-
PDF
- Split View
-
Views
-
Cite
Cite
Xiuzhen Kong, Yali Xiong, Xiaoyun Song, Samuel Wadey, Suhang Yu, Jinliang Rao, Aneesh Lale, Marco Lombardi, Riccardo Fusi, Rahul Bhosale, Guoqiang Huang, Ethylene regulates auxin-mediated root gravitropic machinery and controls root angle in cereal crops, Plant Physiology, Volume 195, Issue 3, July 2024, Pages 1969–1980, https://doi.org/10.1093/plphys/kiae134
- Share Icon Share
Abstract
Root angle is a critical factor in optimizing the acquisition of essential resources from different soil depths. The regulation of root angle relies on the auxin-mediated root gravitropism machinery. While the influence of ethylene on auxin levels is known, its specific role in governing root gravitropism and angle remains uncertain, particularly when Arabidopsis (Arabidopsis thaliana) core ethylene signaling mutants show no gravitropic defects. Our research, focusing on rice (Oryza sativa L.) and maize (Zea mays), clearly reveals the involvement of ethylene in root angle regulation in cereal crops through the modulation of auxin biosynthesis and the root gravitropism machinery. We elucidated the molecular components by which ethylene exerts its regulatory effect on auxin biosynthesis to control root gravitropism machinery. The ethylene-insensitive mutants ethylene insensitive2 (osein2) and ethylene insensitive like1 (oseil1), exhibited substantially shallower crown root angle compared to the wild type. Gravitropism assays revealed reduced root gravitropic response in these mutants. Hormone profiling analysis confirmed decreased auxin levels in the root tips of the osein2 mutant, and exogenous auxin (NAA) application rescued root gravitropism in both ethylene-insensitive mutants. Additionally, the auxin biosynthetic mutant mao hu zi10 (mhz10)/tryptophan aminotransferase2 (ostar2) showed impaired gravitropic response and shallow crown root angle phenotypes. Similarly, maize ethylene-insensitive mutants (zmein2) exhibited defective gravitropism and root angle phenotypes. In conclusion, our study highlights that ethylene controls the auxin-dependent root gravitropism machinery to regulate root angle in rice and maize, revealing a functional divergence in ethylene signaling between Arabidopsis and cereal crops. These findings contribute to a better understanding of root angle regulation and have implications for improving resource acquisition in agricultural systems.
Introduction
Root angle, also known as gravitropic setpoint angle (GSA), refers to the angle at which a root emerges relative to the gravity vector while growing away from the vertical primary root (PR; Roychoudhry et al. 2013). This is a crucial root architectural trait that plays a key role in enabling plants to adapt to various soil environments, such as phosphate deprivation, water scarcity, and salinity (Osmont et al. 2007; Rogers and Benfey 2015). A steep root angle can enhance drought tolerance by promoting water uptake from deep soils (Uga et al. 2013), whereas a shallow root angle can increase phosphate uptake from topsoils (Liao et al. 2001; Péret et al. 2014; Huang et al. 2018) and mitigate yield loss by staying away from salt accumulated in deep soils (Kitomi et al. 2020). Thus, a better understanding of the underlying mechanisms that control root angle can pave the way for breeding crop cultivars better adapted to abiotic stressors associated with global climate change.
To date, a multitude of genes accountable for regulating root angle have been identified in cereal crops. In rice, Rice Morphology Determinant (RMD), a type II formin (OsFH5), localizes on the surface of statoliths in root cap cells and regulates crown root angle by dampening statolith sedimentation in gravity-sensing process (Zhang et al. 2011; Huang et al. 2018); DEEPER ROOTING1 (DRO1), a plasma membrane-localized protein, appears not to affect early auxin response genes of gravitropic signaling in root meristem zone (Uga et al. 2013); AUXIN RESISTANT 1 (OsAUX1), an auxin influx carrier, affects root angle by influencing basal auxin transport in gravitropic process (Bennett et al. 1996; Giri et al. 2018); soil-surface rooting 1 (SOR1)/MHZ2 is a RING finger E3 ubiquitin ligase, and its knockout mutants show reduced gravitropic response and soil-surface rooting phenotype (Hanzawa et al. 2013; Chen et al. 2018). In barley (Hordeum vulgare) and wheat (Triticum aestivum), ENHANCED GRAVITROPISM1 (EGT1), an F-box and Tubby domain-containing protein, appears to control root angle by regulating cell wall stiffness (Fusi et al. 2022); EGT2, encoding a STERILE ALPHA MOTIF (SAM) domain-containing protein, is likely involved in gravitropic response by counteracting the auxin-mediated positive gravitropic signaling pathway (Kirschner et al. 2021). In maize, CBL-interacting serine/threonine-protein kinase 15 (ZmCIPK15) modulates root angle in response to external nitrogen (Schneider et al. 2022); Maize Root System Architecture3.1 (ZmRSA3.1), a member of the AUX/IAA protein family, and ZmRSA3.2, an ortholog of Arabidopsis Formin Homology (AtFH1), were found to contribute to the regulation of root angle and root depth (Ren et al. 2022). Overall, some of these genes are suggested to alter auxin transport or signaling to modulate root gravitropic response machinery, thus controlling root angle. However, in contrast to the well-established understanding of the role of auxin in model plant Arabidopsis, the involvement of other hormones and their interplay with auxin in regulating gravitropism in crop species remain inadequately understood, thereby impeding the advancement of dissecting the underlying mechanisms governing root angle in crops.
Ethylene, a gaseous hormone, plays crucial roles in plant growth and stress responses. Through studies conducted in the dicot model plant species Arabidopsis, a linear ethylene signaling pathway has been established. In order to trigger the ethylene response, ethylene needs to be perceived by 5 ethylene receptors, namely ETHYLENE RESPONSE 1 (ETR1), ETHYLENE RESPONSE SENSOR 1 (ERS1), ETR2, ERS2, and EIN4 (Ju and Chang 2015). Subsequently, the signal is transduced by CONSTITUTIVE TRIPLE RESPONSE 1 (CTR1) and EIN2 to activate transcriptional reprogramming (Zhao et al. 2021). Afterward, EIN3/EIL1 family of transcription factors receives signals from upstream sources and proceeds to activate downstream genes in response to external cues (Dolgikh et al. 2019). In rice, several regulators have been found, despite the primary ethylene signaling components being conserved compared to Arabidopsis. One such regulator is MHZ1, a rice histidine kinase (OsHK1) that physically interacts with the GAF domain of OsERS2 and in conjugation with the OsEIN2-mediated pathway to regulate ethylene-regulated root growth (Zhao et al. 2020a). Another regulator, MHZ3, is an uncharacterized membrane protein localized to the endoplasmic reticulum (ER) and interacts with the Nramp-like domain of OsEIN2, which is required for the proper accumulation of OsEIN2 protein (Ma 2018). The protein MHZ9, consisting of a glycine–tyrosine–phenylalanine domain protein, is localized in RNA processing bodies (Huang et al. 2023). The C-terminal domain of MHZ9 interacts with OsEIN2, and the N-terminal domain directly binds to the EIN3 BINDING F-BOX 1/2 (OsEBF1/2) mRNAs to inhibit translation. This inhibition allows for the accumulation of the transcription factor OsEIL1, which then activates the downstream signaling (Huang et al. 2023). MHZ11 is localized to the membrane of ER and harbors acyl-hydrolyzing activity (Zhao et al. 2020b). MHZ11 may decrease sterol levels, thereby interfering with receptor–OsCTR2 interactions and OsCTR2 phosphorylation for triggering ethylene signaling (Zhao et al. 2020b). These discoveries are now enabling us to explore the involvement of ethylene in regulating various biological processes under both developmental and environmental contexts.
The phytohormones ethylene and auxin are key regulators of plant growth and development, and their intricate interplay has been extensively studied (Muday et al. 2012). In Arabidopsis and rice, ethylene has been found to act upstream of auxin biosynthesis, transport, and response pathways (Stepanova et al. 2005; Růžička et al. 2007; Stepanova et al. 2007; Swarup et al. 2007). For example, ETHYLENE RESPONSE FACTOR1 (AtERF1) directly activates the expression of WEAK ETHYLENE INSENSITIVE2/ANTHRA NILATE SYNTHASE α1 (AtWEI2/AtASA1), which encodes a rate-limiting enzyme in tryptophan (Trp) biosynthesis and affects PR elongation (Mao et al. 2016). Additionally, OsEIL1 has been shown to bind to the promoters of MHZ10/OsTAR2 and OsYUCCA8 (OsYUC8) to regulate ethylene response in PRs (Qin et al. 2017; Zhou et al. 2022). In the Trp-dependent pathways for auxin biosynthesis, the TAA/YUC pathway dependent on indole-3-pyruvic acid (IPyA) is considered as the major route for auxin production in plants (Zhao 2018). In this pathway, TAA converts Trp into IPyA (Stepanova et al. 2008), which is then converted to the final product, indole-3-acetic acid (IAA), by the rate-limiting step catalyzed by YUCs (Mashiguchi et al. 2011). Knockout mutants of genes involved in this auxin biosynthesis pathway (i.e. AtASA1, AtTAA1, OsTAR2, and OsYUC8) exhibit ethylene-insensitive phenotypes in PR growth and soil compaction response (Stepanova et al. 2005, 2008; Huang et al. 2022; Zhou et al. 2022). Thus, the inhibition of PR growth by ethylene appears to be largely dependent on auxin signaling (Swarup et al. 2007; Giri et al. 2018). Therefore, it seems reasonable to assume that ethylene could also influence the auxin signaling-mediated root gravitropic machinery. However, previous studies on core ethylene signaling mutants in Arabidopsis have provided distinct evidence, demonstrating that these mutants do not display any defects in gravitropism (Buer et al. 2006). Consequently, the prevailing consensus is that ethylene signaling likely does not play an important role in determining root angle in Arabidopsis, as well as in crop plants.
Our study has uncovered noteworthy positive function of ethylene in regulating root gravitropism and root angle, particularly in crop plants, which contradicts prevailing assumption. By investigating several rice ethylene signaling mutants, we have observed a shallow root angle resulting from reduced auxin levels and responses in the root tips and a consequent decrease in root gravitropism. Moreover, we found that mutants defective in auxin biosynthesis in the root tip exhibited phenotypic defects similar to those observed in ethylene mutants, affecting both root gravitropism and angle. Notably, exogenous auxin treatment rescued these defects in both ethylene and auxin mutants, validating an interplay between the 2 hormones in controlling root gravitropism and root angle. The present study provides evidence that the mechanism of ethylene-mediated auxin biosynthesis in the root tip, which controls root gravitropism and root angle, is conserved in maize and potentially other cereal crops. Consequently, these results highlight the functional divergence of ethylene signaling between the model plant Arabidopsis and cereal crops, especially in regulating root angle.
Results
Ethylene-insensitive mutants exhibit shallow root system in rice
Auxin is a crucial factor in determining root angle in Arabidopsis and rice (Bennett et al. 1996; Abas et al. 2006; Giri et al. 2018; Huang et al. 2018), as auxin signaling mutants show reduced root angle (Bennett et al. 1996; Okushima et al. 2005; Giri et al. 2018). Ethylene acts upstream of auxin, and the precise role of ethylene in determining root angle, particularly in cereal crops such as rice, remains unknown. This lack of understanding is partly due to the fact that ethylene mutants in the model plant Arabidopsis have been demonstrated to exhibit no gravitropism defects (Buer et al. 2006). Our research has now confirmed these observations and further revealed that ethylene mutants do not display any PR gravitropism defects (Supplementary Figs. S1 and S2). Additionally, the treatment with 100 nM 1-aminocyclopropane-1-carboxylic acid (ACC) did not significantly affect the lateral root angle phenotype in either the wild type (WT) or any mutants (Fig. 1, A to K). To investigate whether ethylene plays any role in root angle determinant in rice, we characterized root system architecture (RSA) of ethylene-insensitive mutants (osein2/mhz7 and oseil1/mhz6) and WT plants. Our findings revealed that 7-d-old seedlings of osein2 and oseil1 exhibited a shallow crown root angle than WT plants (Fig. 1, L to O). To further validate the phenotypic observations of the seedlings in the paper column, we analyzed the RSA of ethylene-insensitive mutants and WT using the soil monolith sampling method (Uga et al. 2013). Consistently, mature plants of oseil1 and osein2 revealed a larger crown root angle than WT (Supplementary Fig. S3), mimicking the results from the paper column conditions. These observations indicate that ethylene plays distinct roles in regulating RSA in Arabidopsis and rice.
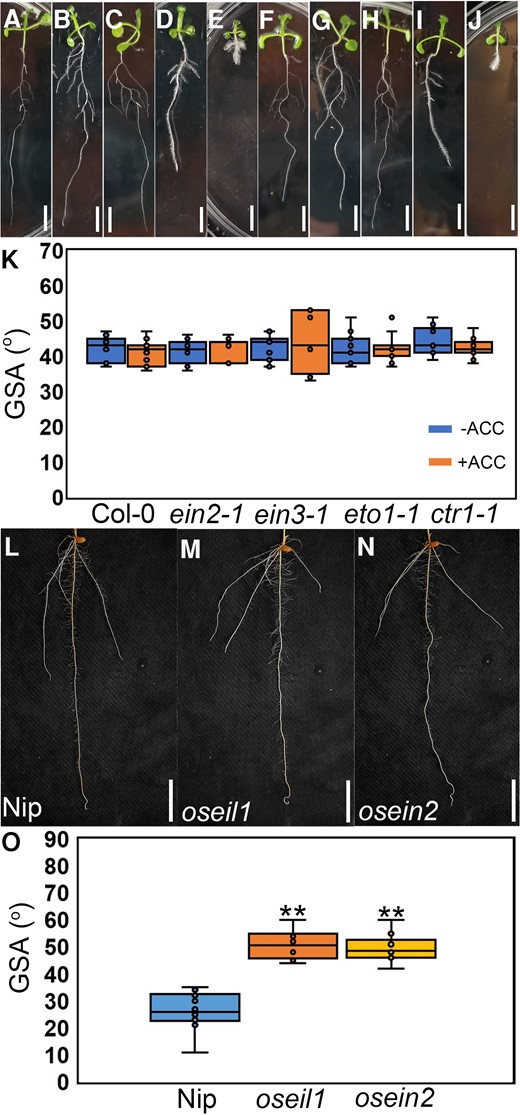
Ethylene-insensitive mutants exhibit shallow root system in rice. A to E) Representative images of root systems of WT (Col-0), ein2-1, ein3-1, eto1-1, and ctr1-1 (from left to right) without 100 nM ACC treatment after 8 d of growth. Scale bars = 1 cm. F to J) Representative images of root systems of WT (Col-0), ein2-1, ein3-1, eto1-1, and ctr1-1 (from left to right) with 100 nM ACC treatment after 8 d of growth. Scale bars = 1 cm. K) GSA analysis (absolute angle of emergence) of Col-0, eto1-1, ctr1-1, ein2-1, and ein3-1 with or without 100 nM ACC treatment. n = 11. The horizontal bar within the box represents median. The top and bottom of the box represent the 0.75 and 0.25 percentiles, respectively. The upper and lower whiskers extend to 1.5 times the interquartile range, with outliers shown as black dots. L to N) Representative root systems of Nip (WT) L), oseil1M), and osein2N) after 8-d growth. Scale bars = 1 cm. O) GSA analysis of Nip, oseil1, and osein2 after 8-d growth. **P < 0.01 from 1-way ANOVA with Tukey's multiple comparison test, n = 10. The horizontal bar within the box represents median. The top and bottom of the box represent the 0.75 and 0.25 percentiles, respectively. The upper and lower whiskers extend to 1.5 times the interquartile range, with outliers shown as black dots.
Ethylene-insensitive mutants exhibit impaired root gravitropism
Different root classes, such as primary, lateral, and crown roots, emerge at a specific angle to build a radiating system (Roychoudhry et al. 2013). The competition between gravitropic and antigravitropic (AGO) mechanisms controls the GSA (Roychoudhry et al. 2013; Huang et al. 2018). To investigate whether ethylene plays a role in either of these mechanisms, we conducted gravitropic bending response assays by turning the plate 90°. We found that osein2 and oseil1 mutants had reduced primary and crown root gravitropism and did not respond to external ACC treatment (Fig. 2; Supplementary Fig. S4), indicating that the gravitropic response machinery of these ethylene mutants was impaired. Normal root elongation was observed in ethylene-insensitive mutants that displayed less response to external ACC treatment (Fig. 2). Notably, we observed that treatment with ethylene precursor ACC and 1-methylcyclopropene (1-MCP) enhanced and reduced crown root gravitropism in WT, respectively (Fig. 2; Supplementary Fig. S5A). This corresponded with the development of deeper and shallower root system in ACC-treated and 1-MCP-treated plants, respectively (Supplementary Fig. S5B). These results suggest that ethylene affects the gravitropic machinery in roots and alters crown root angle, and this process necessitates operative ethylene signaling.
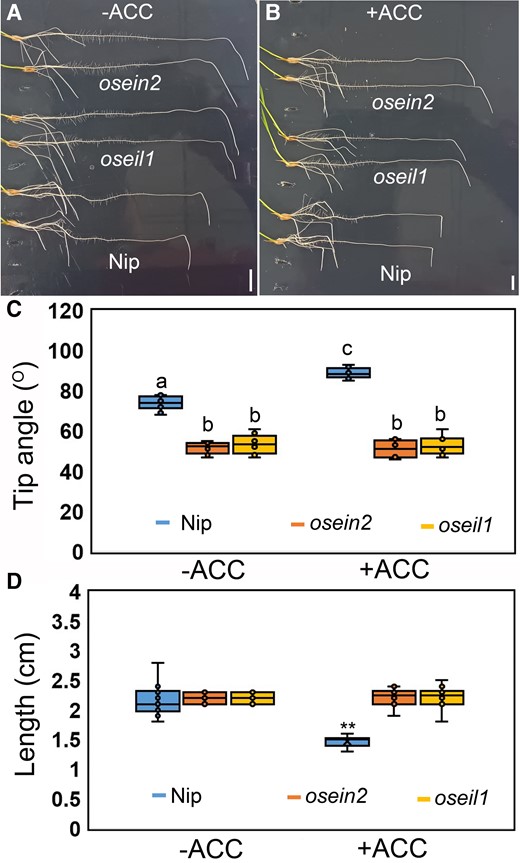
Ethylene-insensitive mutants exhibit reduced gravitropism. A and B) Representative images showing the root gravitropic bending responses of crown roots of Nip and oseil1 and osein2 mutants without A) and with B) 100 µM ACC treatment. Scale bars = 1 cm. C) Measured root tip angle of crown roots of Nip (WT) and oseil1 and osein2 mutants with or without ACC treatment after 8-h gravistimulation. Different letters in the box plot indicate significant differences, P < 0.01 from 1-way ANOVA with Tukey's multiple comparison test, n = 10. The horizontal bar within box represents median. The top and bottom of the box represent the 0.75 and 0.25 percentiles, respectively. The upper and lower whiskers extend to 1.5 times the interquartile range, with outliers shown as black dots. D) Measured elongation length of crown roots of Nip and oseil1 and osein2 mutants with or without ACC treatment after 8-h gravistimulation. **P < 0.01 from 1-way ANOVA with Tukey's multiple comparison test, n = 10. The horizontal bar within the box represents median. The top and bottom of the box represent the 0.75 and 0.25 percentiles, respectively. The upper and lower whiskers extend to 1.5 times the interquartile range, with outliers shown as black dots.
Auxin biosynthesis is affected in the ethylene-insensitive mutants
In numerous growth and developmental processes, ethylene exerts its effects through crosstalk with other phytohormones. Studies on rice roots have shown that auxin and abscisic acid (ABA) act downstream of ethylene (Ma et al. 2014; Yin et al. 2015; Huang et al. 2022). To investigate the involvement of auxin and/or ABA in ethylene-mediated root gravitropism and root angle phenotypes, we measured their basal levels in WT and osein2 root tips. Our hormone profiling analysis revealed that osein2 roots had decreased levels of auxin, but maintained normal levels of ABA compared to WT roots (Supplementary Fig. S6). This finding suggests that auxin, not ABA, plays an important role in ethylene-mediated root gravitropism under normal conditions. In support of this notion, we found that external auxin treatment fully restored the gravitropic defects observed in osein2 and oseil1 mutants, as well as in osers2d, an ethylene-insensitive mutant (Yin et al. 2015; Fig. 3, A and B; Supplementary Fig. S7). Furthermore, normal root growth length was observed in these ethylene-insensitive mutants (Fig. 3C; Supplementary Fig. S7). These results demonstrate that ethylene regulates auxin accumulation to modulate root gravitropic response.
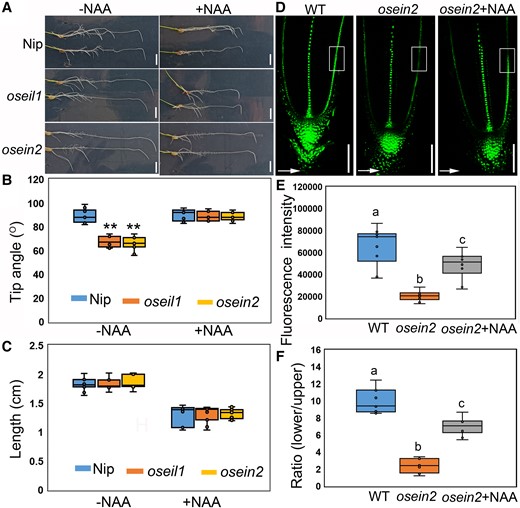
Auxin acts downstream of ethylene in regulating root gravitropism. A) Representative images of Nip (WT) and oseil1 and osein2 mutants after 8-h gravistimulations without and with 10 nM NAA treatment. Scale bars = 1 cm. B) Measured tip angles of 7-d-old PRs of Nip and oseil1 and osein2 mutants after 8-h gravistimulation. n = 11. **P < 0.01 from 1-way ANOVA with Tukey's multiple comparison test. The horizontal bar within the box represents median. The top and bottom of the box represent the 0.75 and 0.25 percentiles, respectively. The upper and lower whiskers extend to 1.5 times the interquartile range, with outliers shown as black dots. C) Measured elongation length of PRs of Nip and oseil1 and osein2 mutants with or without NAA treatment after 8-h gravistimulation. n = 11. The horizontal bar within the box represents median. The top and bottom of the box represent the 0.75 and 0.25 percentiles, respectively. The upper and lower whiskers extend to 1.5 times the interquartile range, with outliers shown as black dots. D) Representative confocal images showing DR5-VENUS i.e. auxin response gradient after 4-h gravistimulation in the 7-d growth seedlings of Nip and osein2 with or without 10 nM NAA treatment. Scale bars = 100 µm. Arrows represent the direction of gravity. E) Box plot showing the quantitative intensity of fluorescence signals in the region of interest (ROI) of the lower side of early elongation zone. Different letters indicate significant differences, P < 0.01 from 1-way ANOVA with Tukey's multiple comparison test, n = 11. The horizontal bar within the box represents median. The top and bottom of the box represent the 0.75 and 0.25 percentiles, respectively. The upper and lower whiskers extend to 1.5 times the interquartile range, with outliers shown as black dots. F) Box plot showing the fluorescence intensity ratio of lower side to upper side in the ROI of early elongation zone. Different letters indicate significant differences, P < 0.01 from 1-way ANOVA with Tukey's multiple comparison test, n = 11. The horizontal bar within box represents median. The top and bottom of the box represent the 0.75 and 0.25 percentiles, respectively. The upper and lower whiskers extend to 1.5 times the interquartile range, with outliers shown as black dots.
When roots are exposed to gravity, auxin is redistributed to the lower side of the root tip, leading to an enhanced response that impedes cell elongation and results in root bending during gravitropism (Band et al. 2012; Yang et al. 2017). To investigate whether auxin response is affected in ethylene mutants during root gravitropism, we employed a widely used rice auxin reporter DR5-VENUS to monitor auxin gradient ratio of the lower to upper side and also auxin distribution on the lower side of roots. Confocal imaging showed reduced auxin response in osein2 roots compared to WT roots following a 4-h gravity stimulation (Fig. 3, D to F), suggesting reduced auxin accumulation and thus auxin response in osein2. Additionally, we found that reduced auxin of osein2 roots could be partially restored by exogenous treatment with NAA (Fig. 3, D to F), providing evidence that auxin biosynthesis is impaired in rice ethylene mutants.
Functional MHZ10 is required for ethylene-mediated auxin biosynthesis
The ethylene signaling component OsEIL1 has been shown to directly activate OsYUC8 and MHZ10 genes involved in auxin biosynthesis during ethylene-mediated inhibition of root elongation (Qin et al. 2017; Zhou et al. 2022). The transgenic lines ProMHZ10::VENUS-N7 and ProOsYUC8::GUS provided evidence that MHZ10 exhibited predominant expression in cortex, quiescent center, columella, and stele of the root, while OsYUC8 was mainly expressed in root apex (Supplementary Fig. S8). In order to determine the potential involvement of OsYUC8 and/or MHZ10 in the ethylene-mediated gravitropic response in roots, the expression levels of OsYUC8 and MHZ10 in ethylene mutants were monitored. Interestingly, the expression of MHZ10 (rather than OsYUC8) was reduced in ethylene-insensitive mutants (Supplementary Fig. S9), implying that MHZ10-mediated auxin biosynthesis was involved in ethylene-mediated gravitropism in normal conditions. To verify this assumption, experiments were conducted using osyuc8-2 and mhz10-1 mutants under both control and gravistimulated conditions. The osyuc8-2 mutant lacks any transcripts due to the T-DNA insertion (Woo et al. 2007), while mhz10-1 harbors an A-to-G base substitution at a 41-bp position upstream of the start codon, which disrupts its binding affinity to OsEIL1 (Zhou et al. 2022). Consistently, mhz10-1 rather than osyuc8-2 exhibited reduced root gravitropism, phenocopying that of ethylene-insensitive mutants (Fig. 4, A to C; Supplementary Fig. S10). Additionally, auxin response during gravitropic bending response was attenuated in mhz10-1 roots, which were crossed with DR5 reporter, compared to the WT DR5 reporter (Supplementary Fig. S11). These findings suggest that MHZ10-dependent auxin biosynthesis is required for ethylene-mediated root gravitropism under normal conditions. It is plausible that OsYUC8 may not be involved in ethylene-mediated local auxin biosynthesis during gravitropism, or other OsYUCs could have overlapping roles with OsYUC8, given there are 14 OsYUCs present in rice.
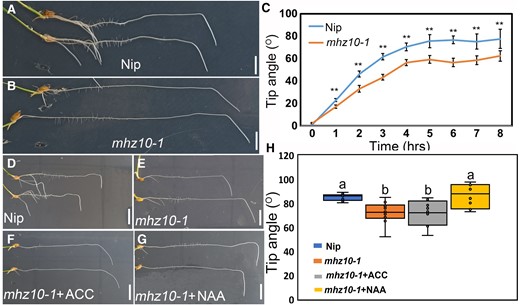
MHZ10-mediated auxin biosynthesis acts downstream of ethylene in regulating root gravitropism. A and B) Representative images of gravitropic responses of PRs in Nip (WT) A) and mhz10-1B). Scale bars = 1 cm. C) Tip angle analysis of 7-d growth PR of Nip and mhz10-1. Error bars are ± Sd, n = 11. **Indicates significant differences, P < 0.01 from Students’ t test. D to G) Representative root images of 7-d growth seedlings after 8-h gravistimulation of Nip under control treatment and mhz10-1 mutant under control, 100 µM ACC, and 10 nM NAA treatments. Scale bars = 1 cm. H) Measured tip angles of D to G). Different letters indicate significant differences, P < 0.01 from 1-way ANOVA with Tukey's multiple comparison test, n = 10. The horizontal bar within the box represents median. The top and bottom of the box represent the 0.75 and 0.25 percentiles, respectively. The upper and lower whiskers extend to 1.5 times the interquartile range, with outliers shown as black dots.
To confirm the role of MHZ10 in ethylene-mediated auxin biosynthesis, we tested the responsiveness of mhz10-1 to exogenous auxin and ethylene treatments. Consistent with our initial hypothesis, exogenous NAA treatment rescued the gravitropic defects of mhz10-1 (Fig. 4, D to H). Additionally, mhz10-1 did not show any response to exogenous ACC treatment (Fig. 4, D to H), suggesting that ethylene regulates root gravitropism in a MHZ10-dependent manner. We also examined whether MHZ10 regulates RSA like ethylene signaling mutants. Remarkably, mhz10-1 exhibited shallow RSA in the paddy field at a mature stage, similar to that of ethylene-insensitive mutants (Supplementary Figs. S3 and S12). These results underscore the importance of ethylene-mediated auxin biosynthesis in the root tip for the formation of steep root system by modulating root gravitropism.
Conserved ethylene–auxin biosynthesis mechanism regulates root angle in maize
We investigated whether the ethylene–auxin regulatory mechanism regulating root angle in rice is also conserved in other cereal crops, such as maize. To disrupt ethylene signaling transduction in maize, we selected ZmEIN2 as the target gene as there was no known functionally redundant gene for this gene (Gallie and Young 2004; Zhou et al. 2019). As no mutant was available for ZmEIN2 in public maize transposon insertion collections, we used CRISPR-Cas9 technology (Dong et al. 2018) to knockout ZmEIN2. We obtained 3 CRISPR edits leading to frameshifts in the CDS of ZmEIN2 gene, resulting in 3 allelic mutants: zmein2-1, zmein2-2, and zmein2-3 with 569 and 21 bp deletions, respectively (Supplementary Fig. S13A). All 3 allelic mutants of ZmEIN2 exhibited reduced gravitropism (Supplementary Fig. S13, B to D), similar to what was observed in the abovementioned rice studies. Furthermore, the gravitropic defects of zmein2 mutants could be rescued by exogenous NAA treatment (Supplementary Fig. S13, B to D). Consistently, zmein2-1 formed a shallow root system with less response to ACC treatment (Fig. 5), while it shaped a deep root system after auxin treatment (Fig. 5). These results suggest that the ethylene–auxin biosynthesis-mediated root gravitropism pathway, which controls root angle, is conserved in rice and maize and possibly other cereal crops.
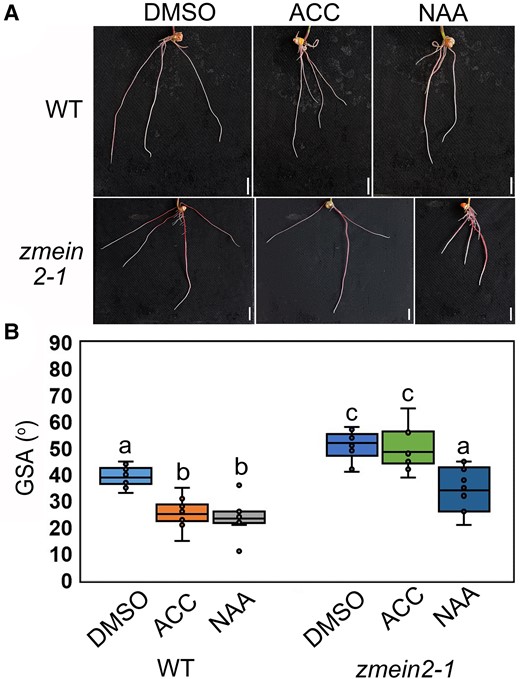
Maize ethylene-insensitive mutant also exhibits shallow root system. A) Representative images of 7-d growth root systems of WT and zmein2-1 under control treatment (DMSO) and zmein2-1 under 100 µM ACC and 100 nM NAA treatments. Scale bars = 1 cm. B) Measured GSA of WT and zmein2-1 grown under control treatment and zmein2-1 with 100 µM ACC and 100 nM NAA treatments after 7-d growth. Different letters indicate significant differences, P < 0.01 from 1-way ANOVA with Tukey's multiple comparison test, n = 10. The horizontal bar within the box represents median. The top and bottom of the box represent the 0.75 and 0.25 percentiles, respectively. The upper and lower whiskers extend to 1.5 times the interquartile range, with outliers shown as black dots.
Discussion
The orientation of roots is a key determinant of a plant's capacity to obtain nutrients and water from various layers of soil. Consequently, it is considered as a desirable trait for crop improvement and breeding. Deviations in root angle can affect the spatial distribution of roots in the soil layers, thereby influencing stress tolerance. For instance, a shallow root system can efficiently capture phosphate from the topsoil (Huang et al. 2018), whereas a steep root system facilitates access to mobile water and nitrate from deeper soil layers (Uga et al. 2013). Therefore, modifying RSA by fine-tuning root angle is an important objective for enhancing the resilience and sustainability of crops (de Dorlodot et al. 2007; Rogers and Benfey 2015). However, the understanding of molecular mechanisms governing root angle in major crops such as rice and maize is currently limited. Our study has shown that ethylene-mediated auxin biosynthesis plays a critical role in the development of deep root systems in rice and maize (Fig. 6). Specially, our findings indicate that ethylene positively influences root gravitropism via stimulating MHZ10-mediated auxin biosynthesis (Fig. 6). Notably, our results revealed that ethylene-insensitive mutants shaped shallow RSA with diminished MHZ10-mediated auxin biosynthesis; in contrast, ethylene-sensitive mutants display deep RSA with upregulated MHZ10-mediated auxin biosynthesis (Fig. 6). This interplay between ethylene and auxin signaling pathways in regulating root angle is also conserved in maize (Fig. 5). Taken together, our findings suggest that ethylene-mediated auxin biosynthesis is essential for the appropriate root angle in rice and maize, as well as potentially other cereal crops.
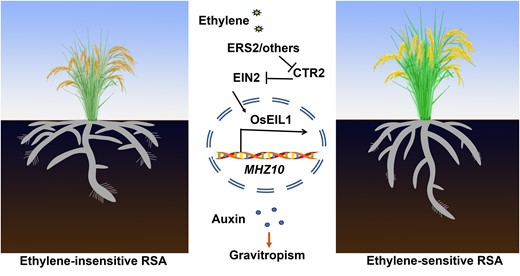
A proposed model for how endogenous ethylene regulates RSA. During the developmental process of root system, endogenous ethylene was perceived by ethylene receptors, which fail to activate CTR2, reducing its repression on OsEIN2. OsEIL1 is directly responsible for activating the expression of MHZ10, an auxin biosynthesis gene, which is necessary for the formation of deep RSA via enhancing gravitropism. Ethylene-insensitive mutants exhibit shallow root system ascribed to reduced gravitropism.
Auxin plays a critical role in regulating root angle, as it operates in both gravitropic and AGO mechanisms (Giri et al. 2018; Huang et al. 2018). While ethylene is known to act upstream of auxin, particularly in regulating root hair expansion and PR elongation (Rahman et al. 2002; Růžička et al. 2007; Swarup et al. 2007), its role in controlling root angle remains unclear. In Arabidopsis thaliana, the role of ethylene in these mechanisms is not fully explored, possibly due to the observation that ethylene-insensitive mutants (Atein2 and Atetr1) have similar or slightly better gravitropic curvature than the control plants (Buer et al. 2006). However, the utilization of ACC was shown to attenuate the gravitropic response of WT roots (Buer et al. 2006), suggesting a negative role in root gravitropism. Our research also confirmed that ethylene signaling mutants in Arabidopsis do not exhibit gravitropism defects as evidenced by the absence of lateral and PR angle defects (Fig. 1, A to K; Supplementary Fig. S2). Interestingly, we observed that ACC treatment enhanced root gravitropism in rice, and ethylene signaling mutants showed reduced gravitropism (Fig. 2; Supplementary Figs. S4 and S7). These findings suggest that the divergence of the regulatory mechanisms controlling root angle may be attributed to morphological differences between the tap root and fibrous root systems.
As a multifunctional hormone, the interplay of ethylene and other hormones has been extensively studied in rice (Růžička et al. 2007; Swarup et al. 2007; Qin et al. 2017; Huang et al. 2022). For instance, OsEIL1, an essential component of ethylene signaling, has been shown to bind to the promoters of OsYUC8 and MHZ10 in an ethylene-mediated response (Qin et al. 2017; Zhou et al. 2022). OsEIL1-OsYUC8 cascade was recently found to modulate root compaction response (Huang et al. 2022). In this work, mutant of MHZ10 (rather than OsYUC8) showed reduced gravitropism (Fig. 4, A to C; Supplementary Fig. S10), suggesting existence of specific ethylene–auxin regulatory modules for particular growth responses. MHZ10/OsTAR2 has 3 homologs in rice, namely OsTAR1, OsTARL1, and OsTARL2 (Guo et al. 2020). OsTAR1 has been found to regulate grain development, and no aminotransferase activity was detected for OsTARL1 and OsTARL2 (Yoshikawa et al. 2014). Thus, it is possible that MHZ10 plays a major role in root development including root gravitropism. While, there are 14 functional YUCs, at least 3 OsYUCs (OsYUC5, OsYUC8, and OsYUC11) were induced by ethylene in roots (Qin et al. 2017). Presumably, with other OsYUCs playing redundant roles, OsYUC8 alone may not have a clear or primary role in regulating ethylene-mediated root gravitropism.
What is the relevance of ethylene in regulating root angle? The biosynthesis of ethylene is a highly regulated process that can be activated by diverse abiotic and biotic stresses (Savada et al. 2017). The interactions between ethylene and stress signals are pivotal in determining root system plasticity. It is widely accepted that steep RSA is beneficial in absorbing water from deep soil profiles (de Dorlodot et al. 2007). Considering that water deficiency induces ethylene production in rice (Apelbaum and Yang 1981), and our research show that ethylene can enhance crown root gravitropism and root angle (Fig. 2), we can speculate that the regulation of root angle by ethylene may enable plants to develop steep root system during water stress, helping them escape drying topsoils or access water in subsoil profiles.
ABA is suggested to act downstream of ethylene in root–ethylene response in rice (Yin et al. 2015). Its accumulation is crucial for mitigating drought stress and promoting root gravitropism in maize (Feng et al. 2022). The strong induction of ZmDRO1 by external ABA or drought leads to a larger downward root angle and a deeper RSA (Feng et al. 2022). Consistently, our findings demonstrate that ethylene has a favorable effect on root gravitropism in both rice and maize through the stimulation of auxin biosynthesis (Fig. 3; Supplementary Fig. 6). However, in Arabidopsis, ABA appears to have antagonistic effects or no effects on root gravitropism, particularly in the context of hydrotropism (Dietrich et al. 2017; Miao et al. 2021). Similarly, mutants with impaired ABA signaling do not exhibit any defects in root gravitropism (Dietrich et al. 2017; Miao et al. 2021). These observations suggest that ABA assumes different functions in dicot (Arabidopsis) and monocot (rice and maize) plants. Therefore, it is possible that, consistent with the observation for ABA, ethylene also has different functions in regulating root gravitropism and root angle in Arabidopsis and rice.
In summary, our study has yielded valuable insights into the functions of the ethylene–auxin cascade that ethylene regulates auxin biosynthesis in the root tip of rice and maize. This regulation ultimately affects root gravitropism, leading to alterations in root angles, a phenomenon that differs from Arabidopsis. These findings have the potential to contribute to the optimization of deep RSA in response to changing environmental conditions.
Materials and methods
Plant materials and growth condition
All rice (Oryza sativa) and maize (Zea mays) plants for seed generation were cultured in paddy field of Shanghai (30°N, 121°E) and Sanya (18°N, 109°E), China, in summer and winter, respectively. The backgrounds of osein2/mhz7-1, oseil1/mhz6-1, osers2d, and ostar2/mhz10-1 were in Nipponbare (Nip; Ma et al. 2013), and osyuc8-2 was in Hwayoung (HWY; Qin et al. 2017). Seeds were germinated on moist, sterilized filter paper for 4 d in the dark at 28 °C in a growth chamber with 70% relative humidity. Seeds of Arabidopsis (A. thaliana) ecotype Col-0 (WT) and ethylene signaling mutants (eto1-1, ctr1-1, ein2-1, and ein3-1) were surface sterilized with 70% (v/v) ethanol for 1 min and 20% (v/v) bleach with 0.01% (v/v) Triton X-100 for 8 min, followed by 5 washes with sterile water and stratified at 4 °C for 48 h in the dark. Germinated seeds were grown on 1/2 MS plates supplemented with 1% Phytagel, at pH 5.8 in a growth room (22 °C, 16-h day/8-h night, 100 to 120 µmol/m2/s).
Maize mutant generation
The knockout target was chosen based on its high specificity in relation to the KN5585 genome (http://crispr.hzau.edu.cn/CRISPR2/). The knockout vector was designed and its sequence verified using the Pcxb053 backbone (Liu et al. 2020). Subsequently, the plasmid was introduced into Agrobacterium tumefaciens strain EHA105 through electroporation. The transformation experiments were carried out by Wimi Biotechnology (http://www.wimibio.com/). The process of Agrobacterium-mediated maize transformation has been previously described (Liu et al. 2020). Maize immature embryos measuring 1.5 to 1.8 mm were extracted from ears collected 10 d after pollination and placed in 2.0-mL tubes containing 1.8 mL of inoculation medium. These embryos were then exposed to an Agrobacterium suspension (consisting of inoculation medium with 200 mM of acetosyringone and Agrobacterium cells) for a duration of 5 min, after which they were transferred onto cocultivation medium. Excess liquid was eliminated using pipettes. The immature embryos were positioned with the scutellum side facing upward on the medium and incubated in darkness at a temperature of 23 °C for a period of 48 to 72 h for cocultivation. Following cocultivation, immature embryos were subsequently transferred to a resting medium and cultured for a period of 5 to 7 d. The resulting calluses were then transferred to a selection medium containing glufosinate-ammonium at a concentration of 10 mg/L. These calluses were incubated in darkness at a temperature of 28 °C for a duration of 2 weeks, after which they were transferred to a fresh selection medium for an additional 2 weeks. The calluses that exhibited resistance were then placed on a regeneration medium and incubated under a light intensity of 5,000 lx at a temperature of 25 °C for a period of 14 to 21 d. The regenerated shoots were subsequently transferred to a rooting medium and exposed to a light intensity of 5,000 lx at a temperature of 25 °C for a duration of 14 d. Prior to planting the plantlets in a greenhouse, leaves were sampled for PCR analysis. Primers are listed at Supplementary Table S1.
Root system observation
To observe root system of Nip, osein2, oseil1, and mhz10-1 in the soil, we used a modified trench method (Uga et al. 2013). Plants were grown in paddy field with well irrigation in Shanghai, China, in the summer of 2020 and 2021. After 100 d of planting, the whole soil monolith (20 cm × 20 cm × 5 cm) was picked up from the soil. Then, the soil monolith was carefully fixed on a board (with 10-cm nails). Finally, the root systems were gently washed out with flowing water. The GSA was measured at the emergence site of crown roots. For root system observation on a paper column, the seeds were germinated in darkness for 4 d, and then the germinated seeds were transferred to a paper column wrapped in aluminum foil for an additional 7 d. The GSA was measured at the emergence site of crown root.
NAA and ACC treatments
The rice seeds were germinated under constant darkness at 28 °C for 3 d and then transferred into 96-well plates without bottom floating on water with 18-h lightness and 6-h darkness at 28 °C. For ACC treatment, germinated seedlings were transferred onto the surface of 1% agar with or without addition of 100 µM ACC for 2 h at vertical conditions and then were placed horizontally for 24 h. Photographs were taken automatically every 1 h with a digital camera (Canon) under the control of ZoomBrowser EX software (Canon). The PR curvature was defined as the angle formed between the growing direction of the apex and the horizontal base line and was measured on the digital images using ImageJ (http://rsb.info.nih.gov/ij/). The maize seeds were germinated for 2 d under darkness at 30 °C, and the germinated seeds were transferred into a paper column containing 100 µM ACC or 100 nM NAA. After 4-d growth, the root system was imaged and analyzed. GSA of crown roots was measured at the insertion site between the crown root and PR with reference to the gravity vector. Arabidopsis WT and ethylene signaling mutants were grown on 1/2 MS 1% agar plates initially for 3 d, during which no lateral roots were visible. Equally germinated seedlings were transferred to fresh 1/2 MS 1% agar plates supplemented with 0 and 100 nM ACC and grown for 6 d. Plates were imaged using Nikon D5100 camera, and the lateral root angle (measured at the insertion site between lateral root and PR) was quantified using the angle tool in ImageJ. For Arabidopsis gravitropic assay, the seeds were grown on 1/2 MS 1% Phytagel plates for 3 d in darkness and kept at 4 °C, and then the germinated seeds were cultured vertically for 4 d with 12-h lightness/12-h darkness prior to 8-h gravistimulation. The plates were imaged by camera and then analyzed by ImageJ.
Hormone measurements
Rice seeds were germinated for 4 d in darkness kept at 28 °C, and then the germinated seeds were transferred on plates at 28 °C in 16-h lightness/8-h darkness period. After 7 d of growth, the seedlings were transferred on new 1% agar plates for 24 h. The seedlings were oriented horizontally by rotating the plates counterclockwise at a 90° angle for a duration of 6 h, and 1-cm crown root tips were harvested and stored in the liquid nitrogen. Hormone measurements were performed and analyzed at Metware Company (https://www.metware.cn/).
Gravitropism analysis
The rice/maize seeds were germinated under constant dark at 28 °C for 3 d and then transferred into 96-well plates without bottom floating on water with 18-h light and 6-h dark at 28 °C. The 3-d-old seedlings were placed on 1% agar in normal condition for 1 h and then were placed horizontally by rotating the plates counterclockwise. Photographs were taken automatically every 20 min by a digital camera (Canon) under the control of ZoomBrowser EX software (Canon). The PR curvature was defined as the angle formed between the growing direction of the apex and the horizontal base line and was measured on the digital images using ImageJ (http://rsb.info.nih.gov/ij/).
Confocal imaging
DR5-VENUS reporter seeds were germinated in the dark for 4 d and then transferred onto agar surface to grow for another 5 d under 28 °C. The roots were observed via Leica Laser Scan Microscope (SP5) using an excitation wavelength of 488 nm, intensity less than 10, collection bandwidth of 500 to 550 nm, and gain around 800. Confocal images were analyzed via ImageJ (https://imagej.nih.gov/ij/).
Accession numbers
Sequence data from this article can be found in the GenBank/EMBL data libraries under the following accession numbers: OsYUC8 (Os03g0162000); OsEIN2/MHZ7 (Os07g0155600); OsEIL1/MHZ6 (Os03g0324200); OsERS2 (LOC_Os05g06320); OsTAR2/MHZ10 (Os01g0169800); AtCTR1 (AT5G03730); AtEIN2 (AT5G03280); AtEIN3 (AT3G20770); AtETO1 (AT3G51770); and ZmEIN2 (GRMZM2G068217).
Acknowledgments
We thank Prof. Jinsong Zhang for kindly providing mhz10-1/ostar2 and osers2d seeds. We especially appreciate the respected Prof. Dabing Zhang for his helpful comments on the manuscript, who passed away on June 22, 2023.
Author contributions
X.K., R.B., and G.H. designed the project. X.K., Y.X., X.S., S.Y., S.W., and J.R. performed all the assays. X.K., Y.X., X.S., S.Y., S.W., A.L., M.L., R.F., and J.R. analyzed and discussed the data. G.H., R.B., and X.K. wrote the manuscript.
Supplementary data
The following materials are available in the online version of this article.
Supplementary Figure S1. Genotyping verification of the mutants used in this study.
Supplementary Figure S2. Ethylene-insensitive mutants exhibit no obvious changes in PR angle in altered gravitropic conditions in A. thaliana.
Supplementary Figure S3. Ethylene-insensitive mutants exhibit shallow root system.
Supplementary Figure S4. Ethylene-insensitive mutants exhibit reduced gravitropism.
Supplementary Figure S5. Roots impaired in ethylene signaling show reduce gravitropism and shallow RSA.
Supplementary Figure S6. Auxin accumulation is reduced in osein2.
Supplementary Figure S7. Auxin treatment restores the gravitropic responses of ethylene receptor mutant (osers2d).
Supplementary Figure S8. Expression pattern of MHZ10 and OsYUC8 in PR.
Supplementary Figure S9. MHZ10 expression is reduced in ethylene-insensitive mutants.
Supplementary Figure S10. Auxin biosynthesis mutant (osyuc8-2) shows similar gravitropic responses to WT with or without ACC treatment.
Supplementary Figure S11. Auxin response is disrupted in the mhz10-1 mutant.
Supplementary Figure S12.mhz10-1 mutant exhibits shallow root system.
Supplementary Figure S13. Knockout mutants of ZmEIN2 exhibit reduced gravitropism.
Supplementary Table S1. Primers used in this study
Funding
This work was supported by the National Natural Science Foundation of China (32101651 and 32130006), Shanghai Rising Star Program (22QA1404200), China Innovative Research Team, Ministry of Education, and Programme of Introducing Talents of Discipline to Universities (111 Project, B14016). R.B. acknowledges support from the Future Food Beacon Nottingham Research fellowship, and BBSRC (Biotechnology and Biological Sciences Research Council) Discovery Fellowship (BB/S011102/1), and BBSRC New Investigator Research Grant (BB/X014843/1), and BBSRC funded Delivering Sustainable Wheat Partner Grant (BB/X018806/1). S.W. acknowledges BBSRC DTP studentship. A.L. acknowledges the Nottingham Future Food Beacon and Puri Fellowship. R.F. acknowledges BBSRC funded BREAKTHRU grant (BB/W008874/1).
Data availability
All study data are included in the main text and supporting information.
Dive Curated Terms
The following phenotypic, genotypic, and functional terms are of significance to the work described in this paper:
References
Author notes
Xiuzhen Kong, Yali Xiong and Xiaoyun Song contributed equally.
The authors responsible for distribution of materials integral to the findings presented in the article in accordance with the policy described in the Instructions for Authors (https://dbpia.nl.go.kr/plphys/pages/General-Instructions) are Guoqiang Huang ([email protected]) and Rahul Bhosale ([email protected]).
Conflict of interest statement. The authors declare that they have no competing interests.