-
PDF
- Split View
-
Views
-
Cite
Cite
Hongxia Wang, Fuyuan Ding, Liang Ma, Yuhao Zhang, Recent advances in gelatine and chitosan complex material for practical food preservation application, International Journal of Food Science and Technology, Volume 56, Issue 12, December 2021, Pages 6279–6300, https://doi.org/10.1111/ijfs.15340
- Share Icon Share
Abstract
Both gelatine and chitosan are safe, odourless, edible, biodegradable, biocompatible, cost-efficient and commercially available biopolymers, which promotes the possibility of forming complex. Many factors, including origin, molecular weight, deacetylation degree, pH, salts, concentration, temperature, active materials, modifiers, etc., could impose effect on the process of producing gelatine-chitosan complex. The formed gelatine-chitosan complex has excellent physicochemical properties such as antibacterial property and antioxidant property, and could effectively preserve practical food (such as meat, vegetables and fruits). This article comprehensively reviews recent advances in factors affecting the complex forming process and practical food preservation application of gelatine-chitosan complex. It aims to provide an overview of the value of gelatine-chitosan complex as an effective preservation alternative in food technology.

Introduction
As popular natural by-product biomaterials containing multi-biofunctions, gelatine and chitosan are widely researched nowadays and act as a potential preservation alternative with excellent bioactive preservation properties in food fields. Gelatine, present in different parts of both land and marine animals, is a denatured fibrous protein derived from collagen by partial hydrolysis, containing repeating sequences glycine, proline, hydroxyproline, etc. As a traditional water-soluble biopolymer with a triple helix, it has been a commonly applied protein owing to its wide range of characteristics, such as abundance, availability, biodegradability, non-toxicity, biocompatibility, high water-binding capacity, emulsifying properties, barrier ability and low cost (Otálora, Wilches-Torres, & Gómez Castaño, 2020; Hosseini, Soofi, & Rezaei, 2021; Zhang et al., 2021), making it more appropriate for using in food industry.
Chitosan, a natural cationic linear polysaccharide composed of N-acetyl-d-Glucosamine groups linked by O-glucosidic bonds β(1 → 4), is an unbranched polymer obtained from deacetylation of chitin. It originates from natural sources such as the exoskeletons of crustaceans and arthropods (such as prawn, shrimp, crab shells) (Gamiz-Gonzalez, Correia, Lanceros-Mendez, Sencadas, Gomez Ribelles, & Vidaurre, 2017; Roy, Salaun, Giraud, Ferri, & Guan, 2017; Rezaee, Askari, EmamDjomeh, & Salami, 2018; Abirami et al., 2021), mushroom (Janesch, Jones, Bacher, Kontturi, Bismarck, & Mautner, 2020), silkworm chrysalis (Simionato, Villalobos, Bulla, Coró, & Garcia, 2014) and even microorganisms (Hassainia, Satha, & Boufi, 2018; Abirami et al., 2021). It is the most abundant biopolymer after cellulose and is extensively considered as a renewable preservative material, with safety, inherent antimicrobial properties, compatibility, antioxidative activities and edibility. When combining these two above materials with high intermolecular binding potential, there is often the synergistic effect of advantages (such as antioxidant properties in gelatine and antimicrobial properties in chitosan) and the minimising effect of disadvantages (such as none antimicrobial properties, poor water barrier properties and strong tendency to swell of gelatine) (Zhang et al., 2021), which is more effective for food preservation than each component alone (Gómez-Estaca, Gómez-Guillén, Fernández-Martín, & Montero, 2011; Amjadi, Nazari, Alizadeh, & Hamishehkar, 2020). Many factors would affect the formation of gelatine-chitosan complex, such as gelatine origin, molecular weight (MW), degree of deacetylation of chitosan (DD), pH, salts, concentration, temperature, active materials and modifiers.
As far as we know, there is no review article reporting factors affecting gelatine-chitosan complex and practical food preservation. Synthetic preservatives in food products are strictly controlled due to potential side effects (Vafania, Fathi, & Soleimanian-Zad, 2019). Many consumers, therefore, prefer natural preservation like gelatine-chitosan complex. This review aims at discussing factors affecting gelatine-chitosan complex and summarizing practical food preservation application (Fig. 1), which has a promising future due to the consumer's preference for safe and high-quality food.
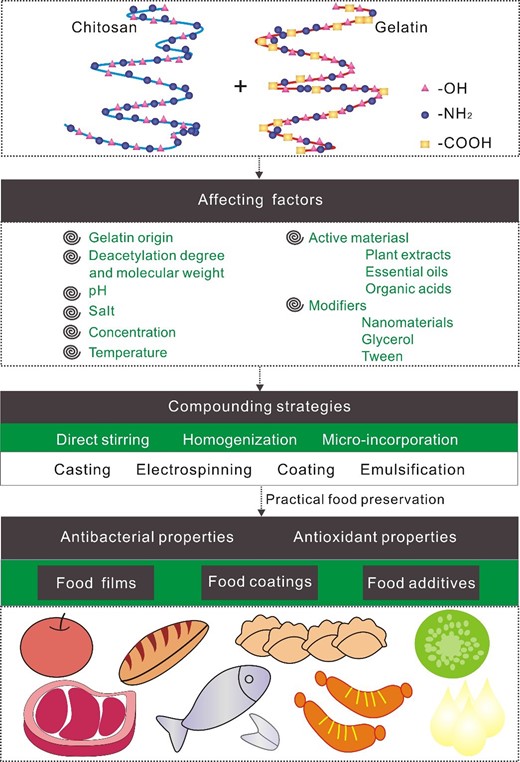
Affecting factors and food preservation application of gelatine-chitosan complex.
Affecting factors
The interactions between gelatine and chitosan affect their miscibility, and as a result they affect structure and properties of the complex. Electrostatic interactions mainly occur between COO− of gelatine and NH3+ of chitosan, and hydrogen bonds are formed within and between polymer chains involving CO, OH, NH2, etc. These interactions can facilitate formation of multiple complexes, which are influenced by many factors. As for the basic matrix formed by gelatine and chitosan, the factors including gelatine origin, MW, DD, pH, salts, concentration and temperature could impose great effects on (as being illustrated in Fig. 2): (1) the interaction between gelatine and chitosan, e.g., the changes in electrostatic interaction or/and hydrogen bonds, (2) the complex structure, e.g., compact or loose, (3) the affinity and compatibility of the complex, e.g., good miscibility or poor miscibility, and (4) the solubility of gelatine and chitosan, and therefore the stability.
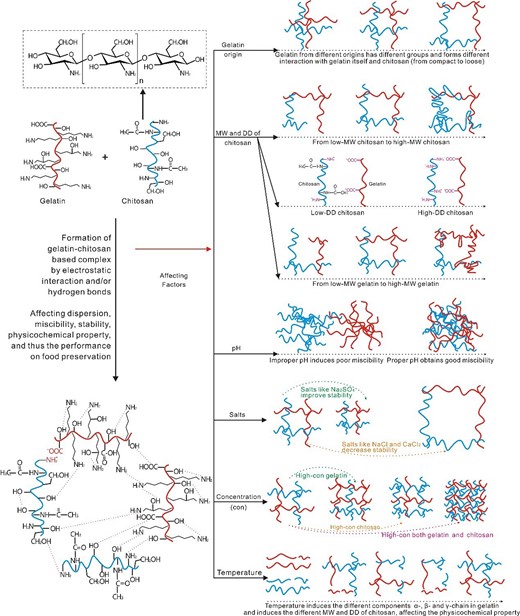
Factors (gelatine origin, MW, DD, pH, salts and concentration) influencing the gelatine-chitosan complex formation process.
Effect of gelatine origin
Gelatine is a denatured form of collagen but differs from collagen in its chemical composition (Sun et al., 2021), with origins from bone and skin collagen of bovine, porcine, rabbit, camel, fish, etc. (Ghaderi, Hosseini, Keyvani, & Gómez-Guillén, 2019; Benbettaieb, Mahfoudh, Moundanga, Brachais, Chambin, & Debeaufort, 2020; Dai et al., 2020; Huang, Tu, Sha, Wang, Hu, & Hu, 2020; Sezer, Okur, Oztop, & Alpas, 2020; Hosseini et al., 2021). Gelatine origin could lead to different number of triple-helixes structure, solubility, interactions nature, variable contents of proteins, peptides and nucleic acids (Duconseille, Astruc, Quintana, Meersman, & Sante-Lhoutellier, 2015).
Among origins of commercial gelatine, mammalian pigskin accounts for 46.0%, cowhide 29.4% and pig and bovine bones 23.1% (Huang et al., 2020). Pork gelatine is problematic for Muslim consumers owing to Halal considerations around gelatine, and Muslims are not allowed to consume any porcine derived food products according to the Islamic rules; therefore, Halal gelatine is crucial as Muslims are predicted to account for 31% of the world population by 2060 (Rohman, Windarsih, Erwanto, & Zakaria, 2020; Yuswan et al., 2021). The source of gelatine is not only an issue for Muslims but also of concern to followers of Judaism (kosher) and Hinduism (vegetarian). Jewish people are prohibited from consuming porcine meat and certain denominations of Christianity also prefer not to use porcine products, while Hindus are prohibited from consuming bovine meat. Currently, other sources of gelatine are widely researched, including Halal ovine gelatine (Lv, Huang, Ding, Xiao, Zhang, & Xiong, 2019), camel skin gelatine (Abuibaid, AlSenaani, Hamed, Kittiphattanabawon, & Maqsood, 2020), fish gelatine (Krishna, Nindo, & Min, 2012; Altan Kamer, Palabiyik, Işık, Akyuz, Demirci, & Gumus, 2019) and other aquatic animals (Lin, Regenstein, Lv, Lu, & Jiang, 2017). Especially, fish gelatine, is an encouraging alternative for mammalian-based gelatine, since it does not have any safety or religion issue (Ghaderi et al., 2019; Kan, Liu, Yong, Liu, Qin, & Liu, 2019; Hu, Yao, Qin, Yong, & Liu, 2020; Lin et al., 2020). Some fish-skin gelatine rich in polypeptide chains, with 31.6% of glycine, 10% of proline and 7.9% of hydroxyproline, had strong film forming capacity from solutions with low gelatine concentrations (Zhang, Dutilleul, Li, & Simpson, 2019).
The interactions between gelatine and chitosan were found to be stronger in the blends made with fish-skin gelatine than in the blends made with bovine-hide gelatine (Gómez-Estaca et al., 2011). Fish gelatine-chitosan complex was more water resistant (˜18% water solubility for tuna vs. 30% for bovine) and more deformable (˜68% breaking deformation for tuna vs. 11% for bovine) than the bovine gelatine-chitosan complex. The water solubility could be attributable to both the amino acid profile and the MW distribution, and the breaking deformation differences are attributed to different imino acid contents, giving bovine-hide gelatine a higher degree of molecular rigidity. This molecular interaction also resulted in the alternations in breaking strength, in which fish gelatine-chitosan shows the higher breaking strength (20.04 N) than bovine gelatine-chitosan composite. These excellent properties would potentially protect food from the water-induced destruction and spoilage, and guard food from the external forces or stresses to keep the integrity and maintain quality of foods, thus promoting the utilisation as self-standing packaging material (Gómez-Estaca et al., 2011).
Gelatine could be produced from many sources. Aiming at precise market and special consumers (like halal concerns), the alternative to pork gelatine and bovine gelatine is emerging and is broadening, such as camel, ovine and fish gelatine. Overall, gelatine origin would affect the components and inherent characteristics of gelatine, and would form different interaction with chitosan. Therefore, to obtain gelatine-chitosan complex with the aims at special consumers or the special performance, it is important to choose proper gelatine from different origins for further application.
Effect of MW and DD
MW is defined as the number of sugar units per polymer molecule in chitosan, and DD of chitosan determines the ratio between d-glucosamine and N-acetyl -d-glucosamine units in polymer chains (Hamdi, Nasri, Amor, Li, Gargouri, & Nasri, 2020). Chitosan, though it can be dissolved in aqueous solutions of numerous organic acids, is inherently water-insoluble as regards their large MW and strong intramolecular hydrogen bonding, severely restricting the applications. High-MW chitosan is soluble in acids with higher viscosity and low-MW chitosan is more soluble in neutral aqueous solvents with low viscosity, due to the chain length and entanglement (Tsao, Chang, Lin, Wu, Han, & Hsieh, 2011). Crystal size, crystallinity, morphological character, elasticity, tear strength, biofunctional activities of chitosan are also dependent on the MW (Bakshi, Selvakumar, Kadirvelu, & Kumar, 2020). High MW of chitosan promotes the excellent transparency, elongation at break, tensile strength, smooth morphology, denser structure and antibacterial property; furthermore, deacetylation process of chitosan brings about a change in MW (Y. Liu et al., 2020).
pKa is of vital importance for processing chitosan. DD over 50% is considered to be chitosan, and below 50% is called chitin. DD determines the content of amine groups (NH2) on the chitosan chain, and the increase in DD makes the charged groups of chitosan in the dilute acid solution to increase due to the protonation of the amine, leading to the increase of positive charge density (Hamdi et al., 2020). pKa is highly dependent on DD due to the calculation (-NH2+H2O ⇔ -NH3++OH−, Ka = [-NH2][H3O−]/[NH3+], and pKa = −log Ka). The soluble-insoluble transition occurs at a pKa around 6.2–6.5 (Gatto, Ochi, Yoshida, & da Silva, 2019), therefore indicating the important effect of DD on pKa and solubility. With increase in DD, the chitosan chain becomes more flexible, thus tending to form a random coil with more intramolecular hydrogen bonds within the chain, resulting in the chitosan chains being less entangled and more ellipsoid in shape (Bakshi et al., 2020). Mechanical properties are generally weaker than those of low-DD microsphere and the low-DD chitosan chain are more extended and have stronger intermolecular interactions, which make the chains more entangled. As for high-MW chitosan and low-MW chitosan, though their chemical structures are the same, the amine density of low-MW is greater than that of high-MW chitosan (Tsao et al., 2011), conducting high positively charged densities, thus leading to the change of pKa values. Thereby, MW and DD could be considered as extremely important chitosan’s structural traits, modulating its macromolecular chains properties (Gamiz-Gonzalez et al., 2017; Hamdi et al., 2020), which is closely associated with chitosan applicability (Gatto et al., 2019; Bakshi et al., 2020).
As for the antibacterial activity of chitosan on relevant food poisoning microorganism, it could be attributed to that (1) NH3+ groups of chitosan interact with negatively charged carboxylic acid groups of the macromolecules of the bacterial cell wall and cause distortion, deformation and microbial death (Bi et al., 2021), illustrating the importance of high DD. (2) For the same DD, the effectiveness of chitosan in disrupting pre-formed biofilm (post-treatment) increases with decreasing its MW, which is due to that at lower MW, chitosan infiltration and adsorption at the interface between the solid surface and the adherent bacteria forming a biofilm are easier and more pronounced (Hamdi et al., 2020). (3) Chitosan alters cell permeability of both the outer and inner membranes, thus leaking the intracellular of the bacteria with the limited growth (Handayasari, Suyatma, & Nurjanah, 2019; Kumorek et al., 2020). (4) Chitosan causes the cell aggregation of most bacterial species and membrane damages, resulting in cell death (Liu et al., 2020). (5) Chitosan can penetrate nuclei, bind DNA, inhibit its replication ability and lead to significant changes in the gene expression (Kumorek et al., 2020). (6) Chitosan can also chelate internal nutrients and essential metals that are important for microbes (Wang, Qian, & Ding, 2018). The distinctly discernible antimicrobial effects of chitosan against bacteria were often also maintained in the gelatine-chitosan complex (Gómez-Estaca et al., 2011).
As for gelatine, MW distribution depends on origin as well as the gelatine manufacturing process, which causes molecular degradation. Fish gelatines have slightly lower MWs than those present in porcine gelatine (Duconseille et al., 2015). The structure is also influenced by MW distribution of gelatine chains, as α-chains have MW around 110 000 g mole−1 that could form β and γ chains with MWs of 200 000 g mole−1 and 300 000 g mole−1, respectively (Duconseille et al., 2015). Type A gelatine has pI (isoelectric point) around 6–9.5 from collagen by acid hydrolysis and type B gelatine has pI around 4.5–5.6 from collagen by alkaline treatment (Marangon, Martins, Leite, Santos, Nitschke, & Plepis, 2017; Mwangi, Lim, Low, Tey, & Chan, 2020). Acid treatment is most suitable for the less covalently crosslinked collagens in pig or fish skins, while alkali pretreatment is most suitable for the more complex collagens in bovine skins (Zhang, Xu, Zhang, Wang, Lorenzo, & Zhong, 2020). α- and β-chains of gelatine were significantly greater in type A gelatine than in type B gelatine lacking in amide groups (Islam et al., 2021; Xiao et al., 2021). Type A gelatine has a positive charge over a wider range of pH values, among which low-MW gelatine has lower pI value around 6 and high-MW gelatine has higher pI value around 6.5 (Surh, Decker, & McClements, 2006), due to that high MW is correlated with the higher contents of amino acids involved in cross-link formation (Duconseille et al., 2015). Moreover, pI value decreases with the decrease in MW, probably due to deamidation of glutamine and asparagine to glutamic acid and aspartic acid in low MW gelatine (Tan, Karim, Uthumporn, & Ghazali, 2020). When the pH is lower or higher than pI, the net charges of protein molecules are greater and the solubility is increased by the repulsion forces between chains. In contrast, when the total net charges of protein molecules are zero, the hydrophobic–hydrophobic interaction increases, thereby leading to the precipitation and aggregation of gelatine at the pI (Bkhairia, Mhamdi, Jridi, & Nasri, 2016).
Therefore, gelatine and chitosan could form complexes in the oppositely charged pH region via electrostatic complexation, or in the same charged pH region via hydrogen bonds (Wang & Heuzey, 2016). MW and DD of chitosan are among key factors, which affect its performance as a complex matrix containing gelatine (Atay, Fabra, Martínez-Sanz, Gomez-Mascaraque, Altan, & Lopez-Rubio, 2018). The chitosan DD influences the intermolecular and intramolecular interactions of this polymer and, therefore, also implicates in changes of its properties including antimicrobial activity, water vapour permeability, water solubility and swelling (Rodrigues, Marangon, Martins, & Plepis, 2021). The effectiveness of chitosan in preventing microbial adhesion was strongly influenced with the lowest inhibition values for lower DD and lower MW (Hamdi et al., 2020). The interactions between gelatine and chitosan were stronger in the blends made with chitosan of higher MW or higher DD than the blends made with lower MW or DD (Liu, Ge, Lu, Dong, Zhao, & Zeng, 2012). Higher MW and higher DD of chitosan could also promote gelatine-chitosan solutions to have higher modulus due to more reactive groups in chitosan. Moreover, gelatine that composited with chitosan of higher MW or higher DD, had higher tensile strengths (72.4 MPa for 550 kDa vs. 62.2 MPa for 200 kDa; 63.2 MPa for 93.6% DD vs. 38.0 MPa for 76.5% DD) and higher glass transition temperatures (130 °C for 550 kDa vs. 108 °C for 200 kDa; 108 °C for 93.6% DD vs. 103 °C for 76.5% DD) (Liu et al., 2012). However, on the other hand, the intrinsic viscosity of chitosan solutions (especially the ones from intermediate and high MW) limited its content in the blend compositions (Atay et al., 2018), as it greatly affected the rheological properties of the solutions, having an impact on their sprayability for gelatine-chitosan based complex.
Overall, high DD and high MW of chitosan could be adopted for gelatine-chitosan complex obtaining high antibacterial properties and high mechanical properties. For more other functional properties of gelatine-chitosan complex, appropriate MW and DD are necessary to be detailly designed.
Effect of pH
pH treatment/adjustment was reported to have important influence on film properties, including residuals distribution, clusters or non-uniform surfaces, structural properties, optical performance, etc. (Kumrueng, Sawanthai, Tubtimtae, & Ponhan, 2020; Leite, Moreira, Mattoso, & Bras, 2020). Especially for biopolymers, the effect of acidic pH on the physicochemical properties is important for the actual production of biopolymers film (Xu, Liu, Wang, Goff, & Zhong, 2020). For example, the optical density of the biopolymer systems increases with the increase of the pH value (Vshivkov, Soliman, Kluzhin, & Kapitanov, 2019), or the stability of some films varies with the pH value. Some films assembled at pH 5 were unstable at pH 8.5, and all films disassembled at pH 7.4 (Kumorek et al., 2020). pH alteration could also change properties of chitosan and gelatine, respectively, which further adjust the complex film’s performance.
At pH < pI, the gelatine solution showed a net positive charge, which was attributed to the protonation of the amino groups (-NH3+), while at pH > pI the solution showed a net negative charge, due to ionisation of the carboxyl groups (-COO−) (Leite et al., 2020). At pI, the swelling rate, sedimentation and viscosity of gelatine are the lowest, thereby rendering it difficult to form complex with chitosan (Ma et al., 2020). In acidic environment (pH < pKa), the amino groups of chitosan are protonated and their positive charges can interact with polyanions such as gelatine at a pH lower than its isoelectric point (Benbettaïeb, Chambin, Karbowiak, & Debeaufort, 2016). Benbettaieb et al found that preparation at a pH = 5.5 between the pI of gelatine (pI = 4.5) and pKa of chitosan (pKa = 6.2–6.5) permits to generate the maximum amount of electrostatic interactions (Benbettaieb et al., 2020). Another group believed that gelatine containing amino acids with positive (amine) and negative (carboxyl) ionisable groups behaved as a polyampholyte, while chitosan was a copolymer of glucosamine (containing ionisable amine groups) and n-acetyl glucosamine, and therefore behaved as a polyelectrolyte (Pinto Ramos, Sarjinsky, Alizadehgiashi, Mobus, & Kumacheva, 2019). After blending, the formation of polyelectrolyte complexes occurred through electrostatic interaction, thus forming the complex.
As mentioned above, pH should be preferably about 4.5–6.5 to favour electrostatic interaction between gelatine (type B) and chitosan, or facilitate the hydrogen bonds between gelatine (type A) or chitosan, thus helping reach the desirable complex. During large-scale production, pH should be precisely considered for optimal performance.
Effect of salts
Ions (e.g., Na+, Li+, Ca2+, Mg2+, Al3+, Cl− and SO42−) play crucial roles in various application environment, which also impose great impact on the properties and performance of materials (Ding, Chen, Sui, & Wang, 2010). The binding of Ca2+ ions onto the dimyristoylphosphatidylcholine headgroups could lead to charging of the monolayers and electrical double-layer repulsion in the film. There is also some indication for a weak binding of Na+ ions (Toca-Herrera, ller, Krustev, Exerowa, & Mo¨hwald, 1998). It is interesting to find that many positive and negative ions could form interaction with the hydroxyl groups of biopolymer chains (Jiang, Li, Luo, Zhao, & Hou, 2016), and the interaction between inorganic salts and biopolymer would further influence recrystallization and crystallinity. MgCl2·H2O and CaCl2 could even act as suitable plasticiser for biopolymers based films (Jiang et al., 2016).
When a salt is added to a water bath, the equilibrium degree of swelling of a polyelectrolyte is strongly affected, due to the condensation of mobile counter-ions around charged functional groups which results in screening effect (Drozdov & deClaville Christiansen, 2015). Formula for ionisation degree of chains α = αmaxKa/[k(Ka/k + b*n+bathexp(-φ))] (αmax, welling degree; Ka, acid dissociation constant; k, water molarity; b, a decrease in ionization degree driven by ion-pairs; n+bath, positive ions fraction; φ is dimensionless electrostatic potential), accounts for screening of functional groups by surrounding segments and formation of ion-pairs (Drozdov, Sanporean, & deClaville Christiansen, 2016). Types of anions (e.g., F−, Cl−, Br− and I−), cations (Na+, K+ and Li+) and biopolymer (polyampholyte and polyelectrolyte) matter and have different screen effect on the electrostatic attractive forces, resulting in the various behaviours (Manning, 1969; Kudaibergenov, 1999; Lee & Chiu, 2002; Gao, Gawel, & Stokke, 2014), including swelling, collapsing and stability; high ionic strengths could largely screened charges (Wielema & Engberts, 1990; Qu et al., 2021). Salt screens the charge repulsion on large scales that extends the chain of polyelectrolyte, and only at higher salt concentrations the charge attraction that compacts the polyampholyte locally, is screened, causing the chain to swell (Dobrynin, Colby, & Rubinstein, 2004). Nonspecific, purely electrostatic charging and salt screening effects are included nonlinearly in the electrostatic energy (ΔΔGel(α;cs) = ΔP(α;cs)/ρg; ΔP(α;cs), net osmotic pressure inside the globule; ρg, a mean monomer number density) (Heyda, Soll, Yuan, & Dzubiella, 2014). The presence of the screening effect by salts on the attractive interactions in polyampholyte gelatine with the addition of salt could impose great effect on the complex properties (Qiao & Cao, 2013).
As for films, gelatine displayed a polyampholyte behaviour in which swelling and dissolution were enhanced in salt solutions due to the weaker ionic cross-linking between the charged amine and carboxyl groups and attraction between COO- and NH3+ groups of gelatine being screened in NaCl solutions, resulting in expansion of polymer network (Pinto Ramos et al., 2019). The swelling and dissolution of the chitosan films was reduced with increasing NaCl concentration due to the polyelectrolyte behaviour of this biopolymer, in which ionic repulsion between NH3+ groups of chitosan was screened, resulting in contraction of polymer network. On another hand, gelatine-chitosan complex films followed the behaviour of gelatine, due to the polyampholyte behaviour dominating in the gelatine-chitosan complex in NaCl solutions (Pinto Ramos et al., 2019). A lower degree of swelling than that for the gelatine films in water was attributed to the formation of ionic cross-links between the amine groups of chitosan and the excess deprotonated carboxyl groups of gelatine. Agitation resulted in an increase in the rate of dissolution, as it reduced film integrity and provided convective current of the dissolution medium, thus enhancing transport properties. Furthermore, the release of small ionic and zwitter-ionic molecules from the films was enhanced in NaCl solutions due to the screened attraction between these molecules and the polymer matrix (Pinto Ramos et al., 2019).
Ionic solutions could influence polyampholyte behaviour of gelatine, polyelectrolyte behaviour of chitosan and therefore the properties of gelatine-chitosan complex, finally affecting the application environment, which should be studied comprehensively for food preservation with high effectiveness.
Effect of concentration
Concentrations of gelatine and chitosan determine the amount of their active groups, functional groups and special structure like helices in the complex system, and further influence the electrostatic interactions and hydrogen bonds among them, leading to the differently structured matrix of gelatine-chitosan complex.
Gelatine concentration plays an important role (Ghaderi et al., 2019) and low-content gelatine-chitosan solution was more sensitive to shear rate and had higher viscosity (Liu et al., 2020). With increasing gelatine content (0%–50%), water vapour permeability of the complex increased from 0.785 to 0.818 g mm/kPa h m2, which was assumed that gelatine reduced intermolecular hydrogen bonds between chitosan and poly(vinyl alcohol) resulting in more surface polar groups exposed to water vapour, whereas water solubility was reduced probably due to the formation of new hydrogen bonds among molecules, reducing the number of free hydroxyl groups and limiting polymer molecules from binding to water molecules via hydroxyl groups (Ghaderi et al., 2019). Furthermore, the increasing gelatine improved ultraviolet–visible-light barrier of the complex with the transmission of ultraviolet–visible-light decreasing from 40.78% to 19.66%, which may be attributed to the high content of aromatic amino acids such as tyrosine and tryptophan in the protein-based structure and, in a less extent, phenylalanine and disulphide bonds, that are able to absorb radiation (Mohammadi et al., 2018; Ghaderi et al., 2019; Cao et al., 2020). The addition of chitosan (from 1% to 100%) into gelatine lead to conformational changes in the gelatine polypeptide chains and subsequent the decreases in the random coils, single helices and disordered structures, and significantly increased elongation at break (from 3.52% to 39.821%) of the gelatine-chitosan complex (Mohammadi et al., 2018). The increase of chitosan content (0%–9%) in the gelatine-chitosan based complex promoted a further decrease of bacterial growth (from ˜4 to ˜3.4 log CFU mL−1) (Uranga, Puertas, Etxabide, Dueñas, Guerrero, & de la Caba, 2019), and even enhanced food preservation up to 14 days (with no fungal growth being observed on the packed seeds) (Noorbakhsh-Soltani, Zerafat, & Sabbaghi, 2018).
As discussion above, gelatine concentration and chitosan concentrations in gelatine-chitosan complex matter greatly, influencing the formation of the structure and therefore the properties. Different foods have different requirements for preservative protection, such as mechanical property, UV light barrier property or antibacterial activity, and thus specific concentration of gelatine and chitosan should be accurately designed for achieving the preservation purposes.
Effect of temperature
There are many reports indicating temperature affecting the biopolymers processing in food fields. Temperature could tune interaction sites (Mansson, Peng, Crassous, & Schurtenberger, 2020), alter motion of particles, change solution viscosity (Agyare, Addo, & Xiong, 2009) and influence the chemical composition, structural and functional properties (Guo et al., 2021), finally influencing the practical applications (Zheng, Luo, Yao, Lu, & Bu, 2014; Da Silva et al., 2019). Temperature effects on gelatine, chitosan and gelatine-chitosan based complex were therefore discussed.
As for gelatine, the increased extraction temperature (45–75 °C), due to the extreme hydrolysis, could (1) increase α-Amino acid group content and antioxidative activities (Kittiphattanabawon, Benjakul, Visessanguan, & Shahidi, 2010), (2) increase yield (inactivate those proteases and yield gelatine with less degradation) but decrease the gel strength, with larger strands and larger voids (Kaewruang, Benjakul, Prodpran, & Nalinanon, 2013), (3) result in the degradation of the major protein components (i.e., α-, β- and γ-chain) of gelatine and increase the low-MW peptides, with high solubility, (4) lift the surface hydrophobicity with more exposed hydrophobic groups and surface activity of gelatine, (5) decrease the isoelectric points and (6) affect capacity, viscosity, stability and thereby the practical application (Tan et al., 2020).
Chitosan was reported as insoluble in sulphuric acid at room temperature with possible solubility in hot boiling solutions, though chitosan was soluble in several acids such as acetic, citric, lactic and hydrochloric acids (Zamani, Edebo, Sjöström, & Taherzadeh, 2007). During extraction, dilute sulphuric acid at 120 °C for 20 min could dissolve chitosan and the extracted chitosan was recovered from the acid solution by precipitation at lower temperatures (Zamani, Edebo, Niklasson, & Taherzadeh, 2010). On another hand, chitosan at extraction temperature 90 °C showed better physicochemical properties compared to the one prepared at 40 °C, resulting in an increase in amino groups (–NH2) and lower MW (Boudouaia, Bengharez, & Jellali, 2019). Biomass decreased with increasing extraction temperatures, i.e., 13 g L−1 at 20 °C and 11.6 g L−1 at 28 °C (Aili, Adour, Houali, & Amrane, 2019). It should be noted that high temperature may result in the degradation of chitosan (Gamiz-Gonzalez et al., 2017), which needs to be considered during chitosan processing for application; moreover, the extent of depolymerisation of chitosan increased, as expected, with elevated temperatures and with increasing acetic acid concentrations (Tsao et al., 2011).
In addition to the temperature imposing effects on the raw materials, the formation of gelain-chitosan based complex is affected due to the above-mentioned factors, including different components, variable pI or pKa values that are induced by temperature, leading to different interactions (electrostatic interactions and hydrogen bonds) and subsequently the physicochemical properties of the complex. When adding active materials (like anthocyanin) that are particularly unstable and are easily affected by light, pH, temperature, oxygen, and other environmental factors, the treatment at a low temperature in the dark for 4 h was important for gelatine-chitosan-anthocyanin complex preparation (Ma et al., 2020). These researchers found that the optimal temperature for gelatine-chitosan-anthocyanin complex nanoparticles storage was 4 °C and the optimal release temperature was 15 °C.
Overall, temperature has great influence on the inherent characteristics of raw materials (gelatine and chitosan), the formation process of gelatine-chitosan complex, the storage of the final complex and the further storage and application conditions.
Functional materials
Incorporation of functional materials by directly blending, nanoincorporation or additive-assisting stirring to enhance physical properties and biochemical properties of the chitosan-gelatine complex is of vital importance to fabricate high-performance preservation materials (Xiong, Chen, Warner, & Fang, 2020; Xu, Wei, Jia, & Song, 2020; Yadav, Mehrotra, Bhartiya, Singh, & Dutta, 2020; Yeddes et al., 2020; Zhao, Wei, Xu, & Han, 2020). Overall, the commonly used functional materials include active materials and modifiers and their addition into the gelatine-chitosan matrix (Fig. 3), could distinctively (1) improve bioactive and physicochemical properties, including antimicrobial activity (bacteria, fungi and virus) and antioxidant activity, (2) alter the mechanical property, colour characteristics and barrier property, (3) assist in the dissolving of materials, enhance homogeneous dispersion, make uniform film-forming solutions and promote high stretchability and sealability of gelatine-chitosan complex and (4) inhibit food damage and production of off-odours and off-tastes.
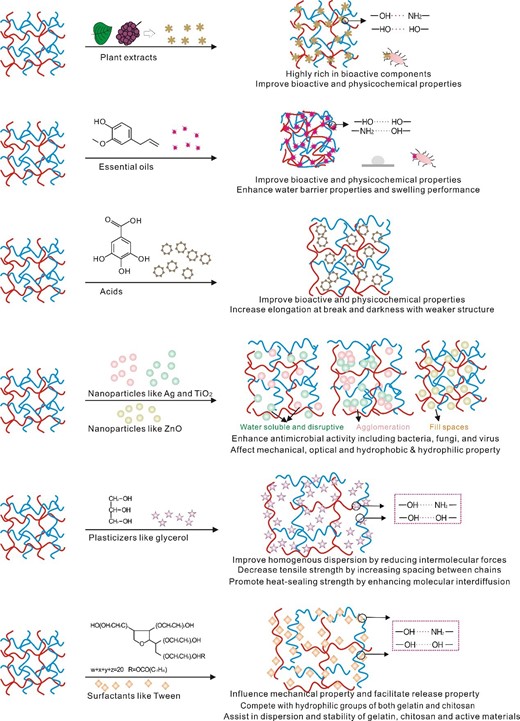
Active materials
Addition of active materials is often utilised to improve bioactive properties of the gelatine-chitosan complex (Roy & Rhim, 2021). In combination with Fig. 3, The applicability of these active materials in gelatine-chitosan complex by is based on (1) Active materials could help scavenge free radicals, chelate metal ions (Wang et al., 2020), improve antioxidant performance (related to the active materials’ special structure like 1,8-cineol, ρ-cymene, phytol, eicosanoic acid, cis,cis,cis-7,10,13-hexadecatrienal and α-irone) (Bonilla & Sobral, 2019) and enhance antimicrobial activity (related to the active materials’ special structure like monoterpene changing the cell structure causes the leakage of cytoplasm) (Sarmast, Fallah, Habibian Dehkordi, & Rafieian-Kopaei, 2019). (2) Active materials could increase the materials viscous behaviour (Bertolo, Martins, Horn, Brenelli, & Plepis, 2020), raise elongation at break values like a plasticising effect due to a reduction of intermolecular forces along biopolymer chains (Bonilla, Poloni, Lourenço, & Sobral, 2018), enhance the thickness (induced by insertation of active materials into the network of film matrix), facilitate water vapour barrier (attributed to the free volume in the polymer matrix was greatly reduced by the extract incorporation) and promote light barrier (due to the light scattering effect of the extract) (Kan et al., 2019). (3) On another hand, stable incorporation of active materials into the complex by electrostatic interaction and hydrogen bonding could overcome the inherent insoluble properties, unstable and volatile characteristics of active materials, and adjust the their sensitivity to factors like temperature, light, pH, oxygen concentration and storage time (Y. Wang et al., 2020). (4) The release properties of active materials from the complex, by the special diffusion routes and the controlled speed, promote the complex as promising active biomaterials for nutritional fortifiers, food flavouring agents and especially food preservation (Mousavi, Naseri, Babaei, Hosseini, & Shekarforoush, 2021).
Plant extracts are natural materials and are well known for its high levels of bioactive components. Embedding natural bioactive plant extracts, such as fruit extracts and vegetable extracts, has been a widely used way to enhance the preservation performance of gelatine-chitosan complex (Bertolo et al., 2020). For example, grape seed extract, an inexpensive natural source with flavanols (Shahbazi, 2017), could be added into gelatine-chitosan complex solutions and enhanced antimicrobial and antioxidant activity, while the further addition of nisin did not improve antimicrobial and antioxidant effects, probably due to the gelatine-chitosan coating matrix with compact structure affecting the functional property of grape seed extract and nisin (Xiong et al., 2020). Quercetin, another example, was incorporated into gelatine-chitosan complex and the preparation is illustrated in Fig. 4a, which shows electrostatic interaction and hydrogen bonding among gelatine, chitosan and quercetin (Yadav et al., 2020). The surfaces of the obtained complex film were changed and observed as irregular, uneven rough structure (in Fig. 4b). The final gelatine-chitosan-quercetin based complex presented superior antioxidant activity in comparison to gelatine-chitosan complex, promising its application in food preservation.
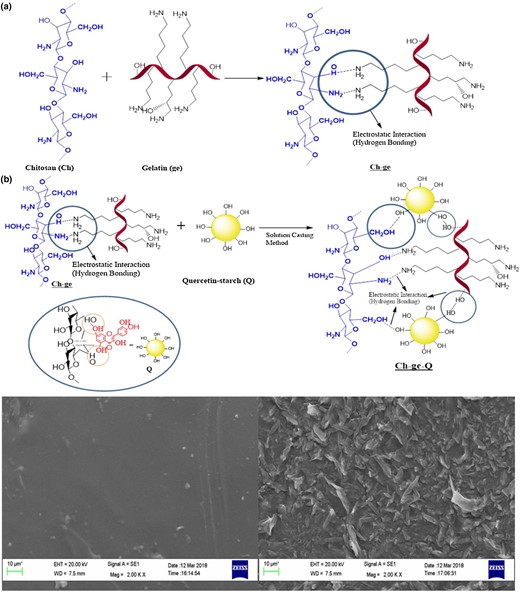
Preparation of gelatine-chitosan complex containing quercetin-starch (a) and the morphology of the complex (b) (Yadav et al., 2020).
Essential oils have long been recognised as valid alternatives to synthetic food additives due to wide-spectrum antibacterial & antifungal capacity (Mohebi & Shahbazi, 2017; Shahbazi, 2017; Vafania et al., 2019). Incorporation of essential oils in biopolymers could overcome its volatile nature and undesirable organoleptic properties (in high doses), improve water barrier properties and inhibit swelling of gelatine-chitosan films; this phenomenon could be attributed to the influence of the hydrophobic nature of essential oil as well as the formation of cross-link network induced by the hydrogen bonds among gelatine, chitosan and essential oil, resulting in a deficiency of the availability of hydrogen groups to water (Yeddes et al., 2020). Gelatine-chitosan-essential oil complex also demonstrated more effective than gelatine-chitosan complex alone, with high antibacterial and antioxidant activity, to retard the physicochemical deterioration and improve freshness indexes in food (Sarmast et al., 2019; Rehman, Jafari, Aadil, Assadpour, Randhawa, & Mahmood, 2020; Yeddes et al., 2020), without any effect on sensory quality of food (Lin, Gu, & Cui, 2019).
Bioactive acids could also act as efficient active materials as incorporated into gelatine-chitosan complex (Benbettaieb et al., 2020). The incorporation enlightened a new way to prevent food loss by effectively scavenging free radicals, absorbing light in the ultraviolet region and chelating transition metals. This allowed to slow or to inhibit the food damages and the production of unpleasant odours and tastes resulting from autoxidative processes (Halim, Kamari, & Phillip, 2018; Rezaee et al., 2018; Rezaee, Askari, & EmamDjomeh, 2020; Uranga et al., 2019). Gallic acid, for example, has several reported bioactivities like bacteriostatic activities, and after addition of gallic acid into gelatine-chitosan complex film, it could improve the physicochemical features and the release properties (Rezaee et al., 2018, 2020), and increase elongation at break (up to 82.63%) and darkness (reducing to 69.44 in L values) with weak structure. Another literature reported that the film based on gelatine-chitosan-gallic acid even exhibited higher antioxidant activities than the trans-cinnamic acid incorporated complex, which was mainly related to the structure of each acid (Benbettaieb et al., 2020).
Active materials including plant extracts, essential oil, bioactive acid, etc., could form electrostatic interaction and hydrogen bonds with gelatine-chitosan complex and could further facilitate bioactivity, mechanical property, barrier property or even the changes in thickness. Overall, the types of active materials should be comprehensively chosen for specific needs and researchers should make targeted choices of active materials for different application environments.
Modifiers
To further improve the structures of gelatine-chitosan complex, modifiers were adopted wisely (Fig. 3), aiming at (1) promoting the complex to have desired performance like higher antibacterial or antioxidant performance (Amjadi, Emaminia, Heyat Davudian, Pourmohammad, Hamishehkar, & Roufegarinejad, 2019; Amjadi et al., 2020), (2) facilitating the stable dispersion of all components (Mohammadi et al., 2018; Cardoso et al., 2019; Sarmast et al., 2019; Uranga et al., 2019; Benbettaieb et al., 2020; Xu et al., 2020), (3) improving compatibility between gelatine and chitosan (Cardoso et al., 2019) and (4) endowing gelatine-chitosan-moringa oil based complex with special characteristics like flexibility and heat-sealing properties (Prateepchanachai, Thakhiew, Devahastin, & Soponronnarit, 2019). The commonly used modifiers contain nanomaterials, glycerol, tween, etc.
Nanomaterials, at least one dimension at nanometre size in a three-dimensional space, are promising in food application. Silver nanoparticles have been reported on strong antibacterial activities due to their surface effects and quantum size effects, and could be prepared by in situ reduction with modified chitosan. The process could greatly reduce toxicity of silver nanoparticles (Cao et al., 2020), reduce the elongation at break (29.52%–25.32%) due to the hindering of the movement of polysaccharides molecules by silver nanoparticles, and decrease UV transmittance due to high crystallinity of silver nanoparticles, the separation of effective electron-hole pairs, and the quantum confinement effect. The combining addition of TiO2-silver nanoparticles could (Lin et al., 2020) (Fig. 3): (1) make the complex film more soluble in water (increasing from ˜20% to ˜30%) due to the hydrophilic nature of TiO2-Ag or due to the disruption of crystal structure of fish gelatine protein by TiO2 particles; (2) reduce the water vapour permeability (the minimum value 2.63 × 10−12 g·cm/cm2·s·Pa) of complex films due to Nano-TiO2 leading to a long and tortuous transport path of water vapour in thin films; (3) increase tensile strength (up to 17.39 MPa) due to the uniform dispersion at low concentrations to interact with certain amino acids in the gelatine matrix, while high concentration of TiO2-Ag tends to cause agglomeration and reduce the contact area and interaction between the nanomaterial and the polymer, resulting in a decrease in the tensile strength (decreasing from 17.39 MPa to 9.014 MPa) (Lin et al., 2020). ZnO nanoparticles could also (1) enhance the water contact angle (up to 92.49°) due to the formation of hydrogen bonds among components, resulting in the reduced free hydrophilic groups in the gelatine structure, (2) decrease meaningfully the water vapour permeability (˜4.5 × 10−7 gm−1 h−1 Pa−1) due to reducing gelatine chains mobility by filling free spaces between chains and reducing the diffusion rate of water vapour through the films, (3) increase tensile strength (up to 19.55 Mpa) and young’s modulus (1121.02 Mpa), due to the formation of interactions among ZnO and gelatine matrix, thus generating a stiff continuous network, reducing the mobility of the polymer chains and reinforcing the mechanical properties (Amjadi et al., 2019, 2020). The incorporation of chitosan nanofibre compensated the negative effect of ZnO nanoparticles on the colour properties of gelatine (Amjadi et al., 2019), which overall furthermore resulted in synergistic effect improving antibacterial (inhibitory zone up to 14.04 mm) and 1,1-diphenyl2-picrylhydrazyl inhibition activity (up to 53.02%) of the gelatine-chitosan based films (Amjadi et al., 2020).
The gelatine-chitosan-nanoparticle complexes have been researched academically and demonstrated the potential high bioactive and mechanical properties in relevant literatures. There is also the practical application investigation onto food like meat products, as Amjadi reported the nanocomposite film for fresh beef preservation (Amjadi et al., 2020). For market application, it is noted that according to the legislation, gelatine is approved as safe (GRAS) by United States Food and Drug Administration, European Commission, Food and Agriculture Organization of the United Nations, Food and Agriculture Organization of the United Nations and China National Standards; moreover, chitosan is approved as safe (GRAS) by United States Food and Drug Administration, European Union regulation, and China National Standards. However, nanoparticles have different recognitions, such as (1) ZnO nanoparticles that been generally recognised as safe (GRAS) by the U.S. Food and Drug Administration (Amjadi et al., 2019, 2020), and (2) silver nanoparticles that has certain toxicity and its release and migration should be lower than the permissible limit, which should be properly addressed to ensure food safety (Echegoyen & Nerin, 2013; Zorraquin-Pena, Cueva, Bartolome, & Moreno-Arribas, 2020). Therefore, in order to promote the practical value of gelatine-chitosan-nanoparticle complex in food preservation, it is important to investigate the toxicity of nanoparticles, study the migration performance and evaluate the effect of gelatine-chitosan-nanoparticles complex on human health.
Glycerol could improve the homogeneous dispersion of biomaterials through interfacial interaction. Glycerol was added as plasticiser and a functional group modifiers by many studies, into chitosan solutions, gelatine solutions, active material solutions or the aforementioned complex solutions to make uniformly dispersed gelatine-chitosan solutions by lowering the embrittlement of materials, and increase the formation of linear gelatine and chitosan chains with less chain entanglement and reduced intermolecular forces, for further bioactive complex production (Mohammadi et al., 2018; Cardoso et al., 2019; Sarmast et al., 2019; Uranga et al., 2019; Benbettaieb et al., 2020; Xu et al., 2020). Addition of glycerol (Fig. 3), (Prateepchanachai, Thakhiew, Devahastin, & Soponronnarit, 2017; Prateepchanachai et al., 2019), in details, reduced the zeta potential because of the insertion of plasticiser molecules in between the biopolymers; reduced viscosity because of the lubrication effect of glycerol helping reducing the resistance between biopolymer chains; decreased the film crystallinity due to the enhancement of amorphous nature; decreased tensile strength by increasing spacing between chitosan chains and probably reduce chain-to-chain hydrogen bonding attraction resulting in facilitated chain movement and weaker chain structure with the increased flexibility of the resulting film; increase the seal strength (the highest value 110.7 N m−1) as heat-sealing probably because glycerol contains three CHOs helping enhance the molecular interdiffusion and chain interactions via hydrogen bonds and lead to better melting and hence the improved sealing behaviour. As for the final films, the addition of glycerol established favourable hydrogen bonds with the polymeric backbone, thereby disrupting intermolecular polymer interactions, yielding to a less dense polymer matrix in which the mobility of polymer chains was facilitated and resulting in the reduced thermal stability (decreasing the degradation temperature from 295 °C to 284 °C); however the protective and strengthening effect of gallic acid at 5% is predominant on the weakening effect of glycerol in the films (Benbettaieb et al., 2020).
Tween, i.e., polysorbate, is a nonionic surfactant and is widely used as emulsifier or solubiliser in food. Tween is actually a series of products of the same type, such as tween 20, tween 40, tween 60, tween 80, etc. (Rezaee et al., 2018, 2020; Sarmast et al., 2019; Dai et al., 2020; Yadav et al., 2020). Tweens have different nature of the hydrophobic group, in which Tween 20 refers to the straight hydrocarbon chain compared to the olefinic group in the hydrocarbon chain of Tween 80; difference between HLB numbers of Tweens is small; Tween 20 and Tween 80 reveal high stabilizing properties due to higher reduction of the interfacial tension in systems (Bąk & Podgórska, 2012, 2016). Tween 20 as an emulsifier agent could help obtain stable gelatine-chitosan-active material dispersion and decrease film thickness significantly; especially, Tween 20 leads to higher release rate and elongation at break (from 66.34% to 82.63%) (due to amphiphilic properties of tween as surfactant, which can compete with hydrophilic groups of both matrix polymers in adsorption of each other hydrophilic groups) (Rezaee et al., 2018, 2020) (Fig. 3). Tween 80 could also be added into chitosan or gelatine solutions and speed up the dissolving of gelatine and chitosan to prepare the mixture solutions (Shahbazi, 2017; Bonilla et al., 2018; Lin et al., 2019). Furthermore, tween 80 could assist in dispersion of active materials that have limited solubility, before adding to the prepared gelatine-chitosan complex solutions (Lin et al., 2019; Sarmast et al., 2019; Yadav et al., 2020), which were reported to maintain stable with the increased stability and the less loss of active compositions in the complex.
In addition to the abovementioned materials, ethanol, starch, etc., are also used for improving the structure of gelatine-chitosan complex. Overall, these modifiers could endow solutions and complex with designed enhancement in performance.
Preservation application
The gelatine-chitosan based complex could be made into films, coatings, nanomaterials, etc., for further preserving food. As for films, gelatine-chitosan based film-forming solutions could use solution casting method, an easy-operation, low-cost and environmentally friendly method via dishes or plates, to produce gelatine-chitosan complex films for the specially designed application (Ghaderi et al., 2019; Xu et al., 2020); also, electrospinning apparatus was adopted to produce nanofibres based on gelatine and chitosan for film preparation and application onto food like cheese (Cui, Bai, Rashed, & Lin, 2018; Lin et al., 2019). When a coating is undertaken, the gelatine-chitosan based solutions are directly attached to the surface of food, by dipping or immersing food into the solutions, removing, draining, packing and subsequently storing; the layer-by-layer strategy could help in forming gelatine-chitosan bilayers for better physicochemical properties than monolayer-coating (Cardoso et al., 2019); this method is relatively easy, time-saving and energy-saving for food like fillet to achieve protection against the environmental factors such as microbes, water and light (Sarmast et al., 2019; Bertolo et al., 2020; Xiong et al., 2020). Emulsification could also prepare the gelatine-chitosan based emulsion, such as gently dropping copaiba oil to gelatine-chitosan gel under mechanical constant stirring for obtaining emulsion that could work as a new selective agent to control pathogenic bacteria (Marangon et al., 2017); the combination of casting and emulsification could further increase the extensibility of complex films in addition to decreasing water solubility, water vapour permeability and opacity (Larissa Tessaro, Luciano, Quinta Barbosa Bittante, Lourenço, Martelli-Tosi, & José do Amaral Sobral, 2021; Tessaro, Lourenco, Martelli-Tosi, & do Amaral Sobral, 2021). Final bioactive gelatine-chitosan complex illustrates the potential application in preserving food (Zhang et al., 2021).
Overall, the preservation, molecularly, is related to (1) the NH3+ group of chitosan leading to the antibacterial activity, (2) amino acids of gelatine resulting in the antioxidant activity, (3) the formation of the compact structure or the pre-designed structure by electrostatic interaction and hydrogen bonds promoting mechanical properties and high barrier or to water, water vapour, O2, CO2 and light or (4) active materials and modifiers enhancing the above properties, like high active contents with large number of cyclic amine groups which are good electron donors (Amjadi et al., 2020; Xu et al., 2020). Finally, the gelatine-chitosan complex, at high efficacy, could adjust pH and colour, maintain the nutrition value, improve the sensory index and extend shelf life of food (Bonilla & Sobral, 2019; Gedarawatte et al., 2021), which is in detail shown in Table 1.
Application . | Materials . | Results . | References . |
---|---|---|---|
Meat | |||
Pork | Gelatine-chitosan-eugenol coatings | It could effectively delayed the decline in the water- holding capacity, springiness, and cohesiveness of the chilled pork. | Wang et al. (2020) |
Pork | Gelatine-chitosan-nisin/grape sees extract coating | Pork oxidation and microbial spoilage are effectively inhibited. | Xiong et al. (2020) |
Pork | Gelatine-chitosan (tween 80)-tarragon essential oil (tween 80) coating | Coating treatments could significantly inhibit quality deterioration of pork slices, with an improved antioxidant, antibacterial and sensory properties. | Zhang et al. (2020) |
Beef | Gelatine-chitosan-betanin nanoliposome-ZnO-glycerol film | The growth of inoculated bacteria, lipid oxidation, and the changes in the pH and color quality of the beef samples were controlled effectively. | Amjadi et al. (2020) |
Beef | Gelatine-chitosan-glycerol coating | The coatings effectively retarded lipid oxidation and microbial growth (psychrotrophic bacteria, moulds, and yeast), extending the shelf-life from 6 to 10 days. | Cardoso et al. (2019) |
Fillet | Gelatine-chitosan (glycerol)-persian lime (citrus latifolia) peel essential oil (tween 80) coating | The treatment inhibit the microbial growth, retard the physicochemical deterioration, and improve freshness indexes in the fillets. | Sarmast et al. (2019) |
Omega-3 polyunsaturated fatty acids | Gelatine-chitosan coating | The treatment promote the thermal stability and protect it against oxidation. | Hosseini et al. (2021) |
Cheese | Gelatine-chitosan-glycerol-boldo extratct film | The psychrotrophic microorganism growth is inhibited, with a considerably low development of coliforms. | Bonilla & Sobral (2019) |
Cheese | Gelatine-chitosan-moringa oil (tween 80) nanofibers | The nanofibers possessed high antibacterial effect against L. monocytogenes and S. aureus on cheese at 4 °C and 25 °C and negligible impact on the surface color and sensory quality of cheese during 4 days storage. | Lin et al. (2019) |
Vegetables and fruits | |||
Cucumber | Gelatine-chitosan-clove oil (tween 80) nanofibers craft | The color and flavour is maintained well for > 4 days | Cui et al. (2018) |
Grape | Gelatine-chitosan-silver nanoparticle film | The shelf life of the fruit extended for additional two weeks. | Kumar et al. (2018) |
Fresh apple cubes | Gelatine (glycerol)-chitosan-FeIII doped hollow metal-organic framework film | The apple exhibited little oxidation and water loss without bacterial infection. | Zhao et al. (2020) |
Pomegranate seed | Gelatine-chitosan based complex film | No fungal growth was observed on the sample packed in the produced films during the test period (14 days) | Noorbakhsh-Soltani et al. (2018) |
Food additives | |||
Corn oil | Gelatine-chitosan-glycerol-ε-polylysine/astaxanthin film | Lipid oxidation was retarded with less than 5 nmol/mL of malondialdehyde detected when incubation at 60 °C for 59 h. | Xu et al. (2020) |
Copaiba oil | Gelatine-chitosan emulsion | Copaiba oil combined to gelatine-chitosan showed the reduced toxicity, the decreasing necessary concentration consumption | Marangon et al. (2017) |
Sausage | Gelatine-chitosan-thyme essential oil nanofiber | The treated sausages had the best odour and taste, since the aroma of the essential oil can be masked by encapsulation, indicating a good alternative to 120 ppm nitrite in the formulation of sausage | Vafania et al., (2019) |
Application . | Materials . | Results . | References . |
---|---|---|---|
Meat | |||
Pork | Gelatine-chitosan-eugenol coatings | It could effectively delayed the decline in the water- holding capacity, springiness, and cohesiveness of the chilled pork. | Wang et al. (2020) |
Pork | Gelatine-chitosan-nisin/grape sees extract coating | Pork oxidation and microbial spoilage are effectively inhibited. | Xiong et al. (2020) |
Pork | Gelatine-chitosan (tween 80)-tarragon essential oil (tween 80) coating | Coating treatments could significantly inhibit quality deterioration of pork slices, with an improved antioxidant, antibacterial and sensory properties. | Zhang et al. (2020) |
Beef | Gelatine-chitosan-betanin nanoliposome-ZnO-glycerol film | The growth of inoculated bacteria, lipid oxidation, and the changes in the pH and color quality of the beef samples were controlled effectively. | Amjadi et al. (2020) |
Beef | Gelatine-chitosan-glycerol coating | The coatings effectively retarded lipid oxidation and microbial growth (psychrotrophic bacteria, moulds, and yeast), extending the shelf-life from 6 to 10 days. | Cardoso et al. (2019) |
Fillet | Gelatine-chitosan (glycerol)-persian lime (citrus latifolia) peel essential oil (tween 80) coating | The treatment inhibit the microbial growth, retard the physicochemical deterioration, and improve freshness indexes in the fillets. | Sarmast et al. (2019) |
Omega-3 polyunsaturated fatty acids | Gelatine-chitosan coating | The treatment promote the thermal stability and protect it against oxidation. | Hosseini et al. (2021) |
Cheese | Gelatine-chitosan-glycerol-boldo extratct film | The psychrotrophic microorganism growth is inhibited, with a considerably low development of coliforms. | Bonilla & Sobral (2019) |
Cheese | Gelatine-chitosan-moringa oil (tween 80) nanofibers | The nanofibers possessed high antibacterial effect against L. monocytogenes and S. aureus on cheese at 4 °C and 25 °C and negligible impact on the surface color and sensory quality of cheese during 4 days storage. | Lin et al. (2019) |
Vegetables and fruits | |||
Cucumber | Gelatine-chitosan-clove oil (tween 80) nanofibers craft | The color and flavour is maintained well for > 4 days | Cui et al. (2018) |
Grape | Gelatine-chitosan-silver nanoparticle film | The shelf life of the fruit extended for additional two weeks. | Kumar et al. (2018) |
Fresh apple cubes | Gelatine (glycerol)-chitosan-FeIII doped hollow metal-organic framework film | The apple exhibited little oxidation and water loss without bacterial infection. | Zhao et al. (2020) |
Pomegranate seed | Gelatine-chitosan based complex film | No fungal growth was observed on the sample packed in the produced films during the test period (14 days) | Noorbakhsh-Soltani et al. (2018) |
Food additives | |||
Corn oil | Gelatine-chitosan-glycerol-ε-polylysine/astaxanthin film | Lipid oxidation was retarded with less than 5 nmol/mL of malondialdehyde detected when incubation at 60 °C for 59 h. | Xu et al. (2020) |
Copaiba oil | Gelatine-chitosan emulsion | Copaiba oil combined to gelatine-chitosan showed the reduced toxicity, the decreasing necessary concentration consumption | Marangon et al. (2017) |
Sausage | Gelatine-chitosan-thyme essential oil nanofiber | The treated sausages had the best odour and taste, since the aroma of the essential oil can be masked by encapsulation, indicating a good alternative to 120 ppm nitrite in the formulation of sausage | Vafania et al., (2019) |
Application . | Materials . | Results . | References . |
---|---|---|---|
Meat | |||
Pork | Gelatine-chitosan-eugenol coatings | It could effectively delayed the decline in the water- holding capacity, springiness, and cohesiveness of the chilled pork. | Wang et al. (2020) |
Pork | Gelatine-chitosan-nisin/grape sees extract coating | Pork oxidation and microbial spoilage are effectively inhibited. | Xiong et al. (2020) |
Pork | Gelatine-chitosan (tween 80)-tarragon essential oil (tween 80) coating | Coating treatments could significantly inhibit quality deterioration of pork slices, with an improved antioxidant, antibacterial and sensory properties. | Zhang et al. (2020) |
Beef | Gelatine-chitosan-betanin nanoliposome-ZnO-glycerol film | The growth of inoculated bacteria, lipid oxidation, and the changes in the pH and color quality of the beef samples were controlled effectively. | Amjadi et al. (2020) |
Beef | Gelatine-chitosan-glycerol coating | The coatings effectively retarded lipid oxidation and microbial growth (psychrotrophic bacteria, moulds, and yeast), extending the shelf-life from 6 to 10 days. | Cardoso et al. (2019) |
Fillet | Gelatine-chitosan (glycerol)-persian lime (citrus latifolia) peel essential oil (tween 80) coating | The treatment inhibit the microbial growth, retard the physicochemical deterioration, and improve freshness indexes in the fillets. | Sarmast et al. (2019) |
Omega-3 polyunsaturated fatty acids | Gelatine-chitosan coating | The treatment promote the thermal stability and protect it against oxidation. | Hosseini et al. (2021) |
Cheese | Gelatine-chitosan-glycerol-boldo extratct film | The psychrotrophic microorganism growth is inhibited, with a considerably low development of coliforms. | Bonilla & Sobral (2019) |
Cheese | Gelatine-chitosan-moringa oil (tween 80) nanofibers | The nanofibers possessed high antibacterial effect against L. monocytogenes and S. aureus on cheese at 4 °C and 25 °C and negligible impact on the surface color and sensory quality of cheese during 4 days storage. | Lin et al. (2019) |
Vegetables and fruits | |||
Cucumber | Gelatine-chitosan-clove oil (tween 80) nanofibers craft | The color and flavour is maintained well for > 4 days | Cui et al. (2018) |
Grape | Gelatine-chitosan-silver nanoparticle film | The shelf life of the fruit extended for additional two weeks. | Kumar et al. (2018) |
Fresh apple cubes | Gelatine (glycerol)-chitosan-FeIII doped hollow metal-organic framework film | The apple exhibited little oxidation and water loss without bacterial infection. | Zhao et al. (2020) |
Pomegranate seed | Gelatine-chitosan based complex film | No fungal growth was observed on the sample packed in the produced films during the test period (14 days) | Noorbakhsh-Soltani et al. (2018) |
Food additives | |||
Corn oil | Gelatine-chitosan-glycerol-ε-polylysine/astaxanthin film | Lipid oxidation was retarded with less than 5 nmol/mL of malondialdehyde detected when incubation at 60 °C for 59 h. | Xu et al. (2020) |
Copaiba oil | Gelatine-chitosan emulsion | Copaiba oil combined to gelatine-chitosan showed the reduced toxicity, the decreasing necessary concentration consumption | Marangon et al. (2017) |
Sausage | Gelatine-chitosan-thyme essential oil nanofiber | The treated sausages had the best odour and taste, since the aroma of the essential oil can be masked by encapsulation, indicating a good alternative to 120 ppm nitrite in the formulation of sausage | Vafania et al., (2019) |
Application . | Materials . | Results . | References . |
---|---|---|---|
Meat | |||
Pork | Gelatine-chitosan-eugenol coatings | It could effectively delayed the decline in the water- holding capacity, springiness, and cohesiveness of the chilled pork. | Wang et al. (2020) |
Pork | Gelatine-chitosan-nisin/grape sees extract coating | Pork oxidation and microbial spoilage are effectively inhibited. | Xiong et al. (2020) |
Pork | Gelatine-chitosan (tween 80)-tarragon essential oil (tween 80) coating | Coating treatments could significantly inhibit quality deterioration of pork slices, with an improved antioxidant, antibacterial and sensory properties. | Zhang et al. (2020) |
Beef | Gelatine-chitosan-betanin nanoliposome-ZnO-glycerol film | The growth of inoculated bacteria, lipid oxidation, and the changes in the pH and color quality of the beef samples were controlled effectively. | Amjadi et al. (2020) |
Beef | Gelatine-chitosan-glycerol coating | The coatings effectively retarded lipid oxidation and microbial growth (psychrotrophic bacteria, moulds, and yeast), extending the shelf-life from 6 to 10 days. | Cardoso et al. (2019) |
Fillet | Gelatine-chitosan (glycerol)-persian lime (citrus latifolia) peel essential oil (tween 80) coating | The treatment inhibit the microbial growth, retard the physicochemical deterioration, and improve freshness indexes in the fillets. | Sarmast et al. (2019) |
Omega-3 polyunsaturated fatty acids | Gelatine-chitosan coating | The treatment promote the thermal stability and protect it against oxidation. | Hosseini et al. (2021) |
Cheese | Gelatine-chitosan-glycerol-boldo extratct film | The psychrotrophic microorganism growth is inhibited, with a considerably low development of coliforms. | Bonilla & Sobral (2019) |
Cheese | Gelatine-chitosan-moringa oil (tween 80) nanofibers | The nanofibers possessed high antibacterial effect against L. monocytogenes and S. aureus on cheese at 4 °C and 25 °C and negligible impact on the surface color and sensory quality of cheese during 4 days storage. | Lin et al. (2019) |
Vegetables and fruits | |||
Cucumber | Gelatine-chitosan-clove oil (tween 80) nanofibers craft | The color and flavour is maintained well for > 4 days | Cui et al. (2018) |
Grape | Gelatine-chitosan-silver nanoparticle film | The shelf life of the fruit extended for additional two weeks. | Kumar et al. (2018) |
Fresh apple cubes | Gelatine (glycerol)-chitosan-FeIII doped hollow metal-organic framework film | The apple exhibited little oxidation and water loss without bacterial infection. | Zhao et al. (2020) |
Pomegranate seed | Gelatine-chitosan based complex film | No fungal growth was observed on the sample packed in the produced films during the test period (14 days) | Noorbakhsh-Soltani et al. (2018) |
Food additives | |||
Corn oil | Gelatine-chitosan-glycerol-ε-polylysine/astaxanthin film | Lipid oxidation was retarded with less than 5 nmol/mL of malondialdehyde detected when incubation at 60 °C for 59 h. | Xu et al. (2020) |
Copaiba oil | Gelatine-chitosan emulsion | Copaiba oil combined to gelatine-chitosan showed the reduced toxicity, the decreasing necessary concentration consumption | Marangon et al. (2017) |
Sausage | Gelatine-chitosan-thyme essential oil nanofiber | The treated sausages had the best odour and taste, since the aroma of the essential oil can be masked by encapsulation, indicating a good alternative to 120 ppm nitrite in the formulation of sausage | Vafania et al., (2019) |
Preservation: meat
Meat or ingredient high in fat or protein are usually vulnerable to microbial, physical and biochemical changes (Mohebi & Shahbazi, 2017; Xiong et al., 2020). Gelatine-chitosan complex could be applied to preserving these products, including pork, beef, fish, etc.
As for pork, a highly perishable food product and susceptible to oxidation and microbial spoilage, the gelatine-chitosan complex could effectively inhibit the increases in pH, total volatile basic nitrogen, thiobarbituric acid-reactive substances and colour of pork (Wang, Zhang, & Ding, 2020; Xiong et al., 2020; Zhang, Liang, Li, & Kang, 2020). It also delayed the decline in the water-holding capacity, springiness and cohesiveness of pork, and the storage period could be even extended by 8 d. Non-coated pork had a higher degree of protein oxidation, which may be due to the direct reaction of meat with oxygen in the package and the intermediate materials generated during the protein oxidations could further accelerate oxidative chain reactions (Xiong et al., 2020), while chitosan and gelatine could form a compact structure and reduce the moisture/oxgen permeability.
As for beef, preservation should, as a matter of priority, extend shelf life and delay the main indicators of quality depreciation including loss of red colour, development of unpleasant aromas and odours, and superficial dehydration. The lipid oxidation and microbial growth (psychrotrophic bacteria, moulds and yeast) could be effectively retarded by gelatine-chitosan coatings, with the coated beef possessing the lower pH values, the lower weight loss and the prevented metmyoglobin accumulation on the beef surface, extending the shelf life from 6 to 10 days (Cardoso et al., 2019). Especially, the layers of gelatine-chitosan coatings matter greatly in preserving beef steaks. The bilayer achieved by immersing monolayer sample in the film-forming solution and dripping until the new layer had dried, with 2.0 times thickness of the monolayer, had higher antioxidant effectiveness values than monolayer samples, since (1) the bilayer presented a greater thickness and, consequently, lowered oxygen permeability, finally inhibiting oxidation, and (2) it may also be a result of the antioxidative property of chitosan and the bilayer has more chitosan than the monolayer (Cardoso et al., 2019). Moreover, the gelatine-chitosan complex films could also wrap beef, and effectively control growth of inoculated bacteria, lipid oxidation, and the changes in the pH and colour quality of beef samples, resulting in a longer shelf life (Amjadi et al., 2020).
Fish products are one of the rich sources of proteins, n-3 polyunsaturated fatty acids, vitamins and minerals, and their shelf life is limited due to the spoiled microorganisms and autolytic enzymes (Sarmast et al., 2019). Preservation application of gelatine-chitosan complex onto fish is attributed to that chitosan could bind ferrous ion (released from fish), initiating lipid oxidation. Moreover, it could retard physicochemical deterioration, improve freshness indexes, and enhance the quality and shelf life of rainbow trout fillets, which is suitable for long term preservation of fish under superchilled condition (Sarmast et al., 2019). On another hand, there is an interesting report in which gelatine-chitosan complex could enhance physicochemical stability of Omega-3 polyunsaturated fatty acids concentrates (from fish)-loaded nanoliposomes, and could also effectively protect it against oxidation (Hosseini et al., 2021).
Currently, the research of gelatine-chitosan complex onto meat is gradually increasing, and the further study and application onto large amount of meat products should be broadened, improving effectiveness and consumers acceptability of meat.
Preservation: vegetables and fruits
Vegetables or fruits high in water content are also vulnerable to microbial spoilage or mechanical spoilage. Bacterial infections, due to their potential to be a public health hazard, have caused an increasing social concern, and foodborne illness is often associated with consumption of infected solely fresh products, including vegetables and fruits. Gelatine-chitosan complex with high antibacterial activity, could not only eliminate the bacterial biofilms, but also maintain sensory quality (colour, smell, taste and overall acceptability) of cucumber (Cui et al., 2018). Also, the grape wrapped with gelatine-chitosan complex was still fresh without putridity and the surface maintained smoothness without any leakage of the juice, illustrating the satisfactory preservation effect (Kumar, Shukla, Baul, Mitra, & Halder, 2018), while plastic (polyethylene) film wrapped red grapes showed obvious mildew appearance containing several mouldy spots and sticky juice also leaked to the surface, emitting a strong rotten smell. The fresh-cut apples could be preserved by gelatine-chitosan-FeIII doped hollow metal-organic framework based films and apple cubes exhibited the best condition with little oxidation and water loss without bacterial infection on fifth day (Zhao et al., 2020), as displayed in Fig. 5a. Zhang et al also preserved apples by gelatine-chitosan-tannic acid films with low water vapour and oxygen permeability, thereby alleviating cell oxidative damages, decreasing weight loss, delaying browning degree, inhibiting the lipid oxidase activity and decreasing the malondialdehyde content during 10 d of storage under 4 °C (Zhang et al., 2021), as shown in Fig. 5b. Therefore, gelation-chitosan complex could improve the shelf-life and display-life of vegetables and fruits.
Preservation: food additives
Safe food without the use of chemical preservatives has become a significant consumer demand, among which natural additives are promising alternatives. Chitosan could work as a highly effective natural preservative with rapid and long-term bacteriostatic effects. Food additives based on gelatine and chitosan are generally recognised as an effective strategy, due to its non-toxicity and low-cost merit as compared with chemical methods (Goncalves, Grosso, Rabelo, Hubinger, & Prata, 2018; Wang, Wang, Ding, Zhang, Reed, & Zhang, 2018b; Hafsa, Smach, Mrid, Sobeh, Majdoub, & Yasri, 2021).
Gelatine-chitosan complex could be added into high-fat food for protection application. The lipid oxidation of corn oil was retarded after addition of gelatine-chitosan based film, with less than 5 nmol mL−1 of malondialdehyde detected when incubation at 60 °C for 59 h (Xu et al., 2020). Also, in the emulsion form, the use of copaiba oil combined to gelatine-chitosan showed the reduced toxicity, the decreasing necessary concentration consumption and the shorter response time comparatively to the isolated complexes (Marangon et al., 2017), illustrating a new way towards the development of copaiba oil-based products to control Staphylococcus aureus infections. Interestingly, some authors nanoencapsulated thyme essential oil in gelatine-chitosan nanofibres and approved the efficiency of protecting antioxidant activity of essential oil, which was followed by adding this nanofibres into sausage (Vafania et al., 2019). It was found that this nanofibre complex could be a suitable nitrite substitute for meat products.
The gelatine-chitosan complex could work as food additives for incorporating, protecting, delivering and releasing of active materials, aiming at preserving food. However, the possible interaction with food ingredients should be systematically analysed to ensure functionality and safety.
Perspectives
The high demand for food safety and quality has stimulated exploration of bioactive food preservation, like gelatine-chitosan complex. There are many factors affecting the complex formation process, including gelatine origin, MW, DD, pH, salts, concentration, temperature, active materials, modifiers, etc. The complex could have excellent physicochemical properties (e.g., antibacterial property and antioxidant property) and could preserve practical food. Despite the potential application onto food preservation, there are still challenges for gelatine-chitosan complex to satisfy food safety standards and reach the industrial application.
Future developments in gelatine-chitosan complex should be considered in the following aspects: (1) Other factors including chemical modification, purity and molecular structure of gelatine and chitosan should be explored for optimising the formation of chitosan-gelatine complex; (2) More active materials and modifiers should be studied to make gelatine-chitosan complex more functional; (3) More food preservation applications of gelatine-chitosan complex should be explored, including solid, semisolid and fluid foods; (4) Impact of gelatine-chitosan complex on human health and environment should be carefully evaluated with high-standard professional method. Though there are many challenges for gelatine-chitosan complex to meet the market standards and consumer requirements, the bioactive complex based on gelatine and chitosan are promising in food preservation applications.
Acknowledgements
The authors acknowledge the support from Natural Science Foundation of Chongqing (cstc2020jcyj-msxmX0995), Fundamental Research Funds for the Central Universities of China (SWU119069), National Natural Science Foundation of China (31671881; 31972102), Chongqing Research Program of Basic Research and Frontier Technology (cstc2018jcyjA0939), and Jiangsu University for senior Intellectuals (20JDG18).
Author contribution
Hongxia Wang: Funding acquisition (equal); Methodology (equal); Project administration (equal); Writing-review & editing (equal). Fuyuan Ding: Investigation (equal); Validation (equal). Liang Ma: Resources (equal). Yuhao Zhang: Funding acquisition (equal).
Conflict of interest
We confirmed that there is no potential competing conflict of interest.
Ethical approval
Ethics approval was not required for this research.
Peer review
The peer review history for this article is available at https://publons.com/publon/10.1111/ijfs.15340.
Data availability statement
The data that support the findings of this study are available from the corresponding author upon reasonable request.
Reference
(This reference analyzed the effect of active material (extract) on gelatin-chitosan complex and the application on cheese. Edible gelatin-chitosan that blended films containing Boldo extract with application on sliced Prato cheese did not allow psychrotrophic microorganism growth with a considerably low development of coliforms shown in sliced Prato cheese samples.)
(This reference analyzed the effect of gelatin origin on the properties of gelatin-chitosan complex. The results indicated that fish gelatin could promote higher water resistance and deformation ability.)
(This reference analyzed factors (salts) affecting the complex between gelatin and chitosan. It provided insight into the behavior of gelatin-chitosan in varying ionic environments, thus enabling enhanced design for a broad range of food applications.)
(This reference analyzed factors (chitosan concentration and essential oil) affecting properties of the gelatin-chitosan complex. This reference also showed that gelation-chitosan nanofiber containing thyme essential oil could work as food additives and could be a suitable nitrite substitute for meat products.)
(This reference analyzed the effect of active materials (tannic acid) on the mechanical and barrier properties of gelatin-chitosan complex for fruit preservation. The obtained films illustrated better performance on decreasing weight loss, delaying browning degree, inhibiting the lipid oxidase activity and decreasing the malondialdehyde content during 10 d of apple storage under 4 ◦C.)