-
PDF
- Split View
-
Views
-
Cite
Cite
Kelly E. Pollock, Damaiyah Stevens, Kathleen A. Pennington, Rose Thaisrivongs, Jennifer Kaiser, Mark R. Ellersieck, Dennis K. Miller, Laura Clamon Schulz, Hyperleptinemia During Pregnancy Decreases Adult Weight of Offspring and Is Associated With Increased Offspring Locomotor Activity in Mice, Endocrinology, Volume 156, Issue 10, 1 October 2015, Pages 3777–3790, https://doi.org/10.1210/en.2015-1247
- Share Icon Share
Pregnant women who are obese or have gestational diabetes mellitus have elevated leptin levels and their children have an increased risk for child and adult obesity. The goals of this study were to determine whether offspring weights are altered by maternal hyperleptinemia, and whether this occurs via behavioral changes that influence energy balance. We used 2 hyperleptinemic mouse models. The first was females heterozygous for a leptin receptor mutation (DB/+), which were severely hyperleptinemic, and that were compared with wild-type females. The second model was wild-type females infused with leptin (LEP), which were moderately hyperleptinemic, and were compared with wild-type females infused with saline (SAL). Total food consumption, food preference, locomotor activity, coordinated motor skills, and anxiety-like behaviors were assessed in wild-type offspring from each maternal group at 3 postnatal ages: 4–6, 11–13, and 19–21 weeks. Half the offspring from each group were then placed on a high-fat diet, and behaviors were reassessed. Adult offspring from both groups of hyperleptinemic dams weighed less than their respective controls beginning at 23 weeks of age, independent of diet or sex. Weight differences were not explained by food consumption or preference, because female offspring from hyperleptinemic dams tended to consume more food and had reduced preference for palatable, high-fat and sugar, food compared with controls. Offspring from DB/+ dams were more active than offspring of controls, as were female offspring of LEP dams. Maternal hyperleptinemia during pregnancy did not predispose offspring to obesity, and in fact, reduced weight gain.
Obesity rates have increased over recent decades, along with associated health problems such as cardiac disease and diabetes. The Centers for Disease Control reported that in 2009–2010, 35% of adults and 17% of children in the United States were obese (1). The Developmental Origins of Health and Disease hypothesis posits that the in utero environment can have lifelong effects on the fetus (2). For example, children born to mothers who are obese or have gestational diabetes mellitus have a higher risk of becoming obese themselves (3–8). Mothers suffering from obesity and/or gestational diabetes also have elevated concentrations of the hormone leptin (9–13). Leptin is a multifunctional peptide involved in energy homeostasis (14), immunity (15), reproduction (16, 17), and brain development (18). We have previously demonstrated that high maternal leptin, in combination with maternal nutrient restriction during early pregnancy, results in greater obesity and insulin resistance in female offspring given a high-fat diet (HFD) (19). Because leptin is high in both obese and gestationally diabetic mothers, we hypothesize that it may be involved in predisposing their offspring to obesity.
Ultimately, obesity results from a mismatch between calorie intake and calorie expenditure, and both are highly dependent on behavior. There is evidence that activity, food intake, and other behaviors are affected by the in utero nutritional environment. Human studies show an association between children's behavioral activity (20), motor coordination (20, 21), and anxiety with maternal obesity and/or gestational diabetes. Maternal diet during pregnancy is also likely to impact children's feeding behavior (22). Altered feeding behavior (ie, increased preference for fatty foods) (22) and increased anxiety (23) could lead to increased risk for obesity and associated diseases in adulthood. Other behavioral changes identified in offspring could have a negative impact on the lives of those children, such as increased hyperactivity (20, 23), and decreased fine and gross motor coordination (20, 21), which could last into adulthood.
Rodent studies demonstrate that maternal nutrition and/or obesity during gestation change offspring behavioral activities (24–26), including, motor coordination (26), anxiety (27, 28), and feeding behavior (24, 29–32). For example, offspring of both rat and mouse mothers fed obesogenic diets were hyperphagic (30, 31) and had greater preference for high-fat foods, which was associated with changes in the mesolimbic reward system (29, 32). The precise aspects of the maternal environment during obesity or diabetes that are responsible for altering offspring behavior are not well understood, and a variety of experimental paradigms have been used to test maternal obesity, obesogenic diets and/or maternal diabetes. Regardless, altered maternal nutrition and obesity during pregnancy are associated with altered maternal leptin concentrations and leptin resistance, which could play a role in programming offspring behavior.
The current study sought to determine whether hyperleptinemia during pregnancy alters offspring behavior in a way that increases the risk of weight gain. We used 2 mouse models of maternal hyperleptinemia, a genetic and a pharmacological model, and evaluated behavior longitudinally. The hypothesis was that maternal hyperleptinemia would increase offspring food consumption and preference for palatable food, decrease locomotor activity and motor coordination, and increase anxiety.
Materials and Methods
Animals
All animal procedures were approved by the University of Missouri Institutional Animal Care and Use Committee and performed in accordance with the National Institutes of Health Guide for the Care and Use of Laboratory Animals. Animals were housed in a 12-hour light, 12-hour dark cycle at 68°F–79°F with 30%–70% humidity.
Four Leprdb/+ male mice (strain B6.BKS(D)-Leprdb/J; stock number 000697) obtained from The Jackson Laboratory were mated to 8 C57Bl/6 wild-type females bred at the University of Missouri to establish the Leprdb colony. Genotyping was performed according to Yamashita et al (33) with minor modifications. Tail clips were incubated at 55°C for 3–18 hours in lysis buffer (50mM KCl, 10mM Tris-HCl [pH 8.3], 2mM MgCl2, 0.1-mg/mL gelatin, 0.45% Nonidet P-40, and 0.45% Tween 20), then incubated at 95°C for 10 minutes. Eight hundred nanograms of gDNA were PCR amplified, and products were cut with AccI, then resolved on a 3.5% agarose gel and visualized with SYBR Green (Lonza).
Experiment 1. Glucose tolerance in Leprdb/+pregnant mice
Wild-type C57Bl/6 control females (WT n = 7) were mated with Leprdb/+ males and congenic Leprdb/+ (DB/+ n = 9) females were mated with wild-type males. Dams were maintained on TestDiet AIN-93 g (7% kcal fat). At d18.5 of gestation, they were fasted for 6 hours and then given 2-mg/g ip glucose, and blood was sampled at 0, 15, 20, 30, 60, and 120 minutes. At 0 and 30 minutes, additional blood was collected for measurement of insulin, and a terminal blood sample was collected via cardiac puncture after glucose concentrations had returned to baseline for measurement of leptin.
Experiment 2. Offspring of hyperleptinemic, db/+ dams
Wild-type C57Bl/6 control females (WT n = 13) were mated with Leprdb/+ males and congenic Leprdb/+ (DB/+ n = 13) females were mated with wild-type males, resulting in 7 and 3 litters, respectively. A second set of C57Bl/6 controls (WT n = 12) mated with Leprdb/+ males and Leprdb/+ (DB/+ n = 24) females mated with WT males resulted in 6 and 7 litters, respectively. Reciprocal crosses were performed so that litters from WT and DB/+ dams would contain the same proportion of wild-type and Leprdb/+ offspring in utero and during lactation, but only wild-type offspring were used for postweaning analyses. At d16.5, maternal glucose, insulin, leptin, and weights were determined after a 4-hour fast. At weaning, 1 male and 1 female wild-type offspring from each litter were randomly selected for testing of food consumption and food preference at 4–5, 11–12, and 19–20 weeks of age. An additional male and female wild-type offspring from each litter were randomly selected for testing of locomotor activity, coordinated motor skills, and anxiety-like behaviors at weeks 4–6, 11–13, and 19–21 (Figure 1A). Offspring evaluated for feeding behaviors were housed individually so that food consumption of individuals could be measured. Those being evaluated for all other behaviors were group housed.
Experimental timeline and maternal values
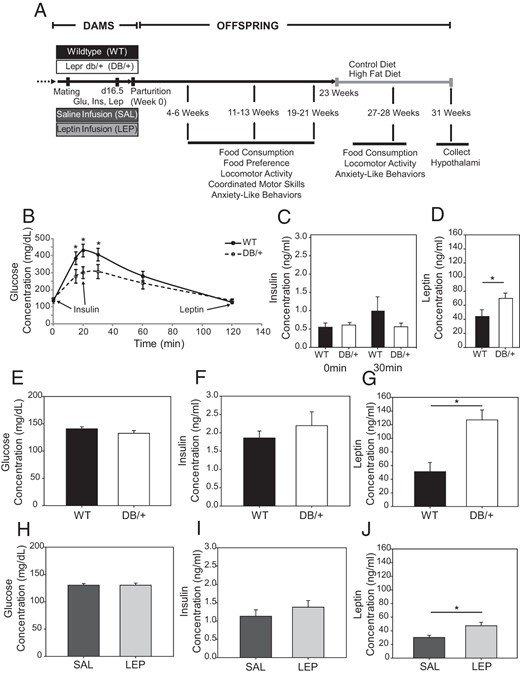
A, Timeline for maternal treatments and behavioral analyses of wild-type offspring in experiments 2 and 3. B–D, Maternal glucose tolerance test at gestational day 18.5 (experiment 1). Data are presented as averages ± SE and were analyzed by two-way ANOVA. Serum insulin, measured at 0 and 30 minutes (C), and leptin measured at 120 minutes (D) of glucose tolerance test (n, WT = 7 and DB/+ = 9). E–J, In experiments 2 and 3, maternal serum was taken at d16.5 for fasting blood glucose (E and H), insulin (F and I), and leptin (G and J) of WT and DB/+ (E–G) and SAL and LEP (H–J) dams (n: WT = 13, DB/+ = 12, SAL = 14, and LEP = 14) (*, P < .05). Fasting glucose, insulin, and leptin are presented as averages ± SE and were analyzed by using Student's t test.
Offspring from the first set of matings were maintained on the standard chow diet (CD) (LabDiet 5008; Purina) throughout the study. Two males from the second group of matings were also maintained on standard chow in order to achieve an adequate sample size for this diet group. All other offspring from the second group of matings were placed on a high-fat, high-sucrose diet (HFD) (45% kcal/fat DIO HFD D12451; Research Diets) beginning at age 23 weeks. Behaviors were then retested at 27–28 weeks. Offspring with free access to food were euthanized in early afternoon for collection of blood and hypothalami at 31 weeks of age.
Experiment 3. Offspring of hyperleptinemic, LEP-infused dams
Recombinant mouse leptin (Sigma-Aldrich) was reconstituted in saline according to manufacturer's guidelines. Alzet Mini-Osmotic Pumps (Model 2004; Durect Corp), releasing 0.25 μL/h for 28 days, were filled with 1.4-μg/μL leptin solution or saline following manufacturer's instructions. A saline or leptin pump was then inserted sc between the scapulae of WT C57Bl/6 females. Mice were allowed to recover for 3–4 days and then placed with WT males until a copulatory plug was detected or 5 days had elapsed. Initially, 11 SAL (infused with saline) females and 12 LEP (infused with leptin) females were mated, producing 4 SAL and 6 LEP litters, respectively. A second set of 14 SAL and 13 LEP females produced 10 SAL and 8 LEP litters, respectively. At d16.5, fasting glucose, insulin, leptin, and dam weights were measured. At weaning, 2 male and 2 female offspring from each litter were randomly selected for behavioral analyses as described above for WT and DB/+ offspring (Figure 1A). At age 23 weeks, offspring from the second group of matings were placed on the HFD, whereas offspring from the first group were maintained on the CD. Behaviors were then retested at 27–28 weeks. Offspring were sacrificed for blood and tissue collection at age 31 weeks.
Offspring behavioral tests
Feeding behavior
Wild-type offspring were evaluated for total amount of food intake (ages 4, 11, and 19 wk) and food preference (5, 12, and 20 wk). For 7 days at each age, consumption of a powdered CD mixed with an equal weight of water was measured at 12-hour intervals by using specialized dishes (34). Food preference was examined by introducing a highly palatable diet (PD) (50% LabDiet 5008 Meal, 50% sweetened condensed milk) (35) in addition to the CD in identical dishes and measuring the consumption of each every 12 hours. At age 28 weeks, offspring were assessed for food consumption over 5 days on their respective diets (CD or HFD).
Locomotor activity
Locomotor activity was assessed at 5, 12, 20, and 27 weeks using automated open- field activity monitors (Med Associates) interfaced to a computer running Med Associates Activity Monitor (version 4.31) software, according to procedures used previously in the Center for Translational Neuroscience (36). The apparatus consisted of a transparent box surrounded by banks of infrared sensors connected to the computer. Across 3 sessions (1 session/d) mice were placed in the center of the apparatus and allowed to move freely for 30 minutes. Dependent measures were total distance traveled and distance traveled over each 5-minute increment.
Coordinated motor skills
At 4,11, and 19 weeks, coordinated motor skills (the ability to synchronize limb movements and maintain balance) were assessed by the University of Missouri Center for Translational Neuroscience using a rotarod apparatus (ENV-577M; Med Associates) as previously described (36). Across 3 consecutive sessions (1 session/d) mice were tested on the rotarod, which rotated at an increasing velocity for up to 5 minutes, and the dependent measure was latency to fall.
Anxiety-related behaviors
Anxiety-related behaviors were measured at 6, 13, 21, and 28 weeks via the elevated-zero maze (36, 37) in the University of Missouri Center for Translational Neuroscience. The maze was divided into 4 quadrants of equal size, 2 with walls and 2 open. The gray acrylic track was 10 cm wide × 105 cm in diameter × 72 cm from the floor. The only light source was an incandescent lamp 2 m above the maze. White noise was played continuously. On a single session at each age, mice were placed facing out of a closed maze area and the dependent measure was the ratio of time spent in the closed vs open areas of the maze. Each 5-minute session was video recorded, and then 2 independent observers, blinded to offspring treatment group, recorded time spent in open and closed arms, the number of times the mice entered open or closed areas, and the number of times they explored (looked over) the edge in the open areas.
Blood and serum assays
Blood glucose concentrations were measured using a OneTouch Ultra Glucose Meter (Life Scan, Inc). A rat/mouse insulin ELISA (intrassay variation, 8.5%; Millipore) and a mouse leptin ELISA kit (intrassay variation, 15.5%; Millipore) were used according to manufacturer's procedures, but with primary antibody incubation extended to overnight.
Real-time RT-PCR
RNA was extracted from hypothalami of 1 male and 1 female per litter by homogenizing in Trireagent (Sigma), followed by RNA purification using Nucleospin RNA Clean-up kit protocol (Clontech; Mancherey-Nagel). Genomic DNA was removed by using the Turbo DNA-Free kit (Ambion by Life Technologies). The SuperScript first-strand synthesis system was used to produce cDNA (Invitrogen). SYBR Green (QIAGEN) quantitative PCR was performed for LEP receptor a (Lepra), Leprb, agouti-related protein (Agrp), neuropeptide Y (Npy), and proopiomelanocortin (Pomc). Actin-β (Actb) was used as a control. Primers (Supplemental Table 1) (19) were validated by performing serial dilutions and by analyzing the dissociation curves of the target amplicons. Data were analyzed using a comparative critical threshold method (38).
Statistics
Statistical tests compared each model of hyperleptinemia with its respective control (WT vs DB/+ or SAL vs LEP). Student's t test was conducted on maternal fasting glucose, insulin, and leptin measurements. Repeated measures two-way ANOVA was used to analyze maternal glucose tolerance tests with fixed factors treatment group, time, and treatment group × time as outlined by Littel et al (39). Offspring weights were also analyzed by using repeated measures two-way ANOVA with fixed factors maternal treatment, offspring sex, age, and diet, with offspring nested in mother. Offspring anxiety data at 28 weeks and quantitative PCR data were analyzed using a three-way ANOVA with maternal treatment group, offspring sex and diet (CD or HFD) as factors with offspring nested in mother. All other behavioral data were analyzed by ANOVAs split-plot in space and time with fixed factors diet, sex, age, and days within age as outlined by Steel et al (40) with offspring nested in mother. Least square means were used for post hoc pairwise comparisons.
Results
Maternal glucose, insulin, and leptin concentrations
At pregnancy d18.5, DB/+ dams had slightly better glucose tolerance than the control mothers (Figure 1B). Maternal insulin concentrations were not different (Figure 1C). Leptin levels in the DB/+ dams were significantly higher than in WT dams (P = .05) (Figure 1D).
In the dams whose offspring were used for behavioral testing, there were no significant differences in fasting glucose or fasting insulin concentrations between hyperleptinemic dams and their controls at pregnancy d16.5 (Figure 1, E, F, H, and I). Fasting serum leptin concentrations were significantly higher in DB/+ and LEP dams compared with their controls, WT and SAL, respectively (Figure 1, G and J). DB/+ dams had higher leptin concentrations than the LEP dams, providing a model of severe maternal hyperleptinemia (DB/+) and a model of moderate maternal hyperleptinemia (LEP), respectively. The DB/+ dams also weighed significantly more than the WT dams at day 16.5 (P < .01) (Table 1). There were no differences in litter size among the groups.
. | Weight . | n . | Litter Size . | n . |
---|---|---|---|---|
WT | 33.17 ± 0.7 | 17 | 6.00 ± 0.6 | 13 |
DB/+ | 37.38 ± 0.6a | 16 | 6.36 ± 0.8 | 13 |
SAL | 34.96 ± 0.7 | 11 | 6.45 ± 0.5 | 11 |
LEP | 36.20 ± 0.6 | 15 | 7.27 ± 0.5 | 15 |
. | Weight . | n . | Litter Size . | n . |
---|---|---|---|---|
WT | 33.17 ± 0.7 | 17 | 6.00 ± 0.6 | 13 |
DB/+ | 37.38 ± 0.6a | 16 | 6.36 ± 0.8 | 13 |
SAL | 34.96 ± 0.7 | 11 | 6.45 ± 0.5 | 11 |
LEP | 36.20 ± 0.6 | 15 | 7.27 ± 0.5 | 15 |
P < 0.01.
. | Weight . | n . | Litter Size . | n . |
---|---|---|---|---|
WT | 33.17 ± 0.7 | 17 | 6.00 ± 0.6 | 13 |
DB/+ | 37.38 ± 0.6a | 16 | 6.36 ± 0.8 | 13 |
SAL | 34.96 ± 0.7 | 11 | 6.45 ± 0.5 | 11 |
LEP | 36.20 ± 0.6 | 15 | 7.27 ± 0.5 | 15 |
. | Weight . | n . | Litter Size . | n . |
---|---|---|---|---|
WT | 33.17 ± 0.7 | 17 | 6.00 ± 0.6 | 13 |
DB/+ | 37.38 ± 0.6a | 16 | 6.36 ± 0.8 | 13 |
SAL | 34.96 ± 0.7 | 11 | 6.45 ± 0.5 | 11 |
LEP | 36.20 ± 0.6 | 15 | 7.27 ± 0.5 | 15 |
P < 0.01.
Offspring weights
As they aged, offspring of dams with pregnancy hyperleptinemia gained less weight than their respective controls (Figure 2). Wild-type offspring from DB/+ dams weighed significantly less than offspring from WT dams from 23 to 31 weeks, regardless of sex or diet (P < .05) (Figure 2, B and D). Weights of offspring from LEP dams were significantly lower at 25, 26, and 29–31 weeks, when both dietary groups were combined (P < .05) (Figure 2, F and H). In post hoc pair-wise comparisons, however, weights were only different between SAL and LEP offspring on the HFD. Male offspring weighed more than female offspring overall (P < .01), and offspring placed on the HFD weighed more than those on the control diet (P < .01), but maternal hyperleptinemia reduced offspring weights independently of sex and diet.
Weights of wild-type offspring from (A and B) WT and DB/+ and (C and D) SAL and LEP dams
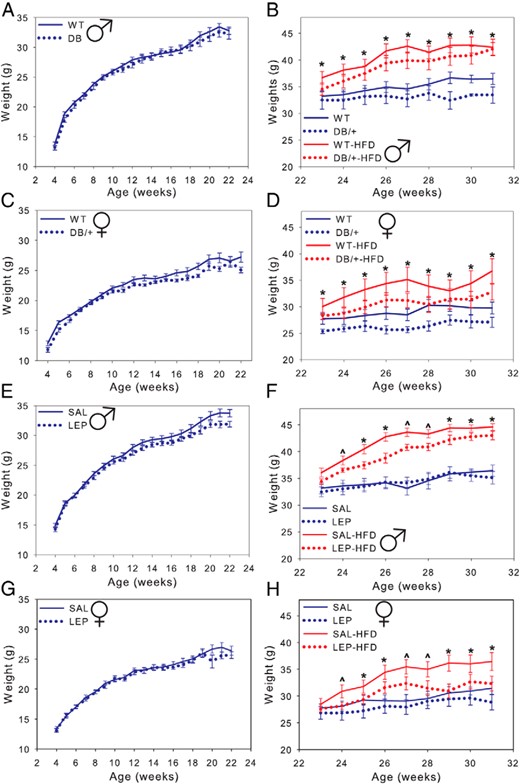
Male offspring are shown in A and C and female offspring in B and D. Offspring were maintained on a CD except where indicated (HFD). Males weighed more than females (P < .01) and offspring on the HFD weighed more than those on a control diet. Data are presented as averages ± SE and were analyzed by using repeated measures two-way ANOVA with offspring nested within dam (n: males, WT = 13; DB/+ = 12; SAL = 14; and LEP = 14 and females, WT = 11; DB/+ = 9; SAL = 14; and LEP = 13) (^, P < .07; *, P < .05, least square means).
Offspring feeding behavior
Total food consumption
Offspring of hyperleptinemic dams consumed somewhat more than offspring of control mothers. At 4 weeks of age, offspring from DB/+ dams consumed more food per gram of body weight on days 1, 5, 6, and 7 (P < .01) (Supplemental Figure 1, A and B) and across days (P < .01) (Supplemental Figure 1, C and D). There were no differences in total food consumed between male offspring of hyperleptinemic and control groups (Figure 3, A and B), although consumption adjusted for body weight was higher in LEP offspring at 4 weeks of age (Supplemental Figure 1F). There was some evidence of greater daily food consumption in female offspring from DB/+ dams at 19 weeks of age, although it was not quite significant (P = .057) (Figure 3C). In pairwise comparisons, females from LEP dams consumed more than females from SAL dams at 11 weeks, both in total and body weight adjusted (P < .05) (Figure 3D, and Supplemental Figure 1, E and F). Overall, females consumed more than males (P < .01), and weight-adjusted consumption declined with age (4 > 11 > 19 wk, P < .01).
Food consumption of male (A and E) and female (C and G) offspring from WT and DB/+ dams, and of male (B and F) and female (D and H) offspring from SAL and LEP dams
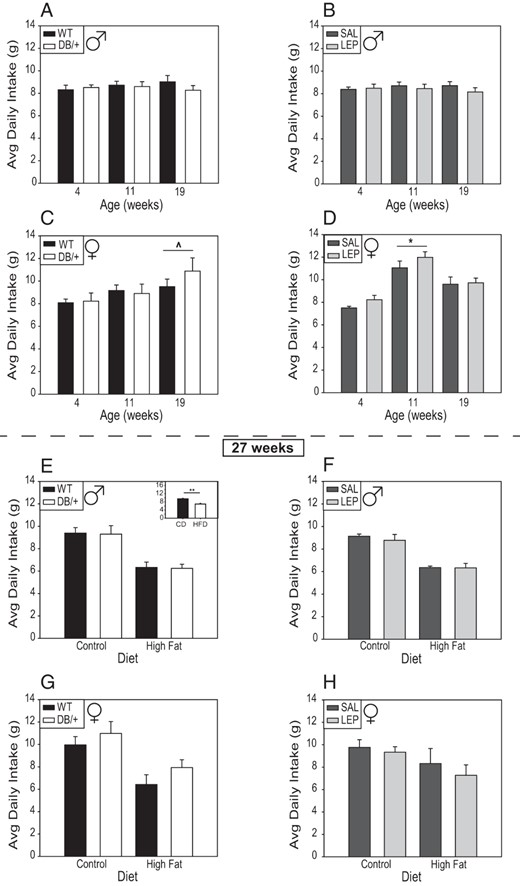
A–D, All animals were fed a standard diet at ages 4, 11, and 19 weeks. E–H, At 23 weeks, half the offspring were switched to a HFD, and then consumption by the mice on each diet was assessed at 27 weeks. Data are presented as averages ± SE and were analyzed by using ANOVA split-plot in space and time with offspring nested within dam (n: males, WT = 10; DB/+ = 10; SAL = 14; and LEP = 12 and females, WT = 9; DB/+ = 6; SAL = 12; and LEP = 13) (^, P < .06; *, P < .05, least square means). E, inset, ** at 27 weeks of age, consumption of control diet was higher than that of the HFD, independent of age or sex.
At 27 weeks, there were no differences in grams of food consumed between offspring of hyperleptinemic and control groups (Figure 3, E–H), although when consumption was adjusted for body weight, offspring of DB/+ dams tended to consume more food (P < .06) (Supplemental Figure 2, A and C), and they did consume more calories (P = .05) (Supplemental Figure 3, A and C). On the control diet, offspring from DB/+ dams consumed more grams of food/gram body weight on days 3 and 5 of the experiment compared with WT controls (Supplemental Figure 2, A and C). Offspring on the HFD consumed significantly less food, and less calories, than those on the control diet, regardless of maternal leptin status (Figure 3E, inset, and Supplemental Figures 2 and 3, E–H).
Food preference
Males consumed more of a highly PD than control diet (CD) regardless of age, with no differences between offspring of hyperleptinemic and control dams (Figure 4, A and B), although when adjusted for body weight, male offspring of DB/+ dams ate more CD than offspring of WT dams (data not shown). Maternal hyperleptinemia in DB/+ mice resulted in female offspring consuming more of CD, and thus more food total, at 5 and 20 weeks of age, but they had a reduced preference for the PD (Figure 4C). The difference at 20 weeks, but not 5 weeks, was maintained when consumption was adjusted for body weight (data not shown). At 12 weeks, female offspring of WT and DB/+ consumed the same amount of both diets. Females from LEP dams consumed more CD than those from SAL dams at 12 weeks (P < .01), and this trend continued at 20 weeks (P < .06) (Figure 4D), at which time offspring of LEP also consumed more CD per gram body weight. At 5 and 20 weeks, all female offspring consumed more PD than CD. Overall, female offspring from hyperleptinemic dams consumed more CD and total food.
Food preference and hypothalamic gene expression
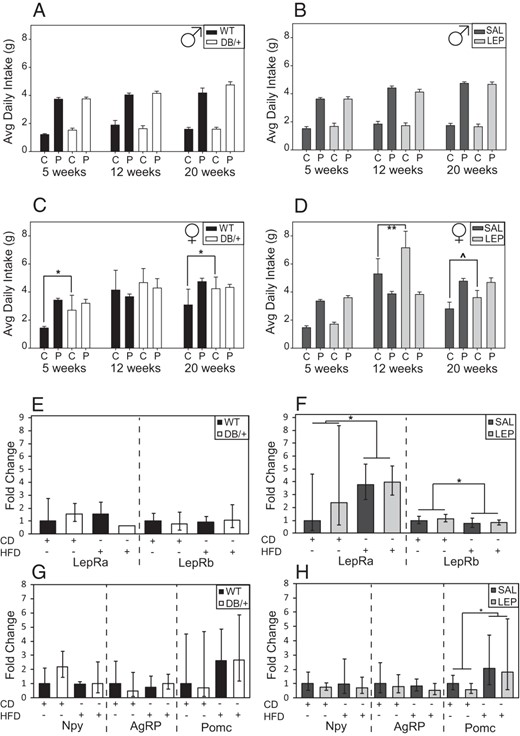
Offspring were presented with both a control diet (C or CD) and a highly PD (sweetened condensed milk mixed with CD) ab libitum. Consumption of each was measured in male (A) and female (C) offspring from WT and DB/+ dams, and of male (B) and female (D) offspring from SAL and LEP dams. Data are presented as averages and were analyzed by ANOVA split-plot in space and time with offspring nested within dam (^, P < .06; *, P < .05; **, P < .01, least square means). Relative mRNA concentrations in offspring hypothalamus at 31 weeks of age, maintained on either the control (CD) or HFD. Lepra and Leprb (E and F) and Npy, AgRP, and Pomc (G and H) genes in offspring from WT and DB/+ (E and G) and SAL and LEP (F and H) dams. Data are presented as averages ± fold change range calculated from ΔCT SDs and were analyzed by using by using three-way ANOVA with offspring nested within dam (*, P < .05, least square means) (n: males, WT = 10; DB/+ = 10; SAL = 14; and LEP = 12 and females, WT = 9; DB/+ = 6; SAL = 12; and LEP = 13).
Offspring hypothalamic gene expression
There were no significant differences related to maternal hyperleptinemia in the expression of select hypothalamic genes related to energy homeostasis and food consumption (LepRa, LepRb, Npy, AgRP, and Pomc) (Figure 4, E–H). Lepra and Pomc expression increased, and Leprb expression decreased, with HFD consumption in offspring of LEP and SAL dams (Figure 4, F and H).
Offspring locomotor activity in open field
Hyperleptinemia in DB/+ dams significantly increased locomotor activity of female and male offspring at 20 weeks of age (Figure 5). Although locomotor activity levels declined with age in male and female offspring of WT dams, they were more consistent over time in offspring of DB/+ dams (group × week interaction, P < .01), and at 20 weeks, activity was significantly higher in offspring of DB/+ dams (Figure 5, A and C). Female offspring from LEP dams displayed significantly higher locomotor activity than SAL at 12 weeks on day 1 and at 20 weeks on day 3 (P < .05). There were no significant differences in locomotor activity between male offspring from LEP and SAL dams at any age (Figure 5B). When locomotor activity was reevaluated at 27 weeks, there were no differences between male offspring of hyperleptinemic and control dams (Figure 5, E and F). After being placed on a HFD, the female offspring from DB/+ dams were significantly more active than offspring from WT dams on days 1 and 2, whereas activity did not differ between offspring of DB/+ and WT on the control diet (Figure 5G). There were no significant differences between female offspring from SAL and LEP dams (Figure 5H). There were no differences between sexes or between offspring of hyperleptinemic and control dams in the pattern of locomotor activity over time when evaluated in 5-minute intervals across each 30-minute session (data not shown); all groups displayed higher levels of activity early in the session and lower levels later, indicating habituation to the open field.
Locomotor activity

Activity in an open field was assessed for 30 minutes per day over 3 days at each age in male (A and B) and female (C and D) offspring at 5, 12, and 20 weeks from WT and DB/+ (A and C) and SAL and LEP (B and D) dams. Male (E and F) and female offspring (G and H) at 27 weeks, on a HFD or control diet. Data are presented as averages ± SE and were analyzed by using ANOVAs split-plot in space and time with offspring nested within dam (n: males, WT = 10; DB/+ = 10; SAL = 14; and LEP = 13 and females, WT = 10; DB/+ = 9; SAL = 14; and LEP = 13) (*, P < .05; **, P < .01, least square means).
Offspring coordinated motor skills
There were no differences in coordinated motor skills among the offspring of hyperleptinemic and control dams, or in relation to sex (Supplemental Figure 4), nor were there differences in learning between groups over the 3 day time course at each age. There were no impairments in rotarod activity that would reflect gross motor skill problems and that would have affected their movement in the locomotor activity or anxiety-related behavior analyses.
Offspring anxiety-related behaviors
There were no significant differences in amount of time spent in the closed sections of the zero-maze between maternal hyperleptinemic groups and their controls at any age (Supplemental Figure 5). In pairwise comparisons, female offspring from DB/+ dams had a higher number of entries into closed and open sections of the maze, compared with those from WT dams (Figure 6B). However, offspring from LEP dams had fewer entries into closed or open areas than their SAL controls independent of age and sex (Figure 6, C and D). At 28 weeks of age, on the control diet, there were significantly more exploratory behaviors (entering either open or closed areas or looking over the edge) in male offspring from DB/+ and LEP dams compared with their respective controls (Figure 6, I and K).
Exploratory behavior
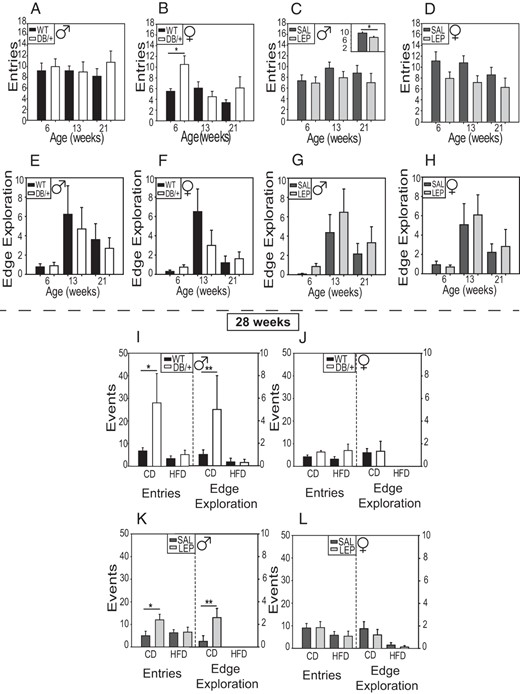
The number of entries into closed and open spaces on the zero maze (A–D) and the number of looks over the edge in open areas (E–H) by offspring at 6, 13, and 21 weeks. Number of entries was decreased in offspring from LEP dams compared with SAL (C, inset). Entries and edge explorations were reassessed at 28 weeks in offspring on the control (CD) and HFD (I–L). Males are shown in A, C, E, G, I, and K and females in B, D, F, H, J, and L. Data are presented as averages ± SE and were analyzed by using by using ANOVAs split-plot in space and time with offspring nested within dam (n: males, WT = 10; DB/+ = 10; SAL = 14; and LEP = 13 and females, WT = 10; DB/+ = 9; SAL = 14; and LEP = 13) (*, P < .05; **, P < .01, least square means).
Discussion
This study examined the role of high maternal leptin during pregnancy in developmental programming of offspring body size and the behaviors that regulate it. Hyperleptinemia is associated with both maternal obesity and GDM (11, 41), both of which predispose offspring to obesity (3, 42). The mechanisms behind this predisposition are unknown, but leptin may play a role (43). Maternal hyperleptinemia, when isolated from maternal obesity or diabetes, did not predispose offspring to excess weight gain. Rather, it had the opposite effect, by reducing weight gain, even on a HFD. Maternal hyperleptinemia also was associated with greater spontaneous locomotor activity, which may account, at least in part, for the differences in body weight (for summary, see Table 2). This indicates a protective role of maternal leptin against obesity in offspring, suggesting that leptin resistance, rather than hyperleptinemia per se, may contribute to the effects of maternal obesity and diabetes on offspring weights.
What Was Observed . | Direction of Change in Offspring From Hyperleptinemic Dams . | What It May Mean . | |
---|---|---|---|
Males . | Females . | ||
Body weight gain | ↓ | ↓ | Maternal hyperleptinemia protects against weight gain |
Food consumption | — | ↑ | Food consumption is associated with increased activity level |
Food preference | — | ↓ | Associated with increase in overall food consumption |
Locomotor activity | ↑ | ↑ | Associated with reduced weight gain |
Coordinated motor skills | — | — | |
Anxiety-like behaviors | — | — | |
Exploratory behaviors | ↑ (DB/+) ↓ (LEP) | ↑ (DB/+) ↓ (LEP) | No clear relation to maternal LEP |
What Was Observed . | Direction of Change in Offspring From Hyperleptinemic Dams . | What It May Mean . | |
---|---|---|---|
Males . | Females . | ||
Body weight gain | ↓ | ↓ | Maternal hyperleptinemia protects against weight gain |
Food consumption | — | ↑ | Food consumption is associated with increased activity level |
Food preference | — | ↓ | Associated with increase in overall food consumption |
Locomotor activity | ↑ | ↑ | Associated with reduced weight gain |
Coordinated motor skills | — | — | |
Anxiety-like behaviors | — | — | |
Exploratory behaviors | ↑ (DB/+) ↓ (LEP) | ↑ (DB/+) ↓ (LEP) | No clear relation to maternal LEP |
What Was Observed . | Direction of Change in Offspring From Hyperleptinemic Dams . | What It May Mean . | |
---|---|---|---|
Males . | Females . | ||
Body weight gain | ↓ | ↓ | Maternal hyperleptinemia protects against weight gain |
Food consumption | — | ↑ | Food consumption is associated with increased activity level |
Food preference | — | ↓ | Associated with increase in overall food consumption |
Locomotor activity | ↑ | ↑ | Associated with reduced weight gain |
Coordinated motor skills | — | — | |
Anxiety-like behaviors | — | — | |
Exploratory behaviors | ↑ (DB/+) ↓ (LEP) | ↑ (DB/+) ↓ (LEP) | No clear relation to maternal LEP |
What Was Observed . | Direction of Change in Offspring From Hyperleptinemic Dams . | What It May Mean . | |
---|---|---|---|
Males . | Females . | ||
Body weight gain | ↓ | ↓ | Maternal hyperleptinemia protects against weight gain |
Food consumption | — | ↑ | Food consumption is associated with increased activity level |
Food preference | — | ↓ | Associated with increase in overall food consumption |
Locomotor activity | ↑ | ↑ | Associated with reduced weight gain |
Coordinated motor skills | — | — | |
Anxiety-like behaviors | — | — | |
Exploratory behaviors | ↑ (DB/+) ↓ (LEP) | ↑ (DB/+) ↓ (LEP) | No clear relation to maternal LEP |
This finding is consistent with the few studies that have examined developmental programming effects of gestational hyperleptinemia. Stocker et al found that leptin administration in rats from d14 through the end of lactation protected offspring from diet-induced obesity and insulin resistance (44, 45). It increased offspring energy expenditure in both sexes and reduced weight gain in male offspring fed a HFD (45). Mouse dams heterozygous for a mutation at the agouti locus can develop hyperleptinemia before the onset of obesity, and offspring born during this period were themselves resistant to obesity (46). In the same study, injecting dams with leptin at d17 reduced offspring weights, food intake, and diet-induced obesity (46). In contrast, female offspring from mouse dams that were food restricted and administered leptin during the first half of pregnancy became obese when given a HFD, suggesting that hyperleptinemia is only beneficial in well-fed dams, or only in late pregnancy (19). With the current study, there is a growing body of evidence demonstrating that leptin during pregnancy protects offspring from becoming obese.
It is difficult to determine whether the effects observed resulted from gestational or lactational hyperleptinemia, or both. LEP females were given leptin up to 3–6 days postpartum. Secondary analysis indicates that offspring from LEP dams who were calculated to only have 3–4 days of postpartum leptin infusion had decreased weights compared with SAL controls, whereas offspring from dams with 5–6 days of postpartum leptin infusion did not differ from controls (data not shown). These data suggest that beneficial effects of maternal hyperleptinemia on offspring weights only occur when offspring are exposed during gestation and very early lactation. The DB/+ dams were not sampled during the lactation period to avoid disturbing offspring, and so serum leptin concentrations at this time were not determined. Lactation is a critical period for programming offspring metabolism (47–49). Leptin injections and even leptin feeding during this time affect the development of hypothalamic appetite control centers, and protect against weight gain, insulin resistance, and preference for high-fat foods (50–52). However, some studies have found that neonatal leptin injection affects only the offspring of dams undernourished during pregnancy, and not those of normally fed dams (53, 54). Neonatal leptin treatments likely alter the leptin surge that naturally occurs in rodents during the early neonatal period and that is necessary for hypothalamic development (55–57), and prenatal leptin may alter the surge as well. Further investigation is needed to definitively separate effects of hyperleptinemia during gestation and lactation and the mechanism of action at each time.
Some potential mechanisms by which maternal hyperleptinemia could reduce offspring weights can be ruled out. It did not suppress maternal weight gain, which was greater in hyperleptinemic dams. The maternal weight differences also suggest that hyperleptinemia did not inhibit maternal food consumption. Although leptin plays a role in neural development, maternal leptin does not directly act on the fetus, because it does not significantly cross into the fetal circulation (58, 59). Thus, maternal hyperleptinemia most likely affects offspring activity and weight via maternal metabolism and hormone production, or by altering placental function. In addition to its appetite-regulating activities, leptin activates the sympathetic nervous system to regulate functions in other tissues, such as renal, vascular, and adipose tissue (60–62). In nonpregnant animals, leptin can stimulate lipolysis and fatty acid oxidation in white adipose tissue, which could affect the availability of free fatty acids to dam and fetus if active in pregnancy (62). Additionally, leptin regulates multiple functions in the placenta, including nutrient transport, trophoblast cell differentiation, and protein synthesis (63–67). Alteration in nutrient availability and transport through the placenta affects fetal growth and development, including neuronal development (68, 69), and is a potential pathway for leptin to alter adult offspring health.
The reduction in offspring weights caused by maternal hyperleptinemia was not explained by decreased food consumption in the offspring themselves. Offspring from hyperleptinemic dams actually consumed more food at some ages in both the food consumption and preference tests, despite reduced preference for the highly PD (for summary, see Table 2). There were also no differences in expression of selected genes associated with food consumption. Thus, maternal hyperleptinemia may contribute to the higher food consumption observed in offspring of obese dams (30, 31), even though it tended to reduce offspring weights. Alternatively, higher food consumption may have resulted from higher activity levels, and not from a direct programming of feeding behavior by maternal hyperleptinemia. Our results also demonstrate that the preference for high-fat foods that has been shown in offspring of high fat-fed mothers (29, 31, 32) is not caused by hyperleptinemia, and may even be prevented by it.
We have identified one behavioral mechanism by which maternal hyperleptinemia reduces offspring weight starting at 23 weeks of age, the programming of higher activity. Both male and female offspring of DB/+ dams were more active at 20 weeks, and the females were more active at 27 weeks when fed the HFD. Female offspring of LEP dams were more active on one of the 3 days at which they were evaluated at 12 and 20 weeks. Locomotor activity was measured briefly in an open field test in this study, which may not necessarily reflect long-term spontaneous physical activity (SPA) or exercise behavior. However, the results of the zero-plus maze generally corroborated the open field test results. Although there were no differences in times spent in the open and closed arms of the maze (a measure of anxiety), female offspring of DB/+ dams entered the 2 arms more often overall, as did male offspring of DB/+ dams on the control diet at 28 weeks. This increase in exploratory behavior may reflect increased spontaneous activity, although offspring of LEP dams entered the 2 arms less often than offspring of SAL dams. Conversely, differences in locomotor activity in the open field test are unlikely to reflect exploration or anxiety, because there was no difference in habituation over the 5-minute increments of the 30-minute sessions.
SPA is a major contributor to energy balance. Energy is expended through reproduction, basal metabolic rate, digestion and food processing, thermoregulation and both voluntary exercise and SPA (70). In developed countries, SPA is a significant source of energy expenditure. Studies in both humans and rodents have shown that obese individuals are less active than individuals of normal weight and that increased SPA protects against obesity (71–74). Two studies observed that obese women sat at least 2 more hours per day, and spent half as much time in activity as lean women (73, 74). Altogether, this suggests that increased SPA may be sufficient to account for decreased body weight in offspring of hyperleptinemic dams.
However, stimulation of offspring activity by maternal hyperleptinemia may also occur under conditions where hyperleptinemia is complicated by maternal obesity or diabetes, and offspring weights are not reduced. Offspring from females on HFDs or with diabetes are more active (20, 23, 25, 26). In the rat, offspring of diabetic mothers treated with insulin were more active than either controls or diabetic mothers with poorly controlled glucose, suggesting that it is not hyperglycemia that affects offspring activity (26) but perhaps leptin, which is elevated in insulin-treated dams. In humans, children whose mothers had GDM display higher levels of hyperactivity associated with attention deficit disorder (20, 23). Maternal hyperleptinemia was not measured in these studies, but it is associated with HFD and diabetes, and thus may explain the observed increase in offspring activity, as we directly demonstrate in the current study.
A strength of this study is the use of 2 independent models of maternal hyperleptinemia. Each has potential complicating factors. Pump surgeries could induce a stress response, although saline pumps were used as a control. The degree of hyperleptinemia that could be achieved with pumps is limited by pump size, the solubility of leptin, and feedback inhibition of endogenous leptin production (75). In the DB/+ model, serum leptin concentrations were higher than achievable with the pump. The Leprdb/+ have only one functional copy of the gene encoding leptin receptor, but the effect at the protein level is unknown. In several studies, Leprdb/+ mice developed GDM (33, 76–78), but that did not occur here. Our findings are consistent with those of Harrod et al (79), who found no difference in glucose tolerance at d18 between Leprdb/+ and Lepr+/+ females on a 4%–6% g/fat diet. Glucose tolerance in Leprdb/+ may be affected by parity, diet, background strain (80), and the cosegregation of the misty allele (81). Paternal genotype may also play a role. Wild-type were mated with DB/+ males to provide the same mix of fetal genotypes as occurs in the DB/+ females. Interestingly, serum leptin levels were higher in these wild-type than in the wild-type SAL females, which were mated to wild-type males. This may be indicative of greater production of the soluble leptin receptor by DB/+ placentas, which contributes to elevated leptin concentrations during pregnancy (82). In another experiment, we found serum leptin concentrations in wild-type females impregnated by wild-type males averaged 25.3 ng/mL, comparable with SAL in this study (our unpublished data K.E.P., L.C.S.).
Overall, results from the 2 models were consistent, with larger effects in the more hyperleptinemic DB/+ model. This supports the conclusion that effects on offspring were due to hyperleptinemia and not other factors. Maternal hyperleptinemia during pregnancy does not predispose offspring to excess weight gain. It reduces offspring weight gain, even after exposure to an obesogenic diet. This is facilitated by an increase in locomotor activity.
Acknowledgments
We thank Dr William Folk for providing support for the glucose tolerance tests for the DB/+ and WT dams at d18.5; the University of Missouri Center for Translational Neuroscience, and the undergraduate research students of the Miller lab for helping conduct the behavioral tests; and Omonseigho Esangbedo and Gerialisa Caesar and undergraduates from the Schulz lab, particularly Ashley Sigafoos, for indispensable help during this study.
This work was supported by the American Diabetes Association Core Research Grant 1–14-BS-181 (to L.C.S.), the American Heart Association Fellowship 13POST16910108 (to K.A.P.), and University of Missouri Mission Enhancement (L.C.S.).
Disclosure Summary: The authors have nothing to disclose.
Abbreviations
- Agrp
agouti-related protein
- Avg
average
- CD
standard chow diet
- d
days postcoitus
- HFD
high-fat diet
- LEP
infused with leptin
- Lepra
LEP receptor a
- Npy
neuropeptide Y
- PD
palatable diet
- Pomc
proopiomelanocortin
- SAL
infused with saline
- SPA
spontaneous physical activity.