-
PDF
- Split View
-
Views
-
Cite
Cite
Chenchen Ji, Haixing Li, Jingli Ding, Lu Yu, Cuncang Jiang, Chuang Wang, Sheliang Wang, Guangda Ding, Lei Shi, Fangsen Xu, Hongmei Cai, Rice transcription factor OsWRKY37 positively regulates flowering time and grain fertility under copper deficiency, Plant Physiology, Volume 195, Issue 3, July 2024, Pages 2195–2212, https://doi.org/10.1093/plphys/kiae187
- Share Icon Share
Abstract
Efficient uptake, translocation, and distribution of Cu to rice (Oryza sativa) spikelets is crucial for flowering and yield production. However, the regulatory factors involved in this process remain unidentified. In this study, we isolated a WRKY transcription factor gene induced by Cu deficiency, OsWRKY37, and characterized its regulatory role in Cu uptake and transport in rice. OsWRKY37 was highly expressed in rice roots, nodes, leaf vascular bundles, and anthers. Overexpression of OsWRKY37 promoted the uptake and root-to-shoot translocation of Cu in rice under −Cu condition but not under +Cu condition. While mutation of OsWRKY37 significantly decreased Cu concentrations in the stamen, the root-to-shoot translocation and distribution ratio in brown rice affected pollen development, delayed flowering time, decreased fertility, and reduced grain yield under −Cu condition. yeast one-hybrid, transient co-expression and EMSAs, together with in situ RT-PCR and RT-qPCR analysis, showed that OsWRKY37 could directly bind to the upstream promoter region of OsCOPT6 (copper transporter) and OsYSL16 (yellow stripe-like protein) and positively activate their expression levels. Analyses of oscopt6 mutants further validated its important role in Cu uptake in rice. Our study demonstrated that OsWRKY37 acts as a positive regulator involved in the uptake, root-to-shoot translocation, and distribution of Cu through activating the expression of OsCOPT6 and OsYSL16, which is important for pollen development, flowering, fertility, and grain yield in rice under Cu deficient conditions. Our results provide a genetic strategy for improving rice yield under Cu deficient condition.
Introduction
Rice (Oryza sativa) is the main source of energy for more than half of the world's population, and feeds more than 65% of the population in China (Zhang et al. 2005). In rice, grain yield is a complex trait mainly determined by panicle number per plant, grain number per panicle, grain weight, fertility, and flowering time (Ikeda et al. 2013; Nutan et al. 2020). Flowering time substantially affects plant fitness and crop yield (Hong and Jackson 2015; Ishimaru et al. 2021).
Copper (Cu) is an essential micronutrient that plays a core role in several biological processes in plants, such as photosynthesis, respiration, antioxidation, cell wall formation, and phytohormone sensing (Maksymiec 1997; Pilon et al. 2006; Burkhead et al. 2009). Cu deficiency may cause stunted growth, deformed or whitened young leaves, damaged apical meristems, and reduced productivity (Burkhead et al. 2009). Recent studies have reported that Cu has a great impact on the flowering time, anther formation, pollen activity, and seed setting in plants (Yan et al. 2017; Andrés-Colás et al. 2018; Zhang et al. 2018; Ishka and Vatamaniuk 2020; Sheng et al. 2021; Ishka et al. 2022). However, the mobility and availability of Cu in soil is affected by various environmental factors, such as moisture, pH, and organic matter (Solberg et al. 1999). The sandy, calcareous, alkaline, black, transitional gray-black, and dark brown soils are prone to be Cu deficient (Solberg et al. 1999; Mengel et al. 2001; Shotyk 2020). There are soils with varying degrees of Cu deficiency in China, especially in the main rice producing areas, including the Northeast, Southwest, and South of China, where 20% to 34.4% of the soil is Cu deficient (Zhang et al. 2020; 2022). Cu becomes a limiting factor for crop yield production in these regions due to its vital role in plant growth and development (Malhi et al. 2005; Li et al. 2006). The grain yield could be increased by 4.9% to 16.1% and 10.1% to 13.8% in wheat (Triticum aestivum) and rice respectively, after the application of copper fertilizers in these areas (Yang et al. 2010; Mo 2018). Therefore, improving the efficiency of uptake, translocation, and utilization of Cu in plants is important for the crop yield production.
In vascular plants, several transporters are involved in Cu uptake and transport, including Cu transporter (COPT), yellow stripe-like protein (YSL), heavy metal ATPase (HMA), and the zinc/iron transporter-like protein (ZIP) (García-Molina et al. 2011; Pilon 2011; Zheng et al. 2012; Huang et al. 2016). The high-affinity Cu transporter COPT family has been reported to have five members in purple false brome (Brachypodium distachyon), six members in Arabidopsis (Arabidopsis thaliana), and seven members in rice (Sancenón et al. 2003; Yuan et al. 2011; Jung et al. 2014). In Arabidopsis, AtCOPT1 plays a major role in Cu acquisition in root and affects pollen development (Sancenón et al. 2004). AtCOPT2 is a plasma membrane-localized Cu transporter that plays a role in both Cu acquisition and distribution, affecting photosynthesis in response to Cu deficiency (Perea-García et al. 2013). In addition, the expression of AtCOPT2 can be induced by cadmium (Cd), which results in a higher Cu accumulation by Cd treatment (Gayomba et al. 2013). AtCOPT3, encoding an intracellular Cu transporter, is mainly expressed in pollen grains and vascular bundles, and has an important role in pollen morphology (Andrés-Colás et al. 2018). AtCOPT5 can be reactivated by Cu removal from anterior vesicles in response to Cu deficiency (García-Molina et al. 2011). AtCOPT6 is mainly expressed in the vasculature and localized on the plasma membrane (Jung et al. 2012). It can interact with itself and with its homolog, AtCOPT1, and involved in the distribution of Cu into nascent tissues and seeds (Jung et al. 2012; García-Molina et al. 2013). However, AtCOPT4 does not function to transport Cu (Sancenón et al. 2003). In rice, all these homologous genes are capable of transporting Cu in yeast (Saccharomyces cerevisiae) individually or by forming complexes with each other. OsCOPT1, OsCOPT5, and OsCOPT6 are induced by Cu deficiency in rice roots (Yuan et al. 2011). The mutation of OsCOPT1 and OsCOPT5 can reduce Cu accumulation in shoots (Yao et al. 2022). AtYSL1, AtYSL2, and AtYSL3 can transport Fe- (iron-), Zn- (zinc-), and Cu-nicotianamine complexes, thereby affect the distribution of these metals in Arabidopsis (DiDonato et al. 2004; Waters et al. 2006; Waters and Grusak 2008). There are 18 members in YSL transporter family in rice, playing different roles in the transport and distribution of metal ions (Koike et al. 2004). Among them, OsYSL16 is involved in Cu distribution and redistribution in rice, and affects pollen development and yield (Zheng et al. 2012; Zhang et al. 2018).
In recent years, the important roles of transcription factors in maintaining Cu homeostasis in plants have been reported. For example, the SQUAMOSA PROMOTER BINDING PROTEIN-LIKE7 (SPL7) has been recognized as a core transcription factor involved in the response to Cu deficiency in Arabidopsis, which mediates Cu uptake and Cu homeostasis through modulating the expression levels of Cu proteins, Cu transporters, and a Cu chaperone directly or indirectly via Cu-miRNAs, including miR397, miR398, miR408, and miR857 (Yamasaki et al. 2009). SPL7 is also required for the FRO4/FRO5 (FERRIC REDUCTASE OXIDASE)-dependent Cu2+-specific reduction to Cu+, which is important for the high-affinity root uptake of Cu in Arabidopsis under Cu deficient condition (Bernal et al. 2012). In addition, another two transcription factors CITF1 and CITF2 (Cu-DEFICIENCY INDUCED TRANSCRIPTION FACTOR) have been reported to act together with SPL7 for Cu uptake into roots, the delivery of Cu to anthers, and transducing energy availability into growth and reproductive organs, which is important for the pollen fertility and yield production (Yan et al. 2017; Schulten et al. 2022). The FIT (FER-LIKE IRON DEFICIENCY-INDUCED TRANSCRIPTION FACTOR) and bHLH Ib members (bHLH38, bHLH39, bHLH100, and bHLH101) are the key regulators of Fe homeostasis, and they can directly bind to the promoters of AtCOPT2, AtFRO4, and AtFRO5 to increase Cu uptake in Arabidopsis under Fe-deficiency condition (Cai et al. 2021). The phytochrome-interacting factors PIF3/4/5 can directly bind to the promoter of miR408 to mediate intracellular Cu redistribution in Arabidopsis leaves (Hao et al. 2022). Furthermore, TCP16 (named after TEOSINTE BRANCHED 1, CYCLOIDEA and PROLIFERATING CELL FACTOR 1) has been reported to bind to the promoter of AtCOPT3 and downregulate its expression to alter Cu content and affect pollen development in Arabidopsis (Andrés-Colás et al. 2018).
WRKY is one of the largest transcription factor families in plants with a highly conserved WRKYGQK sequence and a zinc finger motif that specifically binds to W-box [(T/C)TGAC(T/C)] in the promoters of downstream target genes (Eulgem et al. 2000; Rushton et al. 2010; Chi et al. 2013). How WRKY regulates Cu uptake, translocation, and distribution in rice have been rarely elucidated. In this study, we identified a transcription factor, OsWRKY37, induced by Cu deficiency in rice root, and characterized its regulatory role in the uptake, root-to-shoot translocation, and distribution of Cu in rice. Knockout of OsWRKY37 resulted in a delayed flowering time, and decreased the fertility and grain yield under Cu deficient condition. Further analyses demonstrated that OsWRKY37 was a positive regulator involved in Cu uptake and distribution through directly binding to the promoters of OsCOPT6 and OsYSL16 and upregulating their expression levels. Our results provide a potential genetic strategy for grain yield improvement of rice under Cu deficient condition.
Results
Identification of OsWRKY37 as a transcription factor regulating flowering time under Cu deficient condition
WRKY is one of the largest transcription factor families in plants with various biological functions (Eulgem et al. 2000; Rushton et al. 2010). How WRKY regulates Cu uptake, translocation, and distribution in rice has been rarely elucidated. To identify the potential regulatory factors in response to Cu deficiency in rice, transcriptomic profiles of rice roots treated with different concentrations of Cu at seedling stage were analyzed by RNA sequencing. Eight WRKY transcription factors have been found to be substantially induced by Cu deficiency (Supplementary Tables S1 and S2). To further confirm these results, RT-qPCR was used to analyze the relative expression levels of these WRKY genes in both roots and shoots of rice grown under −Cu and +Cu conditions at seedling stage. Quite similar results were observed that these eight WRKY genes were all induced by −Cu in the roots, while only OsWRKY48, OsWRKY108, and OsWRKY81 were induced by −Cu in the shoots (Supplementary Fig. S1).
To investigate the possible functions of these WRKYs, knockout mutants were created by CRISPR/Cas9-mediated targeted mutagenesis technique (Supplementary Fig. S2). There were 2 bp deletion and 7 bp replacement in OsWRKY37 CR-L13 line, 17 bp deletion and 1 bp replacement in OsWRKY37 CR-L16 line (Supplementary Fig. S2A). There were 1 bp insertion, 2 bp deletion, and 1 bp replacement in OsWRKY48 CR-L8 line, 3 bp replacement and 6 bp deletion in OsWRKY48 CR-L9 line (Supplementary Fig. S2B). There were 6 bp deletion and 4 bp replacement in OsWRKY54 CR-L4 line, 1 bp insertion and 13 bp deletion in OsWRKY54 CR-L10 line (Supplementary Fig. S2C). There were 2 bp replacement and 5 bp deletion in OsWRKY71 CR-L1 line, 4 bp replacement and 4 bp deletion in OsWRKY71 CR-L7 line (Supplementary Fig. S2D). There were 4 bp replacement and 10 bp deletion in OsWRKY74 CR-L11 line, 1 bp insertion, 9 bp deletion, and 1 bp replacement in OsWRKY74 CR-L19 line (Supplementary Fig. S2E). There were 6 bp deletion and 5 bp replacement in OsWRKY77 CR-L1 line, 1 bp insertion, 5 bp deletion, and 4 bp replacement in OsWRKY77 CR-L6 line (Supplementary Fig. S2F). There were 1 bp insertion and 7 bp deletion in OsWRKY81 CR-L3 line, 4 bp replacement and 7 bp deletion in OsWRKY81 CR-L5 line (Supplementary Fig. S2G). There were 1 bp insertion, 5 bp deletion, and 2 bp replacement in OsWRKY108 CR-L3 line, 4 bp replacement and 5 bp deletion in OsWRKY108 CR-L22 line (Supplementary Fig. S2H).
Plants of T2 generation were grown in Cu deficient soil. At heading and flowering stage, we found that the OsWRKY37 knockout lines showed delayed flowering time when compared to the wild type, but not other WRKY knockout mutants (Supplementary Fig. S3A). The heading time of OsWRKY37 knockout lines was about 8 d later than wild type (Supplementary Fig. S3B). As Cu deficiency has a great impact on the flowering time, anther formation, pollen activity, and seed setting in plants (Yan et al. 2017; Andrés-Colás et al. 2018; Zhang et al. 2018; Ishka and Vatamaniuk 2020; Sheng et al. 2021; Ishka et al. 2022), then we speculated that OsWRKY37 was an important regulator involved in Cu uptake and transport in rice plant.
Subcellular localization and transactivation activity of OsWRKY37
The full-length cDNA of OsWRKY37 was fused with GFP, cloned into a transient expression vector initiated by 35S promoter, and transformed into rice protoplasts. Results showed that the green fluorescence signal of OsWRKY37-GFP was completely overlapped with the red fluorescence signal of the nucleus localized marker AtFIB2-mcherry (Supplementary Fig. S4A), which suggested that OsWRKY37 was a nucleus localized protein.
To determine whether OsWRKY37 has transactivation activity, the transactivation assay was performed in yeast. Results showed that yeast cells containing OsWRKY37-pGBKT7 and pGBKT7 plasmids grew normally on SD/-Trp medium (Supplementary Fig. S4B). On SD/-Trp/-His medium, yeast cells containing pGBKT7 plasmid could not grow, while the yeast cells containing OsWRKY37-pGBKT7 plasmid could grow normally and could be colored by X-Gal (Supplementary Fig. S4B), which suggested that OsWRKY37 could activate the expression of downstream reporter genes. Therefore, OsWRKY37 is a functional transcription factor with transactivation activity.
Expression pattern of OsWRKY37
The expression pattern of OsWRKY37 in different tissues of rice at different growth stages was analyzed using RT-qPCR. Results showed that the expression level of OsWRKY37 was relatively low at the seedling stage, while it was relatively high at the flowering stage, followed by those at tillering and grain filling stage (Fig. 1A). The expression level of OsWRKY37 was higher in roots than other tissues at both seedling and tillering stages, while it was constitutively expressed in all tissues except the panicle at the grain filling stage (Fig. 1A). Interestingly, OsWRKY37 was strongly expressed in roots, basal region, old leaves, nodes, and internodes at flowering stage (Fig. 1A). To further confirm this expression pattern of OsWRKY37 in rice plant, a 2,490-bp promoter fragment was constructed to the upstream of GUS reporter gene and transformed into ZH11 to generate ProWRKY37:GUS transgenic lines. GUS activities were observed in root, basal region, node, leaf vein, lamina joint, immature panicle, and anther (Fig. 1, B to L), and relatively higher GUS activities were observed in vascular bundles of leaf blade, lamina joint, and node (Fig. 1, G to J). In situ RT-PCR analyses of the root transverse section showed that OsWRKY37 was expressed in the epidermis, cortex, and stele (Fig. 1M).
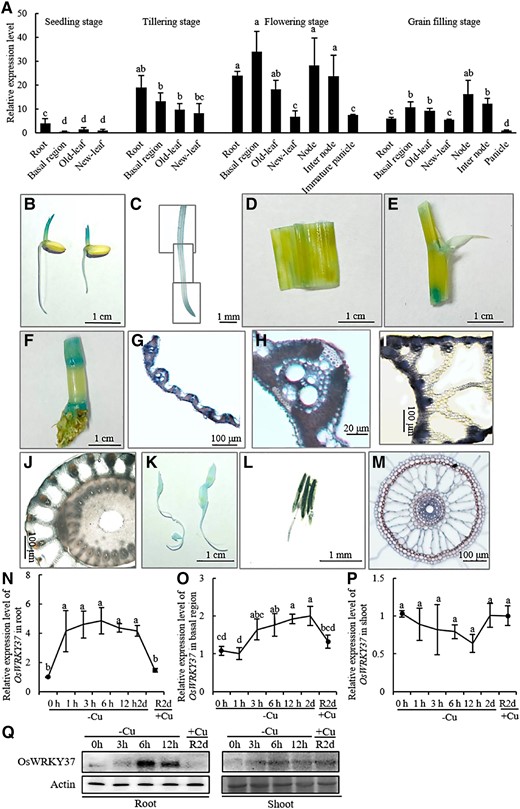
Expression pattern of OsWRKY37 in rice. A) Relative expression levels of OsWRKY37 in different tissues at different growth stages. Rice plant (ZH11) was grown in Yoshida solution until maturation, and different organs and tissues were sampled at different growth stages. The relative gene expression levels were the average means normalized using three internal standards (OsActin, OsHistone H3, and OsEFlα) and calculated using the 2−ΔΔCt method relative to the expression level of OsWRKY37 in panicle at grain filling stage. Data are means ± Sd of three biological replicates. Different letters indicate significant difference at P < 0.05 by Tukey HSD test. (B-l) Histochemical staining for GUS activity of ProWRKY37:GUS transgenic plant. Plants were grown hydroponically in Yoshida solution. B) Seeds after germination for 2 d. Primary root (C), leaf blade (D), leaf sheath (E), stem and node (F), and transverse sections of leaf blade (G), lamina joint (I), and node (J) at tillering stage. (H) The enlarged view of vascular bundle in (G). K) Immature panicles. L) Anther. Images (C), (J), and (L) are digitally extracted for comparison. Image (C) is a composite figure. M) In situ RT-PCR for the expression of OsWRKY37 in the root transverse section of 10-d-old rice seedlings (ZH11). N to Q) Time course response of OsWRKY37 to Cu deficiency and the recovery of Cu. 10-d-old seedlings of ZH11 were precultured with Yoshida solution for 1 wk, and transferred to Yoshida solution without Cu for 0, 1, 3, 6, 12 h, and 2 d, then resupplied with 0.15 μM Cu for 2 d (R2d). N to P) Relative expression levels of OsWRKY37 in root (N), basal region (O), and shoot (P). The relative gene expression levels were the average means normalized using three internal standards (OsActin, OsHistone H3, and OsEFlα) and calculated using the 2−ΔΔCt method relative to the expression level of OsWRKY37 at 0 h. Data are mean ± Sd of three biological replicates. Different letters indicate significant difference at P < 0.05 by Tukey HSD test. The statistical analyses described apply to all statistical analyses in all panels in this figure. Q) The protein levels of OsWRKY37 in root and shoot analyzed by western blot using its antibody. Actin was used as a reference protein.
To further examine the response of OsWRKY37 to Cu deficiency, we analyzed the mRNA level of OsWRKY37 in the roots, basal region, and leaves at 0, 1, 3, 6, 12 h, 2 d after Cu deficiency, as well as 2 d after Cu resupply (R2d). Results showed that the expression level of OsWRKY37 was dramatically induced by Cu deficiency within one and three hours in root and basal region, respectively, and thereafter kept stable, while it was significantly repressed by the resupply of Cu (Fig. 1, N and O). The response of OsWRKY37 to Cu deficiency was more pronounced in roots than in basal region. However, the expression level of OsWRKY37 was not significantly influenced by Cu deficiency in the shoot (Fig. 1P). Similar results were observed in western blot that OsWRKY37 protein was substantially accumulated at 6 and 12 h after Cu deficiency and dramatically decreased at 2 d after Cu resupply in roots, while OsWRKY37 protein was slightly increased after Cu deficiency, but was not changed after Cu resupply in shoots (Fig. 1Q). Results of metal-binding assay showed that the Cu/protein molar ratio of OsWRKY37 was similar to the negative control (TF), while the Cu/protein molar ratio of BSA (a positive control) was significantly higher than the negative control (TF) (Supplementary Fig. S5). These results suggested that both mRNA level and protein level of OsWRKY37 was induced by Cu deficiency, but OsWRKY37 protein could not be able to bind with Cu ion directly.
OsWRKY37 regulates Cu uptake and translocation
To study the physiological role of OsWRKY37 in rice plant, we generated OsWRKY37 overexpression lines driven by 35S promoter. Two independent overexpression lines OE-L2 and OE-L7 showed significantly higher expression levels of both mRNA and protein than wild type (Supplementary Fig. S6, A and B). No OsWRKY37 protein was detected by antibody in two CRISPR lines of OsWRKY37 (Supplementary Fig. S6B). There were no significant changes in the plant height, root length, and dry weight of OsWRKY37 overexpression lines or knockout mutants under either −Cu or +Cu condition, when compared to the wild type (Fig. 2, A and B; Supplementary Fig. S7, A to C). Overexpression of OsWRKY37 significantly increased the Cu concentration and accumulation in the root, basal region, and leaves, as well as the Cu uptake capacity of root under −Cu condition, but not under +Cu condition (Fig. 2, C to F; Supplementary Fig. S7, D and E). The Cu concentrations were increased by 41.3% to 96%, 91.3% to 108.6%, 54.5% to 67.3%, and 67.8% to 119.9% in the root, basal region, old leaves, and new leaves of the overexpression lines, respectively, and the Cu uptake capacity of root was increased by 77.9% to 87.2% under −Cu condition (Fig. 2, C and E). Although no significant changes of Cu concentration were observed in the root, basal region, old leaves, and new leaves of OsWRKY37 knockout mutants, significantly decreased root-to-shoot translocation of Cu was observed under both −Cu and +Cu conditions mainly due to the decreased ratio of shoot dry weight to root dry weight in OsWRKY37 knockout mutants compared to the wild type (Fig. 2, C, D, and G; Supplementary Fig. S7F). The root-to-shoot translocation of Cu was decreased by 2.8% to 3.4% and 8.8% to 13.1% in the knockout mutants under −Cu and +Cu conditions, respectively, when compared to the wild type (Fig. 2G). To further confirm whether OsWRKY37 is involved in Cu translocation from root to shoot, we determined the Cu concentration in xylem sap. Results showed that overexpression of OsWRKY37 significantly increased the Cu concentration in xylem sap under +Cu condition, while knockout mutation of OsWRKY37 significantly decreased the Cu concentration in xylem sap under both −Cu and +Cu conditions, when compared to the wild type (Fig. 2H). These results indicated an important function of OsWRKY37 in the uptake and root-to-shoot translocation of Cu in rice, especially under −Cu condition.
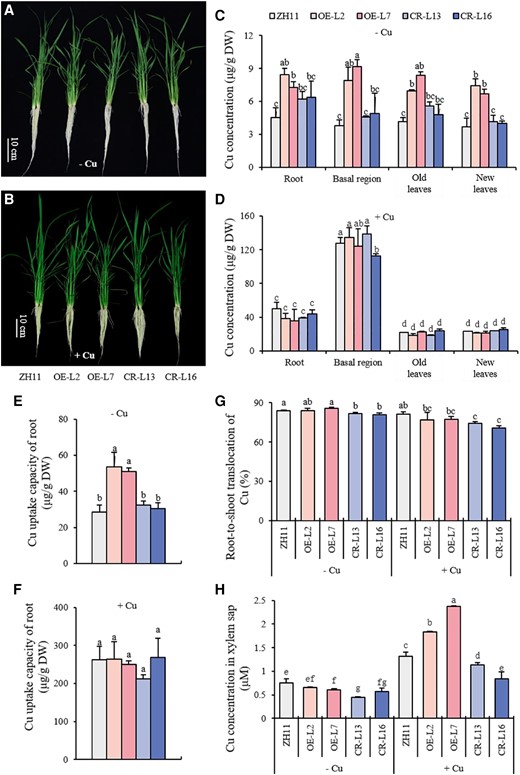
Growth phenotype and Cu status of OsWRKY37 overexpression lines and knockout mutants at tillering stage. Ten-d-old seedlings of OsWRKY37 overexpression lines (OE-L2 and OE-L7), knockout mutants (CR-L13 and CR-L16), and wild-type plant (ZH11) were grown in Yoshida solution with 0 μM Cu (−Cu) and 0.15 μM Cu (+Cu) until tillering stage. A, B) Growth phenotype. Images were digitally extracted for comparison. C, D) Cu concentration. E, F) Cu uptake capacity in root. G) Root-to-shoot translocation of Cu. H) Cu concentration in xylem sap. Data are mean ± Sd of three biological replicates. Different letters indicate significant difference at P < 0.05 by Tukey HSD test. The statistical analyses described apply to all statistical analyses in all panels in this figure.
OsWRKY37 plays an important role in flowering time and grain fertility under −Cu condition
To evaluate the function of OsWRKY37 in yield production, both overexpression lines and knockout mutants of OsWRKY37 together with the wild-type plants were grown in the Cu deficient soil with or without Cu supply until maturation. We found that the OsWRKY37 knockout mutants exhibited a late flowering phenotype under −Cu condition, but not under +Cu condition, when compared to the wild type (Fig. 3A). The flowering time of OsWRKY37 knockout mutants was 4 to 8 d later than the wild type, while the overexpression lines did not show any difference with the wild type (Fig. 3, B and C). Hence, we analyzed the expression levels of flowering related genes in panicles at different Cu levels. Results showed that none of these genes were significantly upregulated by overexpression of OsWRKY37, at the same time, was significantly downregulated by knockout mutation of OsWRKY37, although the expression levels of several flowering related genes changed a lot in OsWRKY37 overexpression lines or knockout mutants (Supplementary Fig. S8).
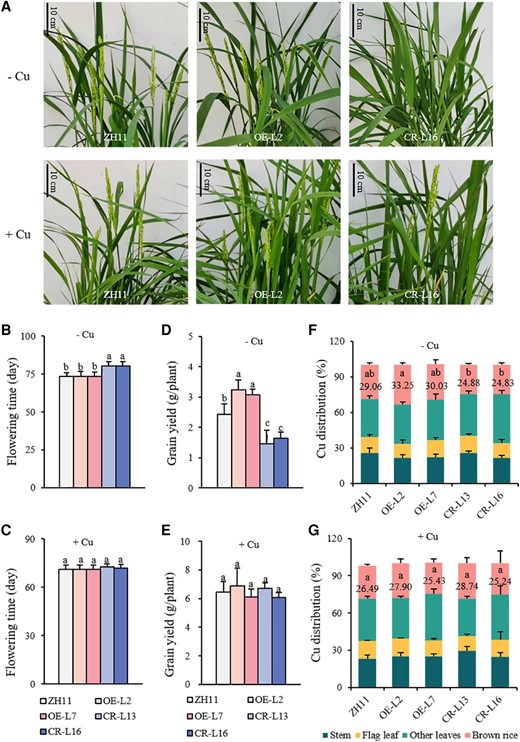
Growth phenotype and Cu status of OsWRKY37 overexpression lines and knockout mutants at reproductive stage. Twenty-d-old seedlings of OsWRKY37 overexpression lines (OE-L2 and OE-L7), knockout mutants (CR-L13 and CR-L16), and wild -ype plant (ZH11) were grown in Cu deficient soil supplied with 0 mg Cu/kg soil (−Cu) and 0.4 mg Cu/kg soil (+Cu) until maturation. A) Growth phenotype at flowering stage. B, C) Flowering time. D, E) Grain yield. F, G) Cu distribution at mature stage. Data are mean ± Sd of three biological replicates. Different letters indicate significant difference at P < 0.05 by Tukey HSD test. The statistical analyses described apply to all statistical analyses in all panels in this figure.
At mature stage, the plant height, grain yield, fertility, and 1,000-grain weight were significantly decreased in the OsWRKY37 knockout mutants under −Cu condition, but not under +Cu condition, when compared to the wild type (Fig. 3, D and E; Supplementary Table S3). The grain yield, fertility, and 1,000-grain weight were decreased by 32.23% to 39.67%, 20.83% to 34.72%, and 15.47% to 16.64%, respectively, in the knockout mutants under −Cu condition (Fig. 3D; Supplementary Table S3). While the grain yield and 1,000-grain weight were significantly increased in the OsWRKY37 overexpression lines under −Cu condition, but not under +Cu condition, when compared to the wild type (Fig. 3, D and E; Supplementary Table S3). The grain yield and 1,000-grain weight were increased by 26.45% to 33.88% and 14.88% to 31.35%, respectively, in the overexpression lines under −Cu condition (Fig. 3D; Supplementary Table S3).
The Cu concentration was significantly decreased in the stem of OsWRKY37 overexpression lines, while it was significantly increased in the brown rice under −Cu condition, which resulted in a higher Cu distribution into the brown rice (Fig. 3F; Supplementary Fig. S9A). The Cu concentration was significantly decreased in the stem and leaves of OsWRKY37 knockout lines, while no changes were observed in the brown rice under −Cu condition, which resulted in a significantly lower Cu distribution into the brown rice (Fig. 3F; Supplementary Fig. S9A). The Cu concentrations in the leaves of the overexpression lines were significantly higher than those of the wild type, while the knockout lines did not exhibit any differences to the wild type under +Cu condition (Supplementary Fig. S9B). However, there were no significant changes in the Cu distribution in either overexpression lines or knockout mutants under +Cu condition (Fig. 3G). These results implied that OsWRKY37 was of great importance in the distribution of Cu from the source (leaf) to the sink (grain), which was critical for yield formation under −Cu condition. We also analyzed the concentrations of Fe, Mn, and Zn in different organs of OsWRKY37 overexpression lines and knockout mutants. No significant differences in Fe, Mn, and Zn concentrations were observed between OsWRKY37 overexpression lines or knockout mutants and wild-type plants, except that the Mn concentration was significantly decreased in the panicles of OE-L2 and CR-L16, and the Zn concentration was significantly decreased in the new leaves of OE-L2 and CR-L13 (Supplementary Table S4).
To further test whether the reduced grain yield and fertility of the knockout lines is caused by Cu deficiency, both OsWRKY37 overexpression lines and knockout mutants together with the wild-type plants were grown in the Cu deficient soil, and sprayed with 0.1% (w/v) CuSO4 at heading stage. Results showed that the spraying of Cu significantly increased the grain yield, Cu concentration in the straw, as well as the Cu distribution in brown rice of the OsWRKY37 knockout mutants, which were increased by 78.77% to 131.70%, 14.74% to 69.23%, and 11.33% to 14.14%, respectively, while no significant differences were observed in wild type or overexpression lines after Cu spraying (Fig. 4).
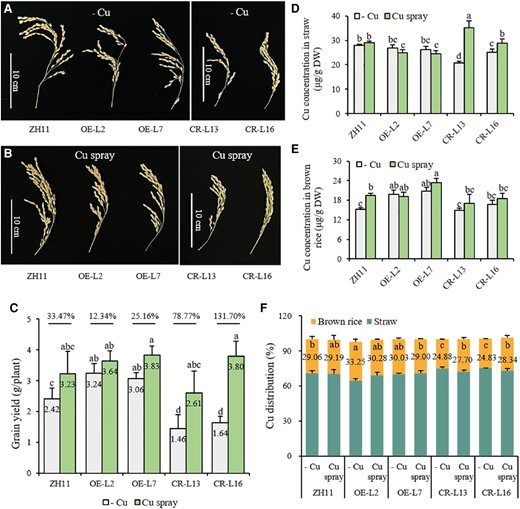
Grain yield and Cu status of OsWRKY37 overexpression lines and knockout mutants after Cu spraying. Twenty-d-old seedlings of OsWRKY37 overexpression lines (OE-L2 and OE-L7), knockout mutants (CR-L13 and CR-L16), and wild-type plant (ZH11) were grown in Cu deficient soil, and sprayed with 0.1% (w/v) CuSO4 (Cu spray) or water (−Cu) at heading stage. A, B) Spikelets. C) Grain yield. D, E) Cu concentration in straw (D) and brown rice (E). F) Cu distribution. Data are mean ± Sd of five biological replicates. Different letters indicate significant difference at P < 0.05 by Tukey HSD test. The statistical analyses described apply to all statistical analyses in all panels in this figure.
OsWRKY37 affects pollen development
Since OsWRKY37 was highly expressed in anthers (Fig. 1L) and the fertility was significantly decreased in OsWRKY37 knockout lines (Supplementary Table S3), we further analyzed the structure of pollen grains in stamens of OsWRKY37 overexpression and knockout lines grown under −Cu condition using a scanning electron microscope. Results showed that the pollen grains of wild type and OsWRKY37 overexpression lines showed baculae and tectum structure with good epidermal surface tissue, while the pollen grains of knockout mutants showed defective outer sexine with much less short baculae and tectum structure (Fig. 5A). In addition, the pollen vitality of the OsWRKY37 knockout mutants was significantly reduced, and the in vitro germination was repressed (Fig. 5, B and C). The pollen vitality and pollen germination rate were decreased by 22.29% to 28.30% and 18.90% to 24.41%, respectively, in OsWRKY37 knockout mutants when compared to the wild type (Fig. 5, D and E). Furthermore, we found that the Cu concentration in the stamen of OsWRKY37 knockout mutants was significantly lower than that of the wild type, which was decreased by 22.79% to 23.42% under −Cu condition (Fig. 5F). The Cu concentration in the stamen was decreased by 4.66% to 17.08% in OsWRKY37 knockout mutants, while it was increased by 13.95% to 23.07% in the overexpression lines under +Cu condition (Fig. 5F).
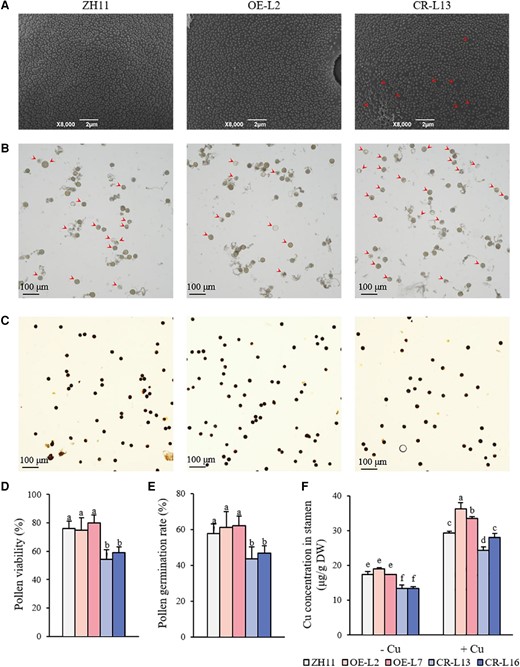
Pollen development in OsWRKY37 overexpression lines and knockout mutants. Ten-d-old seedlings of OsWRKY37 overexpression lines (OE-L2 and OE-L7), knockout mutants (CR-L13 and CR-L16), and wild-type plant (ZH11) were grown in Yoshida solution with 0 μM Cu (−Cu) and 0.15 μM Cu (+Cu) until flowering stage. Electron microscopic scanning of pollen grains (A), in vitro pollen germination (B), and pollen activity (C) of ZH11, OE-L2, and CR-L13 grown under −Cu condition. Arrowheads indicate the defective baculae and tectum structure on the pollen surface (A) and ungerminated pollens (B). Pollen viability (D) and pollen germination rate (E) of ZH11, OE-L2, OE-L2, CR-13, and CR-L16 grown under −Cu condition. F) Cu concentration in stamen. Data are mean ± Sd of three biological replicates. Different letters indicate significant difference at P < 0.05 by Tukey HSD test. The statistical analyses described apply to all statistical analyses in all panels in this figure.
Identification of target genes of OsWRKY37
WRKY transcription factors are reported to bind with the W-box in the promoter regions of downstream genes, and thereafter activate or repress their expression levels (Eulgem et al. 2000; Rushton et al. 2010). To study the possible downstream regulated genes of OsWRKY37, we analyzed the transcriptome of OsWRKY37 knockout mutants and wild-type plants grown in Cu deficient soil for 70 d. Results showed that totally 11 genes, including OsCOPT1/3/4/5/6/7, OsHMA4/5/9, OsYSL16, and OsATX1 (Antioxidant Protein1), involved in Cu transport were differentially expressed between OsWRKY37 knockout mutants and wild type (Supplementary Fig. S10A). After the promoter sequence analyzing, we found that only the promoters of OsCOPT1/2/3/6/7, OsHMA5/9, and OsYSL16 contained W-box (Supplementary Fig. S10B). Then, a yeast one-hybrid (Y1H) assay was performed to study the potential interactions between OsWRKY37 and W-box in the promoter sequences of these Cu transporter genes. Results showed that OsWRKY37 could bind to the promoter regions of OsCOPT6 and OsYSL16, but not other genes (Fig. 6A). When the W-box elements in the promoter of OsCOPT6 and OsYSL16 were mutated, no interactions were observed between OsWRKY37 and mutated promoters (Fig. 6A). Results of transient transcriptional activation experiment in Nicotiana benthamiana showed that proOsCOPT6:GUS and proOsYSL16:GUS co-expressed with 35S:OsWRKY37 significantly elevated the GUS activity in the leaves of N. benthamiana (Fig. 6, B and C). Transient transactivation experiment using dual-LUC also confirmed that 35S:OsWRKY37 co-expressed with proOsCOPT6:LUC and proOsYSL16:LUC significantly increased the LUC activity in the leaves of N. benthamiana (Fig. 6, D to F). Furthermore, the results of electrophoretic mobility shift assay (EMSA) showed OsWRKY37 could directly bind to the promoter fragment of OsCOPT6 and OsYSL16 (Fig. 6, G and H). And the results of ChIP-qPCR analyses showed that OsWRKY37 could bind to the promoter region of OsCOPT6 and OsYSL16 in vivo (Fig. 6I).
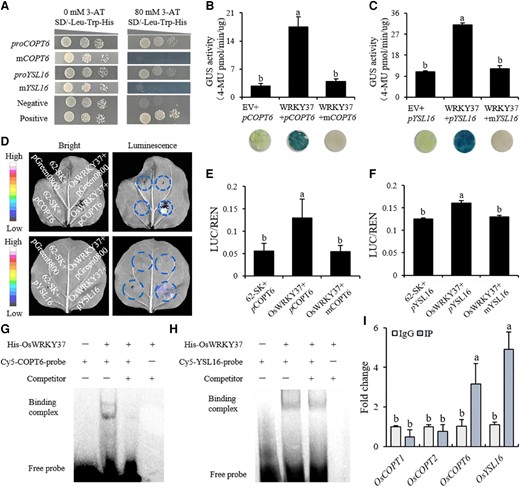
OsWRKY37 directly binds to the promoter region of OsCOPT6 and OsYSL16. A) Y1H assay for the interaction of OsWRKY37 with the promoter of OsCOPT6/OsYSL16 (proCOPT6/proYSL16). mCOPT6/mYSL16, the promoter of OsCOPT6/OsYSL16 with mutated W-box. Negative, co-transformation of pGADT7-p53 and pHIS2 into yeast as a negative control. Positive, co-transformation of pGADT7-p53 and pHIS2-p53 into yeast as a positive control. B, C) GUS staining and GUS activities co-expressing 35S:OsWRKY37 with proOsCOPT6: GUS (B) and proOsYSL16: GUS (C) in the leaves of N. benthamiana. EV, empty vector. D to F) Fluorescence imaging (D) and LUC activities co-expressing 35S:OsWRKY37 with proOsCOPT6: LUC (E) or proOsYSL16: LUC (F) in the leaves of N. benthamiana. 62-SK, empty vector. G, H) EMSA for the interaction of OsWRKY37 with OsCOPT6 (G) or OsYSL16 promoter (H). I) ChIP-qPCR analysis of OsWRKY37 binding to the promoter regions of OsCOPT1/2/6 and OsYSL16. OsCOPT1 and OsCOPT2 were used as negative controls. Data are mean ± Sd of six biological replicates. Different letters indicate significant difference at P < 0.05 by Tukey HSD test. The statistical analyses described apply to all statistical analyses in all panels in this figure.
To further confirm OsWRKY37 could regulate the expression of OsCOPT6 and OsYSL16 gene in rice plant, both in situ RT-PCR and RT-qPCR were performed in wild type, OsWRKY37 overexpression lines and knockout mutants. Results showed that the expression levels of OsCOPT6 and OsYSL16 were closely associated with the OsWRKY37 expression level (Fig. 7). OsCOPT6 was mainly expressed in root, while OsYSL16 was mainly expression in leaves. The expression levels of both OsCOPT6 and OsYSL16 were upregulated in OsWRKY37 overexpression lines, and downregulated in knockout mutants (Fig. 7). Taken together, these results suggested that OsWRKY37 could directly bind to the promoter regions of OsCOPT6 and OsYSL16, and activated their expression levels in rice plant.
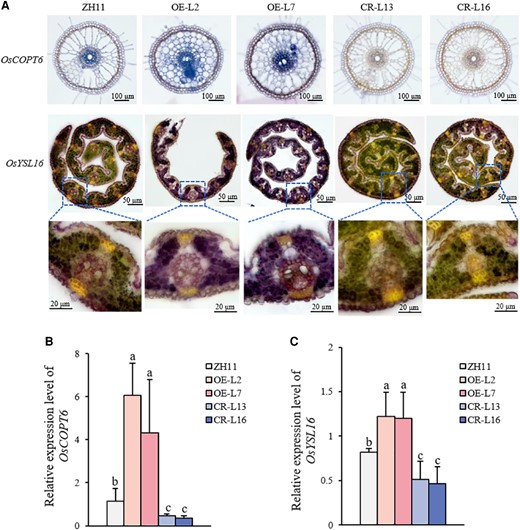
OsWRKY37 positively regulates the expression levels of OsCOPT6 and OsYSL16. A) In situ RT-PCR analyses for the expression of OsCOPT6 and OsYSL16 in OsWRKY37 overexpression lines (OE-L2 and OE-L7), knockout mutants (CR-L13 and CR-L16), and wild-type plant (ZH11). Staining indicates the presence of transcripts in roots and leaves, respectively. Ten-d-old seedlings were grown under −Cu condition for 1 wk. The transverse sections of roots and leaves were used for in situ RT-PCR. Bar = 100 μm. B, C) Relative expression levels of OsCOPT6 (B) and OsYSL16 (C) in OsWRKY37 overexpression lines (OE-L2 and OE-L7), knockout mutants (CR-L13 and CR-L16), and wild-type plant (ZH11). Ten-d-old seedlings were grown under −Cu (0 μM Cu) condition for 5 wk. The relative gene expression levels were normalized using an internal standard (OsActin) and calculated using the 2−ΔΔCt method relative to the expression level in ZH11. Data are mean ± Sd of three biological replicates. Different letters indicate significant difference at P < 0.05 by Tukey HSD test. The statistical analyses described apply to all statistical analyses in all panels in this figure.
OsCOPT6 is involved in Cu uptake in rice
Previous study has reported that OsCOPT6 is a Cu deficiency-induced gene in rice plant, and its encoded protein has Cu transport activity in yeast cells (Yuan et al. 2011). However, the exact role of OsCOPT6 in Cu uptake and transport in rice plant is still unclear. Hence, we analyzed the subcellular localization of OsCOPT6 protein through transient expressing of 35S:OsCOPT6-GFP in the leaves of N. benthamiana. Results showed that green fluorescence signal of OsCOPT6 was completely overlapped with the red fluorescence signal of the membrane-localized protein AtPIP2A (plasma membrane intrinsic protein 2A) (Supplementary Fig. S11). After plasmolysis with 30% (w/v) sucrose, the green fluorescence signal of OsCOPT6 was separated with cell wall, which confirmed that OsCOPT6 was localized to the plasma membrane (Supplementary Fig. S11).
To verify the function of OsCOPT6 in Cu uptake and transport in rice plant, we created the knockout mutants using CRISPR/Cas9-mediated targeted mutagenesis technique. There were 13 bp replacement and 8 bp deletion in oscopt6–7, 7 bp replacement at the first target and an entire deletion at the second target in oscopt6–20, 1 bp insertion at the first target and an entire deletion at the second target in oscopt6–25 (Supplementary Fig. S12). No obvious changes of the root length, plant height, root and shoot dry weight were observed in the knockout mutants of OsCOPT6 under both −Cu and +Cu conditions when compared to the wild type (Supplementary Fig. S13). The Cu concentration in root, basal region, old leaves, and new leaves, and the Cu accumulation in leaves, as well as the Cu uptake capacity of root were significantly decreased in the knockout mutants of OsCOPT6 under −Cu condition, but not under +Cu condition (Supplementary Figs. S14 and S15A, B). However, the root-to-shoot translocation and distribution of Cu did not change obviously in the knockout mutants of OsCOPT6 under both −Cu and +Cu conditions when compared to the wild type (Supplementary Fig. S15, C to F). These results suggested that OsCOPT6 was mainly involved in the Cu uptake in rice plant. Moreover, a delayed flowering time was also observed in the knockout mutants of OsCOPT6 grown under Cu deficient soil (Supplementary Fig. S16).
Discussion
OsWRKY37 acts as a positive regulator for grain yield formation under Cu deficient condition
Promoting sustainable agricultural development and solving food security issues is one of the important challenges in the 21st century (FAO. 2021). The availability of Cu in soils in some regions is one of the factors limiting grain yield, and Cu deficiency can lead to plant sterility and reduced grain yield (Solberg et al. 1999; Burkhead et al. 2009). Therefore, it is necessary to improve the Cu utilization efficiency of crops to achieve increased grain production. Plants respond to Cu deficiency by activating membrane protein systems for uptake and transport, in which transcription factors act as the core regulators (Garcia-Molina et al. 2011, 2013; Andrés-Colás et al. 2018; Sheng et al. 2021). Cu deficiency-induced transcription factors AtSPL7 and AtCITF1 play critical roles in maintaining Cu homeostasis in Arabidopsis (Yamasaki et al. 2009; Perea-Garcia et al. 2013; Yan et al. 2017). The homologues of AtSPL7 in rice, OsSPL9, only sharing 37.1% identity with AtSPL7, can promote Cu accumulation in shoots and grains (Tang et al. 2016). How rice responds to Cu deficiency, and which transcriptional network coordinates Cu uptake, translocation, and distribution to reproductive organs is still not completely known. In this study, we have identified the transcription factor OsWRKY37 in rice, which is in response to Cu deficiency. Our results showed that overexpression of OsWRKY37 significantly increased the Cu concentration in rice plant as well as Cu uptake capacity in root under −Cu condition (Fig. 2, C and E). While knockout mutation of OsWRKY37 significantly inhibited the root-to-shoot translocation of Cu and the distribution to brown rice under −Cu condition (Figs. 2G and 3F). Moreover, knockout mutation of OsWRKY37 significantly affected the fertility and grain yield production in rice under −Cu condition (Fig. 3D; Supplementary Table S3). Our results demonstrate that OsWRKY37 possesses a significant role in the positive regulation of Cu uptake, root-to-shoot translocation, and distribution to reproductive organs, thereafter promotes grain yield formation under Cu deficient condition.
Interestingly, we have also observed that the knockout mutation of OsWRKY37 delayed the flowering time and affected the pollen development with the defects of baculae and tectum structure in the pollen epidermis, decreased pollen activity and in vitro germination rate (Fig. 5). As we known, flowering is the transition point from the vegetative stage to reproductive stage in plants. Finely coordinating and optimizing the balance of these two stages is critical to maximize crop grain yield (Simpson and Dean 2002; Sun et al. 2014). It has been reported that Cu deficiency not only represses plant growth but also delays flowering (Ishka and Vatamaniuk 2020; Sheng et al. 2021). As stamen has a high demand for Cu, insufficient Cu supply reduces the number of pollen and germination rate (Yan et al. 2017; Zhang et al. 2018). Therefore, anther and pollen development in floral organs depends on Cu homeostasis, and the reduced Cu distribution to floral organs may cause sterility (Zhang et al. 2018; Sheng et al. 2021). Our results showed that the knockout mutation of OsWRKY37 significantly reduced the Cu concentration in stamen, especially under −Cu condition (Fig. 5F), which may lead to the late flowering and the breakdown of pollen development. Furthermore, results of the expression analyses of flowering genes in panicles showed that no substantial changes were observed in OsWRKY37 overexpression lines or knockout mutants under either +Cu or −Cu condition (Supplementary Fig. S8), which confirms that the late flowering in OsWRKY37 knockout mutants is not caused by the reduced expression levels of flowering genes.
OsWRKY37 promotes Cu uptake through upregulating the expression of OsCOPT6
COPT is a transporter family with Cu transport ability (Sancenón et al. 2003; Yuan et al. 2011). In Arabidopsis, COPT differentiates into different localizations and functions, playing important roles in Cu uptake, transport, and remobilization (Sancenón et al. 2003, 2004; García-Molina et al. 2011, 2013; Perea-Garcia et al. 2013; Andrés-Colás et al. 2018). OsCOPT6 has been reported to be localized in the yeast plasma membrane, and has a high activity of transporting Cu in the Cu transport-deficient yeast strain (Yuan et al. 2011). OsCOPT6 is induced by Cu deficiency in rice, and may act as a cofactor to help OsCOPT2, OsCOPT3, or OsCOPT4 to localize in the plasma membrane, thereby mediating Cu transport (Yuan et al. 2011). Here, we confirmed the plasma membrane localization of OsCOPT6 by transient expression in the leaves of N. benthamiana (Supplementary Fig. S11). Moreover, in situ RT-PCR clearly showed that OsCOPT6 was highly expressed in the root of rice (Fig. 7). And significantly lower Cu concentration, accumulation, and root uptake capacity were observed in the OsCOPT6 knockout mutants than wild type under −Cu condition (Supplementary Figs. S14 and S15A, B). Therefore, OsCOPT6 is a plasma membrane localized transporter of Cu in the root, which plays an important role in Cu uptake in rice under −Cu condition.
Previously, the transcription factor AtSPL7 has been reported to mediate Cu uptake by positively regulating the expression levels of Cu transporters and FRO4/5 (Yamasaki et al. 2009; Bernal et al. 2012). In addition, the transcription factor AtTCP16 can directly bind with the promoter region of AtCOPT3 and inhibits its expression level to alter Cu content (Andrés-Colás et al. 2018). In our study, results of Y1H, transient expression assay, EMSA, and ChIP-qPCR analyses showed that OsWRKY37 could bind to the W-box in the promoter of OsCOPT6 and positively regulates its expression level (Fig. 6). Results of in situ RT-PCR and RT-qPCR clearly showed that the expression level of OsCOPT6 was upregulated in the root of OsWRKY37 overexpression lines, while downregulated in the root of OsWRKY37 knockout mutants (Fig. 7, A and B). Together with the increased Cu concentration and root uptake capacity of Cu in the OsWRKY37 overexpression lines, we demonstrated that transcription factor OsWRKY37 could promote Cu uptake in rice through upregulating the expression level of OsCOPT6 under −Cu condition (Fig. 8).
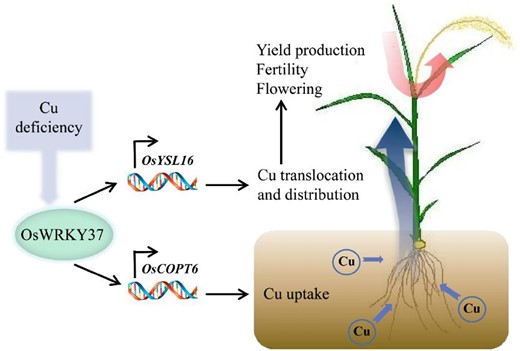
Working model of OsWRKY37 in rice plant. Transcription factor OsWRKY37 could directly bind to the promoter region of OsCOPT6 and OsYSL16, and activated their expression levels, thereby facilitated the uptake and root-to-shoot translocation of Cu, and distributed more Cu into stamen and grains, which was important for the pollen development, flowering, fertility, and grain yield in rice under Cu deficient condition.
OsWRKY37 facilitates Cu distribution through upregulating the expression of OsYSL16
Previous studies have shown that the YSL protein family can transport heavy metal–nicotianamine (HM–NA) complexes and is of great importance in the long-distance transport of metals in plants (Curie et al. 2001; 2009). In Arabidopsis, AtYSL1, AtYSL2, and AtYSL3 can transport Cu–NA and function in the distribution of Cu (DiDonato et al. 2004; Waters et al. 2006; Waters and Grusak 2008). OsYSL16 is mainly expressed in the node, leaf vascular bundle, rachilla, palea, lemma and stamen, and is involved in the distribution and redistribution of Cu in rice plant (Koike et al. 2004; Zheng et al. 2012; Zhang et al. 2018). Similar expression pattern of OsWRKY37 was found in our study, which was also highly expressed in the node, leaf vascular bundle, rachilla, and stamens of rice (Fig. 1). Y1H, EMSA, ChIP-qPCR, and transient expression assay in leaves of N. benthamiana showed that OsWRKY37 could bind to the W-box in the promoter of OsYSL16 and positively regulates its expression level (Fig. 6). Results of in situ RT-PCR and RT-qPCR clearly showed that the expression level of OsYSL16 was upregulated in OsWRKY37 overexpression lines, while downregulated in OsWRKY37 knockout mutants (Fig. 7, A and C). Therefore, in addition to OsCOPT6, OsYSL16 is another downstream regulated gene of OsWRKY37. In addition, our results showed that the knockout mutation of OsWRKY37 significantly inhibited the Cu distribution to reproductive organs, thereafter severely affected the flowering time and pollen development, decreased grain fertility and grain yield production under −Cu condition (Figs. 3 and 5; Supplementary Table S3). Similar results have been reported that knockout of YSL16 in rachilla results in a decrease in Cu content in stamen leading to decreased pollen fertility (Zhang et al. 2018). Knockdown of BdYSL3 results in delayed flowering, altered inflorescence structure, reduced fertility, smaller grain, and decreased protein accumulation (Sheng et al. 2021). Taken together, transcription factor OsWRKY37 plays an important role in the Cu distribution to reproductive organs for the grain yield production in rice by acting as a positive upstream regulator of OsYSL16 under −Cu condition (Fig. 8).
Materials and methods
Generation of transgenic lines
Knockout mutants of OsWRKYs and OsCOPT6 were created in rice (O. Sativa) by CRISPR/Cas9-mediated targeted mutagenesis technique on the background of Zhonghua 11 (Xie et al. 2015). The full-length genomic DNA of OsWRKY37 was amplified with specific primers and inserted into p1301S vector to generate overexpression plants. ProWRKY37:GUS transgenic plants were generated to show the expression pattern of OsWRKY37 in rice plant. More details are described in Supplementary methods.
Subcellular localization
The coding sequence of OsWRKY37 was amplified and cloned into pM999-GFP vector fusing with GFP to create 35S:OsWRKY37-GFP construct, which was transformed into rice protoplasts. The coding sequence of OsCOPT6 was amplified and cloned into p35s-XGFP vector fusing with GFP to create 35S:OsCOPT6-GFP construct, which was transformed into leaves of N. benthamiana. Primers are listed in Supplementary Table S5. More details are described in Supplementary methods.
Transactivation assay in yeast (S. cerevisiae)
To analyze whether OsWRKY37 has the transactivation activity, the full-length cDNA of OsWRKY37 was amplified and inserted into pGBKT7 vector fused with GAL4 DNA binding domain, and transformed into yeast strain AH109. Primers are listed in Supplementary Table S5. More details are described in Supplementary methods.
Hydroponic culture
To investigate the effects of OsWRKY37 and OsCOPT6 on rice growth and Cu transport, seeds of wild type, OsWRKY37 overexpression lines, OsWRKY37 and OsCOPT6 knockout mutants were cultured in Yoshida solution (Yoshida et al. 1976) with (+Cu, 0.15 μM Cu) or without Cu (−Cu, 0 μM Cu). To analyze the expression pattern of OsWRKY37 in rice plant, wild-type plant was grown in Yoshida solution until maturation. To analyze Cu-responsive expression pattern of OsWRKY37, 10-d-old seedlings of wild type were precultured with normal Yoshida solution for 1 wk, and transferred to Yoshida solution without Cu. More details are described in Supplementary methods.
Pot experiment
To investigate the effects of OsWRKY37 on grain yield production and Cu distribution at mature stage, 20-d-old seedlings of wild type, OsWRKY37 overexpression lines, and knockout mutants were planted in the soil fertilized with 0.2 g N/kg soil, 0.1 g P/kg soil, 0.15 g K/kg soil, together with 0 mg Cu/kg soil as −Cu treatment and 0.4 mg Cu/kg soil as +Cu treatment. The basic chemical properties of soil are shown in Supplementary Table S6. More details are described in Supplementary methods.
Determination of trace metals in plant samples
Plant samples harvested from the hydroponic culture and pot experiment were dried at 70 °C for at least three days and digested with 2 mL of mixed acid HNO3/HClO4 (4:1, v/v) at 150 °C overnight. The digested solution was diluted with ddH2O and used to determine the concentrations of Cu, Fe, Mn, and Zn by an atomic absorption spectrophotometer (AGILENT-WFX-ID, Palo Alto, USA). The copper standard solution (GSB 04–1725–2004) was purchased from Guobiao Testing & Certification Co., Ltd (Beijing, China). The calculation methods of root uptake capacity, root-to-shoot translocation, and distribution of Cu were described in Supplementary methods.
RNA extraction and RT-qPCR
To analyze the expression levels of OsWRKYs, OsCOPT6, OsYSL16, and flowering related genes in plant samples, RT-qPCR was performed on a Quant Studio 6 Flex System (Applied Biosystems, Foster City, CA, USA) using Hieff qPCR SYBR Green Master Mix (Low Rox Plus) (YEASEN, Shanghai, China). The relative gene expression levels were normalized using internal standards (OsActin, OsHistone H3, and OsEFlα) and calculated using the 2−ΔΔCt method by CFX Manager software (Bio-Rad, Hercules, USA). Primers are listed in Supplementary Table S5. More details are described in Supplementary methods.
Protein extraction and western blot
To analyze the protein expression level of OsWRKY37 in response to Cu deficiency and resupply of Cu, as well as its protein level in overexpression lines and knockout mutants, western blot was performed according to the previously reported methods (Yao et al. 2022). More details are described in Supplementary methods.
Binding assay of Cu and OsWRKY37
To investigate whether OsWRKY37 can bind with Cu, a metal-binding assay was performed according to the previously reported methods (Luo et al. 2018). More details are described in Supplementary methods.
Cu determination in xylem sap
To investigate the effects of OsWRKY37 and OsCOPT6 on the root-to-shoot translocation of Cu in rice, 10-d-old seedlings of wild type, OsWRKY37 overexpression lines, OsWRKY37 and OsCOPT6 knockout mutants were transferred to Yoshida solution containing 0 μM Cu (−Cu) or 0.15 μM Cu (+Cu). After 30 d, the Cu concentration in xylem sap was determined according to the method described by Deng et al. (2013). More details are described in Supplementary methods.
Pollen structure and activity analyses
To investigate the effect of OsWRKY37 on the pollen structure and activity, the spikelets of wild type, OsWRKY37 overexpression lines, and knockout mutants grown under −Cu (0 μM Cu) condition were collected at the beginning of flowering stage. The structure of pollen grains was observed using a scanning electron microscope (JEOL JSM-6390/LV, Tokyo, Japan). The pollen grains were stained with 1% (w/v) I2-KI to analyze the pollen viability. Mature pollen grains were germinated on a pollen germination medium to analyze the pollen germination rate. More details are described in Supplementary methods.
Cu concentration in stamen
Seedling of wild type, OsWRKY37 overexpression lines, and knockout mutants were grown under −Cu (0 μM Cu) and +Cu (0.15 μM Cu) conditions hydroponically until flowering stage. Stamen from young spikelets of six plants in each line were collected into an Eppendorf tube using the tweezers, which lasted for 1 wk. About 0.02 g fresh sample of stamen was used for the determination of Cu concentration by an atomic absorption spectrophotometer (AGILENT-WFX-ID, Palo Alto, USA).
Transcriptome analyses
To identify the potential regulatory factors in response to Cu deficiency in rice, 10-d-old seedlings of ZH11 were grown hydroponically under −Cu (0 μM Cu) and +Cu (0.15 μM Cu) conditions for 2 wk, and the roots were harvested for transcriptome analyses. Additionally, to analyze the potential downstream genes regulated by OsWRKY37, 70-d-old plants of wild type and OsWRKY37 knockout mutant (CR-L16) grown in Cu deficient soil were sampled for transcriptome sequencing. More details are described in Supplementary methods. The raw data have been submitted in the RSA database (https://www.ncbi.nlm.nih.gov/sra/), and the accession number are PRJNA1061079 and PRJNA1063597.
Interactions between OsWRKY37 and promoters of Cu transport genes
To study the potential interactions between OsWRKY37 and the promoter sequences of candidate Cu transport genes, Y1H assay was carried out. To further confirm the interaction between OsWRKY37 and the promoter sequences of OsCOPT6 and OsYSL16, transient co-expression assay was carried out in the leaves of N. benthamiana. To further study whether OsWRKY37 can directly bind to the W-box in the promoter of OsCOPT6 and OsYSL16, electrophoretic mobility assay was carried out. Primers and probe sequences are listed in Supplementary Table S5. More details are described in Supplementary methods.
In situ RT-PCR
To analyze whether the expression levels of OsCOPT6 and OsYSL16 were directly regulated by OsWRKY37, in situ RT-PCR was performed in the root and shoot of wild type, OsWRKY37 overexpression lines, and knockout mutants. 10-d-old seedlings of wild type, OsWRKY37 overexpression lines, and knockout mutants were grown under −Cu (0 μM Cu) condition for one week. In situ RT-PCR was performed according to the method of Athman et al. (2014). More details are described in Supplementary methods.
ChIP-qPCR analyses
Fifteen-d-old seedlings of 35S:OsWRKY37 transgenic plants were used for ChIP assay. The chromatin DNA was sonicated and incubated with OsWRKY37 antibody (PhytoAB, California, USA) and IgG antibody to isolate protein–DNA complexes. After recovering and purification, the immunoprecipitated DNA was used as the template for qPCR, and the relative fold enrichment was calculated. IgG antibody was used as a negative control. The primers are listed in Supplementary Table S5. More details are described in Supplementary methods.
Statistical analyses
To determine the significant differences, SPSS (Statistical Package for the Social Sciences) was used for statistical analyses. The significances were assessed by one-way and two-way ANOVA (Analysis of Variance). Tukey's HSD (Honestly Significant Difference) was used to test multiple pairwise comparisons. Data were presented as means ± Sd with at least three biological replications. Different letters indicated significant difference at P < 0.05.
Accession numbers
Sequence data from this article can be found in the data library of Rice Genome Annotation Project (http://rice.uga.edu) under accession numbers LOC_Os04g50920 (OsWRKY37), LOC_Os08g35490 (OsCOPT6), and LOC_Os04g45900 (OsYSL16).
Acknowledgments
We thank Prof. Lizhong Xiong (Huazhong Agricultural University) for kindly providing the plasmid of nucleus localized marker 35S:AtFIB2-mcherry, and Prof. Kabin Xie (Huazhong Agricultural University) for kindly providing the CRISPR/Cas9 binary vector system pRGEB32.
Author contributions
C.J. and H.C. designed the experiment; C.J., H.L., J.D., and L.Y. performed the experiments; C.J. analyzed the data; C.J., S.W., C.W., G.D., L.S. and F.X. provided important guidance and critical comments; C.J. wrote the manuscript; H.C. revised the manuscript.
Supplementary data
The following materials are available in the online version of this article.
Supplementary Table S1. Differentially expressed genes in rice root under Cu deficiency.
Supplementary Table S2. FPKM of eight WRKY genes induced by Cu deficiency in rice root.
Supplementary Table S3. Agronomic traits of OsWRKY37 overexpression lines and knockout mutants grown in the soil under −Cu and +Cu conditions.
Supplementary Table S4. Concentrations of Fe, Mn, and Zn in different organs of OsWRKY37 overexpression lines and knockout mutants grown hydroponically under −Cu condition at flowering stage.
Supplementary Table S5. Primers used in this study.
Supplementary Table S6. Basic chemical properties of soil used in the pot experiment.
Supplementary Figure S1. Relative expression levels of eight WRKY genes in rice root and shoot under −Cu and +Cu conditions.
Supplementary Figure S2. Molecular identification of eight WRKY knockout mutants.
Supplementary Figure S3. Growth phenotype and heading time of eight WRKY knockout mutants grown in field at flowering stage.
Supplementary Figure S4. Subcellular localization and transactivation activity of OsWRKY37 protein.
Supplementary Figure S5. Binding assay of Cu and OsWRKY37 protein.
Supplementary Figure S6. Molecular identification of OsWRKY37 overexpressed lines and knockout mutants.
Supplementary Figure S7. Growth parameters and Cu accumulation of OsWRKY37 overexpression lines and knockout mutants at tillering stage.
Supplementary Figure S8. Heat map of relative expression levels of flowering related genes in the panicles of OsWRKY37 overexpression lines and knockout mutants.
Supplementary Figure S9. Cu concentrations in different organs of OsWRKY37 overexpression lines and knockout mutants at mature stage.
Supplementary Figure S10. Differentially expressed Cu transport genes in OsWRKY37 knockout mutants and the W-box in the promoters of Cu transport genes.
Supplementary Figure S11. Subcellular localization of OsCOPT6 protein.
Supplementary Figure S12. Molecular identification of OsCOPT6 knockout mutants.
Supplementary Figure S13. Growth phenotype of OsCOPT6 knockout mutants at tillering stage.
Supplementary Figure S14. The concentration and accumulation of Cu in OsCOPT6 knockout mutants at tillering stage.
Supplementary Figure S15. The uptake, root-to-shoot translocation, and distribution of Cu in OsCOPT6 knockout mutants at tillering stage.
Supplementary Figure S16. Growth phenotype of OsCOPT6 knockout mutants at flowering stage.
Funding
This work was supported by grants from the National Key Research and Development Program of China (2021YFD1901203).
Data availability
The data that support the findings of this study are available from the corresponding author upon reasonable request.
Dive Curated Terms
The following phenotypic, genotypic, and functional terms are of significance to the work described in this paper:
References
Author notes
The author responsible for distribution of materials integral to the findings presented in this article in accordance with the policy described in the Instructions for Authors (https://dbpia.nl.go.kr/plphys/pages/General-Instructions) is Hongmei Cai.
Conflict of interest statement. None declared.