-
PDF
- Split View
-
Views
-
Cite
Cite
Brasilina Caroccia, Teresa Maria Seccia, Maria Piazza, Selene Prisco, Sofia Zanin, Maurizio Iacobone, Livia Lenzini, Giorgia Pallafacchina, Oliver Domening, Marko Poglitsch, Rosario Rizzuto, Gian Paolo Rossi, Aldosterone Stimulates Its Biosynthesis Via a Novel GPER-Mediated Mechanism, The Journal of Clinical Endocrinology & Metabolism, Volume 104, Issue 12, December 2019, Pages 6316–6324, https://doi.org/10.1210/jc.2019-00043
- Share Icon Share
Abstract
The G protein–coupled estrogen receptor (GPER) mediates an aldosterone secretagogue effect of 17β-estradiol in human HAC15 adrenocortical cells after estrogen receptor β blockade. Because GPER mediates mineralocorticoid receptor-independent aldosterone effects in other cell types, we hypothesized that aldosterone could modulate its own synthesis via GPER activation.
HAC15 cells were exposed to aldosterone in the presence or absence of canrenone, a mineralocorticoid receptor antagonist, and/or of the selective GPER antagonist G36. Aldosterone synthase (CYP11B2) mRNA and protein levels changes were the study end points. Similar experiments were repeated in strips obtained ex vivo from aldosterone-producing adenoma (APA) and in GPER-silenced HAC15 cells.
Aldosterone markedly increased CYP11B2 mRNA and protein expression (vs untreated samples, P < 0.001) in both models by acting via GPER, because these effects were abolished by G36 (P < 0.01) and not by canrenone. GPER-silencing (P < 0.01) abolished the aldosterone-induced increase of CYP11B2, thus proving that aldosterone acts via GPER to augment the step-limiting mitochondrial enzyme (CYP11B2) of its synthesis. Angiotensin II potentiated the GPER-mediated effect of aldosterone on CYP11B2. Coimmunoprecipitation studies provided evidence for GPER-angiotensin type-1 receptor heterodimerization.
We propose that this autocrine-paracrine mechanism could enhance aldosterone biosynthesis under conditions of immediate physiological need in which the renin-angiotensin-aldosterone system is stimulated as, for example, hypovolemia. Moreover, as APA overexpresses GPER this mechanism could contribute to the aldosterone excess that occurs in primary aldosteronism in a seemingly autonomous fashion from angiotensin II.
The mechanisms regulating aldosterone biosynthesis remain incompletely known despite several decades of investigation [for review see (1)]. For example, what causes the inappropriate oversecretion of aldosterone in primary aldosteronism (PA), the most common cause of endocrine hypertension in which the renin-angiotensin system is shut off (2), remained unknown until agonistic autoantibodies against the angiotensin type 1 receptor (3, 4), elevated serum levels of PTH (5) that stimulates aldosterone secretion (6), downregulation of TWIK-related acid-sensitive K+ channel 2 (7), and somatic and germline loss- and gain-of-function mutations in both cations and anions channels (8–13) were discovered [for review see (1, 14, 15)].
Multiple observations have also suggested a role for estrogens in the regulation of aldosterone under physiological and pathophysiological conditions (16–19). Accordingly, GPER, a G-protein coupled receptor initially described as an estrogen-specific receptor (20–22) but subsequently discovered to promiscuously bind other steroids (23) and to mediate mineralocorticoid receptor (MR)–independent aldosterone effects in different cell types (24–28), was found to be overexpressed in aldosterone-producing adenoma (APA), a main subtype of human PA (29). In human adrenocortical cells we previously observed that GPER mediates a potent secretagogue effect of 17β-estradiol (E2) on aldosterone when the estrogen receptor β (ERβ) is pharmacologically blocked or molecularly silenced (29). Based on evidence suggesting the possibility of promiscuous activation of GPER by steroids other than E2, we hypothesized that aldosterone could activate GPER and act as its own secretagogue. If proven, this mechanism could be relevant for rapidly and potently enhancing the biosynthesis of aldosterone when the renin-angiotensin-aldosterone system is stimulated. It could be also important for understanding the autonomous aldosterone production in human PA (2, 30). Hence, by acting in an autocrine-paracrine fashion in GPER-overexpressing APA (29, 31) that generate high local concentrations of the hormone, aldosterone could self-perpetuate the hyperaldosteronism despite a blunted renin-angiotensin system. This study was therefore set up to test in vitro this hypothesis using two different models that are characterized by sizable differences of aldosterone production, i.e., a human adrenocortical carcinoma cell line that produces relatively small amounts of aldosterone and strips obtained ex vivo from APA that produce substantial amounts of aldosterone.
Methods
Adrenocortical cell line HAC15
Adrenocortical cell line HAC15, a gift of William E. Rainey (University of Michigan at Ann Arbor, Michigan), was grown in DMEM/F12 supplemented with 10% cosmic calf serum (CCS), 1% glutamine, and 1% antibiotic/antimycotic mixture. For the experiments, the cells were seeded in 12-well plates at 2 × 105 cells per well and grown to subconfluence (80%). Prior to experiment, they were synchronized by incubation for 24 hours with DMEM/F12 medium supplemented with 0.5% dextran-coated charcoal-stripped CCS, 1% glutamine, and 1% antibiotic/antimycotic. Cells were treated with increasing concentrations of aldosterone (from 10−11 to 10−7 M; Sigma-Aldrich, Milan, Italy) for 12 hours. The treatment with 10−7 M aldosterone was then performed at different time points (2, 8, 12, 24, and 48 hours). To establish if aldosterone effect was mediated by MR, glucocorticoid receptor (GR), or GPER receptor, the selective MR antagonist, canrenone (10−5 M; Sigma-Aldrich), or the selective GR antagonist RU486 (10−6 M or 10−5 M; generous gift of Prof. Massimiliano Caprio), or the selective GPER antagonist G36 (32) (10−5 M; Tocris Bioscience, Bristol, UK), were added to fresh media 1 hour before aldosterone treatment.
To establish if angiotensin type-1 receptor (AT1R) and GPER activation interacted in altering CYP11B2 expression and if these two receptors formed heterodimers, HAC15 cells were treated for 12 hours with aldosterone 10−7 M on top of angiotensin II (Ang II) 10−7 M, in the absence or presence of the selective AT1R antagonist irbesartan, G36, and of both antagonists.
Tissues
APA tissues were obtained from consenting patients with a conclusive diagnosis of APA, as established by the five corner criteria, which include (i) biochemical evidence of PA; (ii) lateralized aldosterone secretion by adrenal vein sampling; (iii) detection of an adenoma by imaging (CT or magnetic resonance) and at pathology; (iv) biochemical correction of PA after adrenalectomy; and (v) detection of a CYP11B2-positive adenoma in the resected adrenal cortex at immunohistochemistry with a monoclonal antibody for human CYP11B2 (1, 2).
Briefly, tissues were obtained under sterile conditions in the operating room immediately after excision. For the functional studies, APA tissues were cut into 2- to 3-mm strips, which were transferred into 48-well plates containing 500 μL of serum-free DMEM/F12 culture medium. After six hours of starvation, the strips were treated with 10−7 and 10−8 M aldosterone alone or in presence of 10−5 M G36. All procedures were approved by the local ethics committee and informed written consent was obtained from each patient.
RNA extraction and quantitative real-time PCR
RNA from cells was extracted after treatments with the Roche RNeasy kit (Roche, Monza, Italy) and RNA from tissues with Qiagen RNA extraction kit (Qiagen, Hilden, Germany) following the manufacturer’s protocol. The quantity and quality were also determined by spectrophotometric readings at 260/280/230 nm.
One microgram total RNA from cells and from tissues was reverse transcribed in a final volume of 20 μL using the iScriptTM cDNA Synthesis kit (Bio-Rad, Milan, Italy) following the manufacturer’s recommendations. The RT-PCR reactions were performed in Delphi 1000TM Thermal Cycler (Oracle BiosystemsTM Watertown, MA).
We measured the levels of aldosterone synthase (CYP11B2) mRNA with a real-time RT-PCR by the comparative Ct (2-ΔΔCt) method: each sample was quantified vs the housekeeping gene transcript porphobilinogen deaminase (PBGD), and normalized to the control group. Each experiment was repeated at least five times in duplicate, and the results are presented as fold increase ± SD. Primers used in real-time RT-PCR are reported in an online repository (33).
Immunoblotting
Immunoblotting for CYP11B2, GPER, and AT1R was performed following a standard protocol. In brief, after treatment, cells were homogenized in lysis buffer (Thermo Scientific, Milan, Italy) and protein concentration was determined in the soluble supernatant with BCA (Thermo Scientific). Lysate fraction (50 µg) was separated in a polyacrylamide gel and then electro-blotted onto nitrocellulose or polyvinyldene fluoride membrane (Amersham-Hybond EC, GE Healthcare Life Sciences, Milan, Italy). The membranes were blocked for 30 minutes at room temperature in 5% nonfat dry blocking milk and thereafter incubated overnight at 4°C with a primary mouse monoclonal antibody anti-CYP11B2 (34) (diluted 1/500) or anti-GPER or anti-AT1R. Anti–glyceraldehyde 3-phosphate dehydrogenase antibody was used as the loading control. After washing, membranes were incubated for one hour with an anti-mouse secondary antibody. After that, the band intensity was measured in a VersaDoc Imaging System (Bio-Rad). Images were analyzed by Image Processing and Analysis in Java (Image J, NIH, Madison, Wisconsin). Bands for CYP11B2 were normalized to glyceraldehyde 3-phosphate dehydrogenase.
Small interfering RNA
The expression of GPER was silenced in HAC15 cells by RNA interference using the Nucleofector technology (Amaxa Biosystems, Thermo Scientific) following the manufacturer's protocol. To silence GPER, HAC15 were transfected with 50 nM ON-TARGET plus GPER small-interfering RNA [GPER (siRNA); Dharmacon, Carlo Erba Reagents, Milan, Italy]. An ON-TARGET plus Nontargeting Pool (Dharmacon, Carlo Erba Reagents) transfection was used as control. Cells were seeded in 6-well culture plates at the density of 1 × 106 cells per well. After 24 hours of transfection, cells were treated with aldosterone. Protein extraction was performed after 24 hours of treatment and followed by immunoblotting analysis for GPER and CYP11B2.
Cytosolic calcium ion measurements in CD56+ cells
A pure population of aldosterone-secreting cells from APA was obtained by sequential enzymatic digestion and immune-separation of CD56+ cells on magnetic beads precoated with an antibody specific for neural cell adhesion molecule tissues from three patients, as previously reported (35). At electron microscopy we showed that these CD56+ cells had a high content in mitochondria and lipid droplets, thus entailing the ultrastructural features of aldosterone-producing cell. Moreover, they also showed aldosterone production in vitro (35, 36). CD56+ cells were plated on l-polylysine precoated coverslips two days before the experiments. Cells were loaded with 2 µM Fura-2-AM (Life Technologies, Milan, Italy) diluted in Krebs-Ringer modified buffer containing 0.02% pluronic acid and 250 µM sulfinpyrazone for 20 minutes at 37°C, and then washed two times for 10 minutes with Krebs-Ringer modified buffer. Images were acquired every 2 seconds with a Nikon Ti-E microscope equipped with a Nikon 20x air objective (Nikon, Tokyo, Japan) and the software used for the acquisition was NIS Element AR (Nikon). Exposure time was set to 100 ms. Excitation was performed with a Cairn OptoScan equipped with a 150 W xenon arc lamp. Changes in Fura-2 fluorescence (340/380 nm ratio) were expressed as R/R0, where R is the ratio at time t and R0 is the ratio at the beginning of the experiment. CD56+ cells from APA tissues were treated with aldosterone, Ang II, and E2, the two latter used as control.
Aldosterone measurement in human adrenocortical tissues
We measured aldosterone levels in APA-adjacent tissue and in APA to determine how the concentration of aldosterone that increased CYP11B2 expression in our in vitro experiments compared with those occurring in vivo in these tissues. To this end, adrenal tissue samples (10 to 20 mg wet weight per sample) were ground under liquid nitrogen. The obtained frozen tissue powder was then rapidly dissolved in ice-cold guanidine hydrochloride (6 mol/L) supplemented with 1% (v/v) trifluoroacetic acid at a 100 mg tissue per milliliter concentration. Resulting homogenates were spiked with 500 pg of deuterated aldosterone for internal standardization. Following C18-based solid-phase extraction, samples were analyzed by ultra-pressure liquid chromatography tandem mass spectrometry using a reversed-phase analytical column operating in line with a Xevo TQ-S mass spectrometer (Waters, Milford, MA). The tissue concentration of aldosterone was calculated considering the corresponding response factors determined in calibration curves in tissue extract matrix, under conditions in which the integrated signals exceeded a signal-to-noise ratio of 10.
Coimmunoprecipitation studies
Interaction between AT1R and GPER was investigated in HAC15 cells using Pierce Co-Immunoprecipitation Kit (Thermo Fisher) and following the manufacturer’s protocol, as described in detail in an online repository (33). HAC15 cells harvested from four 15-cm culture dishes were centrifuged and pelleted. Cell pellet was dissolved into the solution for protein isolation. For coimmunoprecipitation studies, protein complexes were immunoprecipitated with anti-AT1R (Cusabio, Paris, France) or anti-GPER antibody (Novus Biologicals, Milan, Italy). The presence of GPER in AT1R immunoprecipitated protein was investigated with immunoblot; the presence of AT1R in GPER immunoprecipitated protein was also verified with immunoblot.
Statistical analysis
Statistical analysis was performed with GraphPad Software™ (version 8.02 for Mac OS X, La Jolla, CA). Nonparametric Mann-Whitney test or one-way ANOVA test and repeated-measures ANOVA were used, as appropriate.
Results
Aldosterone treatment enhances CYP11B2
In concentration-response and time-course experiments for up to 48 hours in HAC15 cells, we found that aldosterone enhanced CYP11B2 gene expression at 10−8 and 10−7 M and that this effect was clearly evident after 12 hours (Fig. 1A and 1B). The latter concentration and time point were therefore used to analyze the changes of CYP11B2 mRNA and protein in the further experiments in HAC15 cells.
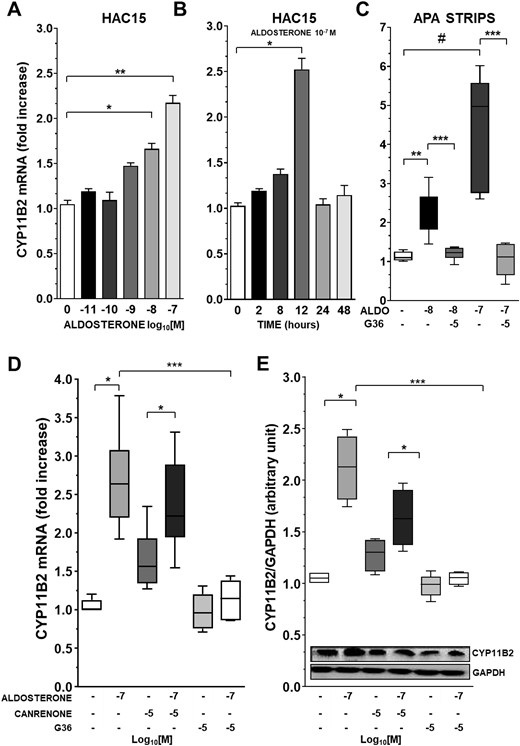
Aldosterone treatment enhances CYP11B2 via GPER activation. (A) CYP11B2 gene expression after exposure of HAC15 cells to increasing concentration of aldosterone. Data represent mean ± SD (n = 5). (B) CYP11B2 expression levels in HAC15 cell exposed for 2, 8, 12, 24, and 48 h to aldosterone 10−7 M. Data represent mean ± SD (n = 5). (C) Aldosterone-induced CYP11B2 mRNA expression in APA strips obtained from four different patients. APA strips were treated with aldosterone 10−8 M and 10−7 M alone or in addition to G36 10−6 M or 10−5 M, respectively. Data represent median and 95% CI of four different experiments performed in triplicate. (D) CYP11B2 gene expression after treatment of HAC15 cells with aldosterone 10−7 M in addition to canrenone 10−5 M or G36 10−5 M. Data represent median and 95% CI of seven different experiments performed in duplicate. (E) CYP11B2 protein expression after treatment with aldosterone 10−7 M alone or in addition to canrenone 10−5 M or G36 10−5 M for 24 h. Data represent median and 95% CI of five different experiments performed in duplicate. ALDO, aldosterone; GAPDH, glyceraldehyde 3-phosphate dehydrogenase. *P < 0.01; **P < 0.001, ***P < 0.0001; #P = 0.002.
To investigate the role of the MR in mediating CYP11B2 gene expression changes we preincubated HAC15 cells with either the MR antagonist canrenone or the GPER antagonist G36. At concentrations between 10−6 and 10−4 M canrenone alone slightly but significantly increased CYP11B2 mRNA (Fig. 1D) (33), but blunted CYP11B2 expression at 10−3 M (33).
Cell viability studies carried out in parallel in HAC15 cells showed a cytotoxic effect of canrenone at the latter concentration (33). Importantly, at 10−5 M, i.e., a concentration 100-fold higher than that of aldosterone, but not cytotoxic, canrenone did not blunt aldosterone-induced CYP11B2 mRNA increase. By contrast, one-hour pretreatment with 10−5 M G36 abolished the CYP11B2 gene expression enhanced by aldosterone (Fig. 1D).
To rule out the possibility that at 10−8 M to 10−7 M aldosterone could activate the GR (37), we treated HAC15 cells with the GR antagonist RU486 at two different concentrations (10−6 M to 10−5 M), alone or in the presence of aldosterone. RU486 neither affected by itself CYP11B2 gene expression, nor did it alter aldosterone-induced CYP11B2 gene expression (33).
The stimulatory effect of aldosterone on CYP11B2 was confirmed at protein levels: HAC15 cell exposure to aldosterone for 24 hours increased CYP11B2 protein expression, whereas G36 abolished this increase and canrenone left it unaffected (Fig. 1E).
Practically identical results were found in strips obtained ex vivo from two female and two male patients with APA on exposure to 10−7 and 10−8 M aldosterone concentrations. The hormone augmented CYP11B2 gene expression; this effect was prevented by G36 (Fig. 1C).
Importantly, in parallel experiments the changes in CYP11B2 gene expression were found to be consistently associated with aldosterone release in the medium (33).
GPER silencing blunted aldosterone-induced CYP11B2 protein expression
We used siRNA technology to confirm the finding that GPER modulates aldosterone synthase expression after aldosterone treatment. After 48 hours of transfection, GPER-silenced HAC15 cells showed a 50% reduction of GPER protein expression, as compared with control HAC15 cells transfected with nontargeting siRNA (Fig. 2A). In the GPER-silenced cells, 10−7 M aldosterone induced no increase of CYP11B2 protein expression after 24 hours, thus confirming that the aldosterone-activated CYP11B2 expression was GPER-mediated (Fig. 2B).
![GPER silencing blunted aldosterone-induced CYP11B2 protein expression. (A) GPER-1 protein levels in HAC15 cells after transfection with GPER siRNA compared with mock-transfected cells. Data represent mean ± SD of four different experiments performed in duplicate. (B) CYP11B2 protein expression after exposure of mock transfected HAC15 cells and of silenced GPER-1 cells to aldosterone 10−7 M. Data represent mean ± SD (n = 4). (C) Representative traces of cytosolic Ca2+ measurement in CD56+ cells obtained from APA performed with the Ca2+-sensitive fluorescent dye Fura-2 after treatment with 10−7 M aldosterone [A], 10−8 M E2, and 10−8 M Ang II. Experiments were performed in CD56+ cells obtained from three different APAs. (D) Tissue concentration of aldosterone in APA and in APA-adjacent tissue. Data represent median and 95% CI, n = 10. GAPDH, glyceraldehyde 3-phosphate dehydrogenase. *P = 0.01; **P < 0.01; #P < 0.001](https://oup.silverchair-cdn.com/oup/backfile/Content_public/Journal/jcem/104/12/10.1210_jc.2019-00043/1/m_jc.2019-00043f2.jpeg?Expires=1750272712&Signature=C4S-6jeBSArMu~dZ6Hq4hgsZXc2nG1KMNcZGLfqZdtzvxoXb2q~WC4LNVg5kCTX-XRNXQc8A4r1GLLBNbrhTFzqCKylVJsUHfizYuxw2JqKbpTshuJ88qsEP35BFgUNhsfk6asn9B3DwzBq79~x2haYgL66olHhKYesygMf1ST-9T-hRCeRn7q2hRmx5NiguL43DEYGuUPfvKkHLF~ZdnfGM7H4zgz7cH9PdWxvnyFQo7nvfLDjUSVoggdsbqeS1vYptlyNr2eomtXXBiHr5lk8uWVB5QcznI81tNbMnS5Nv25i36o6B7pxhPpdXwnQnYrzc1yrtKvhgrXtQwF4XBQ__&Key-Pair-Id=APKAIE5G5CRDK6RD3PGA)
GPER silencing blunted aldosterone-induced CYP11B2 protein expression. (A) GPER-1 protein levels in HAC15 cells after transfection with GPER siRNA compared with mock-transfected cells. Data represent mean ± SD of four different experiments performed in duplicate. (B) CYP11B2 protein expression after exposure of mock transfected HAC15 cells and of silenced GPER-1 cells to aldosterone 10−7 M. Data represent mean ± SD (n = 4). (C) Representative traces of cytosolic Ca2+ measurement in CD56+ cells obtained from APA performed with the Ca2+-sensitive fluorescent dye Fura-2 after treatment with 10−7 M aldosterone [A], 10−8 M E2, and 10−8 M Ang II. Experiments were performed in CD56+ cells obtained from three different APAs. (D) Tissue concentration of aldosterone in APA and in APA-adjacent tissue. Data represent median and 95% CI, n = 10. GAPDH, glyceraldehyde 3-phosphate dehydrogenase. *P = 0.01; **P < 0.01; #P < 0.001
Cytosolic Ca2+ measurements
We measured cytosolic calcium ion (Ca2+) levels after exposure to 10−7 M aldosterone to gain insight into the pathways downstream GPER activation, using the ratiometric Fura-2 Ca2+ indicator and E2 and Ang II, as controls, in CD56+ cells freshly isolated from APA. We found that, although exposure to 10−8 M Ang II caused an increase of cytosolic Ca2+ that was followed by prominent Ca2+ oscillations (Fig. 2C), cytosolic Ca2+ was not affected by E2 or by aldosterone, indicating that the GPER-mediated stimulatory effect of these steroids on CYP11B2 gene expression does not involve detectable increases of cytosolic Ca2+ (Fig. 2C).
Aldosterone concentration in human adrenocortical tissues ex vivo
To investigate how the concentrations of aldosterone that elicited a clear-cut effect on CYP11B2 mRNA levels and aldosterone synthase protein expression compared with those present in the APA tissue, we measured tissue concentrations of aldosterone by using a state-of-the-art ultra-pressure liquid chromatography tandem mass spectrometry spectrometry after C18-based solid phase extraction. We found 3.9 × 104 fmol/g (1.8 × 104 to 9.1 × 104 fmol/g, median, 95% CI) and 1.8 × 106 fmol/g (7.0 × 105 to 3.8 × 106 fmol/g, median, 95% CI) of aldosterone in the APA-adjacent tissue and in APA obtained ex vivo, respectively (Fig. 2D). By assuming 1 g of adrenal tissue to correspond approximately to 1 mL of tissue, we estimated that 1.8 × 106 fmol/g is equivalent to 1.8 × 106 M, i.e. ∼18-fold higher than the highest concentration (10−7 M) of aldosterone used in our in vitro and ex vivo experiments in HAC15 cells and APA strips.
Cross-talk between G protein–coupled receptors AT1 and GPER-1
Because functional interactions between GPCRs have been reported, we investigated whether cross-talk between AT1R and GPER could help understand the mechanism(s) by which aldosterone-induced GPER activation promoted CYP11B2 gene expression. We quantified GPER and AT1R gene and protein expression in APA and APA adjacent tissues by digital droplet PCR, a technique held to furnish absolute copies number of gene transcripts. We found that GPER and AT1R showed comparable mRNA levels; however, immunoblotting studies showed that only GPER was more abundant in APA than in APA adjacent tissue at the protein level (33). Both aldosterone and Ang II increased CYP11B2 gene expression in APA strips (Fig. 3A); when given on top of Ang II, aldosterone potentiated the secretagogue effect of the peptide. Moreover, the additive effect of aldosterone and Ang II was inhibited by either irbesartan or G36 (Fig. 3B). Similarly, in HAC15 cells aldosterone potentiated the effect of Ang II, whereas pretreatment with irbesartan and/or G36 blunted this augmentation (Fig. 3C and 3D).
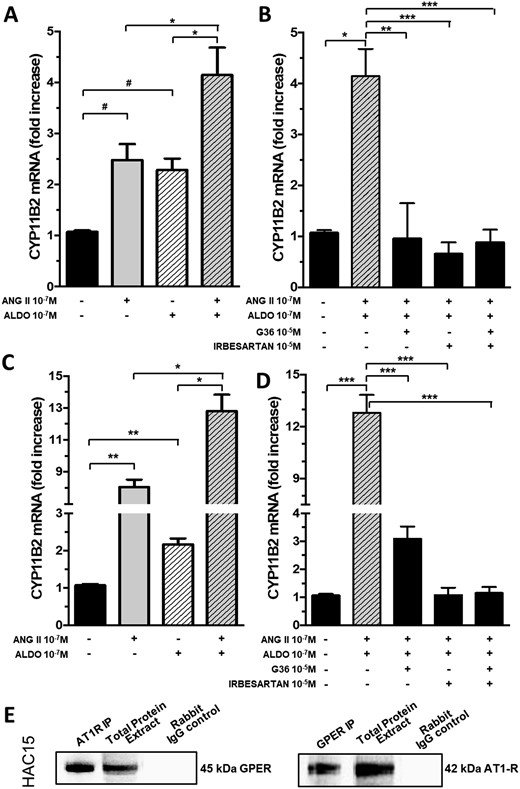
Cross-talk between G protein–coupled receptors AT1 and GPER-1. (A) Aldosterone in addition to Ang II increased the expression of CYP11B2 gene in APA strips. (B) CYP11B2 gene expression in APA strips after pretreatment with aldosterone and Ang II in addition to irbesartan, G36, or both. (C) Aldosterone in addition to Ang II increased the expression of CYP11B2 gene in HAC15 cells. (D) CYP11B2 gene expression in HAC15 cells after pretreatment with aldosterone and Ang II on top of irbesartan or G36, or both. For (A) to (D) data represent mean ± SD of four different experiments performed in duplicate. (E) Representative results of coimmunoprecipitation experiments performed in HAC15 cells; after AT1R immunoprecipitation, GPER protein expression was detected by immunoblot; the presence of heterodimers in GPER-immunoprecipitated protein was also confirmed (n = 3). Rabbit IgG control: negative control. ALDO, aldosterone. #P = 0.04; *P = 0.03; **P < 0.01; ***P < 0.001.
To investigate if the interaction between GPER and AT1R activation could be sustained by heterodimer formation, we performed coimmunoprecipitation experiments in HAC15 cells. GPER protein expression was detected by immunoblot after AT1R immunoprecipitation and vice versa, thus confirming the presence of heterodimers (Fig. 3E).
Discussion
In this study we found that aldosterone stimulated the expression of CYP11B2, the mitochondrial Ca2+- and NADH-dependent step-limiting enzyme of its biosynthesis. This effect occurred both in a female-derived adrenocortical carcinoma cell line (HAC15), and in strips obtained ex vivo at adrenalectomy from a mixed-gender cohort of primary aldosteronism patients with APA (Fig. 1). These are novel important pieces of information on the regulation of aldosterone secretion under physiological an pathophysiological conditions, as several parallel studies (33) showed that increased levels of CYP11B2 mRNA and protein are proxies of enhanced aldosterone release (29). They could also improve understanding of what happens in primary aldosteronism, the most common, albeit often overlooked, cause of endocrine hypertension (2, 30).
By using pharmacological tools to specifically block the MR or the GPER, and also by means of molecular silencing, we could identify GPER as the main mediator of CYP11B2 activation in response to aldosterone (Figs. 1 and 2). The selective MR antagonist canrenone was chosen because it is an active metabolite of spironolactone, which at variance with spironolactone, does not require cytochrome P450 activation (38). In accordance with the concept that MR antagonists are not potent drugs and require a high molar antagonist:agonist ratio to display their antagonistic effects (39), we used canrenone at concentrations 100-fold higher than those of aldosterone and found that canrenone did not blunt the effect of aldosterone.
The effect of canrenone on CYP11B2 expression was further investigated in a concentration-dependent experiment that showed a dual action: at 10−6 and up to 10−4 M canrenone stimulated CYP11B2 gene expression, whereas at 10−3 M it blunted it (33). We contend that the stimulatory effect seen at lower concentrations might involve an off-target antagonist effect on the ERβ (33), because previous studies showed that ERβ blockade unmasks the GPER-mediated stimulatory effect on CYP11B2 expression of steroids as E2 (29). Moreover, the fall of CYP11B2 seen at the highest concentrations of the MR antagonist likely occurred because of a cytotoxic effect as shown by our cell viability experiments based on the 3-(4,5-Dimethylthiazol-2-yl)-2,5-diphenyltetrazolium bromide for dye (MTT assay).
Of further note, the effect of aldosterone on CYP11B2 did not involve the GR, as it was be unaffected by the GR antagonist RU486 (33, 37). As the adrenocortical carcinoma cells HAC15 might not reflect what occurs in vivo in human PA, which is usually caused by an APA, we wished to corroborate the findings by using strips of these tumors obtained ex vivo at adrenalectomy from a mixed-gender cohort of patients presenting with florid clinical PA phenotypes. These results provided additional proof that aldosterone increased CYP11B2 gene expression (Fig. 1C) via GPER, and not the MR or GR, as its effect was abolished by G36 and unaffected by canrenone and RU486 (33).
Regarding signaling pathways, we herein found no detectable changes of cytosolic Ca2+ levels in response to aldosterone (Fig. 2C), consistent with the previous finding that E2-induced GPER-mediated expression of CYP11B2 and aldosterone secretion does not involve the protein kinase A–cAMP signaling pathway but not cytosolic Ca2+ mobilization (29).
Some limitations are, however, to be acknowledged. First, experiments with mitochondria-specific Ca2+-sensitive probes are necessary to investigate the possibility that GPER activation of CYP11B2 can involve Ca2+ influx into the mitochondria. Second, although strips from APA represent the experimental model that is closest to the in vivo conditions, caution is advised before extrapolating our findings to what occurs clinically in patients with PA. Finally, it might be argued that we have merely identified a pharmacological effect of aldosterone, because we used high concentrations of the hormone, possibly higher than those in plasma or in adrenocortical tissue of patients with PA. However, because aldosterone is locally produced in the zona glomerulosa and in APA cells and then released in the interstitial fluid, it might attain local concentrations greater than in the bloodstream. To address the lack of information on aldosterone concentrations in APA tissue, we measured aldosterone concentrations in APA by a state-of-the art tandem mass spectrometry technique. We found that the local tissue concentrations of aldosterone in APA tissue were ∼18-fold higher than those enhancing CYP11B2 expression in vitro (Fig. 2D). As we used exogenous aldosterone as a secretagogue, we could not measure aldosterone release in the medium of our cell and strip preparations because of the impossibility of discriminating between endogenously made and exogenously added aldosterone without using radiolabeled precursors, which are no longer allowed in our laboratory. However, in multiple parallel experiments with Ang II and E2 as secretagogue, we found that changes in CYP11B2 mRNA were consistently followed by aldosterone secretion (33), a finding consistent with the concept that aldosterone is not stored but constitutively secreted.
One additional point deserves discussion. Aldosterone-mediated GPER activation did not require prior ERβ blockade at variance with results with E2 (29). Because data on relative affinity of aldosterone and E2 for human adrenocortical cells is lacking, at present we could only speculate that this might be due to a higher affinity of aldosterone for GPER and/or to lower binding affinity of E2 for GPER than for ERβ (40).
A further intriguing observation in this study was the finding that aldosterone potentiated in an additive fashion the secretagogue effect of Ang II in adrenocortical cells, which suggested a functional interaction between AT1R and GPER. Likely, this resulted from functional heterodimer formation between GPER and AT1R as shown in HAC15 cells (Fig. 3), which were found to be stable at coimmunoprecipitation studies with proper controls despite no prior cross-linking (Fig. 3E).
Based on these in vitro and ex vivo studies, we propose an autocrine-paracrine mechanism whereby aldosterone, acting via GPER, can swiftly increase its own biosynthesis and release, under conditions in which the renin-angiotensin-aldosterone system needs to be rapidly activated to preserve vital organ perfusion as, for example, during acute hypovolemia and dehydration. This mechanism can also play a pathophysiological role in maintaining an inappropriately high secretion of aldosterone in patients with PA, in whom high blood pressure and volume expansion would also be expected to blunt renin and Ang II synthesis and to diminish aldosterone biosynthesis. Finally, we suggest that this autocrine-paracrine mechanism could explain the occurrence of aldosterone-producing cell clusters, which can coexist with APA, and possibly represent an early stage of the latter tumor (41).
Whether this mechanism could be implicated in idiopathic hyperaldosteronism that might be sustained by aldosterone-producing cell clusters (42), and whether this mechanism can be further amplified by Ang II remains to be investigated.
Acknowledgments
We are indebted to Dr. William Rainey for the generous gift of the HAC15 cells, to Dr. Celso Gomez-Sanchez for the generous gift of the monoclonal antibody for human CYP11B2 and for constructive criticisms, and to Prof. Massimiliano Caprio for the generous gift of the selective GR antagonist RU486.
Financial Support: This study was supported by research grants from Regione Veneto (863/01/98) and Unindustria of Treviso and F.O.R.I.C.A. (www.forica.it) (to G.P.R.); grant RF2011-02352318 from the Ministry of Health (to T.M.S.); and grants DOR1670784/16, DOR1743424/17, DOR1842303/18, and BIRD163255/16 from the University of Padova (to T.M.S. and G.P.R.).
Disclosure Summary: The authors have nothing to disclose.
Data Availability: All data generated or analyzed during this study are included in this published article or in the data repositories listed in References.
Abbreviations:
- Ang II
angiotensin II
- APA
aldosterone-producing adenoma
- AT1R
angiotensin type-1 receptor
- Ca2+
calcium ion
- CCS
cosmic calf serum
- E2
17β-estradiol
- ERβ
estrogen receptor β
- GPER
G protein–coupled estrogen receptor
- GR
glucocorticoid receptor
- MR
mineralocorticoid receptor
- PA
primary aldosteronism
- siRNA
small-interfering RNA