-
PDF
- Split View
-
Views
-
Cite
Cite
Paulina Kęska, Joanna Stadnik, Effect of in vitro gastro-pancreatic digestion on antioxidant activity of low-molecular-weight (<3.5 kDa) peptides from dry-cured pork loins with probiotic strains of LAB, International Journal of Food Science and Technology, Volume 56, Issue 12, December 2021, Pages 6268–6278, https://doi.org/10.1111/ijfs.15066
- Share Icon Share
Abstract
The antioxidant properties of low-molecular-weight peptides (<3.5 kDa) obtained from the dry-cured pork loins inoculated with probiotic and potentially probiotic lactic acid bacterial strains were tested after two-stage enzymatic hydrolysis under in vitro conditions. The water soluble fraction (WSF) was found to be a better substrate for enzymatic hydrolysis by proteolytic enzymes, especially when they were extracted from the meat product after 12 months of ageing. Furthermore, protein hydrolysates extracted from the salt soluble fraction (SSF) exhibited better or similar ABTS free radical scavenging activity and Fe2+-chelating activity than those extracted from WSF (despite their less quantity). Moreover, both SSF and WSF exhibited weak ferric-reducing antioxidant activity after two-step enzymatic hydrolysis (A700 averaged 0.138 for the WSF and 0.088 for the SSF). These results might be useful to the meat-processing industry in developing innovative and functional meat products.
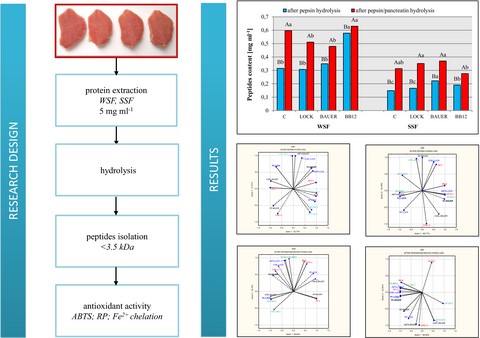
Introduction
In a biological system, free radicals of oxygen, nitrogen and sulphur contain an unpaired electron in their outermost shell which makes them highly active. These free radicals are termed reactive oxygen/nitrogen species (ROS/RNS) and are produced during the processes of cellular metabolism and other functional activities. They play important roles in the processes of cell signalling, apoptosis, gene expression and ion transport. However, excessive production of ROS causes an oxidative imbalance within the human body which may lead to the so-called oxidative stress syndrome, whose primary effect is seen as damage to the DNA, RNA, proteins and lipid molecules. Oxidative stress causes an increased risk of cardiovascular disease, cancer, autism and various other diseases. The human body has developed various protective systems against the harmful effects of ROS. For example, the intracellular antioxidant system based primarily on enzymes can be potentiated by the ingestion of dietary antioxidants which helps to maintain homeostasis in the body (Imam et al., 2017; Liu et al., 2018; Mirończuk-Chodakowska et al., 2018). Therefore, natural peptides derived from enzymatic hydrolysis of various food products, including meat, are currently being explored as new sources of biologically active compounds to enhance human health. This is the so-called ‘functional food’ concept which says that the addition of antioxidants can help the body to reduce the negative effects of oxidative processes. Due to its high protein content, meat is a potentially good source of biologically active peptides. Furthermore, dry-cured meat products are valuable sources of proteins due to the natural process of production, that is a long ageing period, usually around a year. In addition, the biochemical transformation of meat during long-term ageing includes proteolysis with the participation of endogenous and exogenous enzymes. The enzymes might be of microbial origins, such as lactic acid bacteria (LAB) that are responsible for the fermentation during the ageing process. These enzymes might also contribute to the accumulation of biologically active peptides in meat products or produce polypeptide precursors that can be further hydrolysed to active molecules by proteolytic digestion.
The total antioxidant capacity of different food products is usually determined based on different laboratory tests; however, they are often not comparable with each other. Antioxidant capacity is a general term that encompasses various chemical processes and that can be described by the primary mechanism of action. Biomolecules as antioxidant can act by single electron transfer (SET) or hydrogen atom transfer (HAT). In the SET-based methods, the reaction mixture consists of an antioxidant and an oxidant. The latter changes colour when the former transfers an electron to it. SET methods transfer one electron to reduce any compound, including metal ions, carbonyls and radicals. On the other hand, methods involving HAT are recommended for the measurement of antioxidant activity via quenching of free radicals by hydrogen donation. As example, in the 2, 2-azino-bis-3-ethylbenzothiazoline-6-sulfonic acid (ABTS) method, the antioxidant capacity depends on the duration of the reaction, the concentration of the antioxidant, and its activity. In turn, the reducing power (RP) of the antioxidant is tested in relation to the amount of Fe3+ ions present in the reaction system. The principle of RP assay is based on the colorimetric estimation of the absorbance of the reaction mixture. An increase in the absorbance value indicates an increase in antioxidant activity. This assay is based on the electron transfer mechanism which is in contrast to SET- and HAT-based methods. Therefore, RP assay, in combination with other methods, can be very useful in analysing the antioxidant capacity of different antioxidants. The metal-chelating ability of an antioxidant allows us to assess its preventive role against Fenton reaction, and therefore, suggests its preventive role against oxidative damage (Prior et al., 2005; Gülcin, 2012; Santos-Sánchez et al., 2019). Different methods show different trends in the antioxidant activity, therefore, in this study, we aimed to look for a relationship between these trends by using low-molecular-weight peptides (<3.5 kDa) obtained from the dry-cured pork loin after 12 months of ageing. Literature shows that low-molecular-weight peptides have better antioxidant properties than that of large polypeptides or protein hydrolysates (Ajibola et al., 2011; Lorenzo et al., 2018). In addition, a previous study shows the microbial contribution towards the enhanced antioxidant activity of protein hydrolysates (Callegaro et al., 2018). Therefore, the effect of LAB and the biological potential of peptides against oxidative factors has been considered. To evaluate the antioxidant properties of low-molecular-weight peptides (<3.5 kDa), we employed three independent tests with different reaction mechanisms: ferrous ion (Fe2+) chelating activity, ABTS radical scavenging activity and reducing antioxidant power (RP) assay. The model consisted of two-stage enzymatic hydrolysis that simulated the digestive process in the human gastrointestinal tract. According to the hypothesis, the aim of the study is to assess the effect of selected strains of LAB on the antioxidant activity of small peptides (>3.5) obtained from dry-cured pork loin after hydrolysis.
Materials and methods
Sample preparation and product presentation
In this study, pork loins (m. longissimus thoracis) of Polish White Large breed were used. Meat cuts (n = 12) were excised at 24 h postmortem from half carcasses chilled at 4 °C (Lublin, Poland). After 48 h postmortem, all loins were cured with a mixture of 18 g NaCl, 9.7 g curing salt and 0.3 g NaNO3 per kg of loin using a surface massage. To allow the diffusion of the curing salt, all batches were then kept at 4 °C for 24 h. After the curing stage, loins were randomly divided into three experimental batches with three loins in each batch: those inoculated with 0.2% (v/w) probiotic (Lactobacillus rhamnosus LOCK900, Bifidobacterium animalis subsp. lactis BB12) and potentially probiotic (Lactobacillus acidophilus Bauer Ł0938) strains, respectively, transferred onto the meat surface in the amount of 106–107 CFU g−1 of meat (Kęska et al., 2020). In addition, three samples of loins without LAB strains were maintained as the control sample (C). Subsequently, the loins were hung at 16 ± 1 °C in a laboratory ageing chamber with a relative humidity of 75 ± 5% for 14 days. After this, the whole pieces of loins were vacuum-packed and aged at 4 ± 1 °C for 12 months. Three independent experimental series were conducted.
According to a previous study (Kęska et al., 2020), the physicochemical parameters of dry-cured loins with probiotic strains of LAB after 12 months pointed to a high-quality product. Figure 1 shows the cross-section of the loins for a better presentation of the products. The microbial status of dry-cured pork loins was also analysed. Total viable counts were determined using plate count agar after incubation at 30 °C for 72 h (ISO 4833-2:2013). The population of LAB including probiotic bacteria was determined (ISO 15214, 1998), and microbiological tests were performed to determine Enterobacteriaceae counts (ISO 21528-2:2004). The bacterial counts are expressed as log CFU g−1 (n = 3). The results show the good growth and survival of LAB in the case of all study samples (from 6.12 (LOCK) to 7.31 (BAUER)). The abundance of Enterobacteriaceae strains was used to assess the overall hygienic quality of the food products, which was found to be below the detectable level in the study samples (<1.00 CFU g−1) (Table 1).
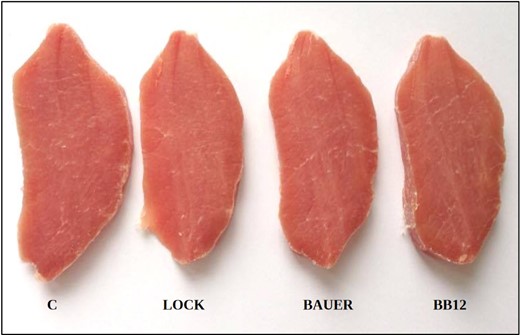
Dry-cured pork loins after 12 months of ageing. C – sample without starter culture; LOCK – sample with probiotic strain Lactobacillus rhamnosus LOCK900; BAUER – sample with potentially probiotic strain Lactobacillus acidophilus Bauer Ł0938; BB12- sample with probiotic strain Bifidobacterium animals ssp. lactis BB-1.
Microbial condition (log CFU g−1) of dry-cured loins with probiotic strains of LAB (mean ± standard deviation)
Parameter . | C . | LOCK . | BAUER . | BB12 . |
---|---|---|---|---|
LAB | 7.08a ± 0.26 | 6.12b ± 0.26 | 7.31a ± 0.27 | 7.08a ± 0.26 |
TVC | 6.82b ± 0.14 | 5.52c ± 0.16 | 7.52a ± 0.16 | 6.94b ± 0.14 |
Enterobacteriaceae | <1 | <1 | <1 | <1 |
Parameter . | C . | LOCK . | BAUER . | BB12 . |
---|---|---|---|---|
LAB | 7.08a ± 0.26 | 6.12b ± 0.26 | 7.31a ± 0.27 | 7.08a ± 0.26 |
TVC | 6.82b ± 0.14 | 5.52c ± 0.16 | 7.52a ± 0.16 | 6.94b ± 0.14 |
Enterobacteriaceae | <1 | <1 | <1 | <1 |
C – sample without starter culture; LOCK – sample with probiotic strain Lactobacillus rhamnosus LOCK900; BAUER – sample with potentially probiotic strain Lactobacillus acidophilus BauerŁ0938; BB12 – sample with probiotic strain Bifidobacterium animals ssp. lactis BB-12.
Microbial condition (log CFU g−1) of dry-cured loins with probiotic strains of LAB (mean ± standard deviation)
Parameter . | C . | LOCK . | BAUER . | BB12 . |
---|---|---|---|---|
LAB | 7.08a ± 0.26 | 6.12b ± 0.26 | 7.31a ± 0.27 | 7.08a ± 0.26 |
TVC | 6.82b ± 0.14 | 5.52c ± 0.16 | 7.52a ± 0.16 | 6.94b ± 0.14 |
Enterobacteriaceae | <1 | <1 | <1 | <1 |
Parameter . | C . | LOCK . | BAUER . | BB12 . |
---|---|---|---|---|
LAB | 7.08a ± 0.26 | 6.12b ± 0.26 | 7.31a ± 0.27 | 7.08a ± 0.26 |
TVC | 6.82b ± 0.14 | 5.52c ± 0.16 | 7.52a ± 0.16 | 6.94b ± 0.14 |
Enterobacteriaceae | <1 | <1 | <1 | <1 |
C – sample without starter culture; LOCK – sample with probiotic strain Lactobacillus rhamnosus LOCK900; BAUER – sample with potentially probiotic strain Lactobacillus acidophilus BauerŁ0938; BB12 – sample with probiotic strain Bifidobacterium animals ssp. lactis BB-12.
Protein extraction
A water soluble fraction (WSF) and a salt soluble fraction (SSF) were extracted from meat samples according to the method described by Molina & Toldra (1992) with the modifications suggested by Fadda et al. (1999). The concentration of protein in the protein extract was determined by the biuret method (Gornall et al., 1949) using Liquick Cor-TOTAL PROTEIN 60 (CORMAY Group, Warsaw, Poland) kit and adjusted to a concentration of 5 mg mL−1 in all variants.
In vitro gastro-pancreatic digestion and isolation of peptides
Figure 2 shows the scheme for the enzymatic hydrolysis of the extracts. All protein extracts of dry-cured pork loins were hydrolysed using pepsin and pancreatin according to the method described by Escudero et al. (2014). To isolate the low-molecular-weight peptides (<3.5 kDa) from the hydrolysates, we fractionated the obtained hydrolysates using membrane ultrafiltration (Spectra/Por®) against phosphate-buffered saline (pH 7.4) without light for 1 h at 37 °C. The peptide content in the obtained fraction was determined by using the trinitrobenzenesulfonic acid method (Adler-Nissen, 1979), and the results are expressed as milligrams per milliliter of leucine equivalent.
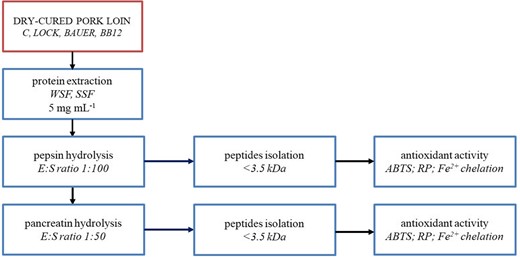
Determination of antioxidant activity
ABTS radical scavenging activity of the peptides was detected according to the method described by Re et al. (1999). Ferrous ion (Fe2+) chelating ability of peptides was determined according to the method described by Decker & Welch (1990). The RP activity of the peptides was determined by the method described by Oyaizu (1986). Analyses were performed in four replications for each series (n = 12).
Statistical analysis
The differences between mean values were found to be significant if their P-value was <0.05 based on Tukey’s range t-test. The results are expressed as mean ± standard deviation (SD). A two-way analysis of variance (ANOVA) at P < 0.05 was used to analyse the effect of the LAB strain and proteolytic steps of digestion. Multivariate analyses (cluster analysis and principal component analysis) were conducted to enhance the presentation of the results. In the case of multidimensional analysis, a hierarchical grouping of data was performed, and the results are presented as a dendrogram by using the ward method and euclidean distance. Data were analysed using Statistica version 13.3 software (Dell, Inc., Round Rock, TX, USA).
Results and discussion
Effect of the digestive steps on the content of low-molecular-weight peptides obtained from dry-cured pork loins with the probiotic strain of LAB
Figure 3 shows the peptide fraction of dry-cured pork loins with the probiotic strain of LAB after enzymatic hydrolysis. In our previous studies, we have shown a significant treatment effect was shown (P < 0.01) and time (P < 0.01) and interaction between them (P < 0.01) on the degree of proteolysis WSF and SSF, pointing to WSF as a better source of peptides. These results can be explained by the fact that degradation of protein during ageing is the sum degradation of muscle proteins to polypeptides and is due to the activity of muscle proteases and only assisted by the LAB’s contribution through acidification of the environment. The long maturation time and the type of strain will thus shape both the structure and composition of proteins (the degree of their degradation) after 12 months, which had a direct impact on the course of hydrolysis in this study.
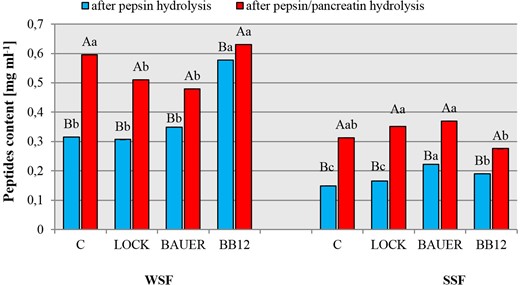
Peptides content after in vitro gastric (by pepsin) and gastric-pancreatic (pepsin/pancreatic) digestion of WSF and SSF from dry-cured pork loins with probiotic strain of LAB after 12 months. WSF – water soluble protein fraction, SSF – salt soluble protein fraction. C – sample without starter culture; LOCK – sample with probiotic strain Lactobacillus rhamnosus LOCK900; BB12 – sample with probiotic strain Bifidobacterium animals ssp. lactis BB-12; BAUER – sample with potentially probiotic strain Lactobacillus acidophilus Bauer Ł0938. a–cWithin the treatment, means followed by the common letters do not differ significantly (P < 0.05); A–BWithin the samples, means followed by the common letters do not differ significantly (P < 0.05).
According to our results, there was a statistically significant (P < 0.05) effect of two-step hydrolysis compared to single-step (pepsin), which agrees with previous reports (You et al., 2010; Wiriyaphan et al., 2015; Callegaro et al., 2018; Martini et al., 2019). WSF was found to be more susceptible to hydrolytic degradation by pepsin than that of SSF (P < 0.05). Furthermore, the number of peptides in SSF was lower than that of WSF despite the same initial amount of protein (5 mg mL−1). These variations might be due to the differences in protein profiles from different classes. Most likely, the spatial structure of proteins affects the availability of cleavage sites for hydrolytic enzymes. It is not always possible to cleave peptide bonds at enzyme-specific cleavage sites. This is especially true in the case of large intact protein molecules, where proteases with narrow specificity cannot access part of the target polypeptide chain. Therefore, in this study, a two-step hydrolysis was used, where the use of pepsin in the first step facilitated the action of the peptidase in the next step (pancreatin), thus releasing peptides and amino acids from meat proteins.
The increased peptide content after enzymatic hydrolysis with pancreatin was as follows: C>LOCK>BAUER>BB12 by 89%, 66%, 37% and 9% respectively. For comparison, in relation to SSF, a lower peptide content was obtained, from 0.22 to 0.15 mg mL−1. In C and LOCK samples, there was more than a twofold increase in the peptide content after enzymatic hydrolysis. In turn, there was an approximately 50% increase in the peptide content in BB12 and BAUER samples, which shows that these batches are less susceptible to degradation by the pancreatic enzyme under the applied analysis conditions.
The dendrogram (Fig. 4) shows trends, in which a clear division of samples can be observed by taking into account the content of peptides after enzymatic hydrolysis, regardless of the analysed fraction (designated as PC-PEP and PC-PAN). In addition, we obtained a clear separation of batches based on the overall antioxidant activity of peptides obtained from dry-cured loin with the probiotic or potential probiotic LAB strain, depending on the hydrolytic step.
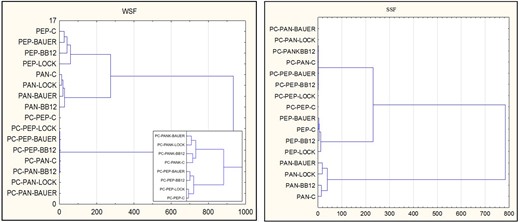
Dendrogram showing the relationship between the stage of in vitro gastric-pancreatic digestion and the content of peptide and their overall antioxidant activity obtained after hydrolysis of water soluble proteins fraction (WSF) and salt soluble protein fraction (SSF) from dry-cured pork loin after 12 months. PC – peptides content, PEP – samples after pepsin hydrolysis, PAN – samples after pancreatin hydrolysis, C – sample without starter culture; LOCK – sample with probiotic strain Lactobacillus rhamnosus LOCK900; BAUER – sample with potentially probiotic strain Lactobacillus acidophilus Bauer Ł0938; BB12 – sample with probiotic strain Bifidobacterium animals ssp. lactis BB-12.
Effect of hydrolysis on the antioxidant activity of low-molecular-weight peptides from dry-cured pork loins with the probiotic strain of LAB
The chemical activity of the oxygen radical is the strongest among pro-oxidative factors. The free radicals of oxygen readily react with molecules such as amino acids, proteins and DNA. Therefore, their removal is probably one of the most important necessities in protecting a living organism against various diseases caused due to oxidative stress (Pizzino et al., 2017). Tables 2 and 3 present the data on antiradical activity of low-molecular-weight peptides (<3.5 kDa) obtained after enzymatic hydrolysis. ABTS test demonstrated the antioxidant activity of the peptides released after enzymatic hydrolysis. This test measures the radical scavenging activity of a given molecule, that is its ability to give a hydrogen atom by a homolytic cleavage to the unstable free radical ABTS•+ (Canabady-Rochelle et al., 2015). The two-step enzymatic degradation of proteins from dry-cured pork loins via simulated gastro-pancreatic digestion increased the antiradical properties of the peptides released. In comparison with the two-step process, the one-step (pepsin) hydrolysis was less efficient in generating antioxidant peptides. However, the observed trend was not reported for all batches. WSF showed stronger antiradical activity (from 53.64% for BB12 to 34.79% for LOCK) after hydrolysis by pepsin (simulation of the stomach) than that of SSF, in which the antiradical activity was about 19.49%. In the second step of hydrolysis (by pancreatin representing pancreatic digestion), there was a significant increase in the antiradical activity by up to 76.77% on an average for WSF and 67.72% for SSF. Stronger peptides with a specific sequence of antioxidant activity were probably formed after the second step of digestion. The observed increase in radical uptake activity by pepsin and pancreatin hydrolysates was the result of the degradation of the native protein structure during proteolysis. As the degree of hydrolysis increased, the polar or charged moieties of the amino acid side chains became more exposed. Moreover, the increased number of peptides and the increased availability of free amino acids during enzymatic digestion provided an additional source of protons and electrons to maintain a high redox potential. The exposed amino acid residues acted as the electron donors and reacted with free radicals and stabilised them. These physicochemical changes may also explain the increased ability of the peptides after the two-step hydrolysis in neutralising ABTS free radicals. Peptides obtained after two-step enzymatic hydrolysis probably are more hydrophilic in nature, which promoted the neutralization of ABTS•+ (Zhu et al., 2008; You et al., 2010). Furthermore, some amino acids with aromatic and bulky side groups strongly contributed to the strong antioxidant activity against free radicals (Ajibola et al., 2011). Increased antioxidant activity during hydrolysis may also be caused by the participation of free amino acids in antioxidant capacity (Clausen et al., 2009). In this study, we used the RP assay to estimate the ability of small peptides as electron donors which can reduce the oxidised intermediates of lipid peroxidation processes, and therefore, can be used as primary and secondary antioxidants (Liu et al., 2017). A higher value of absorbance indicates a higher RP. Tables 2 and 3 show the results of RP assay obtained from WSF and SSF, respectively. In general, WSF after the first step of hydrolysis showed a higher activity as determined by the RP assay than that of SSF. A decrease in antioxidant activity as determined by the RP assay under the influence of two-step in vitro hydrolysis within the WSF and SSF fractions was observed. The exception was the BAUER variants in both fractions, where the differences turned out to be statistically insignificant (P > 0.05). There is no literature data on which sufficiently explained the effect of hydrolysis on the values of RP assay. However, there are reports on the decrease in the values of RP assay with an increase in the degree of hydrolysis for hydrolysate prepared from buckwheat (Tang et al., 2009a) and hemp (Tang et al., 2009b). However, according to Vaštag et al. (2010) there was a correlation between the progress of proteolysis and an increase in the antioxidant potential as determined by RP assay in protein extracts from Petrovská Kolbása during 90 days of ripening. However, according to Park & Chin (2011), the hydrolysis of proteins in WSF and SSF from pork ham by pepsin did not affect the values of RP assay. According to Wu et al. (2003), the RP of free amino acids constitutive of carnosine (i.e. Β-alanine and histidine) was low, but it increased after the formation of the peptide bond. In general, a peptide bond or charged amino and carboxyl groups, or both, appear to participate in the oxidation–reduction mechanism. Thus, the breakdown of the peptide bond during enzymatic hydrolysis seems to be responsible for the loss of the antioxidant capacity of the peptides.
. | C . | LOCK . | BAUER . | BB12 . | Strain of LAB (A) . | Digestion step (B) . | AxB . | . |
---|---|---|---|---|---|---|---|---|
ABTS radical scavenging activity [%] | ||||||||
Gastro digestion | 35.02 ± 2.87Bc | 34.79 ± 2.74Bc | 43.40 ± 1.95Bb | 53.64 ± 2.98Ba | *** | *** | *** | ![]() |
Gastro-pancreatic digestion | 74.36 ± 3.79Ab | 79.35 ± 1.77Aa | 70.48 ± 1.76Ac | 82.91 ± 3.65Aa | ||||
Reducing antioxidant power [A700] | ||||||||
Gastro digestion | 0.433 ± 0.011Ab | 0.523 ± 0.023Aa | 0.093 ± 0.024Ad | 0.331 ± 0.012Ac | *** | *** | *** | ![]() |
Gastro-pancreatic digestion | 0.181 ± 0.036Ba | 0.178 ± 0.012Ba | 0.073 ± 0.016Ac | 0.121 ± 0.014Bb | ||||
Fe2+-chelating activity [%] | ||||||||
Gastro digestion | 55.18 ± 4.50Bb | 57.10 ± 1.13Bb | 86.41 ± 2.89Aa | 91.24 ± 0.19Aa | *** | *** | *** | ![]() |
Gastro-pancreatic digestion | 68.19 ± 4.99Ab | 75.52 ± 0.40Ab | 87.55 ± 3.62Ba | 87.05 ± 1.40Ba |
. | C . | LOCK . | BAUER . | BB12 . | Strain of LAB (A) . | Digestion step (B) . | AxB . | . |
---|---|---|---|---|---|---|---|---|
ABTS radical scavenging activity [%] | ||||||||
Gastro digestion | 35.02 ± 2.87Bc | 34.79 ± 2.74Bc | 43.40 ± 1.95Bb | 53.64 ± 2.98Ba | *** | *** | *** | ![]() |
Gastro-pancreatic digestion | 74.36 ± 3.79Ab | 79.35 ± 1.77Aa | 70.48 ± 1.76Ac | 82.91 ± 3.65Aa | ||||
Reducing antioxidant power [A700] | ||||||||
Gastro digestion | 0.433 ± 0.011Ab | 0.523 ± 0.023Aa | 0.093 ± 0.024Ad | 0.331 ± 0.012Ac | *** | *** | *** | ![]() |
Gastro-pancreatic digestion | 0.181 ± 0.036Ba | 0.178 ± 0.012Ba | 0.073 ± 0.016Ac | 0.121 ± 0.014Bb | ||||
Fe2+-chelating activity [%] | ||||||||
Gastro digestion | 55.18 ± 4.50Bb | 57.10 ± 1.13Bb | 86.41 ± 2.89Aa | 91.24 ± 0.19Aa | *** | *** | *** | ![]() |
Gastro-pancreatic digestion | 68.19 ± 4.99Ab | 75.52 ± 0.40Ab | 87.55 ± 3.62Ba | 87.05 ± 1.40Ba |
A–BWithin the same samples, means followed by the common letter do not differ significantly (P < 0.05);
a–dWithin the same treatment, means followed by the common letter do not differ significantly (P < 0.05).
*P < 0.05.
**P < 0.01.
***P < 0.001.
. | C . | LOCK . | BAUER . | BB12 . | Strain of LAB (A) . | Digestion step (B) . | AxB . | . |
---|---|---|---|---|---|---|---|---|
ABTS radical scavenging activity [%] | ||||||||
Gastro digestion | 35.02 ± 2.87Bc | 34.79 ± 2.74Bc | 43.40 ± 1.95Bb | 53.64 ± 2.98Ba | *** | *** | *** | ![]() |
Gastro-pancreatic digestion | 74.36 ± 3.79Ab | 79.35 ± 1.77Aa | 70.48 ± 1.76Ac | 82.91 ± 3.65Aa | ||||
Reducing antioxidant power [A700] | ||||||||
Gastro digestion | 0.433 ± 0.011Ab | 0.523 ± 0.023Aa | 0.093 ± 0.024Ad | 0.331 ± 0.012Ac | *** | *** | *** | ![]() |
Gastro-pancreatic digestion | 0.181 ± 0.036Ba | 0.178 ± 0.012Ba | 0.073 ± 0.016Ac | 0.121 ± 0.014Bb | ||||
Fe2+-chelating activity [%] | ||||||||
Gastro digestion | 55.18 ± 4.50Bb | 57.10 ± 1.13Bb | 86.41 ± 2.89Aa | 91.24 ± 0.19Aa | *** | *** | *** | ![]() |
Gastro-pancreatic digestion | 68.19 ± 4.99Ab | 75.52 ± 0.40Ab | 87.55 ± 3.62Ba | 87.05 ± 1.40Ba |
. | C . | LOCK . | BAUER . | BB12 . | Strain of LAB (A) . | Digestion step (B) . | AxB . | . |
---|---|---|---|---|---|---|---|---|
ABTS radical scavenging activity [%] | ||||||||
Gastro digestion | 35.02 ± 2.87Bc | 34.79 ± 2.74Bc | 43.40 ± 1.95Bb | 53.64 ± 2.98Ba | *** | *** | *** | ![]() |
Gastro-pancreatic digestion | 74.36 ± 3.79Ab | 79.35 ± 1.77Aa | 70.48 ± 1.76Ac | 82.91 ± 3.65Aa | ||||
Reducing antioxidant power [A700] | ||||||||
Gastro digestion | 0.433 ± 0.011Ab | 0.523 ± 0.023Aa | 0.093 ± 0.024Ad | 0.331 ± 0.012Ac | *** | *** | *** | ![]() |
Gastro-pancreatic digestion | 0.181 ± 0.036Ba | 0.178 ± 0.012Ba | 0.073 ± 0.016Ac | 0.121 ± 0.014Bb | ||||
Fe2+-chelating activity [%] | ||||||||
Gastro digestion | 55.18 ± 4.50Bb | 57.10 ± 1.13Bb | 86.41 ± 2.89Aa | 91.24 ± 0.19Aa | *** | *** | *** | ![]() |
Gastro-pancreatic digestion | 68.19 ± 4.99Ab | 75.52 ± 0.40Ab | 87.55 ± 3.62Ba | 87.05 ± 1.40Ba |
A–BWithin the same samples, means followed by the common letter do not differ significantly (P < 0.05);
a–dWithin the same treatment, means followed by the common letter do not differ significantly (P < 0.05).
*P < 0.05.
**P < 0.01.
***P < 0.001.
. | C . | LOCK . | BAUER . | BB12 . | Strain of LAB (A) . | Digestion step (B) . | AxB . | . |
---|---|---|---|---|---|---|---|---|
ABTS radical scavenging activity [%] | ||||||||
Gastro digestion | 19.27 ± 1.28Bab | 17.44 ± 1.86Bc | 19.42 ± 1.08Bbc | 21.82 ± 2.47Ba | *** | *** | *** | ![]() |
Gastro-pancreatic digestion | 65.03 ± 2.21Ac | 68.69 ± 2.25Ab | 77.19 ± 2.15Aa | 59.98 ± 2.07Ad | ||||
Reducing antioxidant power [A700] | ||||||||
Gastro digestion | 0.103 ± 0.005Ab | 0.121 ± 0.008Aa | 0.102 ± 0.008Ab | 0.101 ± 0.010Ab | *** | *** | *** | ![]() |
Gastro-pancreatic digestion | 0.088 ± 0.006Bb | 0.099 ± 0.006Bb | 0.111 ± 0.012Aa | 0.057 ± 0.006Bc | ||||
Fe2+-chelating activity [%] | ||||||||
Gastro digestion | 84.52 ± 1.02Ac | 86.83 ± 0.29Ab | 86.21 ± 0.36Ab | 90.50 ± 0.60Aa | *** | *** | *** | ![]() |
Gastro-pancreatic digestion | 76.99 ± 0.96Bb | 85.03 ± 0.55Ba | 85.26 ± 0.52Aa | 86.71 ± 2.31Ba |
. | C . | LOCK . | BAUER . | BB12 . | Strain of LAB (A) . | Digestion step (B) . | AxB . | . |
---|---|---|---|---|---|---|---|---|
ABTS radical scavenging activity [%] | ||||||||
Gastro digestion | 19.27 ± 1.28Bab | 17.44 ± 1.86Bc | 19.42 ± 1.08Bbc | 21.82 ± 2.47Ba | *** | *** | *** | ![]() |
Gastro-pancreatic digestion | 65.03 ± 2.21Ac | 68.69 ± 2.25Ab | 77.19 ± 2.15Aa | 59.98 ± 2.07Ad | ||||
Reducing antioxidant power [A700] | ||||||||
Gastro digestion | 0.103 ± 0.005Ab | 0.121 ± 0.008Aa | 0.102 ± 0.008Ab | 0.101 ± 0.010Ab | *** | *** | *** | ![]() |
Gastro-pancreatic digestion | 0.088 ± 0.006Bb | 0.099 ± 0.006Bb | 0.111 ± 0.012Aa | 0.057 ± 0.006Bc | ||||
Fe2+-chelating activity [%] | ||||||||
Gastro digestion | 84.52 ± 1.02Ac | 86.83 ± 0.29Ab | 86.21 ± 0.36Ab | 90.50 ± 0.60Aa | *** | *** | *** | ![]() |
Gastro-pancreatic digestion | 76.99 ± 0.96Bb | 85.03 ± 0.55Ba | 85.26 ± 0.52Aa | 86.71 ± 2.31Ba |
A-BWithin the same samples, means followed by the common letter do not differ significantly (P < 0.05).
a–dWithin the same treatment, means followed by the common letter do not differ significantly (P < 0.05).
*P < 0.05.
**P < 0.01.
***P < 0.001.
. | C . | LOCK . | BAUER . | BB12 . | Strain of LAB (A) . | Digestion step (B) . | AxB . | . |
---|---|---|---|---|---|---|---|---|
ABTS radical scavenging activity [%] | ||||||||
Gastro digestion | 19.27 ± 1.28Bab | 17.44 ± 1.86Bc | 19.42 ± 1.08Bbc | 21.82 ± 2.47Ba | *** | *** | *** | ![]() |
Gastro-pancreatic digestion | 65.03 ± 2.21Ac | 68.69 ± 2.25Ab | 77.19 ± 2.15Aa | 59.98 ± 2.07Ad | ||||
Reducing antioxidant power [A700] | ||||||||
Gastro digestion | 0.103 ± 0.005Ab | 0.121 ± 0.008Aa | 0.102 ± 0.008Ab | 0.101 ± 0.010Ab | *** | *** | *** | ![]() |
Gastro-pancreatic digestion | 0.088 ± 0.006Bb | 0.099 ± 0.006Bb | 0.111 ± 0.012Aa | 0.057 ± 0.006Bc | ||||
Fe2+-chelating activity [%] | ||||||||
Gastro digestion | 84.52 ± 1.02Ac | 86.83 ± 0.29Ab | 86.21 ± 0.36Ab | 90.50 ± 0.60Aa | *** | *** | *** | ![]() |
Gastro-pancreatic digestion | 76.99 ± 0.96Bb | 85.03 ± 0.55Ba | 85.26 ± 0.52Aa | 86.71 ± 2.31Ba |
. | C . | LOCK . | BAUER . | BB12 . | Strain of LAB (A) . | Digestion step (B) . | AxB . | . |
---|---|---|---|---|---|---|---|---|
ABTS radical scavenging activity [%] | ||||||||
Gastro digestion | 19.27 ± 1.28Bab | 17.44 ± 1.86Bc | 19.42 ± 1.08Bbc | 21.82 ± 2.47Ba | *** | *** | *** | ![]() |
Gastro-pancreatic digestion | 65.03 ± 2.21Ac | 68.69 ± 2.25Ab | 77.19 ± 2.15Aa | 59.98 ± 2.07Ad | ||||
Reducing antioxidant power [A700] | ||||||||
Gastro digestion | 0.103 ± 0.005Ab | 0.121 ± 0.008Aa | 0.102 ± 0.008Ab | 0.101 ± 0.010Ab | *** | *** | *** | ![]() |
Gastro-pancreatic digestion | 0.088 ± 0.006Bb | 0.099 ± 0.006Bb | 0.111 ± 0.012Aa | 0.057 ± 0.006Bc | ||||
Fe2+-chelating activity [%] | ||||||||
Gastro digestion | 84.52 ± 1.02Ac | 86.83 ± 0.29Ab | 86.21 ± 0.36Ab | 90.50 ± 0.60Aa | *** | *** | *** | ![]() |
Gastro-pancreatic digestion | 76.99 ± 0.96Bb | 85.03 ± 0.55Ba | 85.26 ± 0.52Aa | 86.71 ± 2.31Ba |
A-BWithin the same samples, means followed by the common letter do not differ significantly (P < 0.05).
a–dWithin the same treatment, means followed by the common letter do not differ significantly (P < 0.05).
*P < 0.05.
**P < 0.01.
***P < 0.001.
The antioxidant peptides may be more effective hydrogen or electron donors after in vitro digestion. Nevertheless, the antioxidant mechanism of antioxidant peptides is still unclear. However, several factors, including MW, structure and hydrophobicity, influence the antioxidant capacity of antioxidant peptides. For example, hydrophobic amino acids are responsible for the reducing force. Also, the degree of hydrolysis will determine the amount and size of the peptides acting as antioxidants.
In this study, a decrease in the antioxidant properties of the hydrolysates was observed after gastro-pancreatic digestion, determined by the RP test. This decrease in reducing ability may be due to the presence of a smaller amount electron donor due to the lower amount of protein products (high degree of hydrolysis) obtained during the 5-hour gastric-pancreatic digestion.
In this study, the observed decrease in the ability of hydrolysis products to give electrons after gastrointestinal digestion was, therefore, most likely due to the specific properties of the enzymes used, resulting in the loss of antioxidant properties, regardless of the analysed fraction as well as the bacterial strains used. According to our results on the assessment of the effect of probiotic strains of LAB, LOCK samples (P < 0.05) exhibited higher and BAUER samples exhibited significantly lower antioxidant activity with respect to the sample subjected to spontaneous fermentation (C) within the WSF fraction. A previous study reported a similar trend in the case of peptides extracted from dry-cured pork meat after 9 months of ageing (Kęska & Stadnik, 2017).
The metal-chelating ability of the compounds can serve as a very important indicator of the potential antioxidant activity of compounds because it has a stabilising effect on the oxidised form of the metal ion by reducing their redox potential (Girgih et al., 2011). Tables 2 and 3 (WSF and SSF, respectively) show ferrous ion chelating ability of low-molecular-weight peptides. The average level of metal-chelating capacity was about 83.45% after two-step hydrolysis in both analysed fractions (except WSF from C samples, 68.19%), which corresponds to the values given in the literature for the dry-cured meat product after simulated gastrointestinal digestion (pepsin/trypsin) (Zhu et al., 2016). More importantly, in this study, the BB12 and BAUER samples were characterised by the highest ferrous ion chelating activity regardless of the analysed fraction and the hydrolysis step. However, the biological activity trend was ambiguous within the analysed variants. In this study, there was an increase in the metal-chelating capacity of WSF after the hydrolysis using the two-step digestion (except for the BB12, Table 2). This observation was not recorded for the SSF, where the value of the analysed parameter generally decreased. Other authors report that chelating activity increases with a decrease in the molecular weight of the peptide (higher degree of cleavage of peptide bonds) due to the effect of enzymatic hydrolysis (Klompong et al., 2007; Jamdar et al., 2010). In this study, the exceptionally high ability of low-molecular-weight peptides in Fe2+-chelating after two-step hydrolysis might be due to the presence of large number of carboxyl and amino groups. Saiga et al. (2003) also found that acidic and basic amino acids may play an important role in the chelation of Fe2+ and Cu2+ ions. The reduction of Fe2+ chelation activity after two-step hydrolysis might be attributed to the disorder of active antioxidant peptide structures after pepsin/pancreatin hydrolysis (Martínez-Palma et al., 2015).
Effect of peptide content on the antioxidant capacity of low-molecular-weight peptides after in vitro gastro-pancreatic digestion
In this study, we calculated Pearson’s correlation coefficient between the PC and the results of antioxidant analysis (ABTS, RP, ferrous ion chelating activity) (Fig. 5). The strongest relationship between PC and the antioxidant test was noted in the ABTS assay. In the case of WSF, after the first step of digestion, a strong positive correlation was noted for the ABTS test for C (R = 0.646) and BAUER (R = 0.788) and a very strong negative correlation for LOCK (R = −0.889). In addition, Liu et al. (2017) observed that the DPPH radical scavenging activity (˜80%) has a positive correlation (R2 = 0.937) with peptide content during the wheat germ fermentation with Bacillus subtilis due to the synergistic effects. After the second step of in vitro enzymatic hydrolysis, the increase in peptide content was not associated with the stronger anti-ABTS radical activity in the samples inoculated with LAB strain. We observed an increase in the antiradical activity in only those samples that had no addition of strains (C); the increase in correlation (R = 0.862) was observed after two-step enzymatic hydrolysis. When we analysed the correlation distribution (Fig. 5), we observed that C and BAUER samples showed similar trends (correlation distributions determined in the Pearson’s correlation test) in the WSF, especially after the first step of hydrolysis.
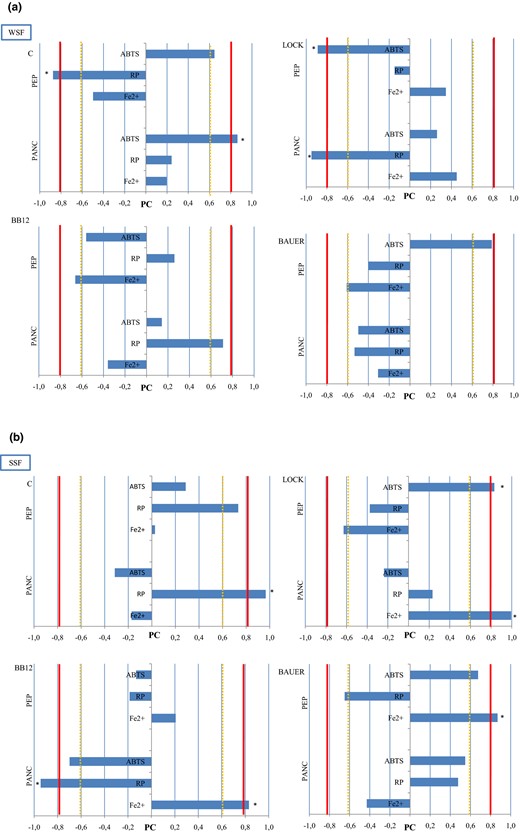
The correlation (Pearson’s correlation coefficient) between peptides content and their antioxidant activity in WSF (a) and SSF (b) obtained from dry-cured pork loin with probiotic strain of LAB after 12 months. The red cut-off line shows a strong correlation (r<0.8), while the yellow line indicates high correlation (r<0.6). Sign * – significance (two-sided) correlation at P < 0.05. PC – peptides content, PEP – samples after pepsin hydrolysis, PAN – samples after pancreatin hydrolysis, C – sample without starter culture; LOCK – sample with probiotic strain Lactobacillus rhamnosus LOCK900; BAUER – sample with potentially probiotic strain Lactobacillus acidophilus Bauer Ł0938; BB12 – sample with probiotic strain Bifidobacterium animals ssp. lactis BB-12; ABTS - antiradical scavenging activity, RP- reducing antioxidant power, FE2+ - chelating activity.
The use of various antioxidant tests to assess the biological activity of peptides
The results of the correlation test between the tests used to determine biological activity are provided in Figure S1. No one specific and strong trends was noted for either WSF or SSF. The influence of the strain as well as the step of hydrolytic breakdown of protein structures into small peptides did not bring an unequivocal answer, which test would be the best to assess the antioxidant potential of meat peptides. The reasons for this state of affairs can be found in the differences in reactive species, mechanisms, conditions, reaction kinetics and methods of quantifying the difficulty in comparing the results of the differences between antioxidant tests. As an example, similar compounds react in both the iron-reducing antioxidant power and ABTS tests, but the reaction pH varies, which can lead to a significant difference in the structure of the same peptide, especially for those containing non-neutral amino acids (Zou et al., 2016).
It should be noted that there was a relationship between ABTS and Fe2+ -chelating activity under the influence of hydrolysis, which suggests a certain similarity of these mechanisms (see Figures S1 and S2). Zhu et al. (2016) observed that the change in the ability to chelate metal ions (Cu2+) and the ability to neutralise ABTS free radical after the in vitro protein digestion was similar suggesting that the two methods may be related. It is possible that the treatment with pepsin disrupted the spatial structure of the peptides, which promoted the binding and trapping of Cu2+ and ABTS+•, resulting in a reduction in chelation capacity (Zhu et al., 2008). However, since the high-affinity metal binding groups were fully exposed or were newly formed via hydrolysis by pancreatic digestion, for example imidazole and carboxyl groups, it is likely that the electrostatic and ionic interactions with metal ions were enhanced, which was also the case in this study, especially in SSF samples from batches with probiotic LAB-directed fermentation (Supplementary material). Furthermore, also Decker et al. (1992) reported that the specific structure of peptides and groups of amino acid side chains played an important role not only in breaking free radical chain reactions but also in chelation of transition metal ions.
In this study, the differences in trends observed for a specific antioxidant test during digestion may be related to a structure–activity relationship. The antioxidant properties of these hydrolysates depend to a large extent on the type of native protein and the operating conditions used to isolate the protein, the specificity of the protease used for hydrolysis, as well as on the degree of hydrolysis. Therefore, all these aspects should be taken into account when selecting the test for the evaluation of antioxidants.
Conclusion
According to our results, dry-cured pork meat, due to the presence of antioxidant peptides, is a good candidate to be utilised as a functional food. In addition, its antioxidant potential can be enhanced by innovative fermentation with LAB. The results of this study indicate that the use of probiotic LAB strains can increase the content of peptides and active antioxidants in the products. This study also found that the antiradical, chelating and metal-reducing activity of the peptides suggest that these products have antioxidant potential that is retained even after the in vitro gastro-pancreatic digestion, but further research is needed to adequately explain the metabolic biotransformation of these biologically active substances in the human body, to enable the assessment of the potential use of dry-cured meat products as a functional food.
Conflicts of interest
The authors declare no conflict of interest.
Author contributions
Paulina Kęska: Conceptualization (equal); Formal analysis (lead); Investigation (lead); Methodology (equal); Writing-original draft (lead); Writing-review & editing (equal). Joanna Stadnik: Conceptualization (equal); Methodology (equal); Writing-review & editing (equal).
Ethical guidelines
Ethics approval was not required for this research.
Data availability statement
The data that support the findings of this study are available from the corresponding author upon reasonable request.
References
This study proves the high stability of ACE inhibitory peptides derived from Spanish dry-cured ham against temperature of processing and gastrointestinal digestion as well as the powerful ACE inhibitory activity of some of the peptides identified in Spanish dry-cured ham. ACE inhibitory activity keeps constant probably because of the presence of the identified antihypertensive peptides as well as small fragments resulting from their degradation occurred after processing and in vitro digestion.
This paper focuses on the major intrinsic non-enzymatic antioxidants, including metal binding proteins, glutathione, uric acid, melatonin, bilirubin and polyamines. This review summarizes the roles and functions of some of the most important internal antioxidants in the human body.
This study provides the first report that acidic peptides as well as basic peptides possess high antioxidant activity. The isolated peptides contained mainly not basic amino acids but mainly acidic amino acid residues.
This study reports for the first time the antioxidant activity of peptides derived from Jinhua dry-cured ham and the antioxidant activity of peptides in the GI digestion system. Obtained results proved that Jinhua dry-cured ham may be a good potential source of antioxidant peptides naturally generated during the dry-curing process and through GI digestion.