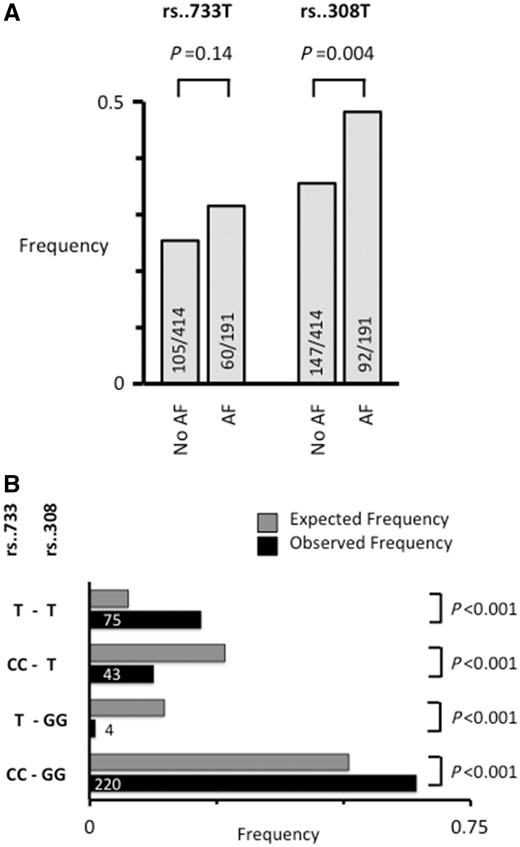
Frequency and segregation of the 4q25 loci rs2200733 and rs13143308. (A) The frequency of the rs2200733T (rs..733T) and rs13143308T (rs..308T) risk variants on chromosome 4q25. Number of patients genotyped are given for each risk variant in patients without (No AF) and with AF. (B) Relative frequency of rs..733 and rs..308 genotypes in 342 patients donating right atrial tissue (black bars). T at rs..733 indicates the presence of at least one risk allele, and T at rs..308 indicates the presence of at least one risk allele at this locus. Grey bars indicate the expected frequency of the same genotypes based on the allele frequencies. P-value for difference between observed and expected frequencies are given on the right.
Atrial tissue samples and/or myocytes from 342 of these patients were used for further clinical, electrophysiological, and/or molecular biological analysis. The clinical and echocardiographic data as well as pharmacological treatments of these patients are summarized in Table 1.
3.2 Impact of the 4q25 risk variant rs13143308T on spontaneous electrical activity
Since the rs2200733 and rs13143308 variants are located next to each other30 and the rs2200733T risk variant occurred together with the rs13143308T risk variant in myocytes isolated from all but one of the patients, the specific effect of the rs2200733T variant could not be measured directly. Therefore, this analysis focused on how the presence of a rs13143308T risk variant affected the calcium homoeostasis and spontaneous electrical activity. First, we analysed the frequency of ITI resulting from extrusion by the NCX of spontaneously released calcium in patients without AF in order to avoid confounding effects of the arrhythmia on calcium homoeostasis and electrical activity. In these patients, the ITI frequency was significantly higher in myocytes from carriers of the rs13143308T risk variant (Figure 2A and B) while the ITI-amplitudes were similar for patients with and without risk alleles (Figure 2C). Current-clamp experiments, performed in myocytes from a subset of patients to determine the net impact of calcium release-induced NCX on the membrane potential, showed a significantly higher frequency of spontaneous membrane depolarizations (DADs) (Figure 2D-E) with unchanged amplitudes (Figure 2F) in patients carrying the risk variant. In three of the patients with risk variants, some of the DADs were large enough to trigger spontaneous action potentials.
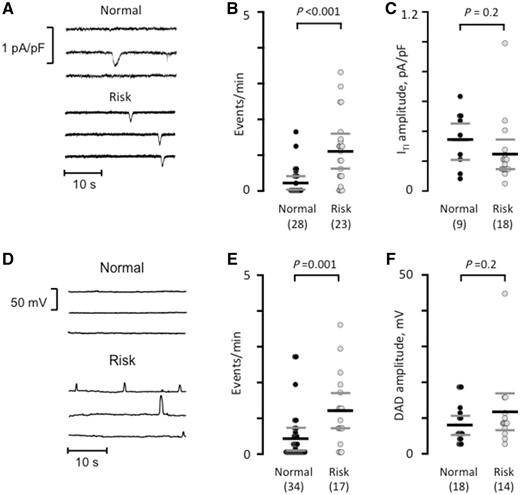
The risk variant rs13143308T increases spontaneous electrical activity. (A) Spontaneous ITI currents in patients with a normal or a risk variant at rs13143308. (B) ITI frequency measured in myocytes without (normal) or with a risk variant. (C) ITI amplitude measured in the myocytes from B. (D) Membrane potential recordings at −80 mV in myocytes from a patient with normal and one with a risk variant. (E) Frequency of spontaneous membrane depolarizations at −80 mV in patients with normal and risk variants. (F) Amplitude of the depolarizations in the myocytes from (E). P-values for the unpaired t-tests (C and F) or Mann–Whitney tests (B and E) are given above data points and the number of patients in parentheses.
3.3 Impact of the 4q25 risk variant rs13143308T on calcium homoeostasis
Using frame-scanning confocal calcium imaging to visualize local calcium release from RyR2 clusters (calcium sparks) in a longitudinal plane of myocytes from patients without risk variants at rs2200733 and rs13143308 (Figure 3A) and with at least one risk variant at rs13143308 (Figure 3B) revealed that the spark frequency was significantly higher in carriers of the risk variant (Figure 3C). This was due to a higher density of spark sites (Figure 3D) rather than a higher firing frequency per site (Figure 3E). Analysis of the kinetics (Figure 4A) and the width (Figure 4B) of calcium sparks showed that the 4q25 risk variants tended to reduce the spark amplitude (Figure 4C) but did neither affect the duration (Figure 4D) nor the width of the calcium sparks (Figure 4E).
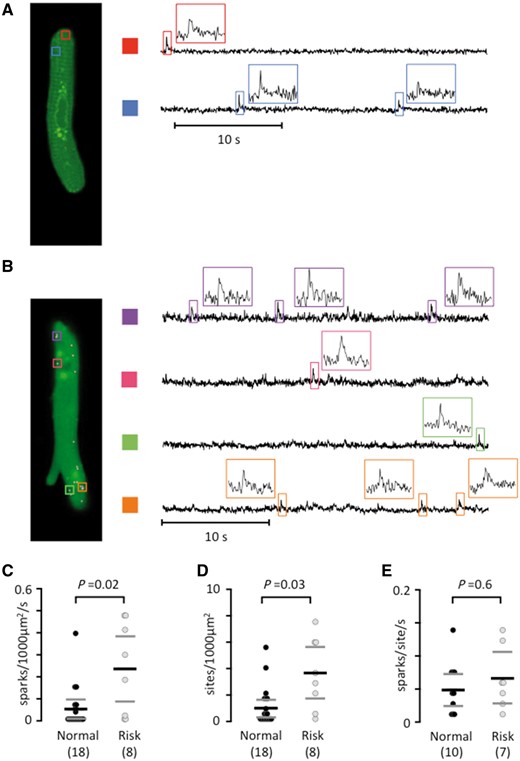
The rs13143308T risk variant increases the calcium spark density. (A) Longitudinal plane of a human atrial myocyte from a patient without a 4q25 risk variant, with indication of two spark sites on the left (colored rectangles). Calcium signals are shown for each spark site on the right. Insets show calcium signals for individual sparks. (B) Sparks in a human atrial myocyte from a patient with a 4q25 risk variant. (C) Spark frequency. (D) Spark sites per cell. (E) Spark firing frequency. P-values from the unpaired t-tests (E) or Mann–Whitney tests (C and D) are given above data points and the number of patients in parentheses.
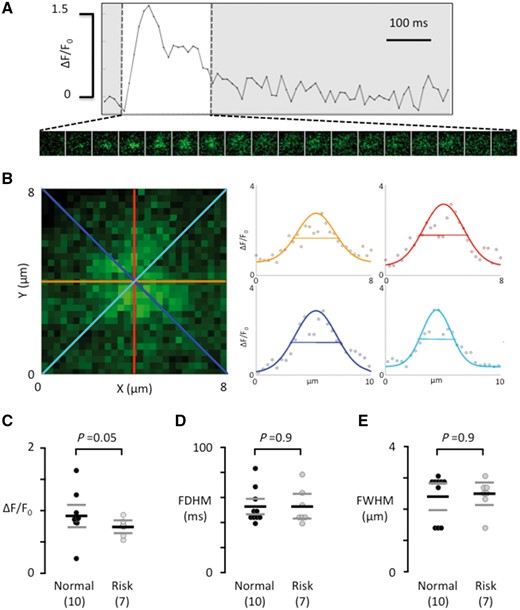
Calcium spark properties do not depend on the genotype at rs13143308. (A) Ca2+ transient from a single spark site. Consecutive 8 × 8 μm images of the calcium spark are shown below the Ca2+ transient during its rise and decay (white window area). (B) Enlarged image of the calcium spark in A, recorded at its maximal amplitude. The profile of the calcium spark is shown on the right for each of the four coloured axes outlined in the image on the left. The width of the calcium spark at half maximum was calculated as the average of the four axes. (C) Calcium spark amplitude. (D) Calcium spark duration at half maximum. (E) Width of the calcium spark at half maximum. Values are from the same myocytes as in Figure 2. P-values for the unpaired t-tests (C) or Mann–Whitney tests (D and E) are given above data points and the number of patients is given in parentheses.
Immuofluorescent labelling of total and ser2808 or ser2814 phosphorylated RyR2 clusters revealed that the presence of a risk variant did not affect the density of RyR2 clusters (0.59 ± 0.10 vs. 0.56 ± 0.07 clusters/µm2). However, it significantly increased the ser2808/RyR2 (Figure 5A) but not ser2814/RyR2 (Figure 5B) intensity ratios, suggesting that the rs13143308T variant favours RyR2 phosphorylation at ser2808 but not at ser2814. The weak ser2814 phosphorylation in the human atrial myocytes is in contrast to a clear ser2814 labelling in mouse ventricular myocytes under the same conditions (Supplementary material online, Figure S2), suggesting that it is not a methodological problem. The caffeine releasable SR calcium load estimated from the time integral of the caffeine-induced NCX current (Figure 5C) was also significantly higher in atrial myocytes from patients with a risk variant (Figure 5D), which could potentially increase the number of RyR2 clusters that reach the threshold for spontaneous calcium release and thereby the density of calcium spark sites. By contrast, plotting the NCX current against the remaining calcium available for extrusion by the NCX in order to assess the NCX activity at different calcium levels (Figure 5E) showed no difference between the resulting slopes for patients with normal and risk variants (Figure 5F) suggesting that the NCX rate is not affected by the rs13143308T risk variant.

RyR2 phosphorylation, SR calcium loading, and SERCA2a expression is increased in patients with a rs13143308T risk variant. (A) Visualization of total RyR2 clusters (green), ser2808 phosphorylated clusters (red), and overlay of the two channels in a human atrial myocyte. The degree of ser2808 phosphorylation was estimated as the ratio of the ser2808/RyR2 intensity for each RyR2 cluster (right panel). (B) Visualization of total RyR2 clusters (green), ser2814 phosphorylated clusters (red), and overlay of the two channels in a human atrial myocyte. The degree of ser2814 phosphorylation was estimated as the ratio of the ser2814/RyR2 intensity for each RyR2 cluster (right panel). White scale bars: 20 μm. (C) Caffeine-induced NCX currents (top) their time integral (middle) and the calcium available for extrusion by the NCX (bottom) for a normal and a risk variant. (D) Caffeine releasable SR calcium content. (E) Relationship between the caffeine-induced NCX current and the calcium available for extrusion by the NCX for a normal and a risk variant. (F) Mean slope of the relationship between NCX activity and calcium available for extrusion. (G) Immunoblots of SERCA2a, CSQ-2, NCX-1, and GADPH in right atrial samples from patients with normal or risk variants at 4q25. GADPH served as reference. (H) Mean SERCA2a, CSQ-2, and NCX-1 expression levels. Values were normalized to the mean expression level in the normal variant. P-values for the unpaired t-tests (D, F, and H) or Mann–Whitney tests (A and B) are given above graphs and the number of patients is given in parentheses.
Western blot analysis of SR calcium regulatory proteins revealed that expression of the cardiac sarco-endoplasmatic reticulum calcium ATPase (SERCA2a) was significantly higher in patients with a rs13143308T risk variant, whereas expression of the SR calcium buffering protein CSQ-2 and the NCX-1 were similar in patients with and without risk variants (Figure 5G–H). Additional analysis revealed that neither PLB expression (72 ± 11% of control, P = 0.24) nor PLB phosphorylation at ser-16 (80 ± 27% of control, P = 0.54) or at thr-17 (69 ± 9% of control, P = 0.09) were different from the levels in controls without risk variants.
3.4 Combined and independent effects of the rs13143308T risk variant and AF
To determine if the rs13143308T risk variant exacerbates calcium-handling disturbances in AF, we determined whether the presence of the risk variant and/or AF had independent effects on the calcium homoeostasis using a linear regression model. Analysis of myocytes from 77 patients showed that both the rs13143308T genotype (P = 0.004) and AF (P = 0.003) independently increased the ITI frequency. Comparison of specific patient groups showed that patients with No AF carrying a risk allele had a five-fold higher ITI frequency than those with No AF and normal alleles at rs2200733 and rs13143308 (Table 2, P < 0.001). On the other hand AF increased the ITI frequency three-fold in patients with the normal allele (P = 0.002). Moreover, the effects of AF and 4q25 risk variants were additive with the ITI frequency being three-fold higher in patients with AF and a risk variant than in patients with AF but with normal variants (P = 0.008) and two-fold higher than in patients with a risk variant but without AF (P = 0.04). Analysis of the DAD frequency (Table 2) showed effects of risk variants and AF similar to those observed on the ITI frequency, i.e. the presence of a risk variant doubled the DAD frequency (P = 0.04) in patients without AF; and when the risk variant occurred in patients with AF the DAD frequency was 2.7-fold higher than in risk carriers without AF (P = 0.01). Analysis of the caffeine-induced NCX current in a subset of 53 patients revealed that the SR calcium load was significantly modified by the genotype (P = 0.001) but not by AF (P = 0.128). Comparison of patient groups revealed a significantly higher SR calcium load in patients without AF that carry the rs13143308T risk variant. There were no differences in SR calcium loading or in NCX-activity between patients with AF carrying normal or risk variants (Table 2).
Effect of rs13143308 variants on ITI and DAD frequency, SR calcium load, and NCX activity in patients without (No AF) and with AF
. | ITI . | DAD . | ||||
---|---|---|---|---|---|---|
4q25: . | Normal . | Risk . | P-value . | Normal . | Risk . | P-value . |
No AF, 95% CI (n) | 0.26 ± 0.09, 0.10–0.41 (28) | 1.33 ± 0.24, 0.91–1.75 (23) | <0.001 | 0.56 ± 0.17, 0.22–0.89 (34) | 1.22 ± 0.26, 0.71–1.72 (17) | 0.04 |
AF, 95% CI (n) | 0.84 ± 0.24, 0.65–1.62 (16) | 2.49 ± 0.61, 0.76–3.40 (10) | 0.008 | 0.95 ± 0.30, 0.36–1.54 (12) | 3.27 ± 1.24, 0.84–5.70 (4) | 0.02 |
P-value | 0.002 | 0.04 | 0.25 | 0.01 | ||
Load | NCX | |||||
4q25: | Normal | Risk | P-value | Normal | Risk | P-value |
No AF, 95% CI (n) | 7.28 ± 0.54, 6.23–8.34 (22) | 10.2 ± 1.4, 7.50–12.82 (16) | 0.03 | 0.81 ± 0.07, 0.67–0.95 (22) | 0.70 ± 0.10, 0.50–0.91 (16) | 0.37 |
AF, 95% CI (n) | 6.93 ± 0.77, 5.42–8.44 (9) | 5.2 ± 0.6, 3.95–6.44 (6) | 0.09 | 0.86 ± 0.09, 0.69–1.03 (8) | 1.00 ± 0.25, 0.51–1.50 (6) | 0.49 |
0.72 | 0.19 | 0.73 | 0.22 |
. | ITI . | DAD . | ||||
---|---|---|---|---|---|---|
4q25: . | Normal . | Risk . | P-value . | Normal . | Risk . | P-value . |
No AF, 95% CI (n) | 0.26 ± 0.09, 0.10–0.41 (28) | 1.33 ± 0.24, 0.91–1.75 (23) | <0.001 | 0.56 ± 0.17, 0.22–0.89 (34) | 1.22 ± 0.26, 0.71–1.72 (17) | 0.04 |
AF, 95% CI (n) | 0.84 ± 0.24, 0.65–1.62 (16) | 2.49 ± 0.61, 0.76–3.40 (10) | 0.008 | 0.95 ± 0.30, 0.36–1.54 (12) | 3.27 ± 1.24, 0.84–5.70 (4) | 0.02 |
P-value | 0.002 | 0.04 | 0.25 | 0.01 | ||
Load | NCX | |||||
4q25: | Normal | Risk | P-value | Normal | Risk | P-value |
No AF, 95% CI (n) | 7.28 ± 0.54, 6.23–8.34 (22) | 10.2 ± 1.4, 7.50–12.82 (16) | 0.03 | 0.81 ± 0.07, 0.67–0.95 (22) | 0.70 ± 0.10, 0.50–0.91 (16) | 0.37 |
AF, 95% CI (n) | 6.93 ± 0.77, 5.42–8.44 (9) | 5.2 ± 0.6, 3.95–6.44 (6) | 0.09 | 0.86 ± 0.09, 0.69–1.03 (8) | 1.00 ± 0.25, 0.51–1.50 (6) | 0.49 |
0.72 | 0.19 | 0.73 | 0.22 |
The ITI and DAD frequencies are given in events/min, the SR calcium load in amol/pF, and the NCX activity in s−1. Values are represented as mean ± SEM and 95% CI. P-values for comparison of the normal and risk variants are given in columns to the right of each comparison. Risk refers to the presence of at least one rs13143308T risk allele. Normal refers to patients with normal rs2200733C and rs13143308G alleles. P-values for comparison of No AF and AF are given below.
CI, confidence interval.
Effect of rs13143308 variants on ITI and DAD frequency, SR calcium load, and NCX activity in patients without (No AF) and with AF
. | ITI . | DAD . | ||||
---|---|---|---|---|---|---|
4q25: . | Normal . | Risk . | P-value . | Normal . | Risk . | P-value . |
No AF, 95% CI (n) | 0.26 ± 0.09, 0.10–0.41 (28) | 1.33 ± 0.24, 0.91–1.75 (23) | <0.001 | 0.56 ± 0.17, 0.22–0.89 (34) | 1.22 ± 0.26, 0.71–1.72 (17) | 0.04 |
AF, 95% CI (n) | 0.84 ± 0.24, 0.65–1.62 (16) | 2.49 ± 0.61, 0.76–3.40 (10) | 0.008 | 0.95 ± 0.30, 0.36–1.54 (12) | 3.27 ± 1.24, 0.84–5.70 (4) | 0.02 |
P-value | 0.002 | 0.04 | 0.25 | 0.01 | ||
Load | NCX | |||||
4q25: | Normal | Risk | P-value | Normal | Risk | P-value |
No AF, 95% CI (n) | 7.28 ± 0.54, 6.23–8.34 (22) | 10.2 ± 1.4, 7.50–12.82 (16) | 0.03 | 0.81 ± 0.07, 0.67–0.95 (22) | 0.70 ± 0.10, 0.50–0.91 (16) | 0.37 |
AF, 95% CI (n) | 6.93 ± 0.77, 5.42–8.44 (9) | 5.2 ± 0.6, 3.95–6.44 (6) | 0.09 | 0.86 ± 0.09, 0.69–1.03 (8) | 1.00 ± 0.25, 0.51–1.50 (6) | 0.49 |
0.72 | 0.19 | 0.73 | 0.22 |
. | ITI . | DAD . | ||||
---|---|---|---|---|---|---|
4q25: . | Normal . | Risk . | P-value . | Normal . | Risk . | P-value . |
No AF, 95% CI (n) | 0.26 ± 0.09, 0.10–0.41 (28) | 1.33 ± 0.24, 0.91–1.75 (23) | <0.001 | 0.56 ± 0.17, 0.22–0.89 (34) | 1.22 ± 0.26, 0.71–1.72 (17) | 0.04 |
AF, 95% CI (n) | 0.84 ± 0.24, 0.65–1.62 (16) | 2.49 ± 0.61, 0.76–3.40 (10) | 0.008 | 0.95 ± 0.30, 0.36–1.54 (12) | 3.27 ± 1.24, 0.84–5.70 (4) | 0.02 |
P-value | 0.002 | 0.04 | 0.25 | 0.01 | ||
Load | NCX | |||||
4q25: | Normal | Risk | P-value | Normal | Risk | P-value |
No AF, 95% CI (n) | 7.28 ± 0.54, 6.23–8.34 (22) | 10.2 ± 1.4, 7.50–12.82 (16) | 0.03 | 0.81 ± 0.07, 0.67–0.95 (22) | 0.70 ± 0.10, 0.50–0.91 (16) | 0.37 |
AF, 95% CI (n) | 6.93 ± 0.77, 5.42–8.44 (9) | 5.2 ± 0.6, 3.95–6.44 (6) | 0.09 | 0.86 ± 0.09, 0.69–1.03 (8) | 1.00 ± 0.25, 0.51–1.50 (6) | 0.49 |
0.72 | 0.19 | 0.73 | 0.22 |
The ITI and DAD frequencies are given in events/min, the SR calcium load in amol/pF, and the NCX activity in s−1. Values are represented as mean ± SEM and 95% CI. P-values for comparison of the normal and risk variants are given in columns to the right of each comparison. Risk refers to the presence of at least one rs13143308T risk allele. Normal refers to patients with normal rs2200733C and rs13143308G alleles. P-values for comparison of No AF and AF are given below.
CI, confidence interval.
Analysis of the L-type ICa, which is strongly reduced in patients with chronic AF, revealed that the risk variants had no effect on ICa amplitude (Figure 6A), the decay of the ICa (Figure 6B), the current-voltage relationship (Figure 6C), or the voltage for half-maximal ICa inactivation (Figure 6D) in patients with or without AF.
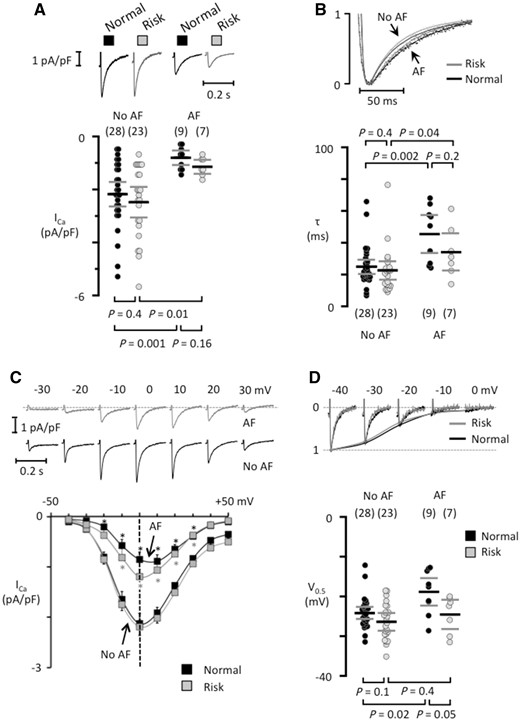
Risk variants at 4q25 do not modify the L-type calcium current in isolated human atrial myocytes. (A) ICa recordings from patients that had normal variants (black traces) or at least one risk variant (grey traces) in the absence of presence of AF. The mean ICa is shown below each trace. (B) Superimposed normalized ICa traces (top panel) and the fast time constant for ICa inactivation (bottom). (C) ICa recordings at different test potentials (top) in myocytes from patients carrying a risk variant, one of them with AF and one without AF (no AF). Current–voltage curves for each patient group (bottom). *indicates a significant difference between AF-patients and those with No AF. (D) ICa tracings recorded with different pre-potentials (top) in myocytes from patients without AF with a normal or a risk variant at 4q25. The voltage for half-maximal ICa inactivation is shown below. The number of patients is indicated for each data set in parentheses and P-values for comparisons are given above (in B) or below graphs (in A and D).
4. Discussion
4.1 Main findings
This study identifies for the first time a common SNP on chromosome 4q25 (rs13143308T) that is associated with functional changes in the calcium homoeostasis and electrical activity of human atrial myocytes, affording an electrophysiological mechanism that may explain the higher incidence of AF in carriers of this variant. Specifically, we show that human atrial myocytes from patients carrying the rs13143308T risk variant display increased SERCA2a expression, SR calcium load, and RyR2 phosphorylation, which likely cause the abnormally high incidence of both calcium release-induced ITI and spontaneous membrane depolarizations observed in these patients (see Figure 7). Since these electrophysiological alterations are recognized hallmarks of AF,4,10 the fact that we observe them even in patients without AF suggests that the rs13143308T variant is a genetic risk marker for AF associated with excessive spontaneous calcium release-induced electrical activity.
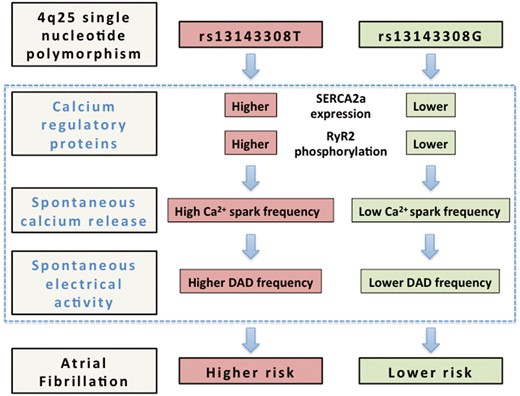
Alterations in the calcium homoeostasis that may confer a higher risk of atrial fibrillation to carriers of the 4q25 risk variant rs13143308T. Schematic outline of the mechanisms identified in the present study (blue rectangle) that may contribute to increase the risk of atrial fibrillation in carriers of the rs13143308T risk variant. Briefly, carriers of this variant present higher SERCA2a expression and RyR2 phosphorylation at ser2808 than carriers of the normal rs13143308G variant, which may account for the higher spark frequency in myocytes from patients with the risk variant. The higher incidence of spontaneous calcium release, in turn, will stimulate electrogenic Na+-Ca2+ exchange, giving rise to the higher incidence of ITI currents in carriers of the risk variant, and hence increase the frequency of DADs large enough to trigger spontaneous atrial electrical activity and induce atrial fibrillation.
4.2 Mechanisms promoting spontaneous SR calcium release in the 4q25 risk variant rs13143308T
Confocal calcium imaging in human atrial myocytes from our patients revealed that the higher incidence of spontaneous calcium waves in carriers of the 4q25 risk variant rs13143308T was due to a larger number of calcium spark sites. Since calcium sparks are produced by opening of the RyR2, a high open probability of the RyR2 has been alluded to account for an increased incidence of spontaneous calcium sparks and waves.35,36 Mechanistically, an increased RyR2 opening may result from several factors, such as luminal calcium activation,37–39 hyperphosphorylation of the RyR2,5,8–10,40 and/or increased SR calcium loading.7,41 In accordance with this, we found that carriers of the rs13143308T risk variant free of AF had higher RyR2 phosphorylation at ser2808, higher expression of SERCA2a without changes in PLB expression or phosphorylation, and higher SR calcium loading. These findings, combined with the lack of concurrent increase in the SR calcium buffering protein CSQ-2, suggest that a greater number of RyR2 could reach the threshold for spontaneous calcium release42 and consequently, lead to an increased calcium spark frequency. Interestingly the increase in SR calcium loading and SERCA2a expression was only observed in risk carriers free of AF. This suggests that the rs13143308T mediated increase in SR calcium loading may precede the occurrence of AF and might contribute to the initiation of the arrhythmia. In support of this assumption, a higher SR calcium load has been found in patients with paroxysmal AF7 but not in patients with permanent AF.4,5,7,8,10
4.3 The 4q25 risk variant rs13143308T as a genetic risk marker of AF associated with spontaneous electrical activity
Electrophysiological analysis showed that the presence of a single risk allele at rs13143308 was associated with a five-fold increase in the ITI frequency (Figure 2A and B) and in the frequency of spontaneous DADs (Figure 2D–E). These findings may provide a mechanistic understanding of observations on the efficacy of ablation therapy in carriers of rs10033464 or rs2200733 risk variants43 that are located next to the rs13143308 locus studied here and expected to co-segregate with it.34,44 Thus, Shoemaker et al.43 found that ablation therapy was less efficient in carriers of the rs2200733 risk variant, which might be expected if the ectopic activity is caused by calcium release-induced DADs that are likely to occur throughout the atrial myocardium. Interestingly, Parvez et al.27 also found that antiarrhythmic drug therapy was less efficient in patients carrying a 4q25 risk variant at the rs10033464 locus, and that carriers of the ancestral (normal) variant with AF responded better to Class III antiarrhythmic drugs while carriers of the risk variant with AF responded better to Class I antiarrhythmic drugs (sodium channel blockers). Given that carriers of the rs10033464 risk variant are expected to carry the rs13143308 risk variant also34,44 and hence have a high incidence of spontaneous calcium release-induced DADs, Class I antiarrhythmic drugs would be expected to attenuate the ability of these DADs to trigger spontaneous action potentials by increasing the threshold for sodium channel activation.
Considering that the ITI amplitude is expected to reflect electrogenic Na+-Ca2+ exchange and hence determine the amplitude of the membrane depolarization, our findings of proportionality between the ITI and DAD amplitudes in patients with and without the risk variant (Figure 2C and F) supports the notion that the DADs could be produced by calcium release-induced ITIs. On the other hand, we cannot rule out that the rs13143308 risk variant also affects other ion channels that could modify the frequency or amplitude of calcium release-induced DADs. In this context, patients with AF have previously been reported to present a reduction in potassium currents in the right atrium that normally help repolarizing the myocyte and stabilize the resting membrane potential.32,45 Thus, the present findings warrant future studies analysing the effect of 4q25 risk variants on the expression and activity of potassium channels that stabilize the resting membrane potential.
A prominent reduction in the ICa density is another characteristic feature of atrial myocytes from patients with AF.5,6,31 However, analysis of the rs13143308T and rs2200733T risk variants showed no effects on the amplitude or the properties of ICa in patients with or without AF; suggesting no role for L-type channels as a functional link between these 4q25 risk variants and AF.
4.4 Study limitations
Due to ethical constrains, our study was limited to the use of human right atrial specimens because they can be routinely obtained at the time of right atrial cannulation during the majority of surgical cardiac interventions that use extracorporeal circulatory support. In contrast, tissue samples from the left atrium are only available in patients undergoing interventions that require opening of the left atrium, such as mitral valve surgery. Even in these cases, the left atrium is often dilated and affected structurally and this has been reported to disturb the calcium homoeostasis.46 Therefore, an unbiased analysis of the calcium homoeostasis and electrical activity is feasible in human right atrial samples but much less so in left atrial samples. Thus, we cannot rule out that some of our findings are specific to the right atrium. Similarly, we cannot rule out that other proteins intervening in the calcium homoeostasis such as junctin, triadin, and sarcolipin might also be affected by the rs13143308 risk variant. Underlying cardiovascular disease or pharmacological treatments could also influence our results, but apart from a lower incidence of ischaemic heart disease and diabetes in patients with the risk variant, which is unlikely to favour spontaneous calcium release, there were no significant differences in the incidence of concurrent diseases or in the drug prescription among patients with and without 4q25 risk variants (see Table 1).
5. Clinical implications and conclusion
Our study identifies the 4q25 rs13143308T risk allele as a genetic marker for AF risk associated with excessive calcium release and spontaneous electrical activity. Clinically, this should provide novel means for (i) Identification of patients with AF or at risk of it, in whom the arrhythmia is associated with defective calcium homoeostasis and (ii) Improvement of stratification and treatment of patients with AF. Specifically, the abnormally high incidence of calcium release observed in patients with the rs13143308T variant settles the bases for testing whether current or novel pharmacological therapies targeting SR calcium release are more efficient in preventing AF in patients with this variant than in patients with normal rs13143308G alleles.
In conclusion, our results identify the rs13143308T risk variant on chromosome 4q25 as a potential biomarker for AF linked to arrhythmogenic calcium release and a new key to understand the complex molecular mechanisms that link AF to perturbations in the calcium homoeostasis.
Footnotes
Time for primary review: 34 days
Acknowledgements
The collaboration of the Department of Cardiac Surgery at Hospital de la Santa Creu i Sant Pau is greatly appreciated.
Conflict of interest: none declared.
Funding
This work was supported by multi-centric grants from Centro Nacional de Investigaciones Cardiovasculares [CNIC-2009-08 to L.H.-M. and D.F.]; a grant from Fundació Marató TV3 [2015-20-30 to L.H.-M.]; and grants from the Spanish Ministry of Economy and Competition [SAF2014-58286-C2-1-R to L.H.-M.] and [DPI2013-44584-R to R.B.]; and from the Spanish Ministry of Health and Consume, Instituto de Salud Carlos III, Red de Investigación Cardiovascular [RD12/0042/0002] and CIBERCV to J.C., and from Fondo Europeo de Desarrollo Regional (FEDER).