-
PDF
- Split View
-
Views
-
Cite
Cite
Katarina Niward, Kristian Ängeby, Erja Chryssanthou, Jakob Paues, Judith Bruchfeld, Pontus Jureen, Christian G. Giske, Gunnar Kahlmeter, Thomas Schön, Susceptibility testing breakpoints for Mycobacterium tuberculosis categorize isolates with resistance mutations in gyrA as susceptible to fluoroquinolones: implications for MDR-TB treatment and the definition of XDR-TB, Journal of Antimicrobial Chemotherapy, Volume 71, Issue 2, February 2016, Pages 333–338, https://doi.org/10.1093/jac/dkv353
- Share Icon Share
Abstract
Fluoroquinolones (FQs) are important in the treatment of MDR-TB and in the definition of XDR-TB. Our objective was to investigate how discrepancies in the phenotypic and genotypic methods for antimicrobial susceptibility testing could affect the interpretation of antimicrobial susceptibility test results.
We analysed MICs of ofloxacin and levofloxacin in Middlebrook 7H10 broth (7H10) as well as sequencing of the quinolone resistance-determining region of the gyrA gene and the MTBDRsl assay in 75 resistant isolates, including MDR and XDR strains of Mycobacterium tuberculosis.
Among 75 resistant isolates, 27 had mutations associated with FQ resistance. Among isolates with resistance mutations in gyrA, 26% (seven of 27) were susceptible to levofloxacin and ofloxacin by phenotypic testing at 1 mg/L and 2 mg/L. The most common mutation was in codon 94 and these isolates had significantly increased MICs of levofloxacin (2–8 mg/L) compared with isolates with mutations in codon 90 (0.25–2 mg/L, P < 0.05). The sensitivity and specificity for the MTBDRsl assay compared with gyrA sequencing were 96% and 98%, respectively.
Current critical concentrations may classify up to 26% of isolates with gyrA mutations as susceptible to FQs due to a close relationship between susceptible and resistant populations. These results should be considered while improving clinical breakpoints for M. tuberculosis and may have an impact on the definition of XDR-TB.
Introduction
MDR- and XDR-TB pose extreme challenges for clinicians and healthcare systems worldwide.1 MDR is defined as resistance to isoniazid and rifampicin, and for such strains of Mycobacterium tuberculosis, fluoroquinolones (FQs) in combination with injectable drugs such as aminoglycosides, constitute the backbone in the therapeutic regimen.1 XDR is defined as MDR plus resistance to any FQs and to at least one of the three second-line injectables (amikacin, capreomycin and kanamycin), and is even more difficult to treat. Among the FQs, the newer levofloxacin and moxifloxacin are preferred over ofloxacin.1 FQs are considered bactericidal against M. tuberculosis, and resistance to FQs is mostly associated to mutations in the gyrA gene (64%; 786 of 1220 isolates from 42 studies) and to a lesser extent in the gyrB gene (5.6%; 30 of 534 isolates from 14 studies) as outlined in a recent systematic review.2,3 The most frequent gyrA mutations in codons 90 and 94 can rapidly be detected by the MTBDRsl kit,2,4 a commercially available line probe assay. The sensitivity and specificity for detecting FQ resistance by this assay using conventional antimicrobial susceptibility testing (AST) as a gold standard has been reported in the range 57%–97% and 77%–100%.1,3,4 However, the results of studies correlating genotypic and phenotypic resistance largely depend on the geographical distribution of resistance mutations, as well as the media and breakpoints for susceptibility testing used as gold standard.1,3,5,6
Currently, critical concentrations (from here on labelled as breakpoints) for M. tuberculosis are suggested by the WHO and have not been evaluated by the strategy used for other bacterial pathogens and fungi.1 We have previously suggested that clinical drug susceptibility testing breakpoints for M. tuberculosis should be revised using the approach used by EUCAST.6,7 Along with that strategy, WT distribution of the MICs determines the epidemiological cut-off (ECOFF), which is the highest MIC in the WT distribution. Strains within the WT MIC distribution are by definition devoid of phenotypically detectable mutational resistance mechanisms whereas strains with MIC above the ECOFF are likely to have such mechanisms. The ECOFF may or may not be identical to the clinical breakpoint but, by default, should not be considered for categorization into clinical susceptibility or resistance. The ECOFF must be combined with the other essential cornerstones for determinations of clinical breakpoints such as pharmacokinetic (PK) and pharmacodynamic (PD) data as well as clinical outcome studies to define clinical breakpoints according to the susceptible/intermediate/resistant system. According to this classification, susceptibility is defined as a high likelihood of therapeutic success, intermediate as uncertain clinical effect and finally resistance is defined as a high likelihood of clinical failure.8
As an example of the importance of considering the WT distribution while defining breakpoints, we have shown that the poor reproducibility and accuracy of AST against ethambutol is a result of a poorly defined breakpoint where the WT and resistant strains overlap.5 We have also shown that the WHO recommended breakpoints of 2 mg/L for ofloxacin and up until recently 2 mg/L for levofloxacin (now reduced to 1 mg/L) on Middlebrook 7H10 medium and the liquid MGIT 960 system are probably too high in relation to the ECOFF.1,6,9 Additionally, there are very limited PK/PD and clinical outcome data from prospective studies in support of the current FQ breakpoints.
Our former study,6 which was primarily designed to define the previously unknown WT MIC distribution of FQs for M. tuberculosis, indicated a close relationship between the susceptible and resistant populations. Thus, the aim of the present study was to investigate the current breakpoints to ofloxacin and levofloxacin by comparing phenotypic drug susceptibility testing including MICs in a population consisting mainly of MDR and XDR strains in relation to mutational resistance in gyrA.
Methods
Strains
In total, 75 M. tuberculosis strains from the collection at the Swedish Public Health Institute (FoHM) were included in the study. In Sweden >75% of patients with TB are from sub-Saharan Africa or Asia.5 Thirty-four strains were resistant clinical isolates including MDR and pre-XDR isolates (MDR-TB isolates resistant to either FQs or aminoglycosides) from FoHM isolated in Sweden 2003–10 and were all unique by RFLP performed according to national routine. These 34 clinical strains represented all four major lineages and the largest group consisted of the Beijing strains of the East Asian lineage (15 of 34). The remaining isolates were from external panels including the WHO external quality assessment (EQA) panels for second-line drugs during 2007–09. Among the 75 strains, 21 were defined as MDR without resistance to FQs, 20 were pre-XDR strains where all but two isolates were MDR plus aminoglycoside resistant, 14 were XDR strains, 16 were resistant, non-MDR/XDR isolates and 4 were susceptible isolates. M. tuberculosis H37Rv was used as a control strain.
MIC determinations
The methodology has been described in detail previously.5,6 Briefly, stock solutions (30.7 g/L) were prepared for ofloxacin (Sigma) in 0.2 M NaOH (Merck) and for levofloxacin (Fluka) in DMSO (Sigma). Bacterial suspensions of all strains diluted to the turbidity of 0.5 McFarland were transferred using a 96-stick replicator to Middlebrook 7H10 agar plates with serial 2-fold dilutions from 0.002 to 512 mg/L for all drugs. Drug-free control plates with undiluted and 1:100 diluted bacterial suspensions were replicated in the same way and the plates were incubated at 37°C for 3 weeks. The MIC was defined as the first antibiotic concentration where there was less growth compared with the 1:100 diluted controls of the corresponding strain, i.e. the lowest concentration of drug that inhibited >99% of the bacterial population. M. tuberculosis H37Rv was included in duplicate as a quality control strain.
Detection of mutations in gyrA
DNA was extracted from the M. tuberculosis strains as previously described.10 A 602 nt fragment containing the quinolone resistance-determining region (QRDR) of gyrA was sequenced. Applying the primers gyrA F180, 5′-CCT CGG TTC GTC TGT TGC GTC AAG T-3′ and gyrA R422, 5′-ATC TCC ATC GCC AAC GGG GTC A-3′, PCR and sequencing of the fragment were carried out using a previously described method.11 With the ClustalW algorithm, the sequences were aligned to WT H37Rv's gyrA gene (Rv0006). The nucleotides and codons were designated based on Escherichia coli codon numbering. Additionally, isolates were tested using the GenoType® MTBDRsl kit (Hain Lifescience, GmbH, Nehren, Germany) as previously described.4
Statistical analysis
Comparison of MIC data was performed by the Mann–Whitney U-test. P < 0.05 was regarded as statistically significant.
Results
Quality control
The MIC for the M. tuberculosis H37Rv strain was tested in duplicate with results within ± one MIC dilution step (0.25 and 0.5 mg/L for ofloxacin; 0.125 and 0.25 mg/L for levofloxacin). For the 20 EQA strains, consensus results were available for 16 (5 isolates with FQ resistance and 11 without FQ resistance) where the 7H10 method showed full categorical agreement to the consensus results. All resistant EQA panel isolates had ofloxacin MICs ≥8 mg/L.
MIC distribution of ofloxacin and levofloxacin for the clinical isolates
The MIC distribution and the presence of gyrA mutations as well as the MTBDRsl results of the 75 tested isolates are displayed in Table 1 and Figures 1 and 2. Forty-five strains had no resistance mutations in the QRDR of gyrA and MIC distributions between 0.25 and 0.5 mg/L for ofloxacin and 0.125 and 0.25 mg/L for levofloxacin. One of these 45 isolates had a Thr80Ala substitution, which was not regarded to confer phenotypical resistance, as this substitution has previously been associated with FQ hypersensitivity rather than resistance.12 The MIC for this isolate was in the WT range for ofloxacin and levofloxacin (0.5 mg/L and 0.25 mg/L, respectively (Table 1). Three additional strains, which were WT for the QRDR region of gyrA, had increased MICs at or above the breakpoint for ofloxacin and levofloxacin (Figures 1 and 2). The remaining 27 isolates had resistance mutations in the gyrA gene and MICs of ofloxacin in the range of 1–32 mg/L and of levofloxacin in the range of 0.25–16 mg/L.
Number of strains . | MIC (mg/L) . | Amino acid substitutions in gyrA (sequencing) . | WT band missing (MTBDRsl) . | Resistance mutation (MTBDRsl) . | |
---|---|---|---|---|---|
Ofloxacin . | Levofloxacin . | ||||
19 | 0.25 | 0.125 | no | no | no |
6 | 0.25 | 0.25 | no | no | no |
1 | 0.25 | 1 | no | no | no |
4 | 0.5 | 0.125 | no | no | no |
14 | 0.5 | 0.25 | no | no | no |
1 | 0.5 | 0.25 | Thr80Ala | no | no |
1 | 1 | 0.25 | Ala90Val | WT 2 | mut 1 |
1 | 1 | 0.5 | Ala74Ser | no | mut 3c |
4 | 2 | 1 | Ala90Val | WT 2 | mut 1 |
1 | 2 | 1 | no | no | no |
1 | 2 | 2 | Ala90Val | WT 2 | mut 1 |
1 | 4 | 1 | Ala90Val | WT 2 | mut 1 |
1 | 4 | 1 | no | no | no |
1 | 4 | 2 | Asp94Gly | no | mut 3c |
1 | 4 | 2 | Asp89Asn | WT 1 | no |
2 | 4 | 2 | Ala90Val | WT 2 | mut 1 |
1 | 4 | 2 | Asp94Tyr | no | no |
1 | 4 | 2 | Asp94Asn | no | mut 3b |
5 | 8 | 4 | Asp94Gly | WT 3 | mut 3c |
1 | 8 | 4 | Asp94Tyr | no | mut 3b |
1 | 8 | 4 | Asp94Asn | WT 3 | mut 3b |
1 | 8 | 4 | Asp94Ala | WT 3 | mut 3a |
1 | 8 | 4 | Asp94Gly | no | mut 3c |
2 | 8 | 4 | Ser91Pro Asp94Tyr | no | mut 3b |
1 | 8 | 8 | no | no | no |
1 | 16 | 8 | Asp94Gly | WT 3 | mut 3c |
1 | 32 | 16 | Ala90Val Asp94Asn | WT 2, 3 | mut 1, 3b |
Number of strains . | MIC (mg/L) . | Amino acid substitutions in gyrA (sequencing) . | WT band missing (MTBDRsl) . | Resistance mutation (MTBDRsl) . | |
---|---|---|---|---|---|
Ofloxacin . | Levofloxacin . | ||||
19 | 0.25 | 0.125 | no | no | no |
6 | 0.25 | 0.25 | no | no | no |
1 | 0.25 | 1 | no | no | no |
4 | 0.5 | 0.125 | no | no | no |
14 | 0.5 | 0.25 | no | no | no |
1 | 0.5 | 0.25 | Thr80Ala | no | no |
1 | 1 | 0.25 | Ala90Val | WT 2 | mut 1 |
1 | 1 | 0.5 | Ala74Ser | no | mut 3c |
4 | 2 | 1 | Ala90Val | WT 2 | mut 1 |
1 | 2 | 1 | no | no | no |
1 | 2 | 2 | Ala90Val | WT 2 | mut 1 |
1 | 4 | 1 | Ala90Val | WT 2 | mut 1 |
1 | 4 | 1 | no | no | no |
1 | 4 | 2 | Asp94Gly | no | mut 3c |
1 | 4 | 2 | Asp89Asn | WT 1 | no |
2 | 4 | 2 | Ala90Val | WT 2 | mut 1 |
1 | 4 | 2 | Asp94Tyr | no | no |
1 | 4 | 2 | Asp94Asn | no | mut 3b |
5 | 8 | 4 | Asp94Gly | WT 3 | mut 3c |
1 | 8 | 4 | Asp94Tyr | no | mut 3b |
1 | 8 | 4 | Asp94Asn | WT 3 | mut 3b |
1 | 8 | 4 | Asp94Ala | WT 3 | mut 3a |
1 | 8 | 4 | Asp94Gly | no | mut 3c |
2 | 8 | 4 | Ser91Pro Asp94Tyr | no | mut 3b |
1 | 8 | 8 | no | no | no |
1 | 16 | 8 | Asp94Gly | WT 3 | mut 3c |
1 | 32 | 16 | Ala90Val Asp94Asn | WT 2, 3 | mut 1, 3b |
mut, mutation.
Number of strains . | MIC (mg/L) . | Amino acid substitutions in gyrA (sequencing) . | WT band missing (MTBDRsl) . | Resistance mutation (MTBDRsl) . | |
---|---|---|---|---|---|
Ofloxacin . | Levofloxacin . | ||||
19 | 0.25 | 0.125 | no | no | no |
6 | 0.25 | 0.25 | no | no | no |
1 | 0.25 | 1 | no | no | no |
4 | 0.5 | 0.125 | no | no | no |
14 | 0.5 | 0.25 | no | no | no |
1 | 0.5 | 0.25 | Thr80Ala | no | no |
1 | 1 | 0.25 | Ala90Val | WT 2 | mut 1 |
1 | 1 | 0.5 | Ala74Ser | no | mut 3c |
4 | 2 | 1 | Ala90Val | WT 2 | mut 1 |
1 | 2 | 1 | no | no | no |
1 | 2 | 2 | Ala90Val | WT 2 | mut 1 |
1 | 4 | 1 | Ala90Val | WT 2 | mut 1 |
1 | 4 | 1 | no | no | no |
1 | 4 | 2 | Asp94Gly | no | mut 3c |
1 | 4 | 2 | Asp89Asn | WT 1 | no |
2 | 4 | 2 | Ala90Val | WT 2 | mut 1 |
1 | 4 | 2 | Asp94Tyr | no | no |
1 | 4 | 2 | Asp94Asn | no | mut 3b |
5 | 8 | 4 | Asp94Gly | WT 3 | mut 3c |
1 | 8 | 4 | Asp94Tyr | no | mut 3b |
1 | 8 | 4 | Asp94Asn | WT 3 | mut 3b |
1 | 8 | 4 | Asp94Ala | WT 3 | mut 3a |
1 | 8 | 4 | Asp94Gly | no | mut 3c |
2 | 8 | 4 | Ser91Pro Asp94Tyr | no | mut 3b |
1 | 8 | 8 | no | no | no |
1 | 16 | 8 | Asp94Gly | WT 3 | mut 3c |
1 | 32 | 16 | Ala90Val Asp94Asn | WT 2, 3 | mut 1, 3b |
Number of strains . | MIC (mg/L) . | Amino acid substitutions in gyrA (sequencing) . | WT band missing (MTBDRsl) . | Resistance mutation (MTBDRsl) . | |
---|---|---|---|---|---|
Ofloxacin . | Levofloxacin . | ||||
19 | 0.25 | 0.125 | no | no | no |
6 | 0.25 | 0.25 | no | no | no |
1 | 0.25 | 1 | no | no | no |
4 | 0.5 | 0.125 | no | no | no |
14 | 0.5 | 0.25 | no | no | no |
1 | 0.5 | 0.25 | Thr80Ala | no | no |
1 | 1 | 0.25 | Ala90Val | WT 2 | mut 1 |
1 | 1 | 0.5 | Ala74Ser | no | mut 3c |
4 | 2 | 1 | Ala90Val | WT 2 | mut 1 |
1 | 2 | 1 | no | no | no |
1 | 2 | 2 | Ala90Val | WT 2 | mut 1 |
1 | 4 | 1 | Ala90Val | WT 2 | mut 1 |
1 | 4 | 1 | no | no | no |
1 | 4 | 2 | Asp94Gly | no | mut 3c |
1 | 4 | 2 | Asp89Asn | WT 1 | no |
2 | 4 | 2 | Ala90Val | WT 2 | mut 1 |
1 | 4 | 2 | Asp94Tyr | no | no |
1 | 4 | 2 | Asp94Asn | no | mut 3b |
5 | 8 | 4 | Asp94Gly | WT 3 | mut 3c |
1 | 8 | 4 | Asp94Tyr | no | mut 3b |
1 | 8 | 4 | Asp94Asn | WT 3 | mut 3b |
1 | 8 | 4 | Asp94Ala | WT 3 | mut 3a |
1 | 8 | 4 | Asp94Gly | no | mut 3c |
2 | 8 | 4 | Ser91Pro Asp94Tyr | no | mut 3b |
1 | 8 | 8 | no | no | no |
1 | 16 | 8 | Asp94Gly | WT 3 | mut 3c |
1 | 32 | 16 | Ala90Val Asp94Asn | WT 2, 3 | mut 1, 3b |
mut, mutation.
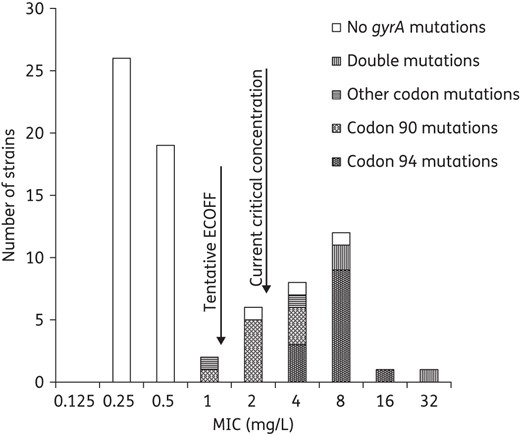
MIC distribution of ofloxacin in relation to mutations in gyrA. Seven isolates with mutations in gyrA were below the current critical concentration (breakpoint) at 2 mg/L. The suggested range for an intermediate group is 1–2 mg/L.
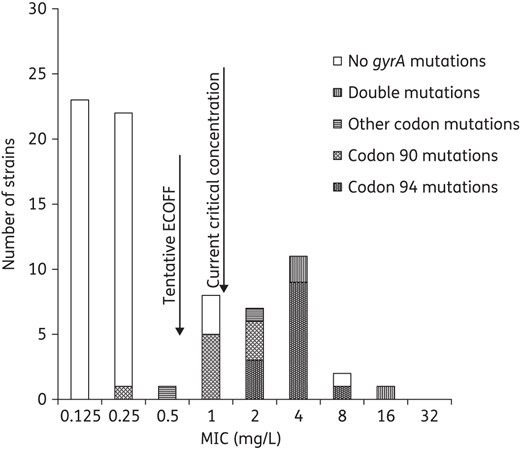
MIC distribution of levofloxacin in relation to mutations in gyrA. Seven isolates with mutations in gyrA were below the current critical concentration (breakpoint) at 1 mg/L. The suggested range for an intermediate group is 0.5–1 mg/L.
Poor correlation between the current breakpoints for ofloxacin and levofloxacin and mutations in gyrA
Twenty-seven strains had mutations in the QRDR of gyrA associated with FQ resistance resulting in amino acid substitutions, predominantly in codon 90 (n = 10; all Ala90Val) and codon 94 (n = 16; out of 8 in Asp94Gly, Table 1). Among isolates with resistance mutations in gyrA, 26% (7 of 27) were susceptible against ofloxacin and 52% against levofloxacin by phenotypic testing at 2 mg/L according to WHO-based breakpoints from 1998 (2 mg/L).13 The breakpoint for levofloxacin was revised by the WHO in 2014 from 2 mg/L to 1 mg/L.1 Applying 1 mg/L as a breakpoint for levofloxacin, 26% (7 of 27) of the isolates with resistance mutations in gyrA were susceptible. Six of the seven isolates that had resistance mutations in gyrA and were susceptible by phenotypic AST showed a mutation in codon 90 of gyrA. The MICs for strains with mutations in codon 94 formed a normal distribution at a significantly higher level (ofloxacin 4–16 mg/L and levofloxacin 2–8 mg/L), than the MICs for strains with mutations in codon 90 (ofloxacin 1–4 mg/L and levofloxacin 0.25–2 mg/L, P < 0.05, Figures 1 and 2). For strains with mutations in codon 90, the ofloxacin MIC distribution was significantly increased compared with the MICs for the strains without any mutations in the gyrA (median MIC 2 mg/L versus median MIC 0.25 mg/L, P < 0.05, Figures 1 and 2). One strain with a gyrA mutation in codon 90 had levofloxacin MIC 0.25 mg/L and ofloxacin MIC 1.0 mg/L. Only two strains had single mutations in other codons than 90 and 94 of the QRDR in gyrA. These mutations resulted in the amino acid substitution Asp89Asn and this isolate was phenotypically resistant to levofloxacin and ofloxacin as previously described.2 Concerning the Ala74Ser substitution, this isolate was fully susceptible with low MIC levels against ofloxacin and levofloxacin (Table 1), which is also in line with previous data.14,15
Single mutations detected in the MTBDRsl assay correlated to higher MIC ranges for mut 3a-c (codon 94; levofloxacin 2–8 mg/L and ofloxacin 4–16 mg/L, n = 12) compared with mut 1 (codon 90; levofloxacin 0.25–2 mg/L and ofloxacin 1–4 mg/L, n = 9). When comparing the results from gyrA sequencing to the results from the MTBDRsl assay, there were two of 27 discrepant results where the MTBDRsl assay in one case did not detect the amino acid substitution Asp94Tyr (mut 3b) and where Asp94Gly (mut 3c) was detected by the MTBDRsl assay but could not be confirmed by the gyrA sequencing. In the former case, the strain was clearly FQ resistant with ofloxacin MIC at 4.0 mg/L and levofloxacin MIC at 2.0 mg/L, whereas in the latter case, the MICs of FQs were closer to the WT distribution (ofloxacin MIC 1.0 mg/L and levofloxacin MIC 0.5 mg/L). The MTBDRsl sensitivity and specificity compared with gyrA sequencing regarding detection of resistance mutations was 96% and 98%, respectively.
Discussion
The present study focused on AST against FQs for M. tuberculosis using both phenotypical and genotypical methods. Our main finding is that levofloxacin- and ofloxacin-resistant strains with specific mutations in the QRDR of gyrA have MIC levels in close relationship to WT organisms. Accordingly, the current breakpoints for FQs may classify up to 25% of isolates with resistance mutations in gyrA as susceptible.
Similar to Sirgel et al.,16 we found no gyrA mutations in strains with an MIC ≤0.5 mg/L for ofloxacin or 0.125 mg/L for levofloxacin corresponding to the WT distribution. We also observed that some mutations in gyrA resulting in amino acid substitutions, most notably Ala90Val, were associated with ofloxacin and levofloxacin MICs close to MICs in the group of strains lacking resistance mechanisms. This is similar to a previous study showing MIC levels of ofloxacin for Ala90Val at 2–6 mg/L.16 Considering the methodological variation of 1 ± 2-fold dilution step for MIC determination, it is likely that if a breakpoint is set near the highest MIC within the WT MIC distribution (i.e. the ECOFF), some strains with resistance mechanisms would have susceptibility test results that vary between S and R upon repeated testing. It should have been important to include such isolates with borderline resistant MICs in the EQA testing panels, but at least in the EQA isolates we investigated, rather highly resistant M. tuberculosis with ofloxacin MICs ≥8 mg/L were selected, which may lead to a false impression of a good agreement for AST to these drugs.
In the rapid MTBDRsl assay, different MIC rages are linked to the mutations detected in this assay such as lower ranges for levofloxacin for mut 1 (0.25–2 mg/L) but higher levels for mut 3a-c (2–8 mg/L), which could have implications for dosing of the FQs long before a more accurate MIC testing could be performed.
We have recently gathered MIC distribution data for ofloxacin and levofloxacin on the EUCAST web page (http://mic.eucast.org/Eucast2/), which mostly included MIC values from resistant isolates, but these data from 1240 individual MIC determinations from different geographical origins and 12 sources show a distribution of susceptible isolates below the currently suggested ECOFF. These results also show a close relationship between the MIC distributions for WT strains when compared with the MIC distribution for resistant strains.6 Introducing an intermediary category for FQs (0.5–1 mg/L as I for levofloxacin and 1–2 mg/L for ofloxacin; Figures 1 and 2) as we have recommended for other anti-tuberculous drugs, and which is used in AST for other bacterial pathogens, could be important to indicate for the clinicians that the clinical efficacy of FQ on isolates with resistance mutations in gyrA is uncertain and that a higher dose and applying therapeutic drug monitoring may be warranted to ensure the clinical efficacy of the drug.
In TB treatment, there is a clear correlation between strains below ECOFF for isoniazid and rifampicin, which also corresponds well to the current breakpoints, and a favourable clinical outcome, which could be exemplified by the markedly increased clinical failure of rifampicin and isoniazid in MDR-TB (i.e. strains with an MIC level above the breakpoint and the ECOFF for isoniazid and rifampicin).1 In the case of isoniazid, patients with isolates showing low-level resistance (typically below 1 mg/L) may be treated with high-dose isoniazid despite mutational resistance in inhA, although this is based on limited prospective clinical data.17 Similarly, FQs may be effective for the treatment of patients with XDR-TB with isolates showing low-grade FQ resistance, but this may be dose dependent and remains to be shown in prospective clinical trials. The results of recent meta-analyses showing maintained efficacy of FQs in the treatment of XDR-TB despite FQ resistance would partly support such strategies.1,18,19 However, as shown by the present study, for FQs, the breakpoints used in such retrospective studies are more problematic than for isoniazid as the separation in the MIC distribution between resistant and susceptible isolates is not as clear and may compromise the classification of FQ resistance and by that also the definition of XDR-TB. Additionally, the breakpoints and FQs used in treatment have changed over time. Consequently, an increase of the current breakpoint for moxifloxacin to 2 mg/L as has been suggested in the latest WHO guidelines16 would classify the majority of strains with resistance mutations in gyrA as moxifloxacin susceptible, but resistant against other FQs such as levofloxacin. To our knowledge, there are neither any prospective clinical trials to support such breakpoints for moxifloxacin nor any clinical studies to confirm that levofloxacin is not as effective as moxifloxacin. The MIC of moxifloxacin is lower than the MIC of the other FQs for M. tuberculosis but this does not automatically indicate an increased efficacy of the drug, as the free serum drug concentrations achieved in patients with TB are lower for moxifloxacin than for the other FQs.20 Although there is no established PK/PD target for FQs in the treatment of TB, free AUC (fAUC)/MIC ≥100 has been suggested, similar to Gram-negative bacilli.21 Thus, at normal doses of levofloxacin, most patients could reach a target at fAUC/MIC 100 up to MIC 1–2 mg/L corresponding to 0.25–0.5 for moxifloxacin.20 This may also cover some of the low-level resistant strains for levofloxacin with resistance mutations in position 90, although the target in the individual patient would depend on the drug concentration achieved versus the actual MIC for the M. tuberculosis strain. The importance of FQs in MDR-TB treatment has been clearly shown18 and therapeutical drug monitoring using both drug concentrations and MIC to adjust the dosing is probably of high clinical relevance in the individual patient.22
Our study has limitations and it would have been important to include even more isolates with FQ resistance. Sequencing of gyrB was not performed and may have addressed potential discrepancies between the current breakpoint and mutations in gyrB, which are much more infrequent than gyrA. Additionally, the inclusion of moxifloxacin would have allowed for analysis of cross-resistance among FQs.
In summary, a mismatch between mutational and phenotypical resistance in the current breakpoint for ofloxacin and levofloxacin where up to 25% of isolates with a resistance mutation in gyrA are classified as susceptible is likely to affect the classification of XDR and the evaluation of FQs in clinical interventional studies. Along with increasing resistance, a re-evaluation of the clinical breakpoints for FQs defined according to the generally accepted criteria for other bacteria is warranted. To avoid misinterpretation, we suggest that isolates phenotypically susceptible to FQs according to current critical concentrations, but with resistance mutations, should not be classified as susceptible, but as intermediate. It may be possible to treat patients with TB with FQs even in the presence of increased MIC levels and resistance mutations such as mutations in codon 90 of the gyrA gene with high-dose FQs during combination therapy; however, clinical evidence from prospective studies is currently lacking.
Funding
The work was supported by the Heart and Lung Foundation, Swedish Society of Antimicrobial Chemotherapy (SSAC), Swedish Medical Association and Marianne and Marcus Wallenberg Foundation.
Transparency declarations
C. G. G. has received speaker's honoraria from AstraZeneca, Cubist, Pfizer and Meda. J. B. has received speaker's honorarium from Pfizer, Jansen and Otsuka. All other authors: none to declare.