-
PDF
- Split View
-
Views
-
Cite
Cite
Qingfu Lang, Peng Xiao, Ming Zhao, Desen Liang, Qinghui Meng, Tiemin Pei, COUP-TFII promotes metastasis and epithelial-to-mesenchymal transition through upregulating Snail in human intrahepatic cholangiocarcinoma, Acta Biochimica et Biophysica Sinica, Volume 52, Issue 11, November 2020, Pages 1247–1256, https://doi.org/10.1093/abbs/gmaa117
- Share Icon Share
Abstract
Intrahepatic cholangiocarcinoma (ICC) arises from cholangiocytes in the intrahepatic bile duct and is the second most common type of liver cancer. The overexpression of COUP-TFII has been observed in several types of malignancies. However, its role in ICC progression remains unclear. In this study, we found that the protein level of COUP-TFII was increased, but the mRNA level was unchanged in ICC tissues. High protein expression was positively associated with tumor size, lymph node metastasis, and poor prognosis in ICC patients. Furthermore, the overexpression of COUP-TFII promoted the proliferation, migration, and invasion of ICC cells in vitro and enhanced tumor growth and metastasis in nude mouse models. Mechanistic studies revealed that COUP-TFII induced epithelial-to-mesenchymal transition in ICC cells by upregulating Snail expression. Moreover, the activation of PI3K/AKT signaling led to the upregulation of COUP-TFII protein expression in ICC. Together, these findings indicate that COUP-TFII promotes epithelial-to-mesenchymal transition and metastasis in ICC and suggest that this protein is a potential target for adjuvant therapy for these patients.
Introduction
Intrahepatic cholangiocarcinoma (ICC) is the second most common primary liver cancer after hepatocellular carcinoma [1]. The incidence of ICC has dramatically increased globally over the last 2 decades, and the 5-year survival rate is less than 10% [2]. Treatment options for this disease remain unsatisfactory, and early surgical resection is still the only possible curative therapeutic method [3]. Most patients are ineligible for curative surgery because of the presence of metastases at the time of diagnosis [4]. Therefore, it is urgent to explore the mechanism of ICC metastasis, which might help identify new therapeutic strategies for this disease.
During the past few years, increasing evidence has highlighted a critical role for epithelial-to-mesenchymal transition (EMT) in promoting cancer metastasis, including in ICC [5,6]. During EMT, differentiated epithelial cells lose apical-basal polarity and acquire characteristics of cell motility with decreased adhesive ability, including the downregulation of epithelial markers such as E-cadherin and the upregulation of mesenchymal markers such as N-cadherin [7]. This process is regulated by various transcription factors such as Snail, Slug, Twist1/2, and ZEB1/2 [8].
COUP-TFII, also called NR2F2, an evolutionary conserved orphan nuclear receptor, plays an important role inthe function and homeostasis of various human tissues and organs [9]. However, recent studies have uncovered a potential role for COUP-TFII in various malignant tumors [10]. Aberrant enrichment of COUP-TFII was observed in prostate cancer, and this expression profile was shown to promote malignant phenotypes in this disease [11]. Furthermore, COUP-TFII promotes the invasiveness and anchorage-independent growth of pancreatic adenocarcinoma cells, and it plays a fundamental role in neoangiogenesis by directly modulating VEGF-C [12]. COUP-TFII also promotes colorectal cancer metastasis by inducing EMT through the direct targeting of Snail [13]. However, the potential role of COUP-TFII in ICC metastasis remains largely unknown. Therefore, in this study, we sought to determine the expression, clinical significance, functional effects, and modes of action of COUP-TFII in ICC.
Materials and Methods
Patients and specimens
Eight or 16 pairs of frozen tumor and matched peritumor samples that were randomly collected from the tissue bank of the First Affiliated Hospital of Harbin Medical University were analyzed by western blot analysis and real-time PCR. A total of 52 paraffin-embedded ICC tumors and matched peritumor tissues were analyzed using immunohistochemistry (IHC). The clinical and prognostic data were collected from 2005 and 2014. All samples used in this study were approved by the Committees for the Ethical Review of Research at the First Affiliated Hospital of Harbin Medical University.
Cell culture and lentivirus infection
CCLP1 cell line was obtained from Shanghai Bioleaf Biotech Co. Ltd (Shanghai, China). HuCCT1 cell line was kindly provided by the Cancer Cell Repository of Tohoku University (Sendai, Japan). These two ICC cell lines were cultured in RPMI 1640 medium (Gibco, Grand Island, USA) supplemented with 10% fetal bovine serum (Gibco) and 100 U/ml penicillin (Gibco) at 37°C under a 5% CO2 atmosphere.
The lentiviral vectors overexpressing human COUP-TFII and Snail (LV-COUP-TFII and Snail) and their corresponding knockdown forms (LV-sh COUP-TFII and LV-sh Snail) were constructed and synthesized by Shanghai GeneChem Corporation (Shanghai, China). The lentiviral vectors were transfected into ICC cells with a multiplicity of infection (MOI) = 60 in the presence of 5 μg/ml polybrene. After transfection for 48 h, the cells were selected for 2 weeks with 2.5 μg/ml puromycin. Cells obtained without subcloning 2 weeks after drug selection were used in the subsequent experiments. Empty vectors were used as the corresponding controls.
The sequences for shRNAs targeting the Snail and COUP-TFII gene are as follows: Snail-sh RNA: 5ʹ-ACCCCAATCGGAAGCCTAACT-3ʹ, COUP-TFII-KD-1: 5ʹ-CCCTCATCCGGGATATGTT-3ʹ, COUP-TFII-KD-2: 5ʹ-GCTTTGGAAGAATACGTTA-3ʹ, and COUP-TFII-KD-3: 5ʹ-CCACATACGGATCTTCCAA-3ʹ. The sequence of control shRNA is: 5ʹ-CCGGCAACAAGATGAAGAGCACCAACTCGAGTTGGTGCTCTTCATCTTGTTGTTTTTG-3ʹ.
Ectopic expression of myr-AKT and treatment with LY294002 or MK2206
PI3K inhibitor LY294002 (L9908) was obtained from Sigma-Aldrich (St Louis, USA). AKT inhibitor MK2206 (S1078) was obtained from Selleck (Shanghai, China). ICC cells were exposed to 15 μM LY294002 or 0.2 μM MK2206 for 24 h and then analyzed by western blot analysis using COUP-TFII, AKT, and p-AKT antibodies. Control vector (pCDNA3.0) and myr-AKT plasmid were from Shanghai GeneChem Corporation. ICC cells were transfected with 1 μg of control vector or pmyr-AKT for 48 h using Lipofectamine 2000 (Invitrogen, Carlsbad, USA) following the manufacturer’s recommended procedure and then analyzed by western blot using COUP-TFII, AKT, and p-AKT antibodies.
Migration and invasion assays
Migration and invasion assays were performed using transwell chambers (8 μm) in the absence (migration) and presence (invasion) of Matrigel. Briefly, cells (1–2 × 105 cells/well) were seeded into the upper chamber with serum-free medium, and 750 μl of 20% fetal bovine serum was added to the lower chamber of the 24-well plate. After 24 h of incubation, the cells on the upper surface of the filter were completely removed by wiping with a cotton swab. Then, the filters were fixed with 4% paraformaldehyde and stained with crystal violet. The cells in three randomly selected visual fields were counted under a microscope.
Growth curve assay
To plot the growth curve, ICC cells were seeded in 6-well plates at a density of 5–6 × 103/well. Viable cells were counted daily for 1 week.
Western blot analysis
Cells were collected from cultured dishes and lysed using lysis buffer. Protein concentrations were determined using the BCA Protein Assay kit (Pierce, Rockford, USA). Protein samples (30 or 40 μg of protein per cell line) were separated by SDS-PAGE using a 6%–12% Tris-Tricine Ready Gel (Bio-Rad, Hercules, USA) and transferred onto nitrocellulose membrane. The membrane was blocked with 5% skim milk for 2 h at room temperature and incubated overnight at 4°C with the primary antibody, followed by incubation with the corresponding horseradish peroxidase-conjugated IgG secondary antibody. The immunoreactive bands were visualized by enhanced chemiluminescence. Band density was measured by densitometry, quantified using Image 1.62 Gel Map Macros (NIH, Bethesda, USA), and normalized to GAPDH in the same membrane.
The primary antibodies against E-cadherin (#3195), β-catenin (#8480), N-cadherin (#13116), Snail (#3879), and Vimentin (#5741) were purchased from Cell Signaling Technology (Danvers, USA). The primary antibody against COUP-TFII was obtained from Abcam (Cambridge, UK; ab211777). The primary antibody against GAPDH (KC-5G4) was from Shanghai Kangcheng biology Co., Ltd (Shanghai, China). The rabbit secondary antibodies (ZB2305 and ZB2301) were purchased from Beijing Zhongshan Jinqiao Biotechnology Co., Ltd (Beijing, China).
Real-time PCR
Total RNA was extracted from cell cultures using the RNAeasy Mini kit (Qiagen, Hilden, Germany). RNA was quantified with Nanodrop ND-1000 spectrophotometer (Thermo, Waltham, USA). Reverse transcription reactions were performed using 1 μg of total RNA and the High Capacity Reverse Transcription kit (Applied Biosystems, Foster City, USA) with random primers, according to the manufacturer’s instructions. Real-time PCR was performed using Power Sybr Green PCR Master Mix with an ABIPRISM 7900HT instrument (Applied Biosystems). The amplification conditions were 50°C for 2 min, 95°C for 10 min, 40 cycles of amplification at 95°C for 15 s, and 60°C for 1 min. The expression levels of indicated mRNAs were determined according to the comparative Ct (-ΔCt) method using β-actin as an internal control. The primers used are listed in Table 1.
Gene . | Primer sequence . |
---|---|
Snai1 | Forward: 5ʹ-TTACCTTCCAGCAGCCCTAC-3ʹ |
Reverse: 5ʹ-AGCCTTTCCCACTGTCCTC-3ʹ | |
Twist | Forward: 5ʹ-CGACGACAGCCTGAGCAACA-3ʹ |
Reverse: 5ʹ-CCACAGCCCGCAGACTTCTT-3ʹ | |
Slug | Forward: 5ʹ-CCTCCATCTGACACCTCC-3ʹ |
Reverse: 5ʹ-CCCAGGCTCACATATTCC-3ʹ | |
Zeb1 | Forward: 5ʹ-AAGTGGCGGTAGATGGTA-3ʹ |
Reverse: 5ʹ-TTGTAGCGACTGGATTTT-3ʹ | |
Vimentin | Forward: 5ʹ-TTGAACGCAAAGTGGAATC-3ʹ |
Reverse: 5ʹ-AGGTCAGGCTTGGAAACA-3ʹ | |
E-cadherin | Forward: 5ʹ-CTGAGAACGAGGCTAACG-3ʹ |
Reverse: 5ʹ-TTCACATCCAGCACATCC-3ʹ | |
β-catenin | Forward: 5ʹ-GAAGAGGCTGAAGGCAAAG-3ʹ |
Reverse: 5ʹ-AGCAGAGGGAATCAAACA-3ʹ | |
N-cadherin | Forward: 5ʹ-AGGATCAACCCCATACACCA-3ʹ |
Reverse: 5ʹ-TGGTTTGACCACGGTGACTA-3ʹ | |
β-actin | Forward: 5ʹ-GCGAGCATCCCCCAAAGTT-3ʹ |
Reverse: 5ʹ-GGGCACGAAGGCTCATCATT-3ʹ |
Gene . | Primer sequence . |
---|---|
Snai1 | Forward: 5ʹ-TTACCTTCCAGCAGCCCTAC-3ʹ |
Reverse: 5ʹ-AGCCTTTCCCACTGTCCTC-3ʹ | |
Twist | Forward: 5ʹ-CGACGACAGCCTGAGCAACA-3ʹ |
Reverse: 5ʹ-CCACAGCCCGCAGACTTCTT-3ʹ | |
Slug | Forward: 5ʹ-CCTCCATCTGACACCTCC-3ʹ |
Reverse: 5ʹ-CCCAGGCTCACATATTCC-3ʹ | |
Zeb1 | Forward: 5ʹ-AAGTGGCGGTAGATGGTA-3ʹ |
Reverse: 5ʹ-TTGTAGCGACTGGATTTT-3ʹ | |
Vimentin | Forward: 5ʹ-TTGAACGCAAAGTGGAATC-3ʹ |
Reverse: 5ʹ-AGGTCAGGCTTGGAAACA-3ʹ | |
E-cadherin | Forward: 5ʹ-CTGAGAACGAGGCTAACG-3ʹ |
Reverse: 5ʹ-TTCACATCCAGCACATCC-3ʹ | |
β-catenin | Forward: 5ʹ-GAAGAGGCTGAAGGCAAAG-3ʹ |
Reverse: 5ʹ-AGCAGAGGGAATCAAACA-3ʹ | |
N-cadherin | Forward: 5ʹ-AGGATCAACCCCATACACCA-3ʹ |
Reverse: 5ʹ-TGGTTTGACCACGGTGACTA-3ʹ | |
β-actin | Forward: 5ʹ-GCGAGCATCCCCCAAAGTT-3ʹ |
Reverse: 5ʹ-GGGCACGAAGGCTCATCATT-3ʹ |
Gene . | Primer sequence . |
---|---|
Snai1 | Forward: 5ʹ-TTACCTTCCAGCAGCCCTAC-3ʹ |
Reverse: 5ʹ-AGCCTTTCCCACTGTCCTC-3ʹ | |
Twist | Forward: 5ʹ-CGACGACAGCCTGAGCAACA-3ʹ |
Reverse: 5ʹ-CCACAGCCCGCAGACTTCTT-3ʹ | |
Slug | Forward: 5ʹ-CCTCCATCTGACACCTCC-3ʹ |
Reverse: 5ʹ-CCCAGGCTCACATATTCC-3ʹ | |
Zeb1 | Forward: 5ʹ-AAGTGGCGGTAGATGGTA-3ʹ |
Reverse: 5ʹ-TTGTAGCGACTGGATTTT-3ʹ | |
Vimentin | Forward: 5ʹ-TTGAACGCAAAGTGGAATC-3ʹ |
Reverse: 5ʹ-AGGTCAGGCTTGGAAACA-3ʹ | |
E-cadherin | Forward: 5ʹ-CTGAGAACGAGGCTAACG-3ʹ |
Reverse: 5ʹ-TTCACATCCAGCACATCC-3ʹ | |
β-catenin | Forward: 5ʹ-GAAGAGGCTGAAGGCAAAG-3ʹ |
Reverse: 5ʹ-AGCAGAGGGAATCAAACA-3ʹ | |
N-cadherin | Forward: 5ʹ-AGGATCAACCCCATACACCA-3ʹ |
Reverse: 5ʹ-TGGTTTGACCACGGTGACTA-3ʹ | |
β-actin | Forward: 5ʹ-GCGAGCATCCCCCAAAGTT-3ʹ |
Reverse: 5ʹ-GGGCACGAAGGCTCATCATT-3ʹ |
Gene . | Primer sequence . |
---|---|
Snai1 | Forward: 5ʹ-TTACCTTCCAGCAGCCCTAC-3ʹ |
Reverse: 5ʹ-AGCCTTTCCCACTGTCCTC-3ʹ | |
Twist | Forward: 5ʹ-CGACGACAGCCTGAGCAACA-3ʹ |
Reverse: 5ʹ-CCACAGCCCGCAGACTTCTT-3ʹ | |
Slug | Forward: 5ʹ-CCTCCATCTGACACCTCC-3ʹ |
Reverse: 5ʹ-CCCAGGCTCACATATTCC-3ʹ | |
Zeb1 | Forward: 5ʹ-AAGTGGCGGTAGATGGTA-3ʹ |
Reverse: 5ʹ-TTGTAGCGACTGGATTTT-3ʹ | |
Vimentin | Forward: 5ʹ-TTGAACGCAAAGTGGAATC-3ʹ |
Reverse: 5ʹ-AGGTCAGGCTTGGAAACA-3ʹ | |
E-cadherin | Forward: 5ʹ-CTGAGAACGAGGCTAACG-3ʹ |
Reverse: 5ʹ-TTCACATCCAGCACATCC-3ʹ | |
β-catenin | Forward: 5ʹ-GAAGAGGCTGAAGGCAAAG-3ʹ |
Reverse: 5ʹ-AGCAGAGGGAATCAAACA-3ʹ | |
N-cadherin | Forward: 5ʹ-AGGATCAACCCCATACACCA-3ʹ |
Reverse: 5ʹ-TGGTTTGACCACGGTGACTA-3ʹ | |
β-actin | Forward: 5ʹ-GCGAGCATCCCCCAAAGTT-3ʹ |
Reverse: 5ʹ-GGGCACGAAGGCTCATCATT-3ʹ |
Immunofluorescence assay
Immunofluorescence assay was performed as previously described [14]. Briefly, cells were washed with PBS, fixed with 4% paraformaldehyde, permeabilized with 0.1% triton X-100, and blocked in 2% BSA for 1 h at room temperature. Then, fixed cells were incubated with anti-E-cadherin antibody (1:50) or anti-N-cadherin antibody (1:50) overnight at 4°C, followed by incubation with Alexa Fluor® 488-conjugated or Alexa Fluor® 555-conjugated secondary antibody (Abcam). After extensive wash, images were collected with a confocal microscope (Leica Microsystems, Wetzlar, Germany).
IHC staining
IHC staining and scoring were performed as previously described [15]. Briefly, fixed specimens were incubated with anti-COUP-TFII antibody (1:200), and then with biotinylated secondary antibody (1:200; Vector Laboratories Inc., Burlingame, USA), followed by treatment with avidin-biotin-peroxidase complex (Vectastain ABComplex; Vector Laboratories Inc.). After extensive wash, diaminobenzidine chromogen was used to produce brown positive staining. The IHC reaction was scored by multiplying the proportion of positive tumor cells (PP: 0 = no positive tumor cell; 1 = <10%; 2 = 10%–50%; 3 = 51%–80%; 4 = >80% positive tumor cells) by their predominant degree of staining (SI: 0 = negative; 1 = weak; 2 = moderate; 3 = strong staining). IHC staining intensity in the tumor cells was scored independently by two pathologists, and the average was taken as the final score. The median score of COUP-TFII was used as the cutoff to segregate the patients into either the high (≥median) or low (<median) group.
Animal studies
Male BALB/c athymic nude mice (5-week-old) were obtained from the Experimental Animal Center of Shanghai Institutes for Biological Sciences (Shanghai, China) and maintained in a pathogen-free environment according to the institutional guidelines for animal care. To establish a subcutaneous xenograft model, 5 × 106 cells suspended in 150 μl PBS were subcutaneously injected into the flanks of mice. The tumors were excised after 6 weeks to calculate their volumes. For the in vivo metastasis assays, 3 × 106 cells were injected into nude mice through the tail vein, and 10 weeks later, tumor metastasis in the lungs was measured using a Berthold NIGHTOWL LB983 imaging machine with D-luciferin (Xenogen, Hopkinton, USA). After imaging, all mice were euthanized, the lungs were excised, and the metastatic nodules were counted following hematoxylin and eosin staining (H&E) staining.
Statistical analysis
GraphPad Prism v. 4.02 (GraphPad, San Diego, USA) or SPSS v. 16.0 (IBM Corp., Armonk, USA) were applied to data analysis. Results were presented as the mean ± SD. The differences between two groups were compared using Student’s t-test, while the differences among three or more groups were analyzed using one-way ANOVA method. All experiments were conducted in triplicate, and P < 0.05 was considered as an indicator of statistical significance.
Results
COUP-TFII expression is positively correlated with poor prognosis of ICC patients
To determine the clinical significance of COUP-TFII expression in ICC, we evaluated samples from a cohort of 52 human patients with ICC using immunohistochemical staining. Results showed that COUP-TFII expression was significantly upregulated in ICC tissues compared with that in adjacent non-tumor liver tissues (Fig. 1A). Similar results were also observed by western blot analysis based on eight paired ICC tissues and adjacent non-tumor liver tissues (Fig. 1B). However, COUP-TFII mRNA levels showed little difference between 16 paired ICC tissues and adjacent non-tumor liver tissues (Fig. 1C), suggesting the post-transcriptional regulation of COUP-TFII expression in ICC. Correlation analysis of COUP-TFII protein levels and clinicopathologic features revealed a significant association between COUP-TFII upregulation and tumor size or lymph node metastasis (Table 2). Most importantly, patients with high COUP-TFII expression had shorter overall survival time and lower disease-free survival rate (Fig. 1D,E).
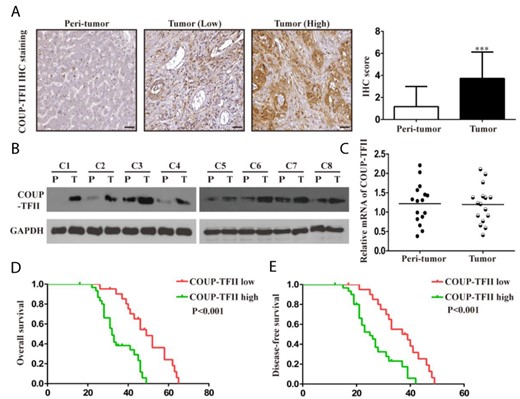
COUP-TFII expression positively correlates with poor prognosis of ICC patients (A) COUP-TFII expression was detected by IHC in 52 pairs of ICC and adjacent non-tumorous tissues using a specific antibody against COUP-TFII. Scale bar: 100 µm. (B) Representative western blot analysis of COUP-TFII expression in 8 pairs of ICC and adjacent non-tumorous tissues. P, peritumor; T, tumor. (C) Real-time PCR analysis of COUP-TFII mRNA expression in 16 pairs of ICC and adjacent non-tumorous tissues. (D, E) Kaplan–Meier plot of overall survival and disease-free survival of patients with ICC stratified by COUP-TFII expression. The log-rank test was used to compare differences between groups (n = 52). Data are expressed as the mean ± SD. The results are representative of three independent experiments. ***P < 0.001.
Relationship between COUP-TFII expression and clinicopathologic features of ICC patients (n = 52)
Variables . | COUP-TFII . | ||
---|---|---|---|
. | Low . | High . | P-value . |
Age | |||
>60 years | 9 | 15 | 0.695 |
≤60 years | 12 | 16 | |
Gender | |||
Male | 12 | 19 | 0.765 |
Female | 9 | 12 | |
Liver cirrhosis | |||
Yes | 10 | 13 | 0.686 |
No | `11 | 18 | |
Tumor size | |||
>5 cm | 6 | 20 | 0.011 |
≤5 cm | 15 | 11 | |
Tumor differentiation | |||
Well/moderate | 11 | 16 | 0.957 |
Poor | 10 | 15 | |
Lymph node metastasis | |||
Yes | 5 | 23 | 0.008 |
No | 16 | 8 | |
Vascular invasion | |||
Yes | 10 | 18 | 0.458 |
No | 11 | 13 |
Variables . | COUP-TFII . | ||
---|---|---|---|
. | Low . | High . | P-value . |
Age | |||
>60 years | 9 | 15 | 0.695 |
≤60 years | 12 | 16 | |
Gender | |||
Male | 12 | 19 | 0.765 |
Female | 9 | 12 | |
Liver cirrhosis | |||
Yes | 10 | 13 | 0.686 |
No | `11 | 18 | |
Tumor size | |||
>5 cm | 6 | 20 | 0.011 |
≤5 cm | 15 | 11 | |
Tumor differentiation | |||
Well/moderate | 11 | 16 | 0.957 |
Poor | 10 | 15 | |
Lymph node metastasis | |||
Yes | 5 | 23 | 0.008 |
No | 16 | 8 | |
Vascular invasion | |||
Yes | 10 | 18 | 0.458 |
No | 11 | 13 |
Relationship between COUP-TFII expression and clinicopathologic features of ICC patients (n = 52)
Variables . | COUP-TFII . | ||
---|---|---|---|
. | Low . | High . | P-value . |
Age | |||
>60 years | 9 | 15 | 0.695 |
≤60 years | 12 | 16 | |
Gender | |||
Male | 12 | 19 | 0.765 |
Female | 9 | 12 | |
Liver cirrhosis | |||
Yes | 10 | 13 | 0.686 |
No | `11 | 18 | |
Tumor size | |||
>5 cm | 6 | 20 | 0.011 |
≤5 cm | 15 | 11 | |
Tumor differentiation | |||
Well/moderate | 11 | 16 | 0.957 |
Poor | 10 | 15 | |
Lymph node metastasis | |||
Yes | 5 | 23 | 0.008 |
No | 16 | 8 | |
Vascular invasion | |||
Yes | 10 | 18 | 0.458 |
No | 11 | 13 |
Variables . | COUP-TFII . | ||
---|---|---|---|
. | Low . | High . | P-value . |
Age | |||
>60 years | 9 | 15 | 0.695 |
≤60 years | 12 | 16 | |
Gender | |||
Male | 12 | 19 | 0.765 |
Female | 9 | 12 | |
Liver cirrhosis | |||
Yes | 10 | 13 | 0.686 |
No | `11 | 18 | |
Tumor size | |||
>5 cm | 6 | 20 | 0.011 |
≤5 cm | 15 | 11 | |
Tumor differentiation | |||
Well/moderate | 11 | 16 | 0.957 |
Poor | 10 | 15 | |
Lymph node metastasis | |||
Yes | 5 | 23 | 0.008 |
No | 16 | 8 | |
Vascular invasion | |||
Yes | 10 | 18 | 0.458 |
No | 11 | 13 |
COUP-TFII promotes the proliferation, migration, and invasion of ICC cells in vitro
To determine whether COUP-TFII has oncogenic functions, we transfected lentiviral vectors overexpressing COUP-TFII into CCLP1 cells with low expression of COUP-TFII (Fig. 2A and Supplementary Fig. S1). Three lenti-shRNAs were also generated to silence endogenous COUP-TFII expression in HuCCT1 cells with high expression of COUP-TFII. COUP-TFII was strongly downregulated by lenti-shRNA1 (KD-1) and moderately downregulated by KD-2 and KD-3 relative to the levels with the control shRNA (Fig. 2A and Supplementary Fig. S1). Therefore, KD-1 was chosen for further experiments. The growth curve assay revealed that COUP-TFII overexpression significantly increased the proliferation of CCLP1 cells relative to the control by the fifth day (Fig. 2B). Conversely, COUP-TFII knockdown significantly suppressed HuCCT1 proliferation by day 5 (Fig. 2B). Transwell migration and matrigel invasion assays showed that the upregulation of COUP-TFII enhanced the migration and invasion of CCLP1 cells, whereas the inhibition of COUP-TFII in HuCCT1 cells decreased cell migration and invasion at 24 h (Fig. 2C,D).
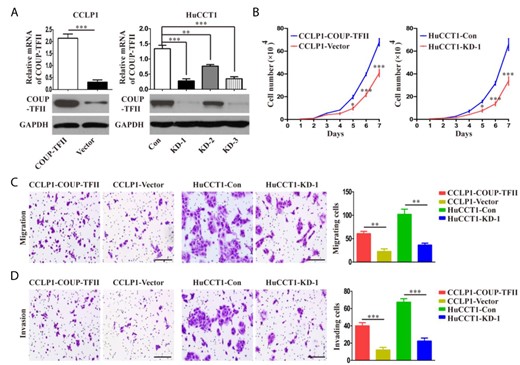
COUP-TFII promotes proliferation, migration, and invasion of ICC cells in vitro (A) The mRNA expression and protein expression of COUP-TFII in ICC cell lines after COUP-TFII knockdown or overexpression. (B) Cell proliferation was detected by growth curve assay in the indicated cell lines. (C, D) The motility and invasion abilities of ICC cells were determined by transwell assays, and statistics are shown with a bar graph. Scale bar: 100 μm. Data are expressed as the mean ± SD. The results are representative of three independent experiments. *P < 0.05, **P < 0.01, and ***P < 0.001.
COUP-TFII promotes the growth and metastasis of ICC in vivo
Furthermore, a subcutaneous xenograft mouse model was established to explore the role of COUP-TFII in tumorigenesis in vivo. Results showed that tumor volume and weight were increased in the COUP-TFII-overexpressing group but were decreased in mice inoculated with COUP-TFII-knockdown cells, as compared with those in the control group (Fig. 3A). To explore the role of COUP-TFII in metastasis in vivo, lung metastasis models were established by injecting stably transfected cell lines into nude mice via the tail vein. The number of nodules and incidence of metastasis were drastically increased in the COUP-TFII-overexpressing group but were significantly decreased in the COUP-TFII-knockdown group, as compared with those in the control group (Fig. 3B-D). The presence of pulmonary metastatic nodules was also tested using H&E on dissected lung tissue (Fig. 3E).
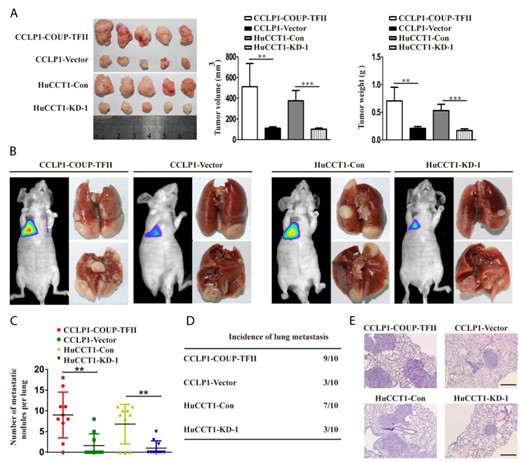
COUP-TFII promotes the growth and metastasis of ICC in vivo (A) COUP-TFII upregulation enhanced CCLP1 cell xenograft tumor growth in nude mice, while COUP-TFII knockdown reduced HuCCT1 growth. (B) Lung metastasis models were established as described in the “Materials and Methods”. Representative bioluminescence images and gross specimens shown for the indicated cell lines. (C) Histogram showing the number of metastatic nodules in the different groups. (D) Incidence data of lung metastasis in the different groups. (E) Images showing representative hematoxylin and eosin staining of lung tissue samples from the different groups. Scale bar: 100 μm. Data are expressed as the mean ± SD. The results are representative of three independent experiments. **P < 0.01, ***P < 0.001.
COUP-TFII induces EMT by upregulating Snail expression in ICC cells
To explore whether COUP-TFII controls the EMT process, the expressions of epithelial and mesenchymal markers were examined. The results of western blot analysis and real-time PCR showed that the epithelial markers E-cadherin and β-catenin were downregulated, whereas the mesenchymal markers N-cadherin and Vimentin were upregulated in COUP-TFII-overexpressing cells compared with those in control cells (Fig. 4A,B). Conversely, COUP-TFII silencing increased the expressions of epithelial markers while suppressing that of mesenchymal markers (Fig. 4A,B). Similar results were obtained in immunofluorescence assays (Fig. 4C). Zeb1, Slug, Snail, and Twist have been found to act as oncogenic transcription factors by suppressing E-cadherin expression [8]. Accordingly, we investigated whether COUP-TFII could promote EMT by regulating the expressions of these transcription factors. Real-time PCR analysis showed that the overexpression of COUP-TFII markedly increased the mRNA expression of Snail but had no significant effect on the mRNA levels of Twist, Slug, or Zeb1 (Fig. 4D). Moreover, the overexpression of COUP-TFII upregulated Snail expression and decreased E-cadherin expression in CCLP1 cells, whereas the inhibition of Snail expression significantly increased the loss of E-cadherin expression induced by COUP-TFII (Fig. 4E). In contrast, the knockdown of COUP-TFII decreased Snail expression and increased E-cadherin expression in HuCCT1 cells, whereas the overexpression of Snail markedly inhibited the increase of E-cadherin expression in COUP-TFII-knockdown cells (Fig. 4E).
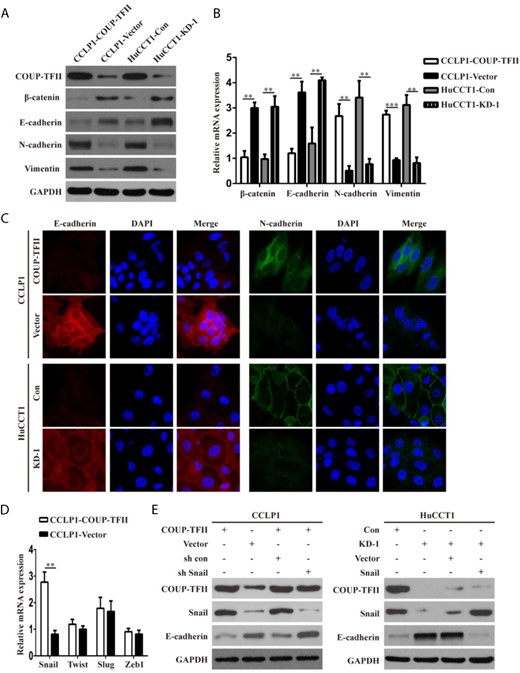
COUP-TFII induces EMT through upregulating Snail expression in ICC cells (A-C) Western blot analysis, real-time PCR, and immunofluorescence staining showing the decreased expressions of epithelial markers (E-cadherin and β-catenin) and enhanced expressions of mesenchymal markers (N-cadherin and vimentin) in CCLP1-COUP-TFII cells. However, knockdown of COUP-TFII resulted in enhanced expressions of epithelial markers and reduced expressions of mesenchymal markers in HuCCT1 cells. (D) Effect of COUP-TFII on expressions of Snai1, Twist, Slug, and Zeb1. (E) Knockdown of Snai1 expression significantly attenuated the loss of E-cadherin expression induced by overexpression of COUP-TFII. In contrast, upregulation of Snail inhibited the increase of E-cadherin expression in HuCCT1-sh COUP-TFII cells. Data are expressed as the mean ± SD. The results are representative of three independent experiments. **P < 0.01, ***P < 0.001.
COUP-TFII promotes ICC invasion and metastasis by increasing Snail expression
We found that the invasive capabilities of ICC cells were repressed by the knockdown of Snail in CCLP1-COUP-TFII cells (Fig. 5A). In contrast, COUP-TFII-knockdown-induced invasive abilities were restored with the ectopic expression of Snail (Fig. 5B). Furthermore, it was shown that tumor lung metastasis was significantly decreased by COUP-TFII knockdown and significantly recovered by Snail overexpression (Fig. 5C–E).
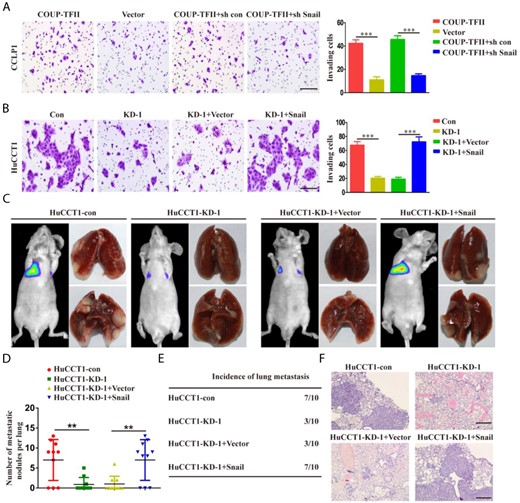
COUP-TFII promotes ICC invasion and metastasis by increasing Snail expression (A) After CCLP1-COUP-TFII cells were infected with LV-sh con or LV-sh Snail, invasion abilities of the cells were detected by transwell assays. (B) After HuCCT1-KD-1 cells were infected with the LV-Vector or LV-Snail, invasion abilities of the cells were detected by transwell assays. (C) Luciferase-expressing cell lines (HuCCT1-sh COUP-TFII, HuCCT1-sh COUP-TFII plus Snai1, and their control cells) were injected via the tail vein into the nude mice. Representative bioluminescence images and gross specimens shown for the indicated cell lines. (D) Histogram showing the number of metastatic nodules in the different groups. (E) Incidence data of lung metastasis in the different groups. (F) Images showing representative hematoxylin and eosin staining of lung tissue samples from the different experimental groups. Scale bar: 100 μm. Data are expressed as the mean ± SD. The results are representative of three independent experiments. **P < 0.01, ***P < 0.001.
Regulation of COUP-TFII protein levels by the PI3K/AKT signaling pathway
A previous report showed that the PI3K/AKT pathway could affect COUP-TFII protein levels [16]; we therefore explored whether this pathway could increase COUP-TFII expression at the post-transcriptional level. Ectopic expression of the active myr-AKT plasmid significantly increased the protein expression of COUP-TFII in CCLP1 cells, whereas administration of the PI3K inhibitor LY294002 or AKT inhibitor MK2206 resulted in significantly decreased protein expression of COUP-TFII in HuCCT1 cells (Fig. 6A). However, the mRNA expression of COUP-TFII showed little change (Fig. 6B). We further analyzed the expression levels of COUP-TFII and p-AKT in clinical ICC samples, and the results showed that COUP-TFII and p-AKT expressions were positively correlated in ICC tissues (Fig. 6C,D).
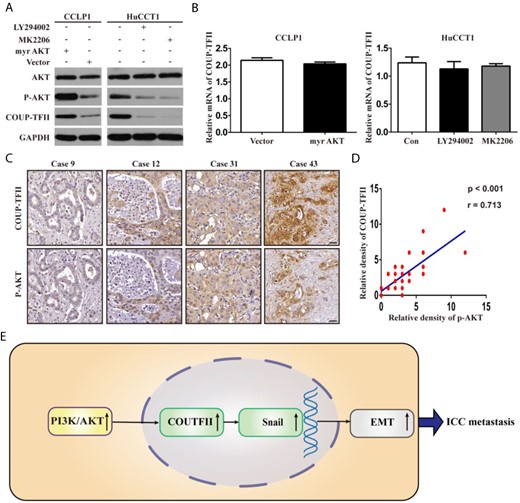
Regulation of COUP-TFII protein level by PI3K/AKT signaling pathway (A,B) After activation or inhibition of PI3K/AKT signaling pathway through ectopic expression of active myr-AKT plasmid or administration of LY294002 or MK2206, the protein and mRNA expressions of COUP-TFII were detected. (C, D) Relative protein densities of COUP-TFII and p-AKT in ICC tissues were shown. COUP-TFII overexpression was significantly positively correlated with p-AKT upregulation in human ICC tissues. (E) Schematic presentation of the mechanism underlying COUP-TFII-facilitated ICC metastasis. Data are expressed as the mean ± SD. The results are representative of three independent experiments.
Discussion
In the present study, we reported that the protein expression of COUP-TFII is frequently upregulated in human ICC and that high expression is associated with tumor status, lymph node status, and poor prognosis in ICC patients. COUP-TFII exhibits tumor-promoting activities with respect to ICC cell growth and metastasis by inducing EMT through the upregulation of Snail. Furthermore, activation of the PI3K/AKT signaling pathway leads to the upregulation of COUP-TFII protein expression in ICC.
Our results revealed that COUP-TFII expression is overexpressed in ICC at the protein level but not at the mRNA level, suggesting that ICC-promoting factors and/or signaling pathways might upregulate COUP-TFII expression in ICC cells at the post-transcriptional level. It was reported that PI3K/AKT signaling is involved in regulating COUP-TFII protein expression [16]. This pathway is a core regulator of cell growth and metastasis and is involved in ICC carcinogenesis and progression [17]. Our results showed that the inhibition or activation of PI3K/AKT signaling led to the reduction or upregulation of COUP-TFII protein expression, with little effect on COUP-TFII mRNA level. Furthermore, we found a positive correlation between COUP-TFII and p-AKT protein expression. Therefore, we speculated that the PI3K/AKT signaling pathway might be the main mechanism leading to the upregulation of COUP-TFII protein expression in ICC.
EMT plays essential roles in invasiveness and metastasis in various malignant tumors, including ICC [18]. During EMT, cancer cells lose cell-cell adhesion and obtain migratory abilities to break away from neighboring cells and invade adjacent cell layers [19]. The role of COUP-TFII in EMT is controversial. Bao et al. [20] reported that COUP-TFII promotes EMT by inhibiting the expression of miR-34a in colorectal cancer. However, Zhang et al. [21] reported that high COUP-TFII expression is associated with increased survival and that its expression inhibits TGF-β-dependent EMT in breast cancer. Our results showed that COUP-TFII increased the expressions of mesenchymal markers (N-cadherin and Vimentin) and decreased the expressions of epithelial markers (E-cadherin and β-catenin). Specifically, the quantitative reduction in the epithelial marker protein E-cadherin paralleled an increase in the mesenchymal marker protein N-cadherin on the cell membrane. Thus, as in colorectal cancer, high COUP-TFII expression can also induce EMT in ICC cells.
Regarding the mechanism whereby COUP-TFII induces EMT, this is probably mediated by several transcription factors, including Zeb1, Slug, Snail, and Twist, which directly repress E-cadherin transcription [22]. Our results showed that the overexpression of COUP-TFII induced EMT by increasing the expression of Snail but not Zeb1, Slug, or Twist. Furthermore, Snail was found to be indispensable for the COUP-TFII-enhanced invasion and metastasis in ICC. Recently, it was found that Snail is a direct transcriptional target of COUP-TFII in colorectal cancer cells [13]. Therefore, COUP-TFII might also induce the EMT process by directly targeting Snail in ICC.
In summary, our data indicated that COUP-TFII is a candidate oncogene that is significantly upregulated by PI3K/AKT signaling and potently induces the EMT process by targeting Snail in ICC (Fig. 6E). With these discoveries, we could further understand the mechanism underlying ICC metastasis, which might provide novel targets for ICC treatment in the future.
Supplementary Data
Supplementary Data is available at Acta Biochimica et Biophysica Sinica online.
Funding
This work was supported by the grants from the National Natural Science Foundation of China (No. 81602050), the Foundation of the First Affiliated Hospital of Harbin Medical University (No. 201713008), the China Postdoctoral Science Foundation\break (Nos. 2018M631947 and 2020T130158), the Heilongjiang Postdoctoral Foundation (No. LBH-Z18125), the Heilongjiang Province General Undergraduate Colleges and Universities Young Innovative Talents Training Plan (No. UNPYSCT-2017063), and the Wu Jieping Medical Foundation (No. 320.6750.16018).