-
PDF
- Split View
-
Views
-
Cite
Cite
Viktoria Gusarova, Serena Banfi, Corey A. Alexa-Braun, Lisa M. Shihanian, Ivory J. Mintah, Joseph S. Lee, Yurong Xin, Qi Su, Vishal Kamat, Jonathan C. Cohen, Helen H. Hobbs, Brian Zambrowicz, George D. Yancopoulos, Andrew J. Murphy, Jesper Gromada, ANGPTL8 Blockade With a Monoclonal Antibody Promotes Triglyceride Clearance, Energy Expenditure, and Weight Loss in Mice, Endocrinology, Volume 158, Issue 5, 1 May 2017, Pages 1252–1259, https://doi.org/10.1210/en.2016-1894
- Share Icon Share
Abstract
Angiopoietin-like protein (ANGPTL)8 is a negative regulator of lipoprotein lipase–mediated plasma triglyceride (TG) clearance. In this study, we describe a fully human monoclonal antibody (REGN3776) that binds monkey and human ANGPTL8 with high affinity. Inhibition of ANGPTL8 with REGN3776 in humanized ANGPTL8 mice decreased plasma TGs and increased lipoprotein lipase activity. Additionally, REGN3776 reduced body weight and fat content. The reduction in body weight was secondary to increased energy expenditure. Finally, single administration of REGN3776 normalized plasma TGs in dyslipidemic cynomolgus monkeys. In conclusion, we show that blockade of ANGPTL8 with monoclonal antibody strongly reduced plasma TGs in mice and monkeys. These data suggest that inhibition of ANGPTL8 may provide a new therapeutic avenue for the treatment of dyslipidemia with beneficial effects on body weight.
Angiopoietin-like protein (ANGPTL)8 acts by inhibiting lipoprotein lipase (LPL) activity and thereby plasma triglyceride (TG) clearance. Hepatic overexpression of ANGPTL8 in mice is associated with hypertriglyceridemia, whereas genetic inactivation of Angptl8 reduced plasma TGs (1, 2). ANGPTL8 is expressed in liver and adipose tissue and its expression is highly upregulated by feeding (1, 3, 4). For this reason, it has been proposed that ANGPTL8 contributes to TG handling during refeeding and directs fatty acids to adipose tissue for storage (2). Consistent with this, Angptl8−/− mice have reduced body weight and fat mass when compared with control mice (2). In this study, we show that ANGPTL8 blockade with a fully human monoclonal antibody decreased plasma TGs and reduced body weight and fat content in mice. These data suggest that ANGPTL8 inhibition is a potential target for the treatment of hypertriglyceridemia with additional benefits on fat content and body weight.
Materials and Methods
Antibodies and protein reagents
REGN3776 was derived using Regeneron’s VelocImmune technology platform (5, 6) and is a fully human monoclonal antibody with high affinity to ANGPTL8 from monkeys and humans. REGN3776 has a human immunoglobulin G (IgG)4 constant region with a stabilizing mutation in the hinge region (serine to proline in position 108 in Genbank no. P01864) to minimize half-antibody formation (7). An isotype-matched antibody with irrelevant specificity was used as control.
Binding studies
The binding of REGN3776 to human and monkey ANGPTL8 was studied using a surface plasmon resonance imaging–based MASS-1 biosensor in 10 mM HEPES (pH 7.4), 150 mM NaCl, 3 mM EDTA, and 0.05% volume-to-volume ratio surfactant Tween 20 (HBS-ET) running buffer at 25°C. The High capacity amine sensor surface was first amine coupled with goat anti-mouse IgG2a polyclonal antibody (SouthernBiotech, no. 1080-01), and ∼30 resonance units of human ANGPTL8 expressed with C-terminal mouse IgG2a Fc tag (hANGPTL8-mFc) or monkey ANGPTL8 expressed with C-terminal mouse IgG2a Fc tag (mfANGPTL8-mFc) was captured. Different concentrations of REGN3776, prepared in HBS-ET running buffer, were later injected over hANGPTL8-mFc or mfANGPTL8-mFc captured surfaces for 4 minutes at a flow rate of 30 µL/min followed by the dissociation of bound monoclonal antibody in HBS-ET running buffer for 10 minutes. Injection of running buffer without analyte was performed to allow subtraction of baseline drift resulting from the natural dissociation of captured monoclonal antibody from the coupled goat anti-mouse IgG2a polyclonal antibody surface. The raw binding data were aligned to the start of the analyte injection followed by subtraction from the reference flow cell. The processed data were later analyzed using Scrubber v2.0c (BioLogic Software), wherein the second reference subtraction using control buffer injections was performed. The association rate, dissociation rate, and dissociation constant were calculated by globally fitting the double reference subtracted binding data to a 1:1 Langmuir binding model with mass transport limitation using Scrubber v2.0c software.
Studies in mice
Humanized ANGPTL8 mice (Angptl8hum/hum mice) were generated on a 75% C57Bl/6 and 25% 129S6/SvEv background using VelociGene technology (8). The VelociGene allele identification number is VG7182. All procedures were conducted in compliance with protocols approved by the Regeneron Pharmaceuticals Institutional Animal Care and Use Committee. REGN3776 and control antibody were diluted with sterile phosphate-buffered saline for subcutaneous (SC) injection into mice.
Single administration studies
Male Angptl8hum/hum mice were maintained on chow diet (5001, LabDiet) and fed ad libitum. To establish a baseline for serum chemistry parameters, serum samples were collected 7 days prior to antibody administration in the nonfasted state. On study day 0, mice were sorted into treatment groups based on their serum TG levels. Mice (n = 5 per group) (ad libitum) were administered single SC injections of REGN3776 or control antibody at the indicated doses. Subsequent serum samples were collected from nonfasted mice during the duration of the study and analyzed for serum chemistry parameters and human ANGPTL8 levels. Post-heparin plasma LPL activity was measured 4 days after single administration of REGN3776 (10 mg/kg). Post-heparin plasma was fractionated on a heparin column to separate hepatic lipase (HL) and LPL as described previously (2). TG hydrolase activity was measured using EnzCheck substrate (Thermo Fisher Scientific) as described elsewhere (9). Lipid tolerance tests were performed 4 days after a single dose of REGN3776 (10 mg/kg). Mice were fasted for 2 hours before intravenous administration of 20% Intralipid (Baxter Healthcare) at 2.5 μL/g body weight. TGs were evaluated in blood collected from the tail at subsequent time points.
Multiple administration studies
Seven days prior to antibody administration (day −7), baseline serum chemistries were measured in nonfasted animals. On day −6, male Angptl8hum/hum mice were placed on a high-fat, high-cholesterol (HFHC) diet (21% fat, 0.21% cholesterol; Research Diets). On study day 0, mice were sorted into treatment groups based on their serum TGs and body weights (n = 8 per group). Mice were injected with REGN3776 or control antibody once a week (SC, 10 mg/kg) for 16 weeks. Body weights were measured weekly. Body composition and metabolic analysis was evaluated at 15 and 14 weeks, respectively. Mice were euthanized 1 week after the last antibody injection, and liver, heart, muscle, and white and brown adipose tissues were collected.
Serum chemistry analyses
Serum TG, total cholesterol (TC), low-density lipoprotein cholesterol (LDL-C) and high-density lipoprotein cholesterol (HDL-C) levels were determined in serum using an Advia 1800 blood chemistry analyzer (Bayer). Lipoprotein separation by high-performance liquid chromatography (HPLC) was performed as described previously (10).
Body composition, metabolic rate, and food intake measurements
Body composition was measured with X-ray microcomputed tomography using the Quantum FX microcomputed tomography preclinical in vivo imaging system (Caliper Life Sciences) as described elsewhere (11). Metabolic cage data were generated using the Oxymax laboratory animal monitoring system (CLAMS; Columbus Instruments). Mice were individually monitored in cages with center feeds for 96 hours. Data generated in the first 24 hours were omitted from the analysis. Food intake was measured continuously and divided into calories consumed per light and dark phase of the light cycle. Oxygen consumption and carbon dioxide production were measured in 17-minute intervals during a 4-day span and plotted over time in hours. Energy expenditure was calculated as a function of the respiratory quotient and the oxygen consumption, normalized to body weight. Additionally, as the groups had divergent body weights at the time of analysis, energy expenditure was expressed as kilocalories per hour per mouse using an adjusted mean body weight of the two groups combined. This was achieved using analysis of covariance with body weight as the covariance, as described (12, 13). Briefly, the adjusted energy expenditure for each animal was calculated as Y = y − b(x − X) and plotted as mean ± standard error of the mean (SEM) for each group, where Y is single animal adjusted energy expenditure (kcal/h), y is single animal energy expenditure (kcal/h), x is single animal body weight (kg), X is adjusted mean body weight of the two treatment groups combined (kg), and b is the slope of the line of energy expenditure plotted vs body weight for each animal and each treatment group calculated by linear regression analysis.
Study in cynomolgus monkeys
The study was performed at Crown Bioscience. Eighteen spontaneous hypertriglyceridemic monkeys were selected based on their nonfasted serum TG levels and divided into three groups. The monkeys were individually housed, had free access to water, and were fed twice daily with a complete nutritionally balanced diet (Shanghai Shilin Biotechnology), enriched with seasonal fruits and vegetables. All animal procedures were approved by the Crown Bioscience Institutional Animal Care and Use Committee and performed according to guidelines approved by the Association for Assessment and Accreditation of Laboratory Animal Care. On day 0 the monkeys were administrated REGN3776 at 3, 7, or 10 mg/kg. Blood (4 mL) was collected into BD sterile venous blood collection tubes (Accu-Chek Active; Roche) from nonfasted animals at 1- to 5-day intervals up to day 45. After TG levels for all animals returned to baseline, animals were allowed to washout for at least 2 weeks, and then five animals were selected for the treatment with saline. Blood was collected on consecutive days after saline administration on the same schedule as the REGN3776-injected groups. Serum TG, TC, LDL-C, and HDL-C levels were measured by an Advia 2400 system (Siemens).
Data analysis
All data are shown as mean ± SEM. Statistical analyses were performed utilizing GraphPad Prism 6.0 software. LPL and HL activities in REGN3776 and control antibody-treated mice were compared by a Welch t test. All other parameters were analyzed by two-way analysis of variance (ANOVA) with repeated measures. If a significant F ratio was obtained with two-way ANOVA, post hoc analysis was conducted between groups with a Bonferroni or Sidak posttest. In the monkey study, the average of each parameter on day −15, −7, and 0 was used as the baseline value.
Results
In vitro characterization of ANGPTL8 blocking antibody
The relative affinities of ANGPTL8 blocking antibody REGN3776 to human, mouse, and monkey ANGPTL8 were compared using surface plasmon resonance. REGN3776 binds human and monkey ANGPTL8 with comparable affinities (Table 1; Supplemental Fig. 1). REGN3776 does not bind mouse ANGPTL8 or human or mouse ANGPTL3 or ANGPTL4 (Table 1).
Summary of Binding Kinetic Parameters for the Interaction of REGN3776 With hANGPTL8-mFc or mfANGPTL8-mFc
Capture Surface . | Kinetic Binding Parameters . | |||
---|---|---|---|---|
ka (M−1 s−1) . | kd (s−1) . | KD (M) . | t1/2 (min) . | |
hANGPTL8-mFc | 8.5 × 104 | 1 × 10−5a | ≤1.17 × 10−10 | ≥1155 |
mfANGPTL8-mFc | 1.16 × 105 | 1 × 10−5a | ≤8.6 × 10−11 | ≥1155 |
mANGPTL8-mmH | NB | NB | NB | NB |
hANGPTL3-His10 | NB | NB | NB | NB |
mANGPTL3-His6 | NB | NB | NB | NB |
hANGPTL4-His6 | NB | NB | NB | NB |
mANGPTL4-His6 | NB | NB | NB | NB |
Capture Surface . | Kinetic Binding Parameters . | |||
---|---|---|---|---|
ka (M−1 s−1) . | kd (s−1) . | KD (M) . | t1/2 (min) . | |
hANGPTL8-mFc | 8.5 × 104 | 1 × 10−5a | ≤1.17 × 10−10 | ≥1155 |
mfANGPTL8-mFc | 1.16 × 105 | 1 × 10−5a | ≤8.6 × 10−11 | ≥1155 |
mANGPTL8-mmH | NB | NB | NB | NB |
hANGPTL3-His10 | NB | NB | NB | NB |
mANGPTL3-His6 | NB | NB | NB | NB |
hANGPTL4-His6 | NB | NB | NB | NB |
mANGPTL4-His6 | NB | NB | NB | NB |
Abbreviations: hANGPTL3-His10, full-length human ANGPTL3 with a C-terminal decahistidine tag; hANGPTL4-His6, full-length mouse ANGPTL4 with a C-terminal hexahistidine tag; ka, association rate; kd, dissociation rate; KD, dissociation constant; mANGPTL3-His6, full-length mouse ANGPTL3 with a C-terminal hexahistidine tag; mANGPTL4-His6, full-length mouse ANGPTL4 with a C-terminal hexahistidine tag; mANGPTL8-mmH, human ANGPTL8 with a C-terminal myc-myc-hexahistidine tag; mfANGPTL8-mmH, monkey ANGPTL8 with a C-terminal mFc tag; NB, no detectable binding under the assay conditions; t1/2, half life.
No dissociation of REGN3776 was observed from hANGPTL8-mFc or mfANGPTL8-mFc captured surfaces, and the kd value was manually fixed to 1 × 105 seconds−1 while fitting the binding curves.
Summary of Binding Kinetic Parameters for the Interaction of REGN3776 With hANGPTL8-mFc or mfANGPTL8-mFc
Capture Surface . | Kinetic Binding Parameters . | |||
---|---|---|---|---|
ka (M−1 s−1) . | kd (s−1) . | KD (M) . | t1/2 (min) . | |
hANGPTL8-mFc | 8.5 × 104 | 1 × 10−5a | ≤1.17 × 10−10 | ≥1155 |
mfANGPTL8-mFc | 1.16 × 105 | 1 × 10−5a | ≤8.6 × 10−11 | ≥1155 |
mANGPTL8-mmH | NB | NB | NB | NB |
hANGPTL3-His10 | NB | NB | NB | NB |
mANGPTL3-His6 | NB | NB | NB | NB |
hANGPTL4-His6 | NB | NB | NB | NB |
mANGPTL4-His6 | NB | NB | NB | NB |
Capture Surface . | Kinetic Binding Parameters . | |||
---|---|---|---|---|
ka (M−1 s−1) . | kd (s−1) . | KD (M) . | t1/2 (min) . | |
hANGPTL8-mFc | 8.5 × 104 | 1 × 10−5a | ≤1.17 × 10−10 | ≥1155 |
mfANGPTL8-mFc | 1.16 × 105 | 1 × 10−5a | ≤8.6 × 10−11 | ≥1155 |
mANGPTL8-mmH | NB | NB | NB | NB |
hANGPTL3-His10 | NB | NB | NB | NB |
mANGPTL3-His6 | NB | NB | NB | NB |
hANGPTL4-His6 | NB | NB | NB | NB |
mANGPTL4-His6 | NB | NB | NB | NB |
Abbreviations: hANGPTL3-His10, full-length human ANGPTL3 with a C-terminal decahistidine tag; hANGPTL4-His6, full-length mouse ANGPTL4 with a C-terminal hexahistidine tag; ka, association rate; kd, dissociation rate; KD, dissociation constant; mANGPTL3-His6, full-length mouse ANGPTL3 with a C-terminal hexahistidine tag; mANGPTL4-His6, full-length mouse ANGPTL4 with a C-terminal hexahistidine tag; mANGPTL8-mmH, human ANGPTL8 with a C-terminal myc-myc-hexahistidine tag; mfANGPTL8-mmH, monkey ANGPTL8 with a C-terminal mFc tag; NB, no detectable binding under the assay conditions; t1/2, half life.
No dissociation of REGN3776 was observed from hANGPTL8-mFc or mfANGPTL8-mFc captured surfaces, and the kd value was manually fixed to 1 × 105 seconds−1 while fitting the binding curves.
ANGPTL8 antibody blockade lowers serum TGs via upregulation of LPL activity
To evaluate the effect of human ANGPTL8 antibody on serum lipid levels, we generated humanized ANGPTL8 mice (Angptl8hum/hum). These mice had slightly increased baseline TGs with other lipids similar to wild-type mice (Supplemental Fig. 2). Single administration of REGN3776 (10 mg/kg) to chow-fed Angptl8hum/hum mice significantly reduced circulating TGs compared with control antibody treatment [Fig. 1(a)], but it did not affect TC levels [Fig. 1(b)]. HPLC separation of serum lipids confirmed that ANGPTL8 inhibition reduced very-low-density lipoprotein TG [Fig. 1(c)]. The reduction in TG was sustained for 14 days and was associated with a marked increase in plasma levels of ANGPTL8 [Fig. 1(e)]. We evaluated the effects of increasing doses of REGN3776 on circulating TGs in chow-fed Angptl8hum/hum mice. Single administration of REGN3776 at 5, 10, and 25 mg/kg caused a progressive reduction in TGs up to 60% of control levels [Fig. 1(f)].
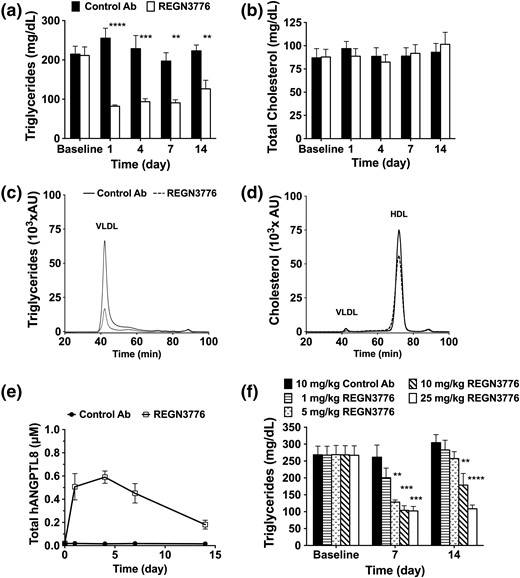
REGN3776 reduces serum TG in Angptl8hum/hum mice. Serum samples were collected from nonfasted male Angptl8hum/hum mice (n = 5 per group) 7 days before (baseline) and at the indicated days following a single SC injection of REGN3776 or control antibody (10 mg/kg). Serum TG (a) and cholesterol (b) were measured enzymatically. Serum from each mouse collected 7 days after treatment with REGN3776 or control antibody was size fractionated by HPLC. TG (c) and cholesterol (d) were measured in each fraction. (e) Total (free plus antibody-bound) serum levels of human ANGPTL8 were measured by enzyme-linked immunosorbent assay. (f) Dose-dependent lowering of serum TGs by REGN3776 in chow-fed Angptl8hum/hum mice measured 7 and 14 days after antibody administration. Values are mean ± standard error of the mean, except for the chromatograms, where only mean values are shown. Statistical analysis was conducted by a two-way analysis of variance with a Sidak correction posttest. **P < 0.01, ***P < 0.001, ****P < 0.0001.
It was previously reported that Angptl8−/− mice increased TG clearance via upregulation of post-heparin plasma LPL activity (2). Consistent with this, we found that ANGPTL8 blockade with REGN3776 increased post-heparin plasma LPL activity without changes in HL activity [Fig. 2(a)]. The increase in LPL plasma activity improved lipid tolerance in Angptl8hum/hum mice receiving REGN3776 [Fig. 2(b)]. These data show that ANGPTL8 blockade with monoclonal antibody lowered plasma TGs through upregulation of LPL activity.
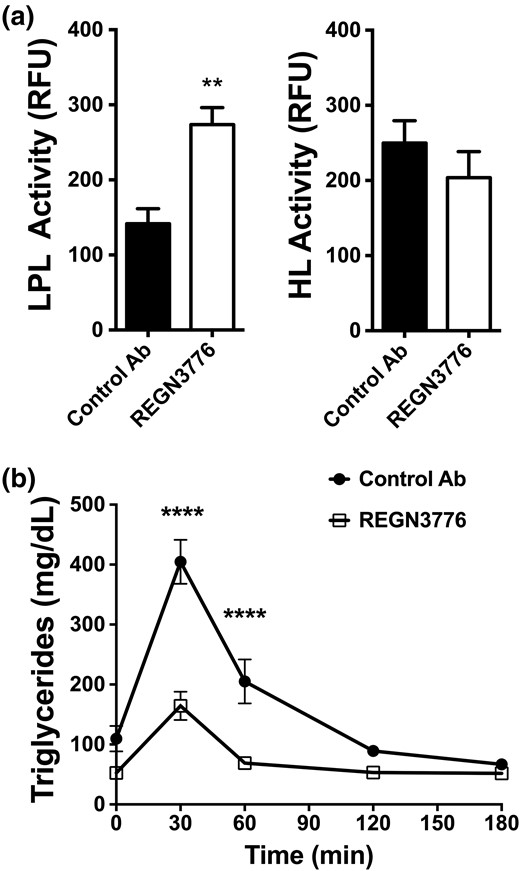
REGN3776 increases LPL activity and improves TG clearance in Angptl8hum/hum mice. (a) Post-heparin plasma LPL and HL activity of chow-fed Angptl8hum/hum mice treated with REGN3776 or control antibody (10 mg/kg, n=5/group). Post-heparin plasma was pooled and fractionated on a heparin column to separate HL and LPL, and TG hydrolase activity was measured. (b) Effect of REGN3776 treatment on plasma TG levels following a lipid tolerance test. Male Angptl8hum/hum mice were treated with REGN3776 or control antibody (10 mg/kg, n = 5 per group) 4 days prior to the intravenous administration of Intralipid (2.5 µL/g of 20% Intralipid). Values are mean ± SEM. Statistical analysis was conducted by a Welch t test (a) and repeated measures two-way ANOVA with a Bonferroni’s posttest (b). **P < 0.01, ****P < 0.0001.
ANGPTL8 antibody inhibition increases energy expenditure and reduces fat content and body weight in Angptl8hum/hum mice
The effects of prolonged inhibition of ANGPTL8 on energy expenditure, body composition, and body weight were evaluated in Angptl8hum/hum mice on an HFHC diet. Weekly administration of REGN3776 (10 mg/kg) for 16 weeks caused marked and sustained reductions in postprandial circulating TGs compared with control antibody [Fig. 3(a)]. ANGPTL8 antibody also caused a gradual body weight reduction that reached statistical significance at week 12 [Fig. 3(b)]. The reduction in body weight correlated with reduced body fat volume as determined by X-ray microcomputed tomography [Fig. 3(c)]. No change in the volume of lean tissue was detected.
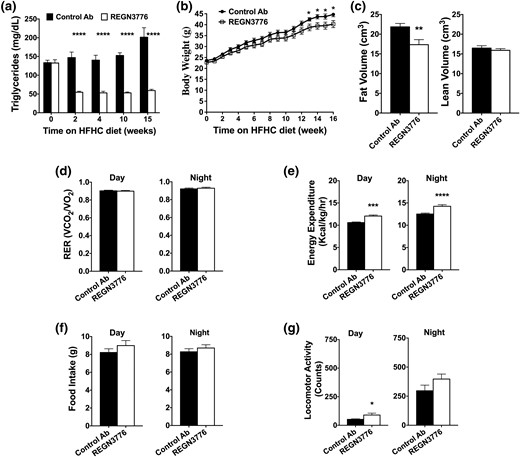
Multiple dose administration of REGN3776 reduces serum TGs, body weight, and fat content and increases energy expenditure in Angptl8hum/hum mice. Serum samples were collected from male Angptl8hum/hum mice (n = 8 per group, 7 weeks old) in nonfasted state. Thereafter, the mice were placed on an HFHC diet. Seven days later, the mice received weekly SC injections of REGN3776 or control antibody (10 mg/kg). Serum samples were collected nonfasted 6 days after each injection. (a) Changes in TG measured over the course of the study. (b) Body weights were monitored weekly. (c) Body composition was measured at 15 weeks. Respiratory exchange ratio (RER) (d), energy expenditure (e), food intake (f), and locomotor activity (g) were measured during dark and light cycles in REGN3776 and control antibody-treated mice. All values are mean ± SEM. Statistical analysis was conducted by repeated measures two-way ANOVA with a Bonferroni correction posttest (a and b) or a Welch t test (c–g). *P < 0.05, **P < 0.01, ***P < 0.001, ****P < 0.0001.
Metabolic cage analysis at week 14 revealed that mice treated with REGN3776 had increased O2 consumption and CO2 production (Supplemental Fig. 3A and 3B). REGN3776 treatment also led to an increase in energy expenditure that was either normalized to body weight [Fig. 3(e)] or calculated without body weight normalization using analysis of covariance with body weight as the covariance (Supplemental Fig. 3C and 3D). Antibody treatment did not affect the respiratory exchange ratio or food intake, but it tended to increase locomotor activity [Fig. 3(d), 3(f), and 3(g)]. The raw traces for all metabolic cage data are shown in Supplemental Fig. 4. The core body temperature remained unchanged by REGN3776 treatment (Supplemental Fig. 5A). REGN3776 did not alter TG content in the liver, heart, muscle, and brown and white adipose tissues (Supplemental Fig. 5B). Consistent with data in Angptl8−/− mice (2, 14), we did not observe an effect of REGN3776 on glucose homeostasis (Supplemental Fig. 6). These data show that ANGPTL8 inhibition reduced body fat content and body weight secondary to increased energy expenditure.
REGN3776 modulates serum TG and HDL-C in dyslipidemic cynomolgus monkeys
Finally, we evaluated the effects of REGN3776 on serum lipids in spontaneous dyslipidemic cynomolgus monkeys. Groups of six monkeys were given a single dose of REGN3776 at 3, 7, or 10 mg/kg. A group of monkeys was selected at the end of the washout period to receive the vehicle. REGN3776 produced robust and sustained dose-dependent reduction in circulating TGs that reached a maximum of −65% (from 323 ± 20 mg/dL to 114 ± 21 mg/dL; P < 0.0001; n = 6) within 1 day following antibody administration [Fig. 4(a) and 4(d)]. Interestingly, all doses resulted in same maximum reduction in TGs; however, the duration of the effects was dose-dependent [Fig. 4(a)]. Inhibition of ANGPTL8 also increased the levels of HDL-C by 30% at all doses [Fig. 4(b) and 4(e)]. No changes were observed in LDL-C [Fig. 4(c) and 4(f)].
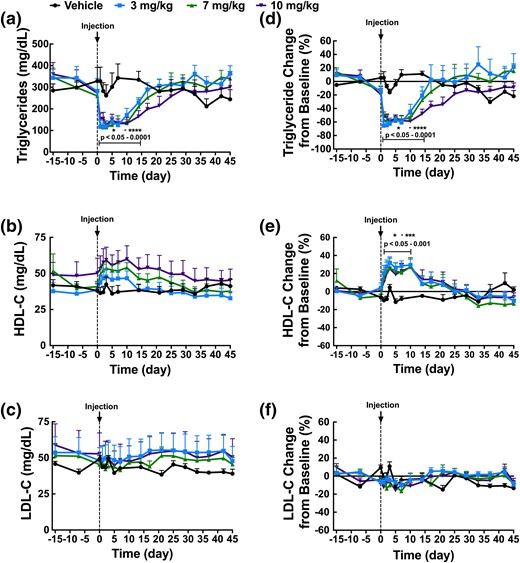
Single-dose administration of REGN3776 to spontaneous hypertriglyceridemic cynomolgus monkeys led to a reduction in plasma TGs and an increase in HDL-C. Baseline serum samples were collected at days −15, −7, and 0 from nonfasted animals. Eighteen monkeys were divided into three groups and administrated REGN3776 (3, 7, or 10 mg/kg). Saline was administered to six monkeys. Serum samples were collected at multiple days and analyzed for TGs (a), HDL-C (b), and LDL-C (c) and also represented as a percentage change from baseline: TGs (d), HDL-C (e), and LDL-C (f). All values are mean ± SEM. Statistical analyses were performed by repeated measures two-way ANOVA with a Sidak posttest. *P < 0.05, **P < 0.01, ***P < 0.001, ****P < 0.0001.
Discussion
In this study, we show that ANGPTL8 blockade with the monoclonal antibody REGN3776 efficiently reduced circulating TGs through upregulation of LPL activity, which is consistent with the phenotype reported in Angptl8−/− animals (2, 14). Additionally, REGN3776 reduced fat accumulation and body weight gain in mice when placed on an HFHC diet. The reduction in body weight was secondary to increased energy expenditure. Moreover, ANGPTL8 blockade in spontaneous hypertriglyceridemic cynomolgus monkeys markedly reduced serum TGs and increased HDL-C. These data suggest that inhibition of ANGPTL8 with a monoclonal antibody may be beneficial for patients with hypertriglyceridemia and obesity.
Antibody inhibition of ANGPTL8 was recently reported to reduce TGs in normolipidemic C57Bl/6 mice by 30%, but required three daily administrations at 30 mg/kg (15). The present study describes the development of a fully human monoclonal antibody to ANGPTL8 that effectively reduced circulating TGs at a single dose of ≤5 mg/kg by 65% in mice and monkeys. ANGPTL8 inhibition reduced plasma TGs through disinhibition of LPL activity. ANGPTL8 antibody inhibition in the cynomolgus dyslipidemic monkeys also increased HDL-C by 30%, consistent with observations reported in human loss of function mutations in ANGPTL8 (16).
During refeeding, LPL activity is upregulated in white adipose tissue and reduced in heart and skeletal muscle (17). Deletion of Angptl8 in mice disrupts these changes in LPL activity; no postprandial reduction in LPL activity occurs in oxidative tissues with refeeding in these mice. As a consequence, TG is delivered to oxidative tissues rather than to adipose tissue. These data suggested that ANGPTL8 acts as a metabolic switch to redirect fatty acids from oxidative tissues to white adipose tissue after food intake (2).
Chronic inhibition of ANGPTL8 with multiple doses of REGN3776 reduced body weight gain and fat accumulation in Angptl8hum/hum mice on an HFHC diet. The body weight reduction in REGN3776-treated mice is likely to result from increased energy expenditure. REGN3776 treatment did not change the respiratory exchange ratio, indicating that it does not alter fuel utilization. Reductions in body weight and adipose tissue mass were reported earlier in chow-fed Angptl8−/− mice (2). Thus, ANGPTL8 is an important regulator of TG storage in white adipose tissue. Additional studies are required to more precisely define the mechanisms responsible for the weight loss in these animals.
In conclusion, we found that ANGPTL8 inhibition with monoclonal antibody REGN3776 reduced circulating TGs in mice and monkeys through upregulation of LPL activity. Multiple dose administration of ANGPTL8 antibody to Angptl8hum/hum mice on an HFHC diet reduced body weight and fat content. Our data suggest that ANGPTL8 inhibition with a monoclonal antibody may be beneficial in patients with hypertriglyceridemia and obesity.
Abbreviations:
- ANGPTL
angiopoietin-like protein
- ANOVA
analysis of variance
- HFHC
high-fat, high-cholesterol
- IgG
immunoglobulin G
- hANGPTL8-mFc
human angiopoietin-like protein 8 expressed with C-terminal mouse immunoglobulin G2a Fc tag
- HBS-ET
10 mM HEPES (pH 7.4), 150 mM NaCl, 3 mM EDTA, and 0.05% volume-to-volume ratio surfactant Tween 20
- HDL-C
high-density lipoprotein cholesterol
- HL
hepatic lipase
- HPLC
high-performance liquid chromatography
- LDL-C
low-density lipoprotein cholesterol
- LPL
lipoprotein lipase
- mfANGPTL8-mFc
monkey angiopoietin-like protein 8 expressed with C-terminal mouse IgG2a Fc tag
- SC
subcutaneous(ly)
- SEM
standard error of the mean
- TC
total cholesterol
- TG
triglyceride.
Acknowledgments
We thank Sandra Kleiner for productive discussion of metabolic cage analysis data.
This work was supported by National Institutes of Health Grant P01 HL20948.
Disclosure Summary: V.G., C.A.A-B., L.M.S., I.J.M., J.S.L., Y.X., Q.S., V.K., B.Z., G.D.Y., A.J.M., and J.G. are employees and shareholders of Regeneron Pharmaceuticals. The remaining authors have nothing to disclose.
References
Author notes
Address all correspondence and requests for reprints to: Viktoria Gusarova, PhD, Regeneron Pharmaceuticals, Inc., 777 Old Saw Mill River Road, Tarrytown, New York 10591. E-mail: [email protected].