-
PDF
- Split View
-
Views
-
Cite
Cite
Yang Liu, Xiaohui Chen, Mingyuan Li, Cunhong Wei, Mingchun Xiao, Jingjing Xiao, Yaqi Zhang, Xuedong Chang, Jing Zou, Dynamics and correlation between volatile compounds and microbial community in chestnut rice wine fermentation, International Journal of Food Science and Technology, Volume 59, Issue 7, July 2024, Pages 5155–5168, https://doi.org/10.1111/ijfs.17257
- Share Icon Share
Abstract
Chestnut rice wine, also called banli mijiu (BLMJ), uses Chinese chestnut (Castanea mollissima Blume) in the fermentation of traditional glutinous rice wine. This study characterised the microbial communities and volatile compounds in BLMJ and explored their correlations. Headspace solid-phase microextraction-gas chromatography–mass spectrometry (HS-SPME-GC–MS) revealed that the major volatile compounds during BLMJ fermentation include eighteen important volatile compounds (OAV > 1). High-throughput sequencing (HTS) demonstrated that the abundance and diversity of bacteria were significantly greater than those of fungi during the fermentation process. Among them, Kropensteria and Saccharomyces were the most abundant bacteria and fungi at the genus level during the fermentation of BLMJ. Spearman's correlation analysis revealed that eighteen important volatile compounds were significantly positively correlated with seven microbial genera (|r| > 0.9, P < 0.01), including six bacterial genera (Rhodococcus, Lactococcus, Pseudomonas, Janthinobacterium, Unclassified_f_Bacillaceae and Burkholderia-Caballeronia-Paraburkholderia) and one fungal genus (Saccharomyces). Therefore, these bacterias and fungi may be core functional microorganisms during BLMJ fermentation. The findings may provide important reference for mediating the microbial community during fermentation to improve the flavour of BLMJ.
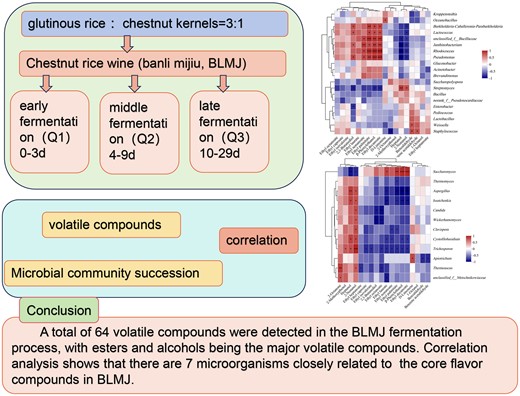
Introduction
Rice wine is a traditional alcoholic beverage in China with a history of over a thousand years and is one of the most popular alcoholic beverages in the world. Rice wine has a simple production process, a sweet and fragrant taste and a low alcohol content. Rice wine, along with beer and wine, is known as one of the three ancient fermented wines in the world (Mao et al., 2023). It is rich in amino acids, peptides, vitamins and oligosaccharides and has certain health benefits. It is widely popular in China. China is the country with the highest export volume of yellow wine in the world. From January to October 2022, the export volume of Chinese yellow wine was 12 000 tons (https://www.chyxx.com/industry/1153741.html).
Volatile compounds are an essential class of characteristic substances reflecting the quality of rice wine and are mainly determined by the raw materials, koji, microorganisms, fermentation techniques and ageing process (Yang et al., 2020). Rice wine is generally produced through open fermentation, which can involve the interaction with microorganisms in the surrounding environment to increase the microorganism diversity. These microorganisms tend to undergo dynamic variations during fermentation with changes in the system conditions (Liu et al., 2020). This process is referred to as microbial community succession (Liu et al., 2020), which can confer rice wine with unique flavour characteristics (Huang et al., 2018; Liu et al., 2020). Therefore, clarifying the relation between microbial community succession and flavour compounds in rice wine can assist understanding of the flavour compound formation during rice wine fermentation.
Chestnut rice wine (banli mijiu, BLMJ) is produced by replacing a portion of glutinous rice with raw chestnut kernels (about 25% of the total raw material weight). After mixing of the chestnut kernels with soaked and drained glutinous rice, the mixture is steamed, cooled, mixed with koji and then fermented in fermentation vessels (Zou et al., 2022). Compared with pure glutinous rice wine without chestnut, BLMJ has a more desirable amino acid structure and stronger antioxidant capacity (Zou et al., 2022). Moreover, addition of chestnut can significantly alter the types and amounts of volatile compounds in the final product (Zou et al., 2022). A total of ninety-eight volatile compounds were detected in BLMJ, among which thirty-eight were identifiable by olfactory perception; in contrast, only seventy-seven volatile compounds were detected in the same pure glutinous rice wine fermentation process, twenty-two of which were identifiable by olfactory perception. More interestingly, only twenty-one common olfactory perceptive volatile compounds were shared by the two types of rice wine (Zou et al., 2022).
The type of microorganisms during the fermentation process is one of the main factors affecting the flavour of rice wine. During the fermentation process of rice wine, Saccharomyces is the main fungus, which is the main producer of ethanol and some flavour substances. There are also significant differences in the types and quantities of alcohols and esters produced by different brewing yeast strains (Yang et al., 2017). In addition, lactic acid bacteria are also the main bacteria in the fermentation process of rice wine. Yang et al. found that different types of lactic acid bacteria have a significant impact on the flavour of rice wine (Yang et al., 2024). Liu et al. found that Pantoea, Lactiplantibacillus, Lactobacillus, Leuconostoc and Weissella are the main dominant bacteria in Hong Qu glutinous rice wine, with Monascus being the main fungus. Among them, Lactiplantibacillus is closely related to the formation of flavour compounds. During the fermentation process of red yeast glutinous rice wine, Lactiplantibacillus increased from 0.1% to 12.9% and Lactobacillus increased from 0.02% to 2.7%. On the 6th day of fermentation, the ester substances in red yeast glutinous rice wine increased most significantly (Liu et al., 2022). The microbial community in different types of rice wine is impacted by temperature, moisty, raw materials and fermentation process, producing different aroma compounds. Thus, understanding the formation of specific flavour compounds in BLMJ during fermentation may help to significantly improve the quality and flavour of rice wine.
In this study, we measured the physicochemical properties, volatile compound content and microbial diversity of BLMJ at different fermentation stages and explored the correlation between microbial community and volatile compounds. The study aims to elucidate the contribution of microbial community to the volatile compounds in BLMJ during fermentation and identify the critical microbial contributors to the formation of flavour.
Materials and methods
Materials
2-Octanol (internal standard, chromatographic purity) was obtained from Sigma-Aldrich Co. (Shanghai, China). The koji starter was obtained from the Beizong Rice Wine Co., Ltd. (Zhangjiakou, China). Glutinous rice is commercially available (Zhangjiakou, China). The other reagents used in this study were obtained from Aladdin Co. (Shanghai, China).
Methods
Chestnut rice wine brewing process and sampling
The brewing of BLMJ was carried out following the method described by Zou et al. (2022). Glutinous rice was soaked in water at 20 °C for 24 h and drained. Chestnut kernels were crushed into rice-sized particles and mixed with glutinous rice in a 1: 3 (w/w) ratio. The mixture was steamed for 35 min, with stirring once in the middle to ensure that the rice particles were soft. After cooling, the rice-chestnut mixture was mixed with water, followed by the addition of koji at a concentration of 10% (w/w) of the raw material dry weight and active dry yeast at a concentration of 0.2% (w/w). The mixture was incubated at 30 °C for 24 h. Subsequently, pure water was added in a 1:1.5 ratio (w/w) and fermentation was carried out at 25 °C for 9 days, with daily stirring from the 3rd day to prevent excessive temperature rise. Once the surface of the fermentation broth became calm, the fermentation vessel was sealed and maintained at 18 °C for 20 days until the end of fermentation.
The entire fermentation period was divided into three stages: early fermentation (0–3 days), middle fermentation (4–9 days) and late fermentation (10–29 days). Samples were collected from the upper and lower layers of the fermentation broth, with 30 g per sample, on day 0, day 1 and day 3 of the early fermentation stage (denoted as D_0, D_1 and D-3, respectively), day 5 and day 9 of the middle fermentation stage (D_5 and D_9) and day 15 and day 27 of the late fermentation stage (D_15 and D_27). The samples were centrifuged at 12 000 rpm for 10 min at 4 °C and the supernatant was collected for volatile compound analysis, while the sediment was used to analyse the microbial diversity. All samples were stored in sterile sealed bags and kept at −80 °C before analysis.
Determination of total reducing sugar (TRS), amino acid nitrogen (AAN), titratable acidity (TA), glucoamylase and α-amylase activity
Determination of titratable acidity (TA), ethanol and amino acid nitrogen was carried out using the methods of Chinese National Standard GB/T 13662–2018. The activity of glucoamylase and α-amylase activity was determined by the method of Ren et al. (2023). The pH of the sample was tested by a pH meter.
Determination of the volatile profile by HS-SPME-GC/MS
The volatile compounds in BLMJ were qualitatively and semi-quantitatively analysed through headspace solid-phase microextraction (HS-SPME) combined with gas chromatography–mass spectrometry (GC–MS). To perform the analysis, 8 mL of supernatant was added to a 20 mL clear round-bottom headspace sample vial, along with 2 g of NaCl and 1 μL of 2-octanol as an internal standard. Carboxen/polydimethylsiloxane (CAR/PDMS) SPME fibre (75 μm, Fused Silica 24 Ga, Manual Holder, Supelco, Bellefonte, PA, USA) was inserted above the liquid surface and allowed to extract the volatile compounds for 50 min at 60 °C.
The GC–MS conditions were as follows: an Agilent 7890 GC system (Agilent Technologies, Santa Clara, CA, USA) was used with a DB-5 quartz capillary column (60 m × 0.25 mm × 0.25 μm, Agilent Technologies, Foster City, CA, USA). The injection port temperature was 250 °C; the temperature programme started at 50 °C, held for 2 min, increased at a rate of 2 °C min−1 to 115 °C, held for 3 min, increased at a rate of 4 °C min−1 to 200 °C, further increased at a rate of 6 °C min−1 to 230 °C and held for 10 min. The carrier gas used was ultrahigh-purity helium, with a flow rate of 1 mL min−1. Electron ionisation (EI) at 70 eV was employed and the mass scanning range was set to m/z 50–550. The volatile flavour compounds were identified by comparing their mass spectra with the National Institute of Standards and Technology (NIST) library (Zou et al., 2022).
Semi-quantitative analysis of flavour compounds in the samples was conducted using the following formula:
In the formula, Cx represents the concentration of a specific flavour compound in BLMJ, μg L−1; Cs indicates the concentration of the internal standard, μg L−1; Ax stands for the peak area of the target flavour compound in BLMJ; and As represents the peak area of the internal standard.
Analysis of the odour activity value
Analysis of odour activity value (OAV) was performed in the following formula:
By calculating the OAV of each volatile compound in BLMJ, the contribution of each compound to the flavour can be determined. It is generally accepted that compounds with an OAV >1 contribute to the flavour, while those with an OAV > 10 are essential flavour compounds.
Microbial community analysis by paired-end illumina MiSeq sequencing
Microbial community structural determination was carried out to elucidate the composition of microorganisms in BLMJ. Microbial DNA was extracted from 0.5 g samples using a PowerSoil Total DNA Isolation Kit (MO BIO, Carlsbad, CA, USA) and the concentration and quality of the extracted DNA were determined by spectrophotometry (NanoDrop 2000; Thermo, Japan) and 1% agarose gel electrophoresis. The V3-V4 region of the bacterial 16S rRNA gene was amplified by PCR using the universal primers 341F (5'-CCTAYGGGRBGCASCAG-3′) and 806R (5'-GGACTACHVGGGTWTCTAAT-3'). The PCR amplification programme consisted of an initial denaturation at 95 °C for 2 min, followed by 25 cycles of denaturation at 95 °C for 30 s, annealing at 55 °C for 30 s and extension at 72 °C for 10 min. Similarly, the ITS1 region of fungi was amplified by PCR using the primers ITS5-1737-F (5'-GGAAGTAAAAGTCGTAACAAGG-3′) and ITS2-2043-R (5′-GCTGCGTTCTTCATCGATGC-3′), with the PCR amplification programme consisting of an initial denaturation at 95 °C for 2 min, followed by 30 cycles of denaturation at 95 °C for 30 s, annealing at 61 °C for 30 s and extension at 72 °C for 10 min (Liu et al., 2020). The PCR products were analysed using 2% agarose gel electrophoresis and the purified amplicons were quantified using an AxyPrepDNA Gel Recovery Kit obtained from AXYGEN Co., Ltd., (Hangzhou, China) and pooled in equimolar amounts. The pooled amplicons were then subjected to sequencing on the Illumina MiSeq PE300 platform by Majorbio Co., Ltd. (Shanghai, China). The Illumina sequencing raw data were deposited in the Sequence Read Archive (SRA) in the National Center for Biotechnology Information (NCBI) database as a BioProject (https://www.ncbi.nlm.nih.gov/sra/PRJNA1006861, accessed on 19 August 2023). The accession number is PRJNA1006861.
The MiSeq sequencing PE reads were assembled using fastp and FLASH software based on the overlapping relationship between the reads. Quality control filtering was performed to obtain high-quality sequences and sequence orientation was corrected to obtain optimised data (Liu et al., 2020). The optimised data were subjected to operational taxonomic unit (OTU) clustering and taxonomic classification analyses (Xie et al., 2021). The non-redundant sequences (excluding singleton sequences) were clustered into OTUs at a 97% similarity cutoff value using Uparse software (version 7.0.1090 http://drive5.com/uparse/) and chimeric sequences were removed during the clustering process to obtain representative sequences for each OUT to generate an OTU table (Liu et al., 2015). To assign taxonomic information to each OTU, the RDP classifier Bayesian algorithm was used to perform taxonomic classification analysis on the representative sequences of OTUs at the 97% similarity. The community species composition of each sample was then determined by various taxonomic groups. The representative OTU sequences were also annotated using the Silva (version 138) and Unite (version 8.0) databases, respectively, with RDP-classifier (v.2.2). The microbial community structure in the samples was analysed based on the OTU clustering results.
Association analysis between the microbial community and volatile compounds
The correlations between microorganisms and volatile compounds during the fermentation process of BLMJ were estimated using Spearman's correlation analysis with heatmap analysis. Furthermore, the relationship between microorganisms and volatile compounds was visualised to represent the contribution of microorganisms to the volatile profile.
Data processing
Each parameter was subjected to three experimental replicates and the experimental data were statistically analysed using SPSS (v.25.0.0) software (IBM, Armonk, NY, USA). The α-diversity index of the samples was calculated using Mothur (version v.1.30.2). The dilution curve, Venn diagram, stacked bar chart of dominant bacterial taxa, principal component analysis (PCA) and heat map depicting the correlation between environmental factors were visualised using Origin 2021 and R software (version 3.3.1).
Results and discussion
Physicochemical properties during the fermentation process
The changes in physicochemical indices during fermentation can reflect the state and degree of fermentation, as well as the microbial state in the fermentation solution to a certain extent (Qian et al., 2023). The physicochemical properties (titratable acidity, pH value, ethanol content, amino acid nitrogen, glycosylase and α-amylase activities) during BLMJ fermentation are shown in Table 1. The activity of amylolytic enzyme can reflect the growth of microbial community and production of saccharide that could be transformed to ethanol. At the early and middle stages of fermentation (0–216 h, D_0–D_9), the activity of α-amylase reached the peak value after 24 h and glycosylase gradually increased and reached the maximum value at 216 h; correspondingly, the reducing sugar content reached the peak value at 24 h (190 g L−1) and gradually decreased, indicating the decomposition of carbohydrates in raw materials to provide carbon source for the microbial community in the fermentation process. Moreover, the amino acid nitrogen content gradually increased and reached 0.17 g L−1 at 24 h, followed by gradual decreases. With the proceeding of fermentation, the titratable acidity gradually increased and reached the maximum value (5.13 g L−1) at 27 days of fermentation and then decreased gradually to become stabilised. At the later stage of fermentation, due to increases in alcohol and total acids, microbial growth and metabolism were inhibited, resulting in a decrease in enzyme activity. These results are generally consistent with those of others research (Ren et al., 2019) (Fig. 1).
Name . | Fermentation days(d) . | ||||||
---|---|---|---|---|---|---|---|
0 . | 1 . | 3 . | 5 . | 9 . | 15 . | 27 . | |
titratable acidity(g L−1) | 2.01 ± 0.01f | 2.05 ± 0.01f | 3.05 ± 0.02e | 4.17 ± 0.03d | 4.95 ± 0.02c | 5.01 ± 0.01b | 5.13 ± 0.02a |
reducing sugar(g L−1) | 78.39 ± 3.23b | 190.09 ± 5.33a | 108.38 ± 6.22a | 54.84 ± 2.10c | 20.68 ± 1.94d | 18.45 ± 2.51d | 14.41 ± 0.33e |
pH value | 6.03 ± 0.02a | 5.97 ± 0.03a | 4.33 ± 0.01b | 4.08 ± 0.04cd | 4.03 ± 0.02d | 4.10 ± 0.04cd | 4.13 ± 0.03c |
Ethanol (v/v%) | 1.25 ± 0.01g | 3.43 ± 0.02f | 7.92 ± 0.03e | 10.01 ± 0.02d | 15.03 ± 0.02c | 15.17 ± 0.03b | 15.97 ± 0.03a |
amino acid nitrogen(g L−1) | 0.13 ± 0.01b | 0.17 ± 0.00a | 0.10 ± 0.00c | 0.05 ± 0.02d | 0.04 ± 0.01d | 0.03 ± 0.01d | 0.03 ± 0.00d |
α-amylase (U mL−1) | 50.33 ± 2.30b | 72.22 ± 1.51a | 48.04 ± 2.62b | 33.46 ± 2.34c | 22.60 ± 1.31d | 15.66 ± 1.60e | 14.82 ± 1.42e |
glucoamylase (U mL−1) | 23.64 ± 0.51de | 25.57 ± 1.20cd | 28.90 ± 1.41c | 32.97 ± 1.51b | 53.33 ± 2.43a | 25.67 ± 2.34cd | 20.32 ± 1.82e |
Name . | Fermentation days(d) . | ||||||
---|---|---|---|---|---|---|---|
0 . | 1 . | 3 . | 5 . | 9 . | 15 . | 27 . | |
titratable acidity(g L−1) | 2.01 ± 0.01f | 2.05 ± 0.01f | 3.05 ± 0.02e | 4.17 ± 0.03d | 4.95 ± 0.02c | 5.01 ± 0.01b | 5.13 ± 0.02a |
reducing sugar(g L−1) | 78.39 ± 3.23b | 190.09 ± 5.33a | 108.38 ± 6.22a | 54.84 ± 2.10c | 20.68 ± 1.94d | 18.45 ± 2.51d | 14.41 ± 0.33e |
pH value | 6.03 ± 0.02a | 5.97 ± 0.03a | 4.33 ± 0.01b | 4.08 ± 0.04cd | 4.03 ± 0.02d | 4.10 ± 0.04cd | 4.13 ± 0.03c |
Ethanol (v/v%) | 1.25 ± 0.01g | 3.43 ± 0.02f | 7.92 ± 0.03e | 10.01 ± 0.02d | 15.03 ± 0.02c | 15.17 ± 0.03b | 15.97 ± 0.03a |
amino acid nitrogen(g L−1) | 0.13 ± 0.01b | 0.17 ± 0.00a | 0.10 ± 0.00c | 0.05 ± 0.02d | 0.04 ± 0.01d | 0.03 ± 0.01d | 0.03 ± 0.00d |
α-amylase (U mL−1) | 50.33 ± 2.30b | 72.22 ± 1.51a | 48.04 ± 2.62b | 33.46 ± 2.34c | 22.60 ± 1.31d | 15.66 ± 1.60e | 14.82 ± 1.42e |
glucoamylase (U mL−1) | 23.64 ± 0.51de | 25.57 ± 1.20cd | 28.90 ± 1.41c | 32.97 ± 1.51b | 53.33 ± 2.43a | 25.67 ± 2.34cd | 20.32 ± 1.82e |
The letters (a, b, c, etc.) in the same row represent the mean values with statistically significant differences obtained through Duncan's post-hoc test. P = 0.05.
Name . | Fermentation days(d) . | ||||||
---|---|---|---|---|---|---|---|
0 . | 1 . | 3 . | 5 . | 9 . | 15 . | 27 . | |
titratable acidity(g L−1) | 2.01 ± 0.01f | 2.05 ± 0.01f | 3.05 ± 0.02e | 4.17 ± 0.03d | 4.95 ± 0.02c | 5.01 ± 0.01b | 5.13 ± 0.02a |
reducing sugar(g L−1) | 78.39 ± 3.23b | 190.09 ± 5.33a | 108.38 ± 6.22a | 54.84 ± 2.10c | 20.68 ± 1.94d | 18.45 ± 2.51d | 14.41 ± 0.33e |
pH value | 6.03 ± 0.02a | 5.97 ± 0.03a | 4.33 ± 0.01b | 4.08 ± 0.04cd | 4.03 ± 0.02d | 4.10 ± 0.04cd | 4.13 ± 0.03c |
Ethanol (v/v%) | 1.25 ± 0.01g | 3.43 ± 0.02f | 7.92 ± 0.03e | 10.01 ± 0.02d | 15.03 ± 0.02c | 15.17 ± 0.03b | 15.97 ± 0.03a |
amino acid nitrogen(g L−1) | 0.13 ± 0.01b | 0.17 ± 0.00a | 0.10 ± 0.00c | 0.05 ± 0.02d | 0.04 ± 0.01d | 0.03 ± 0.01d | 0.03 ± 0.00d |
α-amylase (U mL−1) | 50.33 ± 2.30b | 72.22 ± 1.51a | 48.04 ± 2.62b | 33.46 ± 2.34c | 22.60 ± 1.31d | 15.66 ± 1.60e | 14.82 ± 1.42e |
glucoamylase (U mL−1) | 23.64 ± 0.51de | 25.57 ± 1.20cd | 28.90 ± 1.41c | 32.97 ± 1.51b | 53.33 ± 2.43a | 25.67 ± 2.34cd | 20.32 ± 1.82e |
Name . | Fermentation days(d) . | ||||||
---|---|---|---|---|---|---|---|
0 . | 1 . | 3 . | 5 . | 9 . | 15 . | 27 . | |
titratable acidity(g L−1) | 2.01 ± 0.01f | 2.05 ± 0.01f | 3.05 ± 0.02e | 4.17 ± 0.03d | 4.95 ± 0.02c | 5.01 ± 0.01b | 5.13 ± 0.02a |
reducing sugar(g L−1) | 78.39 ± 3.23b | 190.09 ± 5.33a | 108.38 ± 6.22a | 54.84 ± 2.10c | 20.68 ± 1.94d | 18.45 ± 2.51d | 14.41 ± 0.33e |
pH value | 6.03 ± 0.02a | 5.97 ± 0.03a | 4.33 ± 0.01b | 4.08 ± 0.04cd | 4.03 ± 0.02d | 4.10 ± 0.04cd | 4.13 ± 0.03c |
Ethanol (v/v%) | 1.25 ± 0.01g | 3.43 ± 0.02f | 7.92 ± 0.03e | 10.01 ± 0.02d | 15.03 ± 0.02c | 15.17 ± 0.03b | 15.97 ± 0.03a |
amino acid nitrogen(g L−1) | 0.13 ± 0.01b | 0.17 ± 0.00a | 0.10 ± 0.00c | 0.05 ± 0.02d | 0.04 ± 0.01d | 0.03 ± 0.01d | 0.03 ± 0.00d |
α-amylase (U mL−1) | 50.33 ± 2.30b | 72.22 ± 1.51a | 48.04 ± 2.62b | 33.46 ± 2.34c | 22.60 ± 1.31d | 15.66 ± 1.60e | 14.82 ± 1.42e |
glucoamylase (U mL−1) | 23.64 ± 0.51de | 25.57 ± 1.20cd | 28.90 ± 1.41c | 32.97 ± 1.51b | 53.33 ± 2.43a | 25.67 ± 2.34cd | 20.32 ± 1.82e |
The letters (a, b, c, etc.) in the same row represent the mean values with statistically significant differences obtained through Duncan's post-hoc test. P = 0.05.
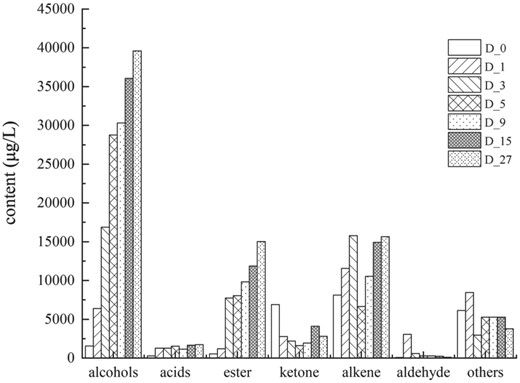
Changes in relative contents of various volatile components during the fermentation of BLMJ.
Volatile compounds during the fermentation of BLMJ
The volatile compounds in BLMJ produced during the fermentation process were analysed using the HS-SPME-GC–MS technique. The volatile compounds with a reliability score ≥ 80 were selected as the main flavour compounds generated during the fermentation (Table S1).
A total of sixty-four volatile compounds were detected during the entire fermentation process, including ten alcohols, five acids, fourteen esters, six ketones, twenty-five alkenes, two aldehydes and two other classes of volatile compounds. At the D_0 stage (0 day), thirty-six volatile compounds were detected, with alkenes accounting for the largest proportion (34.44%) of the total volatile compounds, followed by ketones at 29.25%. Among individual compounds, 2-methoxyphenol was the most abundant, accounting for 25.98% of the total. These volatile compounds were primarily originated from koji and raw materials during the cooking process (Liu et al., 2019).
After 24 h of fermentation (D_1), alkenes remained the main volatile compounds in the fermented broth, accounting for 40.22% of the total volatile compounds and the total amount of alcohols significantly increased (P < 0.5), accounting for 16.48% of the total volatile compounds. The content of ketones significantly decreased, accounting for 7.16%, while that of esters, aldehydes and acids slightly increased. At the mid-fermentation stage (96–216 h, D_4-D_9), the content of alcohols continued to significantly increase, reaching 51.12% of the total volatile compounds at 216 h. The content of esters also showed a considerable increase compared with that at D_1, accounting for 16.57% of the total volatile compounds at 216 h. The content of aldehydes further decreased. At the late fermentation stage, there was no significant change in the proportion of alcohols and esters in the total volatile compounds compared with that at the mid-fermentation stage. In contrast, the proportion of alkenes significantly increased (P < 0.05), reaching 20.12% at 15 days.
At the end of BLMJ fermentation (D_27), the total amount of volatile compounds reached its highest (78 692.09 μg L−1). Except for a slight decrease in ketones, the content of other volatile compounds has increased. Alcohols are the main volatile substances in BLMJ, accounting for 50.33% of the total volatile substances, with β-phenylethanol having the highest content. Esters are the main source of aroma in rice wine. By the 27th day of fermentation, the ester content in BLMJ reached its highest level (15 020.4 μg L−1). Among them, ethyl palmitate, ethyl decanoate, ethyl laurate and ethyl phenylacetate have a higher content, accounting for 80.67% of esters.
At the late fermentation stage, the main alcohol compounds were β-phenylethanol and isoamyl alcohol. It has been reported that yeast synthesises β-phenylethanol through the phenylpyruvic acid pathway, the Shikimate pathway and the Ehrlich pathway (Etschmann et al., 2002), while isoamyl alcohol is produced through the leucine degradation pathway (Yuan et al., 2017). Moreover, ethyl decanoate, ethyl laurate and ethyl palmitate, which have floral and fruity aromas, were the most abundant esters at the late fermentation stage, which are similar to the major esters reported in yellow rice wine (Chen et al., 2013; Yu et al., 2019). Additionally, there were also high contents of 2-octanone, 2-dodecene, tridecane and 2-methoxyphenol at this stage.
As shown in Table 2, at the early fermentation stage (0–72 h, D_0–D_3), fourteen species of main volatile compounds were formed during BLMJ fermentation (OAV >1). At the D_1 day, ethyl octanoate, benzene acetaldehyde and 2-methoxyphenol were the dominant volatile compounds (OAV >10). At the mid-fermentation stage (96–216 h, D_4–D_9), thirteen species of volatile compounds (OAV >1) were detected, among which nine species were dominant volatile compounds (OAV > 10), including four esters. After the fermentation of BLMJ (D_27), there were twelve species of volatile compounds with OAV >1. Among them, ethyl decanoate, ethyl caproate, ethyl octanoate and ethyl isovalerate (conferring fruity and fatty aromas), β-phenylethanol (conferring a rosy and honey aroma) and 2-methoxyphenol (conferring a spicy and clove aroma) were dominant volatile compounds in BLMJ with OAV >10. During BLMJ fermentation, a total of eighteen important volatile compounds (OAV >1) were detected.
Volatile compounds . | Threshold(μg L−1) . | References . | Odour descriptor . | OAV values for different fermentation days . | ||||||
---|---|---|---|---|---|---|---|---|---|---|
0 . | 1 . | 3 . | 5 . | 9 . | 15 . | 27 . | ||||
β-Phenylethanol | 1380 | Pino & Queris (2011) | Rosy, honey | 0.16 | 3.83 | 11.47 | 19.19 | 20.00 | 23.73 | 26.31 |
Heptanol | 425 | Pino & Queris (2011) | Fruity | 2.25 | 1.81 | 0.59 | 0.51 | 0.51 | 0.46 | 0.41 |
1-Octanol | 200 | Pino & Queris (2011) | Fruity | 0.83 | 0.85 | 0.51 | 0.90 | 1.1 | 0.64 | 0.32 |
2-Nonanol | 70 | Fan et al. (2020) | Cucumber | 3.13 | 1.83 | — | — | — | — | — |
Benzyl alcohol | 900 | Chen et al. (2013) | Floral | 0 | 0.03 | 0.01 | 0.01 | 0.01 | — | — |
3-methylthiopropanol | 30 000 | Chen et al. (2013) | Alcoholic, nail polish | — | — | 0.02 | 0.04 | 0.05 | 0.07 | 0.08 |
Isobutanol | 40 000 | Yang et al. (2022) | Nail polish | — | — | 0 | 0.01 | 0.01 | 0.01 | 0.01 |
3-(Methylthio)-propanol | 1000 | Yang et al. (2022) | Meat | — | — | 0.06 | 0.02 | 0.07 | 0.08 | 0.09 |
3-Octanol | 393 | Wang et al. (2014a, 2014b) | Green, mushroom | — | — | 0.11 | 0.04 | 0.23 | 0.01 | — |
2,3-Butanediol | 30 | Yu et al. (2019) | — | — | — | 9.12 | 10.21 | 12.89 | 2.43 | |
Acetic acid | 200 000 | Yang et al. (2022) | Vinegar | 0 | 0 | 0 | 0 | 0 | 0 | 0 |
Hexanoic acid | 420 | Gómez-Míguez et al. (2007) | Cheese, acidic | 0.04 | 0.31 | 0.40 | 0.82 | 0.44 | 0.40 | 0.17 |
Octanoic acid | 500 | Gómez-Míguez et al. (2007) | Sweat, cheese | — | 0.29 | 0.54 | 0.33 | 0.31 | 0.92 | 0.90 |
Decanoic acid | 1000 | Gómez-Míguez et al. (2007) | Sweaty | — | 0.04 | 0.12 | 0.10 | 0.21 | 0.20 | 0.03 |
Ethyl palmitate | 1000 | Rahayu et al. (2017) | Oily | 0.10 | 0.14 | 0.59 | 0.24 | 0.59 | 1.75 | 2.25 |
Ethyl decanoate | 200 | Yang et al. (2022) | Fruity | 0.67 | 1.57 | 15.83 | 19.60 | 19.32 | 30.18 | 31.19 |
Ethyl myristate | 2000 | Yang et al. (2022) | Ether | 0.02 | 0.08 | 0.23 | 0.08 | 0.14 | 0.08 | 0.36 |
Ethyl benzoate | 580 | Yang et al. (2022) | Chamomile, fruity | 0.06 | 0.16 | 0.09 | 0.05 | 0.17 | 0.10 | 0.05 |
Ethyl laurate | 1500 | Yang et al. (2022) | Leaf | 0.07 | 0.10 | 0.79 | 0.45 | 1.27 | 0.18 | 1.56 |
Ethyl nonanoate | 200 | Yang et al. (2022) | Fruity | — | 0.28 | 1.35 | 1.18 | 0.79 | 2.46 | 3.10 |
Ethyl caproate | 14 | Yang et al. (2022) | Apple peel, fruit | — | 0.83 | 33.54 | 25.08 | 7.94 | 15.21 | 10.67 |
Ethyl octanoate | 5 | Yang et al. (2022) | Fruit, fat | — | 38.24 | 145.01 | 316.70 | 259.29 | 230.70 | 144.09 |
Ethyl isovalerate | 3 | Rahayu et al. (2017) | Apple | — | — | 29.26 | 27.76 | 26.42 | 19.12 | 16.48 |
Ethyl heptanoate | 2 | Chen et al. (2019) | Fruity | — | — | 13.00 | 19.67 | 11.43 | — | — |
Benzaldehyde | 990 | Chen et al. (2019) | Almond | 0.08 | 2.10 | 0.46 | 0.16 | 0.16 | 0.14 | 0.09 |
Acetophenone | 65 | Chen et al. (2019) | Floral | 0.68 | 0.58 | 0.60 | 0.55 | 0.52 | 0.34 | — |
2-Octanone | 1100 | Yang et al. (2022) | Apple peel | 6.19 | 2.47 | 1.95 | 1.73 | 1.72 | 3.70 | 2.52 |
Benzene acetaldehyde | 4 | Yang et al. (2022) | Honey | — | 240.13 | 30.16 | 30.26 | 30.31 | 20.11 | 6.10 |
D-Limonene | 30 | Chen et al. (2019) | Citrus, mint | 1.04 | — | — | 0.48 | 0.01 | — | 0.56 |
2-Methoxyphenol | 9.5 | Chen et al. (2019) | Spicy, clove | 644.53 | 878.03 | 310.28 | 554.09 | 553.93 | 554.86 | 396.40 |
Volatile compounds . | Threshold(μg L−1) . | References . | Odour descriptor . | OAV values for different fermentation days . | ||||||
---|---|---|---|---|---|---|---|---|---|---|
0 . | 1 . | 3 . | 5 . | 9 . | 15 . | 27 . | ||||
β-Phenylethanol | 1380 | Pino & Queris (2011) | Rosy, honey | 0.16 | 3.83 | 11.47 | 19.19 | 20.00 | 23.73 | 26.31 |
Heptanol | 425 | Pino & Queris (2011) | Fruity | 2.25 | 1.81 | 0.59 | 0.51 | 0.51 | 0.46 | 0.41 |
1-Octanol | 200 | Pino & Queris (2011) | Fruity | 0.83 | 0.85 | 0.51 | 0.90 | 1.1 | 0.64 | 0.32 |
2-Nonanol | 70 | Fan et al. (2020) | Cucumber | 3.13 | 1.83 | — | — | — | — | — |
Benzyl alcohol | 900 | Chen et al. (2013) | Floral | 0 | 0.03 | 0.01 | 0.01 | 0.01 | — | — |
3-methylthiopropanol | 30 000 | Chen et al. (2013) | Alcoholic, nail polish | — | — | 0.02 | 0.04 | 0.05 | 0.07 | 0.08 |
Isobutanol | 40 000 | Yang et al. (2022) | Nail polish | — | — | 0 | 0.01 | 0.01 | 0.01 | 0.01 |
3-(Methylthio)-propanol | 1000 | Yang et al. (2022) | Meat | — | — | 0.06 | 0.02 | 0.07 | 0.08 | 0.09 |
3-Octanol | 393 | Wang et al. (2014a, 2014b) | Green, mushroom | — | — | 0.11 | 0.04 | 0.23 | 0.01 | — |
2,3-Butanediol | 30 | Yu et al. (2019) | — | — | — | 9.12 | 10.21 | 12.89 | 2.43 | |
Acetic acid | 200 000 | Yang et al. (2022) | Vinegar | 0 | 0 | 0 | 0 | 0 | 0 | 0 |
Hexanoic acid | 420 | Gómez-Míguez et al. (2007) | Cheese, acidic | 0.04 | 0.31 | 0.40 | 0.82 | 0.44 | 0.40 | 0.17 |
Octanoic acid | 500 | Gómez-Míguez et al. (2007) | Sweat, cheese | — | 0.29 | 0.54 | 0.33 | 0.31 | 0.92 | 0.90 |
Decanoic acid | 1000 | Gómez-Míguez et al. (2007) | Sweaty | — | 0.04 | 0.12 | 0.10 | 0.21 | 0.20 | 0.03 |
Ethyl palmitate | 1000 | Rahayu et al. (2017) | Oily | 0.10 | 0.14 | 0.59 | 0.24 | 0.59 | 1.75 | 2.25 |
Ethyl decanoate | 200 | Yang et al. (2022) | Fruity | 0.67 | 1.57 | 15.83 | 19.60 | 19.32 | 30.18 | 31.19 |
Ethyl myristate | 2000 | Yang et al. (2022) | Ether | 0.02 | 0.08 | 0.23 | 0.08 | 0.14 | 0.08 | 0.36 |
Ethyl benzoate | 580 | Yang et al. (2022) | Chamomile, fruity | 0.06 | 0.16 | 0.09 | 0.05 | 0.17 | 0.10 | 0.05 |
Ethyl laurate | 1500 | Yang et al. (2022) | Leaf | 0.07 | 0.10 | 0.79 | 0.45 | 1.27 | 0.18 | 1.56 |
Ethyl nonanoate | 200 | Yang et al. (2022) | Fruity | — | 0.28 | 1.35 | 1.18 | 0.79 | 2.46 | 3.10 |
Ethyl caproate | 14 | Yang et al. (2022) | Apple peel, fruit | — | 0.83 | 33.54 | 25.08 | 7.94 | 15.21 | 10.67 |
Ethyl octanoate | 5 | Yang et al. (2022) | Fruit, fat | — | 38.24 | 145.01 | 316.70 | 259.29 | 230.70 | 144.09 |
Ethyl isovalerate | 3 | Rahayu et al. (2017) | Apple | — | — | 29.26 | 27.76 | 26.42 | 19.12 | 16.48 |
Ethyl heptanoate | 2 | Chen et al. (2019) | Fruity | — | — | 13.00 | 19.67 | 11.43 | — | — |
Benzaldehyde | 990 | Chen et al. (2019) | Almond | 0.08 | 2.10 | 0.46 | 0.16 | 0.16 | 0.14 | 0.09 |
Acetophenone | 65 | Chen et al. (2019) | Floral | 0.68 | 0.58 | 0.60 | 0.55 | 0.52 | 0.34 | — |
2-Octanone | 1100 | Yang et al. (2022) | Apple peel | 6.19 | 2.47 | 1.95 | 1.73 | 1.72 | 3.70 | 2.52 |
Benzene acetaldehyde | 4 | Yang et al. (2022) | Honey | — | 240.13 | 30.16 | 30.26 | 30.31 | 20.11 | 6.10 |
D-Limonene | 30 | Chen et al. (2019) | Citrus, mint | 1.04 | — | — | 0.48 | 0.01 | — | 0.56 |
2-Methoxyphenol | 9.5 | Chen et al. (2019) | Spicy, clove | 644.53 | 878.03 | 310.28 | 554.09 | 553.93 | 554.86 | 396.40 |
The odour threshold in a 40% (v/v) water/ethanol solution; odour threshold in a 12% (v/v) water/ethanol solution for other compounds. Aroma descriptor was derived from the web pages: (http://www.leffingwell.com) (http://flavornet.org/flavornet.html) and (http://www.odour.org.uk/).
Volatile compounds . | Threshold(μg L−1) . | References . | Odour descriptor . | OAV values for different fermentation days . | ||||||
---|---|---|---|---|---|---|---|---|---|---|
0 . | 1 . | 3 . | 5 . | 9 . | 15 . | 27 . | ||||
β-Phenylethanol | 1380 | Pino & Queris (2011) | Rosy, honey | 0.16 | 3.83 | 11.47 | 19.19 | 20.00 | 23.73 | 26.31 |
Heptanol | 425 | Pino & Queris (2011) | Fruity | 2.25 | 1.81 | 0.59 | 0.51 | 0.51 | 0.46 | 0.41 |
1-Octanol | 200 | Pino & Queris (2011) | Fruity | 0.83 | 0.85 | 0.51 | 0.90 | 1.1 | 0.64 | 0.32 |
2-Nonanol | 70 | Fan et al. (2020) | Cucumber | 3.13 | 1.83 | — | — | — | — | — |
Benzyl alcohol | 900 | Chen et al. (2013) | Floral | 0 | 0.03 | 0.01 | 0.01 | 0.01 | — | — |
3-methylthiopropanol | 30 000 | Chen et al. (2013) | Alcoholic, nail polish | — | — | 0.02 | 0.04 | 0.05 | 0.07 | 0.08 |
Isobutanol | 40 000 | Yang et al. (2022) | Nail polish | — | — | 0 | 0.01 | 0.01 | 0.01 | 0.01 |
3-(Methylthio)-propanol | 1000 | Yang et al. (2022) | Meat | — | — | 0.06 | 0.02 | 0.07 | 0.08 | 0.09 |
3-Octanol | 393 | Wang et al. (2014a, 2014b) | Green, mushroom | — | — | 0.11 | 0.04 | 0.23 | 0.01 | — |
2,3-Butanediol | 30 | Yu et al. (2019) | — | — | — | 9.12 | 10.21 | 12.89 | 2.43 | |
Acetic acid | 200 000 | Yang et al. (2022) | Vinegar | 0 | 0 | 0 | 0 | 0 | 0 | 0 |
Hexanoic acid | 420 | Gómez-Míguez et al. (2007) | Cheese, acidic | 0.04 | 0.31 | 0.40 | 0.82 | 0.44 | 0.40 | 0.17 |
Octanoic acid | 500 | Gómez-Míguez et al. (2007) | Sweat, cheese | — | 0.29 | 0.54 | 0.33 | 0.31 | 0.92 | 0.90 |
Decanoic acid | 1000 | Gómez-Míguez et al. (2007) | Sweaty | — | 0.04 | 0.12 | 0.10 | 0.21 | 0.20 | 0.03 |
Ethyl palmitate | 1000 | Rahayu et al. (2017) | Oily | 0.10 | 0.14 | 0.59 | 0.24 | 0.59 | 1.75 | 2.25 |
Ethyl decanoate | 200 | Yang et al. (2022) | Fruity | 0.67 | 1.57 | 15.83 | 19.60 | 19.32 | 30.18 | 31.19 |
Ethyl myristate | 2000 | Yang et al. (2022) | Ether | 0.02 | 0.08 | 0.23 | 0.08 | 0.14 | 0.08 | 0.36 |
Ethyl benzoate | 580 | Yang et al. (2022) | Chamomile, fruity | 0.06 | 0.16 | 0.09 | 0.05 | 0.17 | 0.10 | 0.05 |
Ethyl laurate | 1500 | Yang et al. (2022) | Leaf | 0.07 | 0.10 | 0.79 | 0.45 | 1.27 | 0.18 | 1.56 |
Ethyl nonanoate | 200 | Yang et al. (2022) | Fruity | — | 0.28 | 1.35 | 1.18 | 0.79 | 2.46 | 3.10 |
Ethyl caproate | 14 | Yang et al. (2022) | Apple peel, fruit | — | 0.83 | 33.54 | 25.08 | 7.94 | 15.21 | 10.67 |
Ethyl octanoate | 5 | Yang et al. (2022) | Fruit, fat | — | 38.24 | 145.01 | 316.70 | 259.29 | 230.70 | 144.09 |
Ethyl isovalerate | 3 | Rahayu et al. (2017) | Apple | — | — | 29.26 | 27.76 | 26.42 | 19.12 | 16.48 |
Ethyl heptanoate | 2 | Chen et al. (2019) | Fruity | — | — | 13.00 | 19.67 | 11.43 | — | — |
Benzaldehyde | 990 | Chen et al. (2019) | Almond | 0.08 | 2.10 | 0.46 | 0.16 | 0.16 | 0.14 | 0.09 |
Acetophenone | 65 | Chen et al. (2019) | Floral | 0.68 | 0.58 | 0.60 | 0.55 | 0.52 | 0.34 | — |
2-Octanone | 1100 | Yang et al. (2022) | Apple peel | 6.19 | 2.47 | 1.95 | 1.73 | 1.72 | 3.70 | 2.52 |
Benzene acetaldehyde | 4 | Yang et al. (2022) | Honey | — | 240.13 | 30.16 | 30.26 | 30.31 | 20.11 | 6.10 |
D-Limonene | 30 | Chen et al. (2019) | Citrus, mint | 1.04 | — | — | 0.48 | 0.01 | — | 0.56 |
2-Methoxyphenol | 9.5 | Chen et al. (2019) | Spicy, clove | 644.53 | 878.03 | 310.28 | 554.09 | 553.93 | 554.86 | 396.40 |
Volatile compounds . | Threshold(μg L−1) . | References . | Odour descriptor . | OAV values for different fermentation days . | ||||||
---|---|---|---|---|---|---|---|---|---|---|
0 . | 1 . | 3 . | 5 . | 9 . | 15 . | 27 . | ||||
β-Phenylethanol | 1380 | Pino & Queris (2011) | Rosy, honey | 0.16 | 3.83 | 11.47 | 19.19 | 20.00 | 23.73 | 26.31 |
Heptanol | 425 | Pino & Queris (2011) | Fruity | 2.25 | 1.81 | 0.59 | 0.51 | 0.51 | 0.46 | 0.41 |
1-Octanol | 200 | Pino & Queris (2011) | Fruity | 0.83 | 0.85 | 0.51 | 0.90 | 1.1 | 0.64 | 0.32 |
2-Nonanol | 70 | Fan et al. (2020) | Cucumber | 3.13 | 1.83 | — | — | — | — | — |
Benzyl alcohol | 900 | Chen et al. (2013) | Floral | 0 | 0.03 | 0.01 | 0.01 | 0.01 | — | — |
3-methylthiopropanol | 30 000 | Chen et al. (2013) | Alcoholic, nail polish | — | — | 0.02 | 0.04 | 0.05 | 0.07 | 0.08 |
Isobutanol | 40 000 | Yang et al. (2022) | Nail polish | — | — | 0 | 0.01 | 0.01 | 0.01 | 0.01 |
3-(Methylthio)-propanol | 1000 | Yang et al. (2022) | Meat | — | — | 0.06 | 0.02 | 0.07 | 0.08 | 0.09 |
3-Octanol | 393 | Wang et al. (2014a, 2014b) | Green, mushroom | — | — | 0.11 | 0.04 | 0.23 | 0.01 | — |
2,3-Butanediol | 30 | Yu et al. (2019) | — | — | — | 9.12 | 10.21 | 12.89 | 2.43 | |
Acetic acid | 200 000 | Yang et al. (2022) | Vinegar | 0 | 0 | 0 | 0 | 0 | 0 | 0 |
Hexanoic acid | 420 | Gómez-Míguez et al. (2007) | Cheese, acidic | 0.04 | 0.31 | 0.40 | 0.82 | 0.44 | 0.40 | 0.17 |
Octanoic acid | 500 | Gómez-Míguez et al. (2007) | Sweat, cheese | — | 0.29 | 0.54 | 0.33 | 0.31 | 0.92 | 0.90 |
Decanoic acid | 1000 | Gómez-Míguez et al. (2007) | Sweaty | — | 0.04 | 0.12 | 0.10 | 0.21 | 0.20 | 0.03 |
Ethyl palmitate | 1000 | Rahayu et al. (2017) | Oily | 0.10 | 0.14 | 0.59 | 0.24 | 0.59 | 1.75 | 2.25 |
Ethyl decanoate | 200 | Yang et al. (2022) | Fruity | 0.67 | 1.57 | 15.83 | 19.60 | 19.32 | 30.18 | 31.19 |
Ethyl myristate | 2000 | Yang et al. (2022) | Ether | 0.02 | 0.08 | 0.23 | 0.08 | 0.14 | 0.08 | 0.36 |
Ethyl benzoate | 580 | Yang et al. (2022) | Chamomile, fruity | 0.06 | 0.16 | 0.09 | 0.05 | 0.17 | 0.10 | 0.05 |
Ethyl laurate | 1500 | Yang et al. (2022) | Leaf | 0.07 | 0.10 | 0.79 | 0.45 | 1.27 | 0.18 | 1.56 |
Ethyl nonanoate | 200 | Yang et al. (2022) | Fruity | — | 0.28 | 1.35 | 1.18 | 0.79 | 2.46 | 3.10 |
Ethyl caproate | 14 | Yang et al. (2022) | Apple peel, fruit | — | 0.83 | 33.54 | 25.08 | 7.94 | 15.21 | 10.67 |
Ethyl octanoate | 5 | Yang et al. (2022) | Fruit, fat | — | 38.24 | 145.01 | 316.70 | 259.29 | 230.70 | 144.09 |
Ethyl isovalerate | 3 | Rahayu et al. (2017) | Apple | — | — | 29.26 | 27.76 | 26.42 | 19.12 | 16.48 |
Ethyl heptanoate | 2 | Chen et al. (2019) | Fruity | — | — | 13.00 | 19.67 | 11.43 | — | — |
Benzaldehyde | 990 | Chen et al. (2019) | Almond | 0.08 | 2.10 | 0.46 | 0.16 | 0.16 | 0.14 | 0.09 |
Acetophenone | 65 | Chen et al. (2019) | Floral | 0.68 | 0.58 | 0.60 | 0.55 | 0.52 | 0.34 | — |
2-Octanone | 1100 | Yang et al. (2022) | Apple peel | 6.19 | 2.47 | 1.95 | 1.73 | 1.72 | 3.70 | 2.52 |
Benzene acetaldehyde | 4 | Yang et al. (2022) | Honey | — | 240.13 | 30.16 | 30.26 | 30.31 | 20.11 | 6.10 |
D-Limonene | 30 | Chen et al. (2019) | Citrus, mint | 1.04 | — | — | 0.48 | 0.01 | — | 0.56 |
2-Methoxyphenol | 9.5 | Chen et al. (2019) | Spicy, clove | 644.53 | 878.03 | 310.28 | 554.09 | 553.93 | 554.86 | 396.40 |
The odour threshold in a 40% (v/v) water/ethanol solution; odour threshold in a 12% (v/v) water/ethanol solution for other compounds. Aroma descriptor was derived from the web pages: (http://www.leffingwell.com) (http://flavornet.org/flavornet.html) and (http://www.odour.org.uk/).
Analysis of the microbial community structure during the fermentation process
Dilution curve
The microbial community composition and succession during BLMJ fermentation were analysed using the Illumina MiSeq sequencing platform. The sequences were clustered at a 97% similarity to obtain different OTUs. Based on the Sobs index curve at the OTU level, which is also known as the dilution curve, we observed that with increasing sequencing depth, the dilution curves of bacteria (Fig. 2a) and fungi (Fig. 2b) at different fermentation stages tended to be gradually flattened, indicating a moderate increase in quantity. This suggests that the sequencing depth was adequate and able to reflect the diversity of most microorganisms in the samples (Xie et al., 2021).
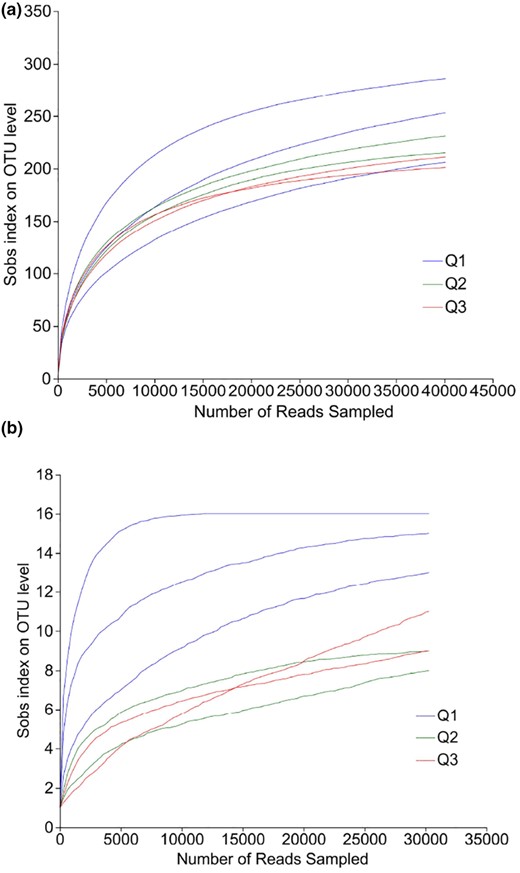
Dilution curves of bacteria (a) and fungi (b) at different fermentation stages of BLMJ. Q1: early fermentation (0–3 days); Q2: middle fermentation (4–9 days); Q3: late fermentation (10–29 days).
Analysis of bacterial and fungal alpha diversity during the fermentation process
At the similarity threshold of 97%, a total of 364 211 bacterial sequences and 274 208 fungal sequences were obtained from the seven samples (Table 3). The coverage of each sample exceeded 99.91%, indicating that the sequencing depth was appropriate and could reflect the microbial diversity in the samples. Table 3 shows that the diversity and richness of the bacterial community reached the peak at 24 h of fermentation and then gradually declined, followed by a slight increase at the late stage of fermentation. In contrast, the diversity of the fungal community showed consistent gradual decreases throughout the fermentation process. The Shannon index was relatively low at D_27; in contrast, the Simpson index, ACE index and CHAO1 index were high, indicating that at 27 days of fermentation, the fungal community in the samples had low diversity but high richness, but the underlying reasons require further investigation. Furthermore, bacteria had both much higher ACE index and CHAO1 index during BLMJ fermentation than fungi, indicating that the abundance and diversity of bacteria were significantly greater than those of fungi during the fermentation process.
Abundance and diversity parameters of bacterial and fungal communities during BLMJ fermentation
Days . | Sequence Count . | Shannon Index . | Simpson Index . | ACE Index . | CHAO1 Index . | Coverage % . | ||||||
---|---|---|---|---|---|---|---|---|---|---|---|---|
Bacteria . | Fungi . | Bacteria . | Fungi . | Bacteria . | Fungi . | Bacteria . | Fungi . | Bacteria . | Fungi . | Bacteria . | Fungi . | |
0 | 51 311 | 57 162 | 1.56 | 0.72 | 0.42 | 0.56 | 169.42 | 12.00 | 161.71 | 12.00 | 0.9991 | 0.9997 |
1 | 59 917 | 30 807 | 2.00 | 0.13 | 0.25 | 0.96 | 185.23 | 11.59 | 188.75 | 11.00 | 0.9991 | 0.9998 |
3 | 51 536 | 33 841 | 1.93 | 0.05 | 0.34 | 0.98 | 184.15 | 11.57 | 183.05 | 10.00 | 0.9995 | 0.9999 |
5 | 50 165 | 30 507 | 1.62 | 0.03 | 0.39 | 0.99 | 145.85 | 6.00 | 141.26 | 6.00 | 0.9995 | 0.9996 |
9 | 53 190 | 37 362 | 1.67 | 0.01 | 0.42 | 1.00 | 146.26 | 29.14 | 147.00 | 9.00 | 0.9995 | 0.9999 |
15 | 47 276 | 30 507 | 1.73 | 0.02 | 0.40 | 1.00 | 129.81 | 11.67 | 134.00 | 7.50 | 0.9996 | 0.9999 |
27 | 50 816 | 54 022 | 1.78 | 0.01 | 0.39 | 1.00 | 122.96 | 75.05 | 122.00 | 31.00 | 0.9997 | 0.9998 |
Total | 364 211 | 274 208 |
Days . | Sequence Count . | Shannon Index . | Simpson Index . | ACE Index . | CHAO1 Index . | Coverage % . | ||||||
---|---|---|---|---|---|---|---|---|---|---|---|---|
Bacteria . | Fungi . | Bacteria . | Fungi . | Bacteria . | Fungi . | Bacteria . | Fungi . | Bacteria . | Fungi . | Bacteria . | Fungi . | |
0 | 51 311 | 57 162 | 1.56 | 0.72 | 0.42 | 0.56 | 169.42 | 12.00 | 161.71 | 12.00 | 0.9991 | 0.9997 |
1 | 59 917 | 30 807 | 2.00 | 0.13 | 0.25 | 0.96 | 185.23 | 11.59 | 188.75 | 11.00 | 0.9991 | 0.9998 |
3 | 51 536 | 33 841 | 1.93 | 0.05 | 0.34 | 0.98 | 184.15 | 11.57 | 183.05 | 10.00 | 0.9995 | 0.9999 |
5 | 50 165 | 30 507 | 1.62 | 0.03 | 0.39 | 0.99 | 145.85 | 6.00 | 141.26 | 6.00 | 0.9995 | 0.9996 |
9 | 53 190 | 37 362 | 1.67 | 0.01 | 0.42 | 1.00 | 146.26 | 29.14 | 147.00 | 9.00 | 0.9995 | 0.9999 |
15 | 47 276 | 30 507 | 1.73 | 0.02 | 0.40 | 1.00 | 129.81 | 11.67 | 134.00 | 7.50 | 0.9996 | 0.9999 |
27 | 50 816 | 54 022 | 1.78 | 0.01 | 0.39 | 1.00 | 122.96 | 75.05 | 122.00 | 31.00 | 0.9997 | 0.9998 |
Total | 364 211 | 274 208 |
Abundance and diversity parameters of bacterial and fungal communities during BLMJ fermentation
Days . | Sequence Count . | Shannon Index . | Simpson Index . | ACE Index . | CHAO1 Index . | Coverage % . | ||||||
---|---|---|---|---|---|---|---|---|---|---|---|---|
Bacteria . | Fungi . | Bacteria . | Fungi . | Bacteria . | Fungi . | Bacteria . | Fungi . | Bacteria . | Fungi . | Bacteria . | Fungi . | |
0 | 51 311 | 57 162 | 1.56 | 0.72 | 0.42 | 0.56 | 169.42 | 12.00 | 161.71 | 12.00 | 0.9991 | 0.9997 |
1 | 59 917 | 30 807 | 2.00 | 0.13 | 0.25 | 0.96 | 185.23 | 11.59 | 188.75 | 11.00 | 0.9991 | 0.9998 |
3 | 51 536 | 33 841 | 1.93 | 0.05 | 0.34 | 0.98 | 184.15 | 11.57 | 183.05 | 10.00 | 0.9995 | 0.9999 |
5 | 50 165 | 30 507 | 1.62 | 0.03 | 0.39 | 0.99 | 145.85 | 6.00 | 141.26 | 6.00 | 0.9995 | 0.9996 |
9 | 53 190 | 37 362 | 1.67 | 0.01 | 0.42 | 1.00 | 146.26 | 29.14 | 147.00 | 9.00 | 0.9995 | 0.9999 |
15 | 47 276 | 30 507 | 1.73 | 0.02 | 0.40 | 1.00 | 129.81 | 11.67 | 134.00 | 7.50 | 0.9996 | 0.9999 |
27 | 50 816 | 54 022 | 1.78 | 0.01 | 0.39 | 1.00 | 122.96 | 75.05 | 122.00 | 31.00 | 0.9997 | 0.9998 |
Total | 364 211 | 274 208 |
Days . | Sequence Count . | Shannon Index . | Simpson Index . | ACE Index . | CHAO1 Index . | Coverage % . | ||||||
---|---|---|---|---|---|---|---|---|---|---|---|---|
Bacteria . | Fungi . | Bacteria . | Fungi . | Bacteria . | Fungi . | Bacteria . | Fungi . | Bacteria . | Fungi . | Bacteria . | Fungi . | |
0 | 51 311 | 57 162 | 1.56 | 0.72 | 0.42 | 0.56 | 169.42 | 12.00 | 161.71 | 12.00 | 0.9991 | 0.9997 |
1 | 59 917 | 30 807 | 2.00 | 0.13 | 0.25 | 0.96 | 185.23 | 11.59 | 188.75 | 11.00 | 0.9991 | 0.9998 |
3 | 51 536 | 33 841 | 1.93 | 0.05 | 0.34 | 0.98 | 184.15 | 11.57 | 183.05 | 10.00 | 0.9995 | 0.9999 |
5 | 50 165 | 30 507 | 1.62 | 0.03 | 0.39 | 0.99 | 145.85 | 6.00 | 141.26 | 6.00 | 0.9995 | 0.9996 |
9 | 53 190 | 37 362 | 1.67 | 0.01 | 0.42 | 1.00 | 146.26 | 29.14 | 147.00 | 9.00 | 0.9995 | 0.9999 |
15 | 47 276 | 30 507 | 1.73 | 0.02 | 0.40 | 1.00 | 129.81 | 11.67 | 134.00 | 7.50 | 0.9996 | 0.9999 |
27 | 50 816 | 54 022 | 1.78 | 0.01 | 0.39 | 1.00 | 122.96 | 75.05 | 122.00 | 31.00 | 0.9997 | 0.9998 |
Total | 364 211 | 274 208 |
Analysis of bacterial and fungal community dynamics during the fermentation process
Dynamic analysis of bacterial community during the fermentation process
With the Illumina MiSeq sequencing platform, a total of 364 211 sequencing reads with an average length of 425 bp were obtained. Based on a sequence similarity threshold of 97%, the seven groups of samples were classified into OTUs, resulting in the generation of 563 OTUs. According to the Venn diagram of bacterial communities at different fermentation stages, each sample exhibited its unique bacterial population. The D_0 stage had the largest number of unique OTUs (a total of 172), while the D_27 stage had the fewest unique OTUs (a total of 46). Throughout the entire BLMJ fermentation process, there were 169 shared OTUs at three fermentation stages, indicating a relatively high similarity in the composition of bacterial communities (Fig. 3a).
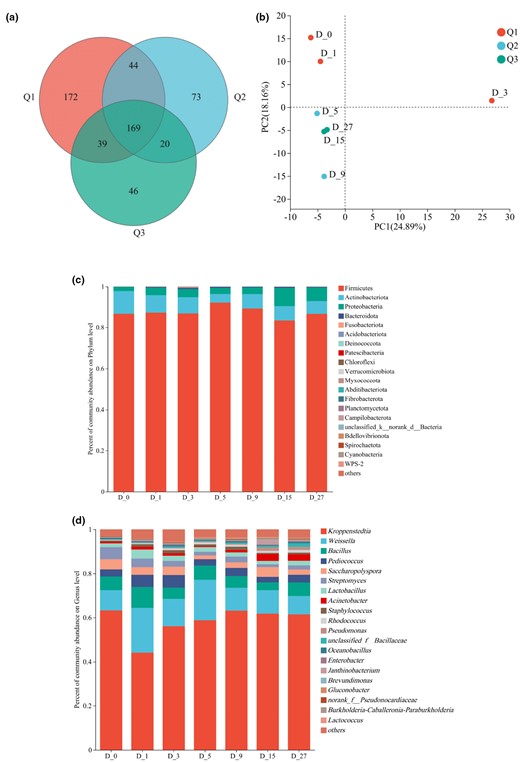
Venn diagram (a), PCA plot (b), phylum level (c) and genus level (d) bacterial community analysis of bacterial OTUs in different fermentation stages of BLMJ. Q1: early fermentation (0–3 days); Q2: middle fermentation (4–9 days); Q3: late fermentation (10–29 days).
Furthermore, PCA of the bacterial community in BLMJ samples at the OTU level also revealed distinct patterns of distribution (Fig. 3b). Specifically, the samples from the early fermentation stage exhibited a scattered distribution, while those from the later fermentation stage were more clustered. This phenomenon suggested that as the fermentation progressed, the bacterial community in the samples tended to be stabilised, which is consistent with the findings of Wang et al. regarding the dynamic changes in bacterial community during the traditional fermentation process of Shaoxing rice wine (Wang et al., 2014a; Wang et al., 2014b).
After alignment, the above 563 OTUs were classified into 21 phyla and 316 genera. The top five abundant phyla were Firmicutes, Actinobacteria, Proteobacteria, Bacteroidetes and Fusobacteria, among which Firmicutes (87.47%), Actinobacteria (7.46%) and Proteobacteria (4.50%) were dominant. Firmicutes emerged as the most dominant phylum during the fermentation of BLMJ, which is consistent with findings in previous studies of microbial communities associated with most rice wine fermentations (Wang et al., 2014a, 2014b; Liu et al., 2019, 2015). The analysis results of bacterial community structure at the phylum level during BLMJ fermentation are shown in Fig. 3c.
At the genus level, the top ten abundant genera comprised Kroppenstedtia, Weissella, Bacillus, Pediococcus, Saccharopolyspora, Streptomyces, Lactobacillus, Acinetobacter, Staphylococcus, Rhodococcus, Pseudomonas, Unclassified_f_Bacillaceae and Actinobacterium, which are somewhat different from the significant microorganisms identified in the fermentation processes of rice wine in the Dazhu, Sichuan and Xiaogan, Hubei regions (Tian et al., 2022). High abundance of Kroppenstedtia is often observed in high-temperature daqu used in sesame-flavoured liquor (Deng et al., 2020; Xie et al., 2020). The abundance of the Kroppenstedtia genus was high on D_0, decreased from D_0 to D_1, gradually increased from D_3 to D_9 (Fig. 3d) and then tended to be stabilised, reaching 61.42% on D_27. The abundance of Weissella and Bacillus (an average of 12.72% and 6.04%, respectively) showed relatively minor fluctuations throughout the fermentation process. During the entire fermentation process, the cumulative abundance of Kroppenstedtia, Weissella, Bacillus, Pediococcus and Saccharopolyspora accounted for an average of 87.33% of the total bacterial genera, indicating their dominance in the BLMJ fermentation process.
Dynamic analysis of fungal community during the fermentation process
Fungal community sequencing of the samples yielded a total of 274 208 sequencing reads with an average length of 431 bp. The coverage of each sample exceeded 99.4%, indicating reliable characterisation of the fungal community in the samples. Based on a sequence similarity threshold of 97%, the seven groups of samples were classified into sixteen OTUs representing two fungal phyla and twelve fungal genera. The fungal Venn diagram at the OTU level (Fig. 4a) reveals that there were no unique OTUs at the mid and late fermentation stages, indicating no significant changes in the fungal community at these fermentation stages. This observation is further supported by the PCA plot, which shows that except for a notable difference in fungal composition between D_0 and D_1, the fungal community in the entire fermentation system tended to be stable with minimal changes from D_3 (Fig. 4b).
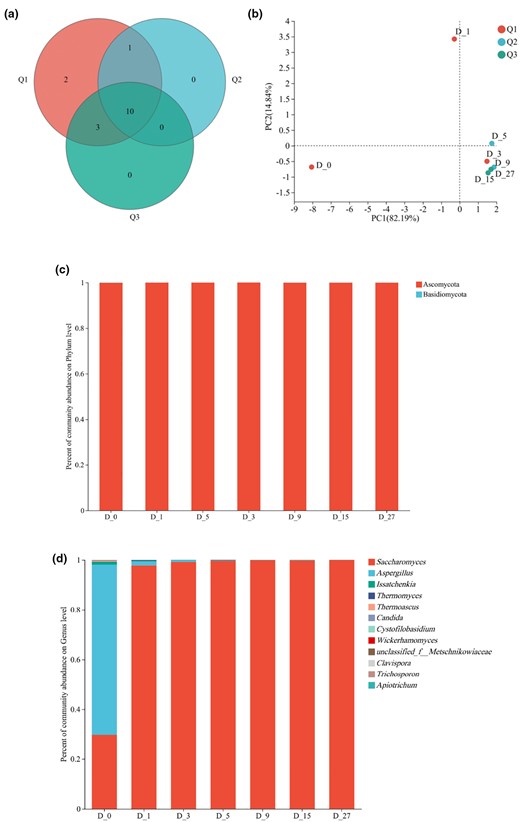
Venn diagram (a), PCA plot (b), phylum level (c) and genus level (d) fungal community analysis of fungal OTUs in different fermentation stages of BLMJ.
Sequencing alignment determined that BLMJ involves fungi from two phyla during the fermentation period: Ascomycota and Basidiomycota. Among them, Ascomycota was the dominant phylum accounting for 99.96%, while Basidiomycota had a relatively low proportion in all sample groups (Fig. 4c). At the genus level, a total of twelve fungal genera were detected throughout the fermentation process. As shown in Fig. 4d, three genera, Saccharomyces, Aspergillus and Issatchenkia, had abundance greater than 0.01% during fermentation. On D_0, the Aspergillus genus accounted for 68.35% of the fungal composition; however, its abundance rapidly declined to 1.58% on the first day of fermentation (D_1) and continued to decrease as the fermentation progressed to reach a negligible level by D_27, which is consistent with the results in previous studies (Ji et al., 2018). On D_0, the Saccharomyces genus accounted for 29.75% of the fungal composition, but the proportion rose sharply to 97.8% on D_1 and continued to increase until D_27 to reach 99.92%. Therefore, Saccharomyces is the predominant fungal genus during the fermentation of BLMJ. Overall, the fungal community structure in BLMJ showed minor changes throughout the fermentation process and the fungal diversity remained relatively low.
Correlation analysis between the microbial community structure and volatile compounds during the fermentation process of BLMJ
Volatile compounds, as an important class of the metabolic products of microorganisms, are closely related to metabolic activities of microorganisms. Here, we performed a correlation analysis between eighteen species of volatile compounds with OAV > 1 during the BLMJ fermentation process and the top twenty abundant bacterial genera and top 10 abundant fungal genera by calculating the Spearman correlation coefficient (r) (Ji et al., 2018). A correlation heatmap was constructed to visualise the relationships between fungi, bacteria and volatile compounds in BLMJ (Fig. 5). In Fig. 5, the X-axis is the volatile compounds and the Y-axis represents the microbial genera. Spearman's correlation coefficient is shown in different colours in Fig. 5, with red indicating positive correlations and blue representing negative correlations. In addition, a darker colour indicates a stronger correlation.
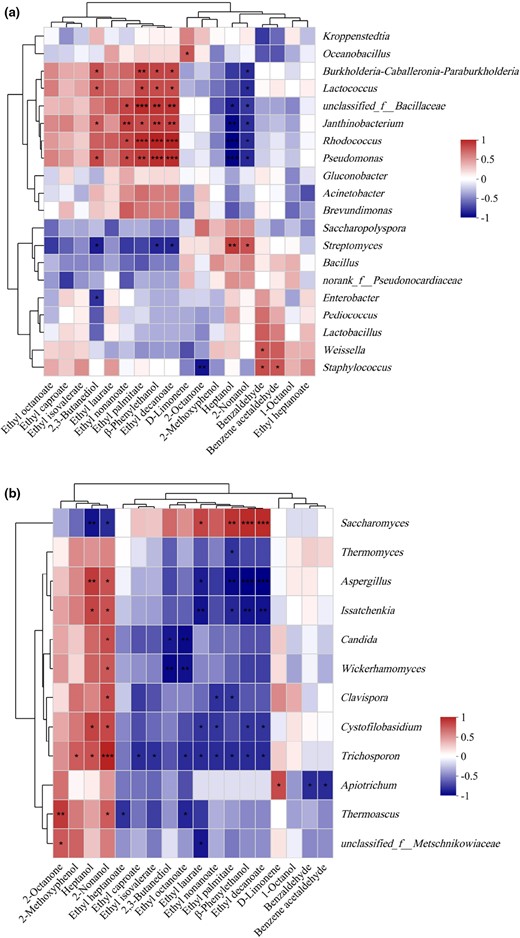
Heatmap analysis of correlations between volatile compounds and major bacterial (a) and fungal (b) genera. *P < 0.05; **P < 0.01; and ***P < 0.001.
As shown in Fig. 5a, there are eleven types of bacteria associated with the main volatile substances during the BLMJ fermentation process (P < 0.05), which are Oceanobacillus, Burkholderia-Caballeronia-Paraburkholderia, Lactococcus, Janthinobacterium, unclassified_f_Bacillaceae, Rhodococcus, Streptomyces, Weissella and Staphylococcus. Although Kroppenstedtia is the most common bacteria in the entire fermentation process of BLMJ, the correlation between Kroppenstedtia and the main volatile components in BLMJ is weak. According to Fig. 5b, there is a positive correlation between Saccharomyces and most of the main volatile substances in BLMJ. In addition, 2-octanone, 2-methoxyphenol, heptanol and 2-nonanol are positively correlated with most fungi.
There are significant positive correlations (P < 0.05) between Burkholderia-Caballeronia-Paraburkholderia, Rhodococcus, Lactococcus, Unclassified_f_Bacillaceae, Pseudomonas, Janthinobacterium with ethyl nonanoate, ethyl palmitate, 2,3-butanediol, β-phenylethanol and ethyl decanoate. However, Heptanol and 2-Nonanol are negatively correlated with these bacteria. Burkholderia-Caballeronia-Paraburkholderia accounts for about 0.1% of bacteria in the 0–5 days and during the mid-fermentation stage, its proportion increases to 0.5%, accounting for 0.37% in the finished BLMJ. Burkholderia-Caballeronia-Paraburkholderia are dominant bacteria in a variety of fermented foods, such as fermented Dongcai, Sufu, Doubanjiang (Yang et al., 2021; Wu et al., 2022; Jiang et al., 2023). A previous study has shown that Burkholderia–Caballeronia–Paraburkholderia play an important role in the flavour formation of fermented Sufu at the early fermentation stage (Wu et al., 2022). However, there have been few reports on how Burkholderia–Caballeronia–Paraburkholderia produce these flavour compounds, possibly due to its ability to produce lipase, which can decompose lipids and produce volatile compounds. The proportion of Lactococcus in the early and middle stages of BLMJ fermentation continues to increase, rising to 0.35% in the 9d and decreasing in the late stage of fermentation, accounting for 0.22% in the finished BLMJ. Lactococcus produces organic acids during its growth and metabolism, which react with alcohols in BLMJ to promote the generation of esters (Wang et al., 2023). During the fermentation process of BLMJ, the proportion of Rhodococcus is on the rise. In the early stage of fermentation, its proportion is about 0.4% and by 27 days of fermentation, its proportion reaches 1.22%. Some studies have shown that Rhodococcus is also a core bacterium in Huangjiu (Zhang et al., 2022). However, there has been limited specific research on the metabolic production of flavour compounds by Rhodococcus. Pseudomonas accounts for a relatively small proportion in the early and middle stages of BLMJ fermentation, with a sudden increase to around 1.49% in the later stages of fermentation. Pseudomonas can break down carbohydrate and produce lactic acid and is one of the dominant bacteria in the fermentation process of Longgang soy sauce and Zhenjiang vinegar (Haung et al., 2021; Yan et al., 2023). The proportion of Janthinobacterium is extremely low in the early and middle stages of fermentation, accounting for 1.92% in the late stage of fermentation and then decreasing in the finished BLMJ. Janthinobacterium can produce violet through metabolism, which has biological activities such as antibacterial, immune regulation and anti-tumour activities (Durán et al., 2016). However, there have been few reports on the volatile compounds produced by Janthinobacterium. The proportion of Unclassified_f_Bacillaceae in the fermentation process of BLMJ continues to increase, accounting for 1.63% in the finished BLMJ. Bacillaceae plays an important role in the brewing of Luzhou flavour Baijiu, producing flavour compounds or flavour precursors such as ethyl ester, 2,3-butanediol, tetracarboxylic compounds, pyrazine, etc. (Zheng et al., 2011; He et al., 2019).
Weissella is the second most abundant genus of bacteria in the BLMJ fermentation process. At D_0, it accounts for 9.13% and at the end of the initial fermentation stage, it accounts for 12.27%. In the middle stage of fermentation, Weissella accounted for 14.27% and in the late stage of fermentation, its proportion decreased to 8.29%. As shown in Fig. 5a, during the fermentation of BLMJ, Weissella was only positively correlated with Benzaldehide.
In the early stage of fermentation, the relative proportion of Staphylococcus gradually increases, reaching 1.38% on the 3d. At the end of fermentation, the relative proportion of Staphylococcus decreased to 0.77%. Staphylococcus is positively correlated with benzaldehyde and benzene acetaldehyde and negatively correlated with 2-octanone. The decrease of 2-octanone in the early and middle stages of fermentation may be related to the growth and reproduction of Staphylococcus. A previous study found that Staphylococcus is one of the main microorganisms that dominate salt, acid, amino nitrogen and carbohydrate metabolism during the fermentation process of Dongcai (Jiang et al., 2023).
Figure 5b presents the relationship between the main volatile compounds in BLMJ and fungal communities. Saccharomyces is positively correlated with various major volatile compounds in BLMJ and is the core fungus in BLMJ fermentation. Saccharomyces only accounts for 29.75% in 0 days and then rapidly proliferates, accounting for over 95% from 1 days to the end of fermentation. It has been widely used in brewing and bread making and can convert carbohydrates in raw materials into ethanol through glycolysis. The ester compounds in BLMJ are mainly produced by Saccharomyces through fermentation with their corresponding precursors by enzymatic ethanolysis with the aid of acyl-CoA (Swiegers & Pretorius, 2005). However, 2-octanone, 2-methoxyphenol, heptanol and 2-nonanol are positively correlated with most fungi in BLMJ and negatively correlated with Saccharomyces.
Since Rhodococcus, Burkholderia-Caballeronia-Paraburkholderia, Unclassified_f_Bacillaceae, Lactococcus, Pseudomonas, Janthinobacterium and Saccharomyces are positively correlated with various major aroma compounds in BLMJ and play essential roles in the formation of alcohols and esters, they may be core microorganisms in the fermentation process of BLMJ. However, it remains to be determined whether the above microorganisms can produce related volatile compounds.
Conclusions
In this study, a total of 64 volatile compounds were detected using the HS-SPME-GC–MS method in the BLMJ fermentation process, with esters and alcohols being the major volatile compounds (Table 2). According to the OAV analysis, eighteen volatile compounds are important aroma compounds in BLMJ, mainly including alcohols and esters (OAV > 1). The dominant bacterial phyla during the fermentation process were Firmicutes, Actinobacteria, Proteobacteria and Ascomycota. Correlation analysis revealed that the microbial genera with major contributions to core flavour compounds are Rhodococcus, Lactococcus, Pseudomonas, Unclassified_f_Bacillaceae, Burkholderia-Caballeronia-Paraburkholderia, Janthinobacterium and Saccharomyces. This study identified the significant flavour-contributing microorganisms during the fermentation process of BLMJ, laying a foundation for subsequent flavour improvement of rice wine.
Acknowledgments
The author thanks Beizong Huangjiu Brewing Co., Ltd. for their contributions in conducting this study.
Author contributions
Yang Liu: Writing – original draft; writing – review and editing; data curation. Xiaohui Chen: Methodology; investigation; validation. Mingyuan Li: Software; supervision. Cunhong Wei: Visualization; formal analysis. Mingchun Xiao: Software; investigation. Jingjing Xiao: Formal analysis; validation; data curation. Yaqi Zhang: Validation; visualization; investigation. Xuedong Chang: Conceptualization; supervision; funding acquisition; project administration; resources. Jing Zou: Supervision; writing – review and editing; funding acquisition; project administration; formal analysis.
Funding statement
This research was funded by the National Natural Science Foundation of China (U22A20545); Kangxian Wild Chestnut Preservation and Processing Innovation Technology Project of Gansu province (144001JH621224031); Hebei Province Key Research and Development Plan Project Key Common Technology Public Relations Special Project for High Quality Agricultural Development (19227119D), and Hebei Agricultural Science and Technology Park (Base) Construction Special Project (20537101D).
Conflict of interest statement
The authors declare that there are no conflicts of interest.
Data availability statement
Data are contained within the article and Table S1.
References
In this article, the author explains the changes in volatile compounds during the fermentation process of Huangjiu, which has certain reference value for the sources of aroma substances in BLMJ.
In this article, the author explains the metabolic pathways by which yeast produces esters, and citing this literature is of great significance for the pathway by which brewing yeast produces esters in BLMJ.
In this article, the author explained the relationship between Lactococcus and the production of esters during the fermentation of pickled Chinese cabbage, which has some reference significance for the production of esters in this BLMJ.
In this article, the author elaborates on various factors that affect the volatile compounds in rice wine. By citing this article, readers can have a more comprehensive understanding of the volatile compounds in BLMJ.
In this article, the author studied the effects of different types of lactic acid bacteria on the flavour of rice wine, which has important reference value for the types of lactic acid bacteria in BLMJ and their impact on the flavour of BLMJ.