-
PDF
- Split View
-
Views
-
Cite
Cite
Narender Kumar, Jerôme Fagart, Philippe Liere, Scott J. Mitchell, Alanah R. Knibb, Isabelle Petit-Topin, Marion Rame, Martine El-Etr, Michael Schumacher, Jeremy J. Lambert, Marie-Edith Rafestin-Oblin, Regine Sitruk-Ware, Nestorone® as a Novel Progestin for Nonoral Contraception: Structure-Activity Relationships and Brain Metabolism Studies, Endocrinology, Volume 158, Issue 1, 1 January 2017, Pages 170–182, https://doi.org/10.1210/en.2016-1426
- Share Icon Share
Abstract
Nestorone® (NES) is a potent nonandrogenic progestin being developed for contraception. NES is a synthetic progestin that may possess neuroprotective and myelin regenerative potential as added health benefits. In receptor transactivation experiments, NES displayed greater potency than progesterone to transactivate the human progesterone receptor (PR). This was confirmed by docking experiments where NES adopts the same docking position within the PR ligand-binding domain (LBD) as progesterone and forms additional stabilizing contacts between 17α-acetoxy and 16-methylene groups and PR LBD, supporting its higher potency than progesterone. The analog 13-ethyl NES also establishes similar contacts as NES with Met909, leading to comparable potency as NES. In contrast, NES is not stabilized within the human androgen receptor LBD, leading to negligible androgen receptor transactivation. Because progesterone acts in the brain by both PR binding and indirectly via binding of the metabolite allopregnanolone to γ-aminobutyric acid type A receptor (GABAAR), we investigated if NES is metabolized to 3α, 5α-tetrahydronestorone (3α, 5α-THNES) in the brain and if this metabolite could interact with GABAAR. In female mice, low concentrations of reduced NES metabolites were identified by gas chromatography/mass spectrometry in both plasma and brain. Electrophysiological studies showed that 3α, 5α-THNES exhibited only limited activity to enhance GABAAR-evoked responses with WSS-1 cells and did not modulate synaptic GABAARs of mouse cortical neurons. Thus, the inability of reduced metabolite of NES (3α, 5α-THNES) to activate GABAAR suggests that the neuroprotective and myelin regenerative effects of NES are mediated via PR binding and not via its interaction with the GABAAR.
Nestorone® (16-methylene-17α-acetoxy-19-nor-pregn-4-ene-3, 20-dione, also known as ST1435) (NES) belongs to the group of “19-nor pregnanes” (1, 2). It has been evaluated as a contraceptive agent and is also being considered as a potential progestin for use in hormone replacement therapy. NES has been synthesized with the objective of creating a highly specific progestin, with selective binding to the progesterone receptor (PR). NES exerts potent progestational and antiestrogenic actions on the uterus and does not interact with estrogen or androgen receptors (1, 3). NES is inactive when given orally to humans or animals (4–7) but is very potent when delivered parenterally, with a progestational activity 100 times higher than that of progesterone (1, 3). Low doses of NES have been found to be very effective in preventing ovulation, allowing its development as a contraceptive in humans in different nonoral delivery systems such as silastic implants, vaginal ring, and transdermal gel (8–11).
Synthetic steroidal progestins are structurally related to testosterone and characterized by a 17β-hydroxyl group (e.g., norethindrone and levonorgestrel) or to the natural hormone progesterone and harbor a 17β-methyl ketone function (e.g., NES). They act by binding to and transactivating PR, a transcription factor belonging to the nuclear receptor superfamily.
Because different progestins differ in their progestational and antiestrogenic activity, we used different approaches to assess the progestational activity of NES and its lack of androgenic effect as compared with levonorgestrel (LNG). In previous experiments, we showed that NES was active in stimulating neuroprotection and neuroregeneration (12, 13) and myelin repair (14–16), and these effects were shown to be exerted via the PR (13, 17). However, we wanted to determine whether part of this neuro-regenerative activity could be mediated via one of the reduced metabolites of NES as exemplified by allopregnanolone [5α-pregnan-3α-ol-20-one; 3α, 5α-tetrahydroprogesterone (3α, 5α-THPROG)], a neuroactive metabolite of progesterone in the brain (17, 18).
Numerous biochemical studies have established a relationship between the chemical structure of steroidal ligands and their ability to transactivate PR and androgen receptor (AR). Moreover, X-ray crystal structures of the ligand-binding domain of PR and AR complexed with various ligands have provided insight into the ligand binding mode (19–22). Our aim was to characterize the activity of NES, a progesterone-related progestin, and its potent analog 13-ethyl NES derivative (23) on PR and AR and to identify the binding contacts responsible for their increased potency and those involved in their selectivity. 13-Ethyl NES was previously synthesized by Gedeon Richter (Hungary) to compare its oral bioactivity with NES. Here, we used 13-ethyl NES to compare PR and AR binding with that of LNG, also a 13 ethyl–substituted but 19-nortestosterone derivative.
In addition to the transactivation studies, we conducted NES metabolite profiling in mice to determine whether NES is metabolized similarly to progesterone and if reduced metabolites of NES are active on the mouse brain tissue. In this regard, some of the reduced metabolites of progesterone are established as potent and selective positive allosteric modulators of the γ-aminobutyric acid type A receptor (GABAAR). Upon administration, these steroids exhibit clear behavioral effects that include anxiolysis, sedation, and analgesia. They are anticonvulsant and at high doses induce a state of general anesthesia (24, 25). The demonstration that certain neurons and glial cells within the central nervous system can synthesize these steroids either de novo or from peripherally derived progesterone has led to the proposal that these steroids (neurosteroids) can additionally function in an autocrine, or paracrine, manner to locally influence GABAergic transmission (26).
Not all progestins exert the same behavioral effects as natural progesterone, possibly due to a lack of conversion into 5α-reduced metabolites or the lack of an interaction with the GABAAR. We therefore assessed the potential of NES progestin to be metabolized in mouse brain tissue and, using electrophysiology, compared the GABAAR modulatory action of one of these metabolites [3α, 5α-tetrahydronestorone (3α, 5α-THNES)] with the progesterone metabolite 3α, 5α-THPROG.
Materials and Methods
Structure-activity experiments
AR and PR transactivation by NES, 13-ethyl NES, and LNG
The expression vectors pchPR code for human progesterone receptor isoform B. The human AR expression vector pcDNA-hAR was kindly provided by G.A. Coetzee. The plasmid pcβgal, which contains the β-galactosidase sequence, was used to standardize the transfection experiments. The reporter vector GRE2Luc was the kind gift from Drs. A. Biola-Vidamment and M. Pallardy. HEK 293T cells were routinely cultured in a high-glucose Dulbecco’s modified Eagle medium (Invitrogene, Cergy Pontoise, France), 20 mM HEPES, 2 mM glutamine, 1X nonessential amino acids, 100 U/mL penicillin, and 100 µg/mL streptomycin supplemented with 10% fetal calf serum (FCS) in a humidified atmosphere at 37°C and with 5% CO2. One day before transfection, the cells were seeded at 3 × 106 cells/80-mm-diameter culture Petri dish and cultured overnight in the same medium. Six hours before transfection, the FCS-supplemented medium was replaced by the same medium supplemented with 10% dextran-charcoal–treated FCS. Transfections were carried out using the calcium phosphate precipitation method. The calcium phosphate precipitate was prepared with one of the steroid receptor expression vectors (0.5 µg pchPRB or 2 µg pchAR) plus 7 µg of the reporter vector GRE2-Luc and 1 µg of pcβgal in 1 mL of 140 mM NaCl, 0.75 mM Na2HPO4, 25 mM HEPES, and 125 mM CaCl2 (pH 7.05) and added to the cells 30 minutes later. After incubating for 16 hours, the transfected cells were washed with phosphate-buffered saline containing 2.5 mM EDTA, trypsinized, and pooled. The transfected cells were replated in 24-well plates (100,000 transfected cells per well). Four hours later, ligands (NES, 13-ethyl NES, or LNG at various concentrations) were added to the transfected cells, and the incubation continued for 24 hours at 37°C. Cells were lysed in 300 µL phosphate-buffered saline 1X, 25-mM glycylglycine, 4 mM EDTA, 15% glycerol, 1% triton X-100, and 15 mM MgSO4 (pH 7.8) supplemented with 2 mM β-mercaptoethanol. The luciferase activities were quantified using a Mithras LB940 microplate reader (Berthold). To standardize the transfection efficiency, the relative light units obtained in the luciferase assay were divided by the optical density obtained in the β-galactosidase assay.
Docking of NES within the ligand-binding pocket of the androgen and progesterone receptors
NES was built using the build fragment function of the Discovery Studio package (version 3.1; Accelrys, San Diego, CA). The C17 side chains of NES were oriented to superimpose those of Ulipristal Acetate (27) After minimization using the charmm27 force field, NES was manually docked within the X-ray crystal structure of the AR and PR LBDs (PDB identification numbers 2AMA and 1A28, respectively) by taking as a guide the probe-accessible volume of each cavity. The orientations of the ligand or protein side chains were fixed during the docking process.
Metabolism studies in brain
Chemicals
NES was synthesized under GMP conditions at Crystal Pharma, Spain. In addition, reduced metabolites of NES, such as 5α-dihydronestorone (5α-DHNES); 20α-dihydronestorone (20α-DHNES); 3α, 5α-THNES; and 3β, 5α-tetrahydronestorone (3β, 5α-THNES), were custom synthesized by Crystal Pharma (Valladolid, Spain) based on structure designs that we intended to explore corresponding to the known progesterone metabolite(s) potentially interacting with the GABA-A receptor (Fig. 1). The structures of synthetic reference reduced metabolites were confirmed, and purity was determined to 95% to 98%.
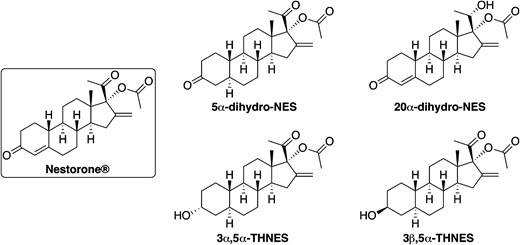
Structures of NES and its tentative metabolites identified by in vitro metabolism studies. Reference metabolites were custom synthesized and used for metabolite profiling, PR transactivation, and GABAA receptor binding studies.
Steroid measurements in mouse brain and plasma by gas chromatography/tandem mass spectrometry
An acute subcutaneous injection of NES (10 µg/mouse or 200 µg/mouse) was administered to 3-month-old C57Bl6 female mice. Mice were euthanized by decapitation after anesthesia with isoflurane at 0.5, 1, and 2 hours postadministration for the low-dose experiment and at 0.5, 1, 2, 4, and 24 hours postadministration for the high-dose experiment (n = 6 per group). Blood samples were collected and centrifuged at 3000 g for 10 minutes at 4°C to obtain plasma samples (200 to 300 µL). The brain (400 to 500 mg) was dissected out on a bed of crushed ice and weighed. The samples were stored at −20°C until gas chromatography/tandem mass spectrometry (GC/MS/MS) analysis.
NES; 5α-DHNES; 20α-DHNES; 3α, 5α-THNES; and 3β, 5α-THNES were determined by GC/MS/MS according to the purification protocol described by Meffre et al. (28) with minor modifications. Extraction was performed by adding 10 volumes of methanol (MeOH), and internal standards were introduced for steroid quantification: 2 ng [2H6]5α-DHPROG (CDN Isotopes, Pointe Claire, QC, Canada) for 5α-DHNES, 2 ng [13C3] androstenedione (Isoscience, King of Prussia, PA) for NES, and 20α-DHNES and 2 ng of epietiocholanolone for 3α, 5α-THNES and 3β, 5α-THNES. Samples were purified and fractionated by solid-phase extraction with the recycling procedure (29). The unconjugated steroid-containing fraction was filtered and further purified and fractionated by high-performance liquid chromatography (HPLC). The HPLC system is composed of a WPS-3000SL analytical autosampler and a LPG-3400SD quaternary pump gradient coupled with a SR-3000 fraction collector (Thermo Fisher Scientific, Waltham, MA). The HPLC separation was achieved with a Lichrosorb Diol column (25 cm, 4.6 mm, 5 µM) (Thermo Fisher Scientific) in a thermostated block at 30°C. The column was equilibrated in a solvent system of 90% hexane and a 10% of a mixture composed of hexane/isopropanol (85/15). Elution was performed at a flow rate of 1 mL/min, first with 90% hexane and 10% of hexane/isopropanol (85/15) for 8 minutes and then with a linear gradient to 100% of hexane/isopropanol (85/15) in 2 minutes. This mobile phase was kept constant for 10 minutes, and a linear gradient to 100% MeOH was applied. The column was washed with MeOH for 15 minutes. The fraction containing 5α-DHNES was collected in the time range 4 to 15 minutes and then derivatized with 50 µL of N-methyl-N-trimethylsilyltrifluoroacetamide/ammonium iodide/dithioerythritol (1000/2/5) for 1 hour at 80°C. NES; 20α-DHNES; 3α, 5α-THNES; and 3β, 5α-THNES were collected in the time range 15 to 28 minutes and were derivatized with 25 µL heptafluorobutyric anhydride and 25 µL anhydrous acetonitrile for 1 hour at 80°C.
Brain and plasma extracts were analyzed by GC/MS/MS with an AI 1310 autosampler (Thermo Fisher Scientific). The Trace 1310 gas chromatograph was coupled with a TSQ 8000 mass spectrometer (Thermo Fisher Scientific). The mass spectrometer was used in tandem mode using argon as the collision gas. Injection was performed in the splitless mode at 250°C (1 min of splitless time), and the temperature of the gas chromatograph oven was initially maintained at 50°C for 1 minute and ramped between 50 to 200°C at 20°C/min, then ramped up to 300°C at 10°C/min, and finally ramped to 350°C at 30°C/min. The helium carrier gas flow was maintained constant at 1 mL/min during the analysis. The transfer line and ionization chamber temperatures were 330 and 200°C, respectively. Electron impact ionization was used for mass spectrometry with ionization energy of 70 eV. GC/MS/MS signals were evaluated using a computer workstation by means of the software Excalibur®, release 3.0 (Thermo Fisher Scientific). Identification of steroids was supported by their retention time and according to 2 or 3 transitions. Quantification was performed according to the more abundant transition for the calibration solutions and for the biological extracts. The GC/MS/MS parameters for identification and quantification of NES and its metabolites are summarized in Supplemental Table 1.
The evaluation of the analytical procedure included the limit of detection, sensitivity, linearity, and intra- and interassay precision. The limit of detection was determined as the lowest amount of compounds that can be measured by GC/MS/MS with a signal-to-noise ratio greater than 3. It ranged from 1 pg for NES and 20α-DHNES to 10 pg for 5α-DHNES; 3α, 5α-THNES; and 3β, 5α-THNES (Supplemental Table 1). Method sensitivity was evaluated by analyzing extracts of 100 mg of mouse brain and of 200 µL of mouse plasma containing progressively lower concentrations of NES and its metabolites to 0.01 ng/g or 0.01 ng/mL. The sensitivity was 0.1 ng/g or 0.1 ng/mL for NES and 20α-DHNES and 1 ng/g or 1 ng/mL for 5α-DHNES; 3α, 5α-THNES; and 3β, 5α-THNES. Linearity was determined by analyzing mice brain samples prepared at concentrations of 0.01, 0.1, 1, and 10 ng/g of NES and by measuring reduced metabolites. The assay was linear in this concentration range, with a coefficient of correlation of 0.995. Intra-assay precision was determined by analyzing 3 replicates per run of 100 mg of mice brain supplemented with 1, 10, and 100 ng/g of NES and its metabolites. Interassay precision was determined by analyzing these samples for a 4-day period. Intra-assay coefficients of variation were 9.3% to 12.5%, 5.6% to 8.3%, and 4.5% to 5.6% for 1, 10, and 100 ng/g of NES and its metabolites, respectively. Interassay coefficients of variation were 10.1% to 13.2%, 7.2% to 9.4%, and 4.8% to 6.5% for 1, 10, and 100 ng/g of NES and its metabolites, respectively.
PR transactivation by NES and its reduced metabolites
Test compounds (NES and its metabolites) were prepared in dimethyl sulfoxide (DMSO). Diluted stock solutions were stored at 4°C. Human PR (hPR) in the test system described below was challenged with 8 concentrations of NES starting at 100 nM and continuing with 1:5 serial dilutions and with 5α DHNES and 3α, 5α THNES starting at 10,000 nM and continuing with 1:5 serial dilutions.
The nuclear receptor assay system for this transactivation experiment used proprietary human cells engineered to provide constitutive, high-level expression of the full-length human PR (Reporter Assay System 96-well Format Assays Product #IB05001; Indigo Biosciences, State College, PA). The reporter vector used in these studies comprised the firefly luciferase gene functionally linked to an upstream PR response element.
A suspension of reporter cells was prepared in cell recovery medium (containing 10% charcoal-stripped FBS). For PR agonist activity assay, 100 µL of the reporter cell suspension was dispensed into wells of a white, cell culture–treated, 96-well assay plate. Immediately prior to assay setup, test compounds were diluted using compound-screening medium containing 10% charcoal stripped FBS to generate 2×-concentration treatment media. A volume of 100 µL of each treatment medium was dispensed into triplicate assay wells predispensed with reporter cells. Assay plates were incubated at 37°C for 24 hours. After the 24-hour incubation period, treatment media were discarded, and 100 μL/well of luciferase detection reagent was added. Relative light units were quantified from each assay well to determine PR agonist activity. The performance of PR agonist assays was validated by performing a reference agonist (progesterone) dose response curve.
Interaction of reduced NES metabolites with GABAA receptors
Chemicals and reagents for electrophysiological studies of WSS-1 cell and mouse cortical neurons.
GABA, tetrodotoxin, and strychnine were obtained from Sigma (Dorset, UK)/Tocris (Abingdon, UK). Kyneurenic acid was from Ascent (Cambridge, UK). The neurosteroid 3α, 5α-THPROG was a gift from Dr. Kelvin Gee. The NES metabolite 3α, 5α-THNES was synthesized by Crystal Pharma (Valladolid, Spain) based on structure designs supplied by the Population Council. Stock solutions of 3α, 5α-THPROG and 3α, 5α-THNES in DMSO were diluted to achieve a final DMSO concentration of ≤0.01%. The vehicle had no effect on the GABA-evoked currents recorded from WSS-1 cells or on the miniature inhibitory postsynaptic currents (mIPSCs) recorded from mouse cortical neurons.
Cell culture and maintenance of WSS-1 cells.
WSS-1 cells were maintained in Dulbecco’s modified Eagle’s medium (DMEM) supplemented with 10% fetal bovine serum, 1% penicillin/streptomycin, and 400 µg/mL geneticin (G-418) used to positively select cells that express GABAARs. Resistance to the antibiotic is conferred by the vector containing a cDNA encoding the α1 subunit (30, 31). The cells were incubated at 37°C with 5% CO2 atmosphere and reseeded into a T25 flask every 3 days (i.e., when the cells were 80% to 95% confluent). For experiments, 20 to 50 μl of solution containing WSS-1 cells was plated onto a 35-mm cell culture dish. These cells were used 17 to 96 hours after plating for electrophysiological experiments. All reagents used were obtained from Invitrogen (Carlsbad, CA).
Electrophysiological studies on WSS-1 cells.
Electrophysiological studies were performed using the whole-cell voltage-clamp technique. WSS-1 cells were voltage clamped at −60 mV using an Axopatch 200B amplifier (Molecular Devices, Sunnyvale, CA) and low pass filtered with a cut-off frequency of 2 kHz. The data were recorded and digitized using a Digidata 1440A interface (Axon Instruments, Union City, CA). Data were acquired at 10 kHz and saved onto a PC hard drive. Currents were analyzed using pCLAMP 10.1.3 software (Axon Instruments). Cells were continually superfused (5 mL/min) with an extracellular solution containing (in mM) NaCl, 140; KCl, 4.7; MgCl2, 1.2; CaCl2, 2.5; glucose, 10; and HEPES, 10 (pH 7.4 with 1 M NaOH). Patch pipettes were pulled from 1.5-mm borosilicate glass capillaries with a micropipette puller (P-87; Sutter Instruments, Novato, CA). Each microelectrode was fire polished and had a final resistance of 1 to 3 MΩ when filled with an electrode solution containing (in mM): CsCl, 140; MgCl2, 2.0; EGTA, 11; ATP (Mg2+ salt) 3; and HEPES, 10 (pH 7.4 with 1 M CsOH). With these intracellular and extracellular solutions, the chloride equilibrium potential was approximately 0 mV. GABA and steroids were rapidly applied for 5 seconds (solution exchange time of 15 to 17 milliseconds) using a 3-barrel Perfusion Fast-Step (SF-77B) solution exchange system (Warner Instruments, Hamden, CT). Solution flow was regulated at 0.3 mL/min by a syringe pump (Cole-Parmer, Vernon Hills, IL). Upon establishment of the whole-cell recording mode, cells were lifted by movement of the recording electrode via the micromanipulator from the base of the dish and positioned within the stream of the control extracellular recording solution. To deliver GABA or GABA plus the steroid, the perfusion barrels were stepped rapidly from side to side, exposing the cell to the test drug solution for 5 seconds. Solution exchanges for each cell were repeated for 4 trials. Experiments were performed at room temperature (20°C to 24°C). All reagents used were obtained from Sigma-Aldrich Co. (St. Louis, MO). All data are expressed as the arithmetic mean ± SEM. Statistical significance of mean data was evaluated using a paired Student t test.
Preparation of murine brain slices for electrophysiology.
The methods were essentially as recently described (32). All rodent studies were approved by the University of Dundee Ethical Review Committee (Home Office Project Licenses 60/4005 and 70/8161, Dr. Belelli) and complied with Schedule 1 of the UK Government Animals (Scientific Procedures) Act, 1986. Cortical slices were prepared from postnatal day20 to 24 C57/Bl6 129 SVJ mice of either sex. Mice were euthanized by cervical dislocation, and the brain dissected and placed in ice-cold oxygenated (95% O2/5%CO2) artificial cerebrospinal fluid containing (in mM): sucrose, 225; KCl, 2.95; NaH2PO4, 1.25; NaHCO3, 26; CaCl2, 0.5; MgSO4, 10; glucose 10 (pH 7.4; 328 to 330 mosmol/L). Coronal brain slices were cut to 300 to 350 μm thickness using a Vibratome series 1000 PLUS Sectioning System (Intracell, Royston, Hertfordshire, UK) and then immediately transferred to a nylon mesh platform housed within a chamber containing circulating oxygenated extracellular solution (ECS) [in mM: NaCl, 126; NaHCO3, 26; KCl, 2.95; NaH2PO4, 1.25; MgCl2, 2; CaCl2, 2; and glucose, 10 (306–309 mOsm)]. Slices remained at room temperature for a minimum of 1 hour before electrophysiological recording.
Voltage-clamp recording.
During recording, cortical slices were perfused (3 to 5 mL/min) with ECS maintained at 35°C, which was recycled to a 50-mL oxygenated reservoir. For whole-cell recordings, an intracellular solution was used containing (in mM) CsCl, 135; HEPES, 10; EGTA, 10; MgCl2, 2; CaCl2, 1; Mg-ATP, 2; and QX-314, 5 (pH 7.2 to 7.3; 290 to 300 mOsm). Patch pipettes were made from thick-walled borosilicate glass (0.95 mm inner diameter; 1.55 mm) (E.D. Garner Glass Co., Claremont, CA) using a PC-10 electrode puller (Narashige, Tokyo, Japan). When filled with intracellular solution, these pipettes had an open tip resistance of 2 to 6 MΩ. Neurons were visually identified with an upright Olympus BX50WI microscope (Olympus, Southall, UK) equipped with infrared/differential interference contrast optics. Pyramidal neurons located within cortical L II/III were identified based on their pyramidal morphology. Neurons were voltage clamped (Vh = −60 mV) using an Axopatch 1D amplifier (Molecular Devices, Sunnyvale, CA) and filtered at 2 kHz. The GABAAR-mediated mIPSCs were isolated by supplementing the ECS with kynurenic acid (2 mM), tetrodotoxin (500 nM), and strychnine (1 μM). Data were acquired and digitized (10 kHz) using a NIDAQ mx card (National Instruments, Austin, TX) and stored directly to a personal computer using WinEDR software (Strathclyde University, Glasgow, UK). Series resistance compensation was applied up to 80%.
Analysis of mIPSCs.
Results
NES structure-activity relationships
Transactivation studies
Transactivation assays performed in HEK293T cells transiently expressing PR revealed that NES and 13-ethyl NES display the same potency to activate PR (EC50, 8.2 pM) [Fig. 2(A)]. These 2 molecules are much more potent than progesterone (EC50, 98 pM) [Fig. 2(A)] and norethindrone (EC50, 53 pM) [Fig. 2(A)], a testosterone-derived progestin characterized by a 13-methyl group. Interestingly, NES and 13-ethyl NES are nearly as potent as LNG, a testosterone-derived progestin characterized by a 13-ethyl group substitution (EC50, 5.8 pM) [Fig. 2(A)]. These results show that the replacement of a methyl group by an ethyl group at the C13 position does not modify the already high potency of NES, whereas it increases that of norethindrone. Transactivation assays performed in HEK293T cells transiently expressing AR revealed that NES and 13-ethyl NES are nearly unable to activate AR [Fig. 2(B)], in contrast to LNG, which is a potent AR agonist (EC50, 1.18 ± 0.01 × 10−10 M) [Fig. 2(B)]. These results show that the ability of progestin to activate AR is greatly ensured by the C17 substituents. The 17β-hydroxyl and 17α-ethynyl groups of LNG are much more efficient than the 17β-methylketone and 17α-acetoxy groups of NES for producing an androgenic response.
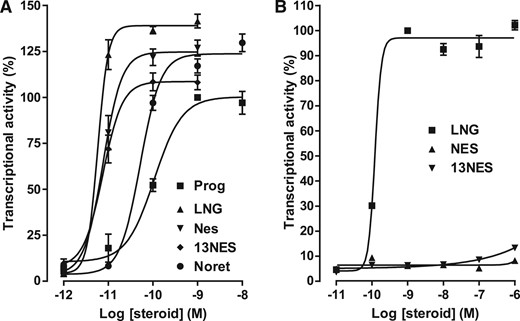
PR and AR transactivation activity in response to progestins. HEK-293T cells transiently expressing hPR (A) or AR (B) were incubated for 16 h with progesterone (Prog), levonorgestrel (LNG), NES, 13 ethyl NES (13NES), or norethindrone (Noret). The cell extracts were assayed for luciferase and β-galactosidase activities. The PR and AR transactivation activity was determined by the luciferase activity normalized by the β-galactosidase activity. Values are the means ± SEM of 3 independent experiments performed in triplicate. GraphPad Prism software was used for curve fitting and calculation of the EC50 values.
NES binding mode to PR and AR LBD
To link the high potency of NES to activate the PR on its chemical structure, we followed a modeling approach based on the X-ray crystal structure of the PR LBD complexed with progesterone (19). Within the ligand-binding cavity of PR, NES can adopt the same positioning as progesterone. The C3 ketone function of NES can be hydrogen bonded to the Gln725 (3.0 Å) and Arg766 (1.7 Å) [Fig. 3(A)], and its 17β-methylketone can be anchored to Cys891 through a CHO hydrogen bond (2.8 Å) [Fig. 3(A)], as is observed for progesterone (19). The main difference between NES and progesterone is the presence of the 17α-acetoxy and 16-methylene groups in NES. Both groups can establish additional stabilizing contacts within the ligand-binding pocket of PR, the 17α-acetoxy group forming van der Waals contacts with Leu715, Leu718, and Phe794 [Fig. 3(B)] (3.3, 4.4, and 3.2 Å, respectively) and the 16-methylene group contacting Tyr890 (3.4 Å). These observations may explain the higher potency of NES as compared with progesterone. Docking studies of the 13-ethyl NES further revealed that this molecule adopts the same anchoring positioning in the LBD as NES and that the 13-ethyl group can be accommodated within the binding pocket at the vicinity of Met909 as the 13-methyl group does (data not shown). This result is in good agreement with the observation that NES and 13-ethyl NES display the same potency in activating PR. A striking difference was observed between LNG and norethindrone because the accommodation of the 13-ethyl group of LNG requires a change in the Met909 orientation, permitting additional stabilizing contacts that are responsible for the higher potency of LNG as compared with norethindrone (22).
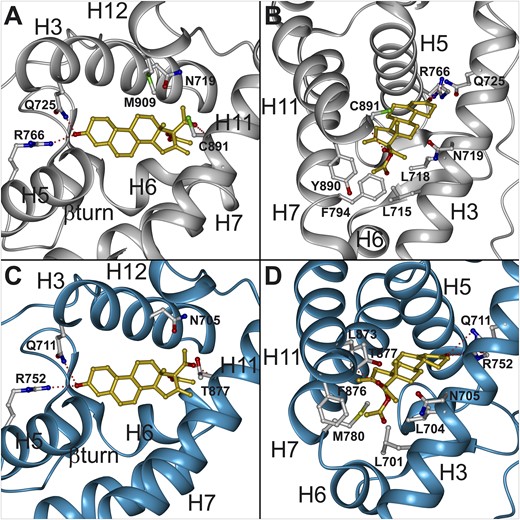
Docking of NES within the PR and AR ligand-binding cavity. (A, B) Accommodation mode of NES within the PR LBD (PDB ID: 1A28). The α-helices of the PR LBD are depicted as gray ribbons. (C, D) NES accommodation within the AR LBD (PDB ID: 2AMA). The α-helices of the AR LBD are depicted as blue ribbons. Some residues in the binding pockets are shown with their carbon, oxygen, nitrogen, and sulfur atoms colored in gray, red, blue, and green, respectively. The carbon and oxygen atoms of the ligand NES are colored in gold and red, respectively. The figure panels were generated using the Dino package (DINO: Visualizing Structural Biology 2002) (http://www.dino3d.org). The view in B and D was obtained by applying 90° rotations (around the x and y axis) on the view of A and C.
We also used a modeling approach to compare the anchoring mode of NES and LNG within AR. LNG docking within the X-ray crystal structure of the AR LBD complexed with dihydrotestosterone (DHT) (PDB ID: 2AMA) reveals that it adopts the same positioning as DHT and that its C3-ketone function can be hydrogen bonded to the Gln711 and Arg752 and its C17-hydroxyl anchored to Asn705 and Thr877 (data not shown). Docking of NES within the AR LBD showed that it can also adopt the same orientation as DHT, with its C3-ketone function hydrogen bonded to the Gln711 and Arg752 (3.5 and 2.9 Å) [Fig. 3(C)]. However, NES is unable to be anchored to Asn705 [Fig. (3C)]. Furthermore, the 17β-methylketone and 17α-O-acetyl group of NES make strong unfavorable contacts with Leu701 (2.0 Å), Leu704 (2.7 Å), Asn705 (2.4 Å), and Met780 (2.9 Å) [Fig. 3(D)]. Thus, NES is not stabilized within the AR binding pocket, leading to an extremely low capacity of this molecule to activate AR.
Metabolite profiling in mouse brain and PR transactivation
Metabolite profiling of NES in mice
After acute administration of a high dose of NES (200 µg/mouse) in female mice, a maximal level of NES was measured at 1 hour postadministration both in plasma (4.7 ± 1.1 µg/mL) [Fig. 4(A)] and brain (0.62 ± 0.09 µg/g) [Fig. 4(C)] with a marked decline to 4 hours postadministration. GC/MS/MS analysis revealed that 5α-reduced metabolites of NES, such as 5α-DHNES, as well as 3α, 5α-THNES and 3β, 5α-THNES, were detected both in plasma and brain but in limited amounts as compared with NES [Fig. 4(A) and Fig. 4(C)]. 5α-DHNES was the most abundant metabolite in brain at 30 minutes postadministration (80.5 ± 25.5 ng/g) [Fig. 5(C)], whereas 3β, 5α-THNES was the major metabolite in plasma at 1 hour postadministration (116.6 ± 26.7 ng/mL) [Fig. 5(A)]. Maximal levels of 5α-DHNES and 3α, 5α-THNES were also found in plasma at 1 hour postadministration (36.3 ± 5.4 and 58.5 ± 17.1 ng/mL, respectively) [Fig. 5(A)]. 20α-DHNES was not detected.
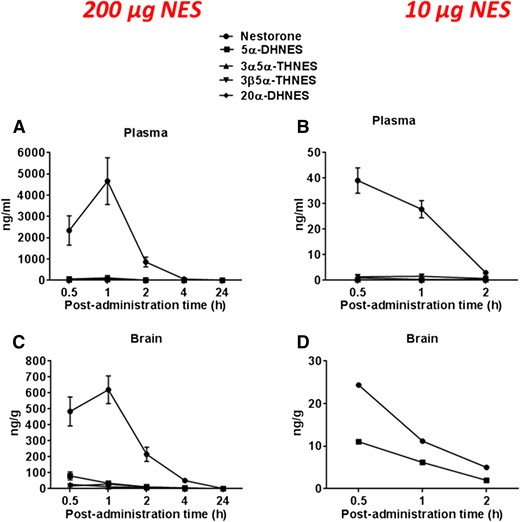
NES metabolism in plasma and brain of female mice after NES administration. NES and its metabolites were measured by GC/MS/MS. Steroid concentrations were measured in plasma after administration of NES at 200 µg (A) and 10 µg (B) and in brain at 200 µg (C) and 10 µg (D) at different postadministration time points. Steroid concentrations are expressed as ng/mL ± SEM or ng/g ± SEM.
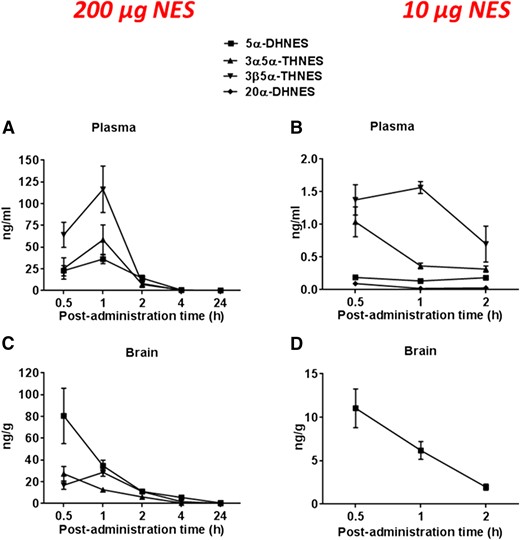
NES metabolism in plasma and brain of female mice after NES administration at 10 and 200 µg/mouse. NES metabolites were measured by GC/MS/MS. Steroid concentrations are measured in plasma after administration of NES at 200 µg (A) and 10 µg (B) and in brain at 200 µg (C) and 10 µg (D) at different postadministration time points. Steroid concentrations are expressed as ng/mL ± SEM or ng/g ± SEM.
In the second experiment, a much lower dose of NES (10 µg/mouse) was administered. Higher levels of NES were found at 30 minutes postadministration both in plasma and brain (39.1 ± 5.0 ng/mL and 24.4 ± 2.5 ng/g) [Fig. 4(B) and Fig. 4(D)], and 5α-DHNES was the only detectable NES metabolite found at comparable levels relative to NES in mice brain (11.0 ± 2.2 ng/g) [Fig. 4(D)]. High concentrations of 5α-DHNES were shown in brain [Fig. 5(D)] as compared with the very low levels found in plasma (0.13 to 0.19 ng/mL) [Fig. 5(B)]. Both forms of THNES (3α, 5α and 3β, 5α) were the major NES metabolites measured in plasma (1.0 to 1.5 ng/mL) [Fig. 5(B)].
PR transactivation with NES and its metabolites
PR agonist activity of NES, 5α-DHNES, and 3α, 5α-THNES was evaluated in the hPR transactivation assay. Based on these results, the putative synthetic NES metabolites possess significantly lower PR agonist activities as compared with NES, the parent molecule. Progesterone, the reference PR agonist, had an EC50 of 650 pM, and NES had an EC50 value of 24 pM, whereas the EC50 of 5α-DHNES and 3α, 5α-THNES were 410 and 2850 pM, respectively. Compared with NES, 5α-DHNES and 3α, 5α-THNES were approximately 17× and 120×, respectively, less potent in activating PR agonist activity. Thus, reduction of NES leads to loss of PR activity, which may be due to the lack of strong binding to PR of 5α-DHNES and 3α, 5α-THNES in this assay system.
Does the NES metabolite 3α, 5α-THNES modulate GABAARs?
The reduced metabolite of progesterone, allopregnanolone (3α, 5α-THPROG), is an established potent positive allosteric modulator of the GABAAR (34–36). Indeed, after in vivo metabolism of progesterone, this steroid enhances GABAAR function and contributes to the behavioral effects of the parent steroid, progesterone (34). As described above, in the in vivo study in mice, NES was partially converted to 5α-DHNES; 20α-DHNES; 3β, 5α-THNES; and 3α, 5α-THNES. Structure-activity studies have revealed that progesterone and 5α-DHPROG have little or no direct effect on GABAAR (26, 35). By contrast, reduction of the 3-ketone group of 5α-DHPROG to a 3α-hydroxy (i.e., 3α, 5α-THPROG) confers potent GABAAR activity (26). Therefore, we investigated whether the equivalent metabolite of NES (3α, 5α-THNES), which crucially incorporates the A ring 3-hydroxy in the α configuration, was similarly active at the GABAAR.
Electrophysiological studies on WSS-1 cells
Preliminary experiments revealed the rapid application of GABA (10 nM to 1 µM) to WSS-1 cells expressing α1βγ2 GABAARs (Vh = −60 mV) produced a concentration-dependent inward current. For all subsequent experiments, a GABA concentration of 3 µM, which gave a response that was ∼10% of the maximum response to GABA (EC10), was used. The coapplication of GABA (3 µM) with 3α, 5α-THPROG (100 nM) produced a large increase of the GABA-evoked response (461 ± 69% of control; n = 4; P < 0.001, paired Student t test) (Fig. 6). Under identical conditions, the NES metabolite 3α, 5α-THNES (100 nM) produced only a modest, albeit significant (P < 0.01, paired Student t test), enhancement of the GABA-evoked response (131 ± 4% of control; n = 10 cells) (Fig. 6). An increased concentration of 3α, 5α-THNES (1 µM) did not produce a further increase of the GABA-evoked current and indeed was now statistically ineffective in this respect (129% ± 13% of control; n = 5 cells) (P > 0.05; paired Student t test) (Fig. 6).
![The Nestorone metabolite 3α, 5α-THNES has little effect on GABAARs. (A) Illustrated are the GABA (3 µM)-induced inward currents recorded from a representative WSS-1 cell in the absence (black trace) and presence (gray trace) of the neurosteroid 3α, 5α-THPROG (100 nM). Note the large enhancement of the GABA-evoked response produced by this neurosteroid. (B) The nestorone metabolite 3α, 5α-THNES (100 nM) produced only a modest increase in the GABA (3 µM)-evoked current. (C) Bar chart summarizing the effect of 3α, 5α-THPROG (100 nM; n = 4) and 3α, 5α-THNES (100 nM, n = 10; 1 µM, n = 5) on the peak amplitude of the GABA-evoked response. **P < 0.01; ***P < 0.001 (Student t test). (D, E) The black traces illustrate averaged mIPSCs recorded from representative mouse cortical pyramidal neurons under control conditions. Superimposed upon these control recordings are representative mIPSCs recorded from cortical neurons obtained from brain slices incubated for ∼2 h in 3α, 5α-THPROG (100 nM) (D) and 3α, 5α-THNES (100 nM) (gray traces) (D). (F) Bar chart summarizing the effect of 3α, 5α-THPROG (100 nM; n = 5 neurons) and 3α, 5α-THNES (100 nM, n = 4 neurons; 1 µM, n = 4 neurons) on the control (n = 25 neurons) mIPSC decay time [quantified as the τw the weighted time constant of decay (ms)]. ###P < 0.001 (1-way ANOVA). Note the large prolongation produced by 3α, 5α-THPROG (100 nM), whereas 3α, 5α-THNES (100 nM) was inert in this respect. The data for the control τw and the τw in the presence of 3α, 5α-THPROG (100 nM) is reproduced from Brown et al. (32).](https://oup.silverchair-cdn.com/oup/backfile/Content_public/Journal/endo/158/1/10.1210_en.2016-1426/3/m_en.2016-1426f6.jpeg?Expires=1750264546&Signature=y001MjP6mAOU4XZ3qWakxFAcgi6rAzSjHRcyjLT5wGNunBMvx0VDfs9JWc0-VdFzaLZvh0YLgj~vMpIGhiNDNwhXTdOHQVelEZWMNkyvNFEm8WDLOjJGZUitjl4EwpTMkDlo~qkCc1kHbETQcN20NJdf8w~TMZzZmA7DlCz0-Pnx6QCcUkoHVxX9qCDEJ7q5VeSK1sbEjuHVDuEICjoCB0G-rVtB4gwLpvSqAcS~9vm0zpEqdhX0CjoB13tcgf9-aKGrYn-JpZUxgtSugYcI1c6Vjw-fBu3MUzm-VSOb~CKwaxRgq7GSporl6lvVy31AbsO0~XfiK5VbTWwalpPNZg__&Key-Pair-Id=APKAIE5G5CRDK6RD3PGA)
The Nestorone metabolite 3α, 5α-THNES has little effect on GABAARs. (A) Illustrated are the GABA (3 µM)-induced inward currents recorded from a representative WSS-1 cell in the absence (black trace) and presence (gray trace) of the neurosteroid 3α, 5α-THPROG (100 nM). Note the large enhancement of the GABA-evoked response produced by this neurosteroid. (B) The nestorone metabolite 3α, 5α-THNES (100 nM) produced only a modest increase in the GABA (3 µM)-evoked current. (C) Bar chart summarizing the effect of 3α, 5α-THPROG (100 nM; n = 4) and 3α, 5α-THNES (100 nM, n = 10; 1 µM, n = 5) on the peak amplitude of the GABA-evoked response. **P < 0.01; ***P < 0.001 (Student t test). (D, E) The black traces illustrate averaged mIPSCs recorded from representative mouse cortical pyramidal neurons under control conditions. Superimposed upon these control recordings are representative mIPSCs recorded from cortical neurons obtained from brain slices incubated for ∼2 h in 3α, 5α-THPROG (100 nM) (D) and 3α, 5α-THNES (100 nM) (gray traces) (D). (F) Bar chart summarizing the effect of 3α, 5α-THPROG (100 nM; n = 5 neurons) and 3α, 5α-THNES (100 nM, n = 4 neurons; 1 µM, n = 4 neurons) on the control (n = 25 neurons) mIPSC decay time [quantified as the τw the weighted time constant of decay (ms)]. ###P < 0.001 (1-way ANOVA). Note the large prolongation produced by 3α, 5α-THPROG (100 nM), whereas 3α, 5α-THNES (100 nM) was inert in this respect. The data for the control τw and the τw in the presence of 3α, 5α-THPROG (100 nM) is reproduced from Brown et al. (32).
Electrophysiological studies on mouse cortical neurons
The GABAARs engineered to be expressed in a cell line may not accurately represent the properties of native neuronal GABAARs. We therefore investigated whether 3α, 5α-THNES influences the function of synaptic GABAARs of mouse cortical pyramidal neurons. We have previously demonstrated that the duration of IPSCs mediated by GABA activation of synaptic GABAARs is prolonged by 3α, 5α-THPROG (32). Due to issues associated with poor penetration of the steroid into the mouse brain slice preparation, we incubated the slice for 2 hours in 3α, 5α-THNES (100 nM or 1 µM). However, under these conditions, the steroid had no effect at either concentration on the mIPSC decay time (Fig. 6; Supplemental Table 1). By contrast, equivalent treatment with 3α, 5α-THPROG (100 nM) produced a large prolongation of the mIPSC decay time (Fig. 6) (32) (see also Supplemental Table 2)
Discussion
Nestorone is a new chemical entity used as a progestin component in female and male contraceptive methods (8–11). It was shown to exert additional noncontraceptive effects that may lead to health benefits (12–16). Previously we showed that NES binds to PR with much greater affinity than progesterone but does not bind to AR. These in vitro results were also confirmed by bioassays in in vivo animal models (3). Our previous results showed the high selectivity of NES in terms of its high progestational activity without any androgenic or estrogenic activity. In this study we show that NES and 13-ethyl NES, 2 progesterone-related structures, with a 13-methyl and 13-ethyl substituent, respectively, display the same high potency to activate hPR. In contrast, LNG, a 19-nortestosterone–related progestin with a 13-ethyl substituent, was shown to be much more potent than norethindrone, the corresponding testosterone-related progestin with a 13-methyl substituent (22). Accordingly, NES and 13-ethyl NES are accommodated in the ligand-binding cavity of PR in a similar mode, creating the same network of stabilizing contacts, as revealed by docking studies. In contrast, the binding mode of LNG and norethindrone to hPR are quite distinct. Thus, the replacement of a methyl group by an ethyl does not modify the activity of progesterone-related progestins, although it increases the activity of testosterone-related progestin structures.
In addition, in this structure-activity relationship study, we showed that the ethylene radical in C16 position for both NES and 13-ethyl NES creates an additional anchor increasing the potent progestational action of NES and 13-ethyl NES. In contrast to LNG, NES did not bind to the ligand binding domain of AR and did not transactivate the AR, confirming its lack of androgenic activity previously shown in an in vivo bioassay (3). The differential action of NES on PR and AR was also confirmed by Attardi et al. (37) using other in vivo models.
In several earlier studies, the neuroregenerative and neuroprotective effects of NES were observed in a stroke model (13, 14), and remyelination effects of NES were observed in a cuprizone demyelinated mice model (14, 15). These findings were shown to be PR related because the effects were not observed in knock-out mice models (14). In our earlier pharmacology studies, we did not investigate if NES converts to reduced metabolites comparable to metabolites formed with progesterone, specifically 3α, 5α-THPROG, a potent neurosteroid (allopregnanolone) with neuroprotective effects (17, 18). Therefore, we assessed whether NES would convert to reduced metabolites in mouse plasma and brain tissue at different time periods after NES subcutaneous administration of low and high NES dose. The low dose (equivalent to a neuroprotective dose in mice) and the high dose (pharmacological) of NES were chosen to show the potential for NES conversion to dihydro- and tetrahydro-NES (equivalent to allopregnanolone formation with progesterone) metabolites using GC/MS/MS. The high dose was used in these experiments to make sure that the reduced metabolites are detected by GC/MS/MS with sufficient sensitivity. The metabolites were identified and quantified by a sensitive and specific GC/MS/MS method using synthetic reference standards (28, 29). We demonstrated the presence of reduced NES metabolites in both brain tissue and plasma when NES is injected in mice subcutaneously. However the concentrations of reduced metabolites detected in brain and plasma appeared to be much lower than the concentration of reduced metabolites obtained with progesterone.
The 5α-DHNES was converted to 3α, 5α-THNES in the brain from mice treated with a high dose of NES, which is similar to the formation of 3α, 5α-THPROG (allopregnanolone) from 5α-DHPROG but at a much lower conversion rate. Indeed, the concentration of 3α, 5α-THNES in the brain tissue samples was very low compared with NES and 5α-DHNES levels in brain tissue samples. In mice injected with a low dose of NES, the 3α, 5α-THNES metabolite was undetectable in brain tissue. Only 5α-DHNES was detectable after injection of the low dose of NES. The high concentration of 5α-DHNES found in the brain as compared with the very low levels found in plasma clearly indicate a high 5α-reductase activity in brain.
Dense expression of the 5α-reductase enzyme in the white matter has been well demonstrated (38). An active 5α-reductase activity has also been shown to be present in peripheral myelinated nerves (39). The localization in myelin membranes may suggest a possible involvement of 5α-reduced metabolites of the different steroids in the process of myelination. However, the major metabolite observed with the low dose of 10 µg/animal (therapeutic dose in demyelination and stroke models) was the 5α-dihydro NES, which would not be expected to interact with the GABAAR, given the presence of keto but not the hydroxyl function at the critical 3 position of the steroid A ring (26).
We investigated whether these reduced metabolites may have potent activity on the PR transactivation and if they modulate the function of the GABAAR (i.e., in common with progesterone, could they act as neuroprotective in the brain). We tested the bioactivity of reduced metabolites compared with NES in the PR transactivation assay. NES was very potent in transactivation of PR, followed by 5α-DHNES and 3α, 5α-THNES. These findings suggest that 5α-DHNES does bind to PR with a binding affinity sufficient to transactivate PR but was almost 17 times less potent than the parent NES in this transactivation. These observations suggested that NES is metabolized in the brain by reductive pathway, but the overall metabolite concentrations may not be enough for GABAAR receptor activation. This finding leads to our conclusion that most neurotropic activities of NES relate to the parent molecule and not to its metabolites.
Although allopregnanolone interacts with the GABAAR and via this interaction is responsible for certain behaviors produced by progesterone such as sedation, the small conversion of NES into 3α, 5α-THNES and its limited interaction with the GABAAR supports the clinical findings of a lack of such adverse events with NES at contraceptive doses (10). In rat studies, evaluating the behavioral profiles indicative of positive allosteric modulation of the GABAAR modulatory activity, another progestin of the same class of 19-norprogesterone structure, trimegestone, was shown to be less active on this likely undesirable endpoint than progesterone and norethindrone acetate, which may translate into fewer mood-related side effects (40). However, the authors did not assess the metabolites of trimegestone in brain tissue in these studies.
In conclusion, we show that NES has strong docking and binding interactions with the ligand binding domain of PR receptor but not AR based on the structure-activity relationship studies. The binding and transactivation properties of NES regarding the PR and AR structures confirm the potent selective progestational activity of NES in vivo as well as its lack of androgenic effect.
In addition, we identified the metabolites of NES produced in the brain tissue and plasma from mice treated with NES and demonstrated that the 3α-hydroxy ring A reduced metabolite of NES exhibit little or no enhancement of GABAAR activity. These results support the conclusion that the beneficial effects of NES on the brain are not related to its metabolite(s) but mainly via its direct high-affinity binding to PR.
Abbreviations:
- 3α
5α-THNES, 3α, 5α-tetrahydronestorone
- 5α-DHNES
5α-dihydronestorone
- 20α-DHNES
20α-dihydronestorone
- 3β, 5α-THNES
3β, 5α-tetrahydronestorone
- AR
androgen receptor
- DHT
dihydrotestosterone
- DMSO
dimethyl sulfoxide
- ECS
extracellular solution
- FCS
fetal calf serum
- GABAAR
γ-aminobutyric acid type A receptor
- GC/MS/MS
gas chromatography/tandem mass spectrometry
- HPLC
high-performance liquid chromatography
- LBD
ligand-binding domain
- LNG
levonorgestrel
- mIPSC
miniature inhibitory postsynaptic currents
- NES
Nestorone
- PR
progesterone receptor
Acknowledgments
We thank Prof. Tim Hales and Dr. Daniel Baptista-Hon for advice on the electrophysiology experiments with WSS-1 cells.
This work was supported by Grant U54HD029990 from the National Institute of Child and Health Development and by the United States Agency for International Development.
Disclosure Summary: R. S.-W. and N. K. are employed by The Population Council, a not-for-profit organization developing Nestorone progestin for contraception. The other authors have nothing to declare.
References
Author notes
Address all correspondence and requests for reprints to: Regine Sitruk-Ware, MD, The Population Council, Center for Biomedical Research, 1230 York Avenue, New York, New York 10065. E-mail: [email protected].