-
PDF
- Split View
-
Views
-
Cite
Cite
Guangyu Luo, Lin Li, Xiaoyu Yang, Yu Yu, Lei Gao, Beixin Mo, Xuemei Chen, Lin Liu, MicroRNA1432 regulates rice drought stress tolerance by targeting the CALMODULIN-LIKE2 gene, Plant Physiology, Volume 195, Issue 3, July 2024, Pages 1954–1968, https://doi.org/10.1093/plphys/kiae127
- Share Icon Share
Abstract
Due to climate change, drought has become a major threat to rice (Oryza sativa L.) growth and yield worldwide. Understanding the genetic basis of drought tolerance in rice is therefore of great importance. Here, we identified a microRNA, miR1432, which regulates rice drought tolerance by targeting the CALMODULIN-LIKE2 (OsCaML2) gene. Mutation of MIR1432 or suppression of miR1432 expression significantly impaired seed germination and seedling growth under drought-stress conditions. Molecular analysis demonstrated that miR1432 affected rice drought tolerance by directly targeting OsCaML2, which encodes an EF-hand chiral calcium-binding protein. Overexpression of a miR1432-resistant form of OsCaML2 (OEmCaML2) phenocopied the mir1432 mutant and miR1432 suppression plants. Furthermore, the suppression of miR1432 severely affected the expression of genes involved in responses to stimulation, metabolism and signal transduction, especially the mitogen-activated protein kinase (MAPK) pathway and hormone transduction pathway in rice under drought stress. Thus, our findings show that the miR1432-OsCaML2 module plays an important role in the regulation of rice drought tolerance, suggesting its potential utilization in developing molecular breeding strategies that improve crop drought tolerance.
Introduction
Rice (Oryza sativa L.) feeds more than half the world's population and plays an essential role in safeguarding global food security (Samal et al. 2018). Changes in climate conditions have recently subjected rice to various stresses (Lesk et al. 2016) of which water deficiency is considered to have the largest effect on its development from the vegetative stage to the reproductive stage. Scarcity of water negatively affects a number of physiological processes in rice, such as decreases in net photosynthetic rate, transpiration rate, stomatal conductance and membrane stability index (Mishra et al. 2018; Zhu et al. 2020), resulting in an average yield reduction of 25% (Zhang et al. 2018b).
MicroRNAs (miRNAs) are a class of small non-coding RNAs of 20-24 nucleotides that are widely present in eukaryotic genomes (Carrington and Ambros 2003; Pandey et al. 2008). Plant miRNAs mediate sequence-specific gene silencing of target genes at the post-transcriptional level by transcript cleavage or translational inhibition (Jeong et al. 2011). miRNAs have been found to target multiple regulatory genes and to be involved in many aspects of plant growth and development; thus, the manipulation of miRNA expression levels is considered to be a promising genetic tool for the improvement of crop agronomic traits (Li and Zhang 2016; Shriram et al. 2016; Li et al. 2017). In addition to regulating plant growth and development, miRNAs also mediate plant responses to multiple biotic and abiotic stresses (Boonburapong and Buaboocha 2007). This is achieved by regulation of specific target genes, most of which are transcription factors, and thus constitutes an important part of the plant stress-response mechanism (Zhou et al. 2013). A substantial number of miRNAs have been reported to be differentially expressed during drought stress in many plant species, such as rice (Zhou et al. 2013; Awasthi et al. 2019), potato (Solanum tuberosum L.) (Zhang et al. 2014), cotton (Gossypium hirsutum L.) (Wang et al. 2013; Lu et al. 2019), barley (Hordeum vulgare L.) (Hackenberg et al. 2015) and mustard (Brassica juncea L.) (Bhardwaj et al. 2014). Moreover, recent studies have demonstrated that some miRNAs, such as miR164 (Fang et al. 2014), miR166 (Zhang et al. 2018a), miR319 (Zhou et al. 2013) and miR408 (Hang et al. 2021), play important roles in regulating plant drought resistance. An improved understanding of the miRNA-mediated regulatory mechanisms involved in drought resistance would help to elucidate the regulatory networks that control drought stress responses and adaptation to water deficiency and may facilitate the genetic engineering of drought-tolerant crop varieties.
Calcium ions (Ca2+) are a critical second messenger involved in plant adaptations to environmental stimuli, and an increased cytosolic Ca2+ concentration is essential for various stress responses (Zeng et al. 2015). To transmit information and activate downstream cellular responses, such Ca2+ signals need to be decoded and relayed to effector systems. This is carried out by a variety of Ca2+-binding sensor proteins, including calmodulins (CaMs)/calmodulin-like proteins (CMLs), Ca2+-dependent protein kinases (CDPKs), and calcineurin B-like proteins (CBLs; Kudla et al. 2018). Most of these Ca2+ sensors are identified by the presence in their sequences of EF-hand motifs, which allow the binding of Ca2+ (Aldon et al. 2018). CaMs are relatively small, conserved Ca2+-binding proteins that contain 4 EF-hand motifs and are found in all eukaryotic cells (McCormack et al. 2005). Plants also possess a broad range of CMLs, which are closely related to CaMs, but are of varying length, harboring 1 to 6 EF-hand motifs; they do not possess other known functional domains. In contrast to the constitutive expression of CaMs, CMLs show distinct expression patterns in different developmental stages or tissues (Zhu et al. 2015; Aldon et al. 2018). Transcriptome data have revealed that CML genes can respond to multiple environmental stresses (Zeng et al. 2015). More importantly, accumulating evidence demonstrates that CMLs play a critical regulatory role in Ca2+ signaling during plant adaptation to abiotic stresses (Yin et al. 2015; Wu et al. 2018). However, at present, CMLs remain the least investigated group of Ca2+ sensors, and the molecular functions and regulatory mechanisms of CMLs involved in stress responses are largely unknown and need further exploration.
Recent studies have shown that microRNA1432 (miR1432) can target the acyl-CoA thioesterase gene (OsACOT), which is involved in the biosynthesis of fatty acids, and thus affects grain weight in rice by regulating the rate of grain filling (Zhao et al. 2019). In addition, miR1432 participates in pathogen defense in rice and contributes to rice blast disease and bacterial blight resistance (Jia et al. 2021; Li et al. 2021). However, it remains largely unknown whether miR1432 has a role in regulating plant drought tolerance. In this study, we found that the expression of miR1432 is substantially induced by drought stress in rice, and blocking miR1432 greatly impairs the growth and development of rice under drought stress conditions, indicating that miR1432 acts as an essential regulator of rice drought resistance. We also demonstrate that the CALMODULIN-LIKE2 (OsCaML2) gene is targeted by miR1432, and miR1432 affects rice drought resistance by negatively regulating the abundance of OsCaML2 transcripts.
Results
miR1432 is strongly induced by drought stress in rice
To investigate the response of miR1432 to drought stress, D-mannitol was used to simulate the effect of drought stress in 10-d-old rice seedlings. Northern blotting showed that levels of miR1432 substantially increased in shoots from 90 min after drought treatment and remained high throughout the treatment time (Fig. 1A). In roots, miR1432 responded to drought stress more promptly and was elevated from 30 min after treatment, and then remained at a high expression level, as in shoots (Fig. 1B). Reverse transcription quantitative PCR (RT-qPCR) analysis further showed that expression of the primary transcript of miR1432 (pri-miR1432) was strongly induced within 30 min after D-mannitol treatment in roots, suggesting that MIR1432 transcription was activated by drought stress (Fig. 1C). In addition, in MIR1432p::GUS transgenic plants, a GUS activity assay showed that GUS expression gradually increased with treatment time, further demonstrating that the miR1432 promoter was activated by drought stress in both shoots and roots (Fig. 1, D and E). Analysis of the miR1432 promoter revealed the existence of TATC-box, TATA-box, ABRE, and Sp1 cis-acting elements (Supplementary Fig. S1A), which are all implicated in drought stress responses (Fujita et al. 2005; Fan et al. 2011; Lv et al. 2017). To understand the spatiotemporal expression pattern of MIR1432, GUS expression assays and RT-qPCR analyses were performed, showing that MIR1432 was expressed in almost all rice organs at various developmental stages, with higher levels in young roots and mature leaves, but lower levels in inflorescences (Supplementary Fig. S1, B to J), which is consistent with previous studies (Zhao et al. 2019). Taken together, these results show that the expression of miR1432 is markedly induced by drought stress, indicating that miR1432 may play an important role in the regulation of drought response in rice.
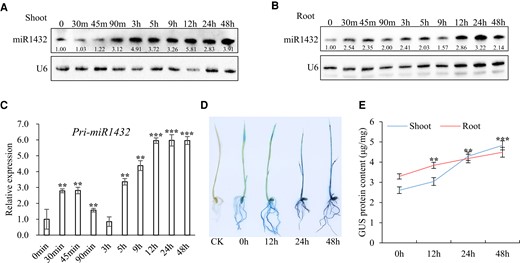
The expression of rice miR1432 is induced by drought stress. A and B) Northern blot to detect the accumulation of miR1432 in rice shoots A) and roots B) at various time points under 150 mM d-mannitol treatment for various times. Three biological replicates were conducted for each blot. U6 served as a loading control. The numbers below the blots indicate the relative abundance of miR1432 compared to the 0-h control sample. m, minutes; h, hours. C) Use of RT-qPCR to detect induced expression of pri-miR1432 in roots of rice seedlings treated with 150 mM d-mannitol. UBQ5 was used as an internal control. The data are presented as means ± Sd. Asterisks indicate a significant difference compared to 0 h (Student's t test: **P < 0.01 and ***P < 0.001). D) GUS staining of 7-d-old seedlings of MIR1432p::GUS transgenic lines following treatment with 150 mM d-mannitol at 0, 12, 24, and 48 h. The WT nontransgenic line was used as the negative control (CK). E) Quantitative analysis of GUS protein content (μg/mg) in shoots and roots of 7-d-old rice seedlings treated with 150 mM d-mannitol for various times. The data are presented as means ± Sd. Asterisks indicate a significant difference compared to 0 h (Student's t test: **P < 0.01 and ***P < 0.001). Each analysis includes 3 independent replicates.
miR1432 acts as an essential regulator of rice drought tolerance
To explore the effect of miR1432 on rice drought tolerance, transgenic miR1432 overexpression lines (OEmiR1432-1 and OEmiR1432-2) and short tandem target mimic lines (STTM1432-1 and STTM1432-2) were generated, which should increase and suppress miR1432 levels, respectively. In addition, CRISPR lines (mir1432-1 and mir1432-2) with mutations adjacent to or in the region of mature miR1432 and miR1432 star, which should affect the biogenesis of miR1432, were obtained (Supplementary Fig. S2A). Northern blot analysis revealed that, compared with wild type (WT), miR1432 expression was significantly inhibited or absent in STTM1432 transgenic lines and mir1432 mutants (Supplementary Fig. S2, B and C), while miR1432 accumulated to higher levels in OEmiR1432 transgenic plants (Supplementary Fig. S2D). These transgenic lines with expected changes in miR1432 expression were selected to evaluate their responses to drought stress. Treatment with 150 mM d-mannitol significantly inhibited seed germination in mir1432 and STTM1432 transgenic lines, while OEmiR1432 showed no obvious changes compared with WT (Fig. 2A; Supplementary Fig. S3A and Table S1). Similarly, under PEG treatment and water-deficiency conditions, mir1432 and STTM1432 lines showed decreased tolerance to drought stress, while OEmiR1432 plants resembled WT (Fig. 2B; Supplementary Figs. S3B and S4 and Table S2). The dehydration rate of mir1432 and STTM1432 plants treated with PEG was significantly higher than that of WT within 2 to 3 h of treatment (Fig. 2C; Supplementary Fig. S3C). Furthermore, the malondialdehyde (MDA) content increased to a much higher level in mir1432 and STTM1432 plants than in WT, reflecting the more severe cellular oxidative damage in mir1432 and STTM1432 transgenic plants caused by drought stress (Fig. 2D; Supplementary Fig. S3D). Free proline is an important osmoregulatory substance in plants, and its elevation after drought stress effectively attenuates stress damage (Singh et al. 1972). As shown in Fig. 2E and Supplementary Fig. S3E, proline content increased during drought stress, but to lower levels in mir1432 and STTM1432 plants than in WT. Further analysis by RT-qPCR showed that drought stress-responsive genes, including rice BASIC LEUCINE ZIPPER46 (OsbZIP46; Tang et al. 2016), MADS-BOX26 (OsMADS26; Khong et al. 2015), GLOSSY1-2 (OsGL1-2; Islam et al. 2009), and WRKY TRANSCRIPTION FACTOR30 (OsWRKY30; Shen et al. 2012), also underwent significant changes in mir1432 and STTM1432 plants after drought stress, suggesting that suppression of miR1432 in rice altered the expression pattern of such genes (Supplementary Fig. S5, A to D). Taken together, our results show that miR1432 acts as an essential regulator of drought tolerance, and suppression of miR1432 expression significantly impairs rice drought tolerance.
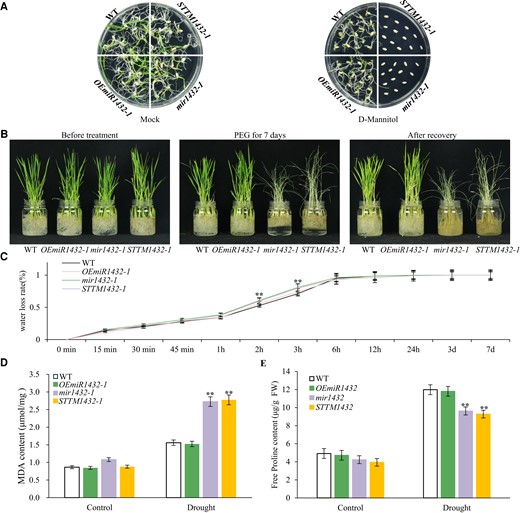
Effect of miR1432 on drought tolerance in rice. A) Seed germination phenotype of OEmiR1432-1, mir1432-1, and STTM1432-1 transgenic lines grown on 1/2 MS supplemented with 150 mM d-mannitol. Mock is the untreated control. B) Drought stress phenotype of 1-month-old OEmiR1432-1, mir1432-1, and STTM1432-1 lines subjected to 20% (w/v) PEG 8000 treatment for 7 d. C) Determination of water loss rate of leaves of OEmiR1432-1, mir1432-1, and STTM1432-1 lines. D) MDA content in OEmiR1432-1, mir1432-1, and STTM1432-1 lines following PEG treatment. E) Proline content in OEmiR1432-1, mir1432-1, and STTM1432-1 lines following PEG treatment. The data in C to E) are presented as means ± Sd. Asterisks indicate a significant difference compared to WT (2-way ANOVA: **P < 0.01 in C to E). At least 3 independent biological replicates were performed with similar results.
We also evaluated the effects of miR1432 on the gross morphology of rice. The grain size of mir1432 and STTM1432 transgenic lines was significantly improved, which is consistent with previous studies (Supplementary Fig. S6, A to D, and Table S3; Zhao et al. 2019; Li et al. 2021). No obvious effects on other agronomical traits were observed in the mir1432, STTM1432, and OEmiR1432 transgenic lines (Supplementary Fig. S6E), however, indicating that the regulation of drought resistance by miR1432 is not primarily due to an effect on rice morphology.
miR1432 targets the calmodulin-like gene OsCaML2 for transcript cleavage
Plant miRNAs function by suppressing target genes by posttranscriptional mRNA cleavage or translational inhibition. Therefore, identifying the target genes of miRNAs is of great importance for understanding their biological roles. To explore the molecular mechanism of how miR1432 regulates rice drought tolerance, we predicted its potential targets using psRNATarget (https://www.zhaolab.org/psRNATarget/) and TarHunter (http://www.biosequencing.cn/TarHunter/; Dai et al. 2018; Ma et al. 2018), revealing a total of 16 candidate targets that encoded members of different protein families (Supplementary Data Set 1). The expression pattern of miRNAs is usually negatively associated with their target genes. Spatial temporal expression analysis showed that the target gene OsCaML2 (Os03g0812400/LOC_Os03g59770) was expressed at a relatively high level in inflorescences while at a lower level in young roots and mature leaves, which is the opposite of the expression profile of pri-miR1432 (Supplementary Figs. S1J and S7). In addition, in contrast to the induced expression pattern of miR1432, transcript levels of OsCaML2 decreased dramatically in response to drought stress (Fig. 3A). More importantly, RT-qPCR results showed that the accumulation of OsCaML2 was elevated in STTM1432 and mir1432 plants, but decreased in OEmiR1432 strains (Fig. 3B), indicating a converse expression pattern between miR1432 and OsCaML2, consistent with a recent report (Jia et al. 2021). Therefore, we focused on OsCaML2 to further study its function in drought resistance regulation.
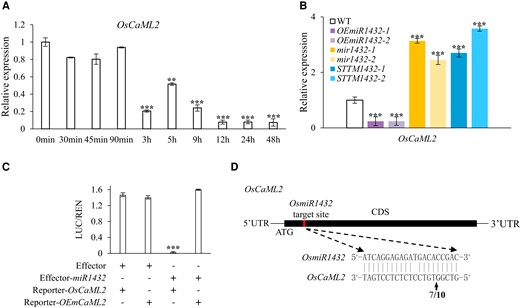
Identification of the miR1432 target gene OsCaML2 in rice. A) RT-qPCR analysis of the expression level of OsCaML2 in WT rice during 150 mM d-mannitol treatment. UBQ5 was used as an internal control. B) Relative expression level of OsCaML2 in OEmiR1432, mir1432, and STTM1432 lines determined by RT-qPCR. UBQ5 was used as an internal control. C) Relative LUC reporter activity in rice protoplasts transiently transformed with OsCaML2/OEmCaML2 with or without the presence of miR1432. Renilla was used as a control for normalization. Relative luciferase activity (LUC/REN) was determined. D)OsCaML2 transcript cleavage site detected by RLM-RACE. Arrow indicates the cleavage site. Numbers indicate the frequency of detected clones matching this site out of the total sequenced clones. In A to C), the data are presented as means ± Sd, and asterisks indicate a significant difference compared to WT or 0 h (Student's t test: **P < 0.01 and ***P < 0.001). Three biological replicates were performed for each experiment.
To determine whether miR1432 can regulate the expression of OsCaML2, we performed a transient coexpression assay in rice protoplasts using the dual-luciferase reporter system. OsCaML2 and OEmCaML2 (which contains 15 mutations in the miR1432 recognition site of OsCaML2 without altering the encoded amino acids) (Supplementary Fig. S8A) were fused with the LUC reporter gene and transformed into rice protoplasts with or without the presence of miR1432. The results showed that the expression of miR1432 suppressed the accumulation of OsCaML2-LUC, but no suppression effect was observed for the OsmCaML2-LUC control, confirming the requirement for the interaction between miR1432 and OsCaML2 (Fig. 3C; Supplementary Fig. S8B). In addition, RNA linker-mediated rapid cDNA end amplification (RLM-RACE) analysis showed that miR1432 could directly cleave OsCaML2 transcripts at a specific site within the miR1432 paring region (Fig. 3D). Together, these data demonstrate that OsCaML2 is a target gene whose transcript is directly cleaved by miR1432 in rice.
Suppression of OsCaML2 by miR1432 contributes to rice drought tolerance
To further investigate whether the cleavage of OsCaML2 RNA mediated by miR1432 affects drought tolerance in rice, we generated transgenic lines overexpressing a miR1432-resistant noncleavable version of OsCaML2 (designated OEmCaML2) under the control of the UBIQUITIN (UBI) promoter. The synonymous mutations introduced into the target site of OEmCaML2 were identical to those used in the dual-luciferase reporter assay (Supplementary Fig. S8A). RT-qPCR analysis confirmed that OEmCaML2-positive transgenic lines overexpressed OsCaML2 (Supplementary Fig. S9A). Next, we subjected the OEmCaML2 transgenic lines to drought stress treatment. As expected, OEmCaML2 exhibited significantly decreased drought resistance compared with WT, resembling the phenotype of mir1432 and STTM1432 transgenic lines under drought conditions (Fig. 4A; Supplementary Fig. S9B and Table S4). Further determination of physiological indexes showed that the MDA content of OEmCaML2-1 transgenic plants was significantly higher than that of WT (Fig. 4B; Supplementary Fig. S9C), while the elevation of free proline was lower in OEmCaML2-1 transgenic plants compared with WT under drought stress conditions (Fig. 4C; Supplementary Fig. S9D). In addition, the expression of drought stress-related genes OsbZIP46, OsMADS26, OsGL1-2, and OsWRKY30 showed changes in OEmCaML2 lines similar to those observed in mir1432 and STTM1432 lines in response to drought stress (Fig. 4, D to G; Supplementary Fig. S9, E to H). Collectively, these results strongly suggest that OsCaML2 is the main target gene acting genetically downstream of miR1432 to regulate rice drought resistance. We also generated caml2 mutants by CRISPR genome editing (Supplementary Fig. S9I), and RT-qPCR results showed that OsCaML2 transcripts were almost completely absent in the caml2 mutants (Supplementary Fig. S9A). However, caml2 mutants showed similar drought tolerance to WT controls (Fig. 4, A to G; Supplementary Fig. S9B), probably because of the functional redundancy of homologous OsCaML2 genes in rice: one example is OsEFH1, whose sequence is very similar to that of OsCaML2 (Jia et al. 2021). These results demonstrate that miR1432 regulates drought tolerance by suppressing the expression of target gene OsCaML2 in rice.
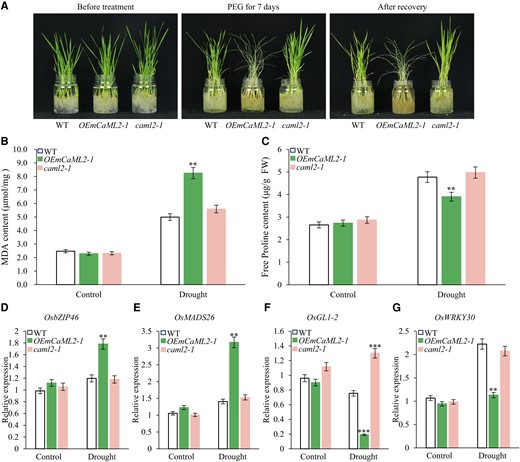
OsCaML2 is involved in the regulation of rice drought stress tolerance. A) One-month-old seedlings of WT, OEmCaML2-1, and caml2-1 plants were subjected to drought stress for 7 d by treatment with 20% (w/v) PEG 8000. B) MDA content in WT, OEmCaML2-1, and caml2-1 seedlings during drought treatment. C) Free proline content in WT, OEmCaML2-1, and caml2-1 seedlings following drought treatment. D to G) RT-qPCR analysis of drought stress-related genes, including OsbZIP46D), OsMADS26E), OsGL1-2F), and OsWRKY30G) in WT plants and OEmCaML2-1 and caml2-1 lines during 150 mM d-mannitol treatment. UBQ5 was used as an internal control. The data in B to G) are presented as means ± Sd, and the asterisks indicate a significant difference compared to WT (2-way ANOVA: **P < 0.01 and ***P < 0.001). At least 3 biological replicates were performed for each experiment.
The miR1432-OsCaML2 module regulates rice drought tolerance via MAPK and hormone signaling pathways
To clarify the role of miR1432 in the regulation of drought stress in rice, we took advantage of RNA sequencing to identify differentially expressed genes (DEGs) in STTM1432 under drought stress conditions and investigated the regulatory gene network downstream of miR1432. The transcriptomes of seedling tissues of STTM1432-1 and WT plants subjected to drought stress were compared. About 25 million uniquely mapped reads per sample were obtained (Supplementary Data Set 2), and the 3 biological replicates showed high correlation coefficients (Supplementary Data Set 3). Data analysis revealed a large number of DEGs in response to drought stress. Approximately 1,540 and 1,504 genes showed significant changes in expression in STTM1432-1 and WT plants under drought conditions, respectively (Supplementary Data Sets 4 and 5). Among these, 251 downregulated and 752 upregulated genes were found exclusively in STTM1432-1, indicating their contribution to the distinct drought-sensitive phenotype of STTM1432-1 plants (Fig. 5, A and B; Supplementary Data Sets 6 and 7). Interestingly, 11 genes were found to be upregulated in STTM1432-1 and downregulated in WT (Supplementary Fig. S10A). Gene Ontology (GO) analysis of the 11 DEGs showed that they were mainly associated with stress responses, suggesting their essential roles in rice drought tolerance (Supplementary Fig. S10B and Data Set 8). GO analysis of DEGs in STTM1432-1 and WT revealed that they were significantly enriched in responses to stimuli, in glutathione metabolism, and in oxidation–reduction processes, which are essential to plant stress responses (Supplementary Fig. S10, C and D, and Data Sets 9 and 10). More importantly, DEGs specific to STTM1432-1 were found to be primarily involved in lipid localization and transport, defense responses, and glutathione metabolism (Fig. 5C; Supplementary Data Set 11). KEGG enrichment analysis showed that the miR1432-specific DEGs were mostly involved in the mitogen-activated protein kinase (MAPK) signaling pathway, plant hormone signal transduction, and glutathione metabolism (Supplementary Fig. S10E and Data Set 12). These observations were consistent with previous findings that these pathways play key roles in regulating plant abiotic stress responses, including drought stress responses (Hwa and Yang 2008; Kong et al. 2011; Zhang et al. 2012). According to DEG analysis, genes related to MAPK signaling and hormone signal transduction were significantly affected under stress conditions and accumulated to a higher level in STTM1432-1 than in WT plants, implying an essential role of miR1432 in altering the expression of these genes (Fig. 5, D and E). These DEGs included TYPE2C PROTEIN PHOSPHATASE (PP2C) genes, SMALL AUXIN UP-REGULATED RNA (SAUR) genes, JASMONATE ZIM-DOMAIN PROTEIN8 (JAZ8), and ANNEXIN1 (ANN1) genes (Fig. 5, D and F). The differential expression patterns of representative genes were further verified by RT-qPCR, and the results showed similar changes to those observed in the RNA-seq analysis (Fig. 5F). We also examined the expression levels of these genes in OEmCaML2-1 plants, where they exhibited expression patterns in response to drought stress that were similar to those observed in STTM1432-1 transgenic lines (Fig. 5F). Taken together, these results indicate that miR1432 is a critical regulatory factor that affects MAPK and hormone signaling pathways, which are both involved in the regulation of drought stress responses in rice.
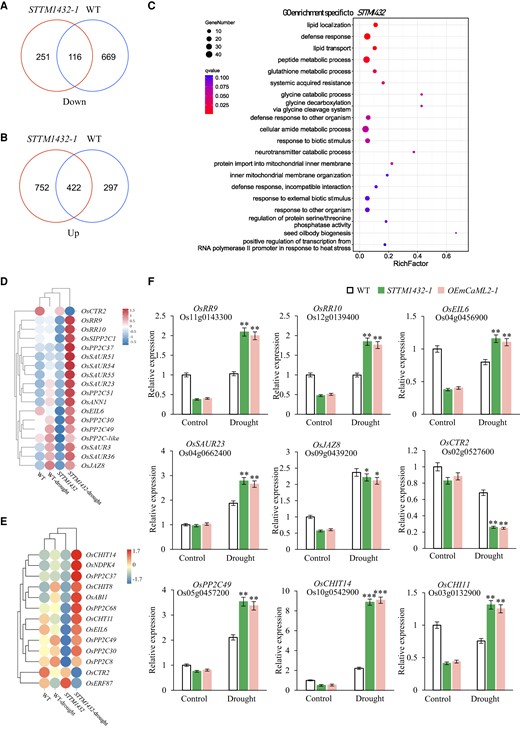
Transcriptome analyses reveal the downstream pathways regulated by miR1432 in rice. A and B) Venn diagram analyses of downregulated A) and upregulated B) DEGs in the seedling tissues of STTM1432-1 and WT plants subjected to drought stress. C) GO enrichment analysis of STTM1432-specific DEGs in response to 150 mM d-mannitol treatment. The top 20 GO terms are shown. D) Heat map representing the transcriptional changes of hormone signal transduction pathway genes in response to 150 mM d-mannitol treatment in STTM1432-1 seedlings. E) Heat map representing the transcriptional changes of MAPK signal pathway genes in response to 150 mM d-mannitol treatment in STTM1432-1 seedlings. F) Expression levels of genes related to MAPK signaling pathway and hormone signal transduction pathway in WT, STTM1432-1, and OEmCaML2-1 plants shown by RT-qPCR. UBQ5 was used as an internal control. The data are presented as means ± Sd. Asterisks indicate a significant difference compared to WT or 0 h (2-way ANOVA: *P < 0.05, **P < 0.01 and ***P < 0.001). Three biological replicates of the experiment were performed with similar results.
Discussion
miRNAs are extensively involved in plant responses to biotic and abiotic stresses and play essential regulatory roles in conferring stress tolerance on a variety of model plants and crop species (Song et al. 2019). miR1432 has been reported to regulate rice grain size and weight: it controls the grain filling rate by targeting OsACOT, which is involved in the biosynthesis of chain fatty acids (Zhao et al. 2019). Furthermore, miR1432 can also participate in pathogen defense and contributes to rice blast disease and bacterial blight resistance (Jia et al. 2021; Li et al. 2021). In this study, we demonstrated that miR1432 can act as an essential regulator of drought tolerance by suppressing the expression of target gene OsCaML2, indicating a critical role for the miR1432-OsCaML2 module in regulating rice drought resistance.
In order to explore the function of miR1432 in regulating rice drought tolerance, we generated miR1432 overexpression, suppression, and CRISPR lines. Following drought stress treatment, the STTM1432 and mir1432 lines exhibited compromised drought resistance, while OEmiR1432 plants showed no significant changes compared with WT (Fig. 2; Supplementary Figs. S3 and S4). The latter finding might be explained if endogenous miR1432 expression was already at a very high level as a result of the drought stress treatment, such that overexpression would have little effect on the plants. It is worth noting that the relationship between gene expression levels and phenotypic outcomes might involve intricate regulatory mechanisms, and not all genes exhibit a linear relationship between their expression levels and phenotypic effects. Certain genes may necessitate precise expression level or reach a threshold to exert certain functions, and overexpression beyond that level may not lead to additional phenotypic changes. Based on our observation of decreased tolerance in mir1432 and STTM1432 transgenic lines, we concluded that miR1432 is a crucial regulator of the drought stress response.
We identified the calmodulin-like gene OsCaML2 as a direct target of miR1432 by RLM-RACE and dual-luciferase reporter assay in rice protoplasts (Fig. 3, C and D). Under drought stress, OsCaML2 exhibits decreased expression levels, contrasting with the elevated expression pattern of miR1432 (Figs. 1, A and B, and 3A). In addition, compared with WT plants, transgenic lines overexpressing miR1432-resistant OsCaML2 (OEmCaML2) showed increased sensitivity to drought stress (Fig. 4A; Supplementary Fig. S9B), which resembles the phenotype of mir1432 and STTM1432 lines (Fig. 2B; Supplementary Figs. S3 and S4), further demonstrating the critical role of the miR1432-OsCaML2 module in regulating drought resistance. Previously, OsCaML2 was reported to be targeted by miR1432 using a transient heterogeneous GFP-based reporter system in Nicotiana benthamiana, and it was also shown to contribute to rice bacterial blight resistance (Jia et al. 2021). Here, we confirmed the interaction between miR1432 and OsCaML2 by various experimental approaches and revealed a role of the miR1432-OsCaML2 module in regulating rice drought tolerance. In addition to OsCaML2, previous studies have identified OsACOT and OsEFH1 as target genes of miR1432 in rice (Zhao et al. 2019; Li et al. 2021). To investigate their potential involvement in miR1432-mediated drought resistance, we examined their expression patterns under drought treatment by RT-qPCR. However, their expression profiles did not show a negative association with the induced expression of miR1432 in response to drought stress (Fig. 1, A and B; Supplementary Fig. S11), indicating that these 2 genes may not be involved in the miR1432-mediated response to drought stress. Transcriptome analyses showed that the suppression of miR1432 specifically affects the expression of genes involved in the MAPK and hormone signaling pathways in rice under drought stress (Fig. 5, D and E). The MAPK cascade is a major signal transducer and plays an essential role in regulating plant drought stress responses via crosstalk with hormone signaling pathways (Lin et al. 2021). To date, although the downstream components of the MAPK cascade responsible for drought stress have been identified, the upstream regulators of the MAPK pathway related to drought stress remain largely unknown. Our study indicates that miR1432 may serve as an upstream regulator of the MAPK cascade by suppressing the expression of OsCaML2, thus determining the fate of plants subjected to drought stress.
OsCaML2 is a calmodulin-like gene encoding an EF-hand chiral calcium-binding protein. So far, a large number of CML genes have been identified, including 50 family members in Arabidopsis (Arabidopsis thaliana) and 32 members in rice (McCormack and Braam 2003; McCormack et al. 2005; Boonburapong and Buaboocha 2007). Previous studies have demonstrated that CMLs can function as intracellular receptors that bind calcium ions and play important roles in regulating plant tolerance to multiple abiotic stresses, including drought stress (Bender et al. 2013; Yin et al. 2015; Zeng et al. 2015). For instance, Arabidopsis AtCML20 has been identified as a negative regulator of drought tolerance: the cml20 mutant is more sensitive to ABA-regulated stomatal movement and shows improved tolerance to drought stress (Wu et al. 2017). In rice, OsCML4 positively regulates drought tolerance, and overexpression of OsCML4 enhances rice tolerance to drought due to improved scavenging of reactive oxygen species (Yin et al. 2015). The transcription factor encoded by OsERF48 can directly bind to the promoter of OsCML16 and activate its transcription, thus affecting the expression of downstream drought-related genes and enhancing root growth and drought tolerance (Jung et al. 2017). In addition, overexpression of OsMSR2 (OsCML24) in Arabidopsis enhances resistance to salt and drought stresses via ABA-mediated pathways (Xu et al. 2011). These studies show that CMLs are multifunctional proteins and may employ different mechanisms in regulating plant drought tolerance. CaMs/CMLs are not enzymes; instead, they interact with downstream target proteins called calmodulin-binding proteins (CBPs), thereby modulating their activities and transducing Ca2+ signals (Zeng et al. 2015). To date, a variety of CaM/CML targets have been identified, including transcription factors, protein kinases, protein phosphatases, ion channels and pumps, metabolic enzymes, and cytoskeletal proteins (Perochon et al. 2011; Cheval et al. 2013; Zeng et al. 2015). In this study, we revealed that OsCaML2 is suppressed by miR1432 and negatively regulates rice drought tolerance by affecting downstream MAPK and hormone signaling pathways; however, the direct targets of OsCaML2 remain unknown. Hence, more emphasis needs to be placed on investigating OsCaML2-interacting proteins, which is crucial for clarifying how OsCaML2 exerts its role in regulating rice drought tolerance at the molecular level.
Among the DEGs specifically to STTM1432 transgenic plants, several PP2C genes were found to be upregulated (Fig. 5, D to F). It has been well demonstrated that PP2Cs negatively regulate MAPK signaling by catalyzing the dephosphorylation of MAPKs, leading to their inactivation (Schweighofer et al. 2007; Fuchs et al. 2013; He et al. 2019; Chuong et al. 2021). Besides, PP2Cs have been found to act as negative regulators of ABA signaling by dephosphorylating and inhibiting SNF1-related protein kinase 2 (SnRK2) and its downstream targets (Hsu et al. 2021; Waadt et al. 2022). Thus, the elevated expression levels of PP2C genes indicate suppression of MAPK and ABA signaling in STTM1432 under drought stress, leading to impaired drought tolerance in STTM1432. In addition, SAUR genes that function in early auxin responses and JAZ8 and ANN1 genes involved in repression of JA signaling were also found to be altered in STTM1432 (Fig. 5, D and F). The changes of gene expression in ABA, auxin, and JA signaling pathways are consistent with previous findings that these hormones play essential roles in plant drought stress responses (Hang et al. 2021; Hsu et al. 2021; Waadt et al. 2022). Moreover, several other DEGs that are specific to STTM1432 also attracted our attention, including PLASMA MEMBRANE INTRINSINC PROTEIN2 (OsPIP2; Lu et al. 2018), which encodes a plasma membrane intrinsic protein that maintains intracellular osmotic balance, and NICOTINAMIDE ADENINE DINUCLEOTIDE PHOSPHATE-MALIC ENZYME3 (OsNADP-ME3; Cheng and Long 2007), which encodes cytoplasmic malic enzyme and has an important regulatory role in response to abiotic stress. OsPR1a (Kothari et al. 2016), which codes for pathogenesis-related 1a protein and is involved in the regulation of abiotic stress responses, was also identified. The expression patterns of these 3 DEGs were further verified by RT-qPCR in STTM1432-1 and OEmCaML2-1 under drought stress conditions (Supplementary Fig. S12). In addition to the genes involved in MAPK and hormone signaling pathways, these 3 genes might also contribute to the miR1432-mediated regulation of drought stress resistance in rice.
In summary, our work suggests a potential signaling pathway mediated by the miR1432-OsCaML2 module that is important in rice drought tolerance (Fig. 6). Under normal conditions, the expression of miR1432 and OsCaML2 in cells is maintained at appropriate levels. However, during drought stress, the expression of miR1432 is substantially increased, thereby suppressing the target gene OsCaML2, which affects the expression of downstream genes involved in MAPK, and hormone signaling and modulates rice drought tolerance (Fig. 6). This study reveals the critical role of miR1432-OsCaML2 module in drought tolerance and expands our understanding of miRNA-mediated complex regulatory networks in plant responses to environmental stress.
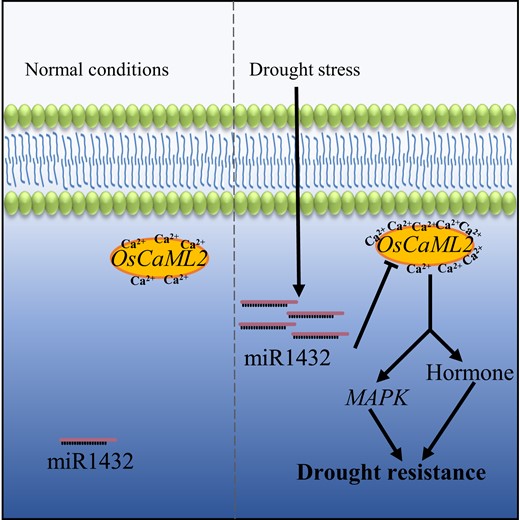
A proposed model of how the miR1432-OsCaML2 regulatory module controls drought tolerance in rice. Under normal conditions, the expression of miR1432 and OsCaML2 in cells is maintained at basal levels. Once challenged with drought stress, miR1432 is markedly induced to suppress the expression of OsCaML2, which regulates the downstream MAPK and hormone transduction pathways and modifies rice drought tolerance. Arrows, positive regulation; blunt arrow, negative regulation.
Materials and methods
Plant materials and growth conditions
WT rice (Oryza sativa L.) or transgenic lines created in the Nipponbare (ssp. japonica) background were propagated in an experimental field in Shenzhen, China (22°N, 113°E), throughout the year. Rice seedlings were grown at day and night temperatures of 32 and 28 °C ± 1 °C, respectively, with a relative humidity of 50% to 60%, and light and dark periods of 16 and 8 h, respectively, before transplantation to paddy fields or treatment. Seeds used for germination experiments were sterilized and then sowed in a growth chamber in 1/2 MS medium with 150 mM d-mannitol. After 7 d, germination status was noted, and the germination rate was statistically analyzed. For RNA-seq library construction, 10-d-old seedlings treated with 150 mM d-mannitol for 48 h were used. For the water-deficit experiment, 1-mo-old rice plants were used. After treatment for 14 d, plants were rewatered, and the survival rate was recorded on the 7th day after water supplementation. The seed setting rate was analyzed after rice maturation. At least 3 independent biological replicates were performed for each experiment.
Vector construction and rice transformation
The region 2,500 bp upstream of pre-miR1432 was cloned from Nipponbare cDNA and inserted in front of the GUS (Hackenberg et al. 2015) reporter gene in pCAMBIA1301 using EcoRI and XbaI to construct the MIR1432p::GUS vector. The STTM vector for miR1432 was constructed as described (Teotia and Tang 2017). sgRNA sequences specific to pre-miR1432 or OsCaML2 were introduced into pCAMBIA1390-OsNLSCas9 downstream of the OsU6 promoter using HindIII and BamHI restriction enzymes to construct the genome editing vector. miR1432 and OsCaML2 sequence information was acquired from miRBase (http://www.mirbase.org/) and RiceData (https://www.ricedata.cn), respectively. The fragment of pre-miR1432 was amplified from the Nipponbare cDNA and inserted downstream of the OsUBI promoter in pCAMBIA1390 using HindIII and BamHI. The target gene OsCaML2 was cloned with 15 mutated nucleotides at the miR1432-targeted site, but without any amino acid changes, and was inserted into pCXUN-FLAG by XcmI digestion, under the control of the OsUBI promoter. All constructs were transformed into Agrobacterium tumefaciens strain EHA105 and then transformed into rice callus by Agrobacterium-mediated transformation (Hiei et al. 1997). The primers used for construction are listed in Supplementary Data Set 13.
Measurements of agronomic traits
After the rice was fully mature, the agronomic traits plant height, effective tiller, flag leaf length, flag leaf width, and spike length were measured in the field. The hundred-grain weight, as well as the length and width of grains, were measured after the collected seeds were dried in a 37 °C oven until the weight remained unchanged. All experiments were carried out with at least 3 replicates.
Measurements of the water loss rate of leaves
The water loss rate of leaves was measured under conditions of constant temperature and humidity. An electronic balance was used to weigh the same area of the leaves for the same period regularly until their weight stabilized. The water loss rate was calculated as a percentage of the reduced weight relative to the initial weight of the blades. Each experiment was performed with at least 3 replicates.
Determination of MDA content
Fresh samples of rice seedlings (0.5 g) were homogenized in 5 mL 5% (w/v) TCA solution. After centrifugation (12,000 × g for 5 min, 4 °C), 2 mL supernatant was taken and 2 mL 0.6% (w/v) thiobarbituric acid (TBA) was added. The mixture was bathed in boiling water for 15 min, allowed to cool, and then centrifuged at 5,000 × g for 10 min. The supernatant was taken to measure the absorbance value at 450, 532, and 600 nm. The MDA concentration was calculated according to the following formula:
where OD532, OD600, and OD450 represent the absorbance value at 532, 600, and 450 nm respectively; V (mL) is the total reaction volume; A is the dilution ratio at the final determination of the sample; and W (mg/mL) is the protein content in the supernatant of the homogenate. All experiments were carried out with at least 3 replicates.
Determination of proline content
Fresh samples of rice seedlings (0.5 g) were homogenized in 5 mL 3% (w/v) sulfosalicylic acid and filtered with filter paper. After addition of an equal volume of acid ninhydrin and glacial acetic acid, the mixture was bathed in boiling water for 30 min. The reaction was then stopped by immersion in an ice bath. The mixture was extracted with 4 mL toluene and centrifuged at 5,000 × g for 5 min, and the supernatant was recovered. The absorbance value at 520 nm was measured with toluene as the control. Proline concentration was determined using a calibration curve and expressed as microgram proline per gram fresh weight. Each experiment was performed with at least 3 replicates.
Measurement of GUS activity
The callus, seedling, seed, leaf sheath, internodes, and inflorescence of MIR1432p::GUS transgenic rice were collected and incubated in dye buffer at 37 °C for more than 3 h. GUS activity was determined by histochemical staining as described by Jefferson et al. (1987). Each experiment was performed with at least 3 replicates.
RNA extraction and RT-qPCR
Total RNA was extracted from rice tissues with TRIzol Reagent (Invitrogen 15596026) following the manufacturer’s instructions. cDNA used for RT-qPCR analysis was synthesized using HiScript III 1st Strand cDNA Synthesis Kit with gDNA wiper (Vazyme R312-01). Pri-miR1432 expression levels were quantified by RT-qPCR. Reverse transcripts were used as templates, and qPCR was performed on a QuantStudio 6 Flex real-time PCR system (ABI) using AceQ qPCR SYBR Green Master Mix (Vazyme Q111-03). The rice UBQ5 gene was used as an internal reference, and its relative expression was determined by 2−ΔΔCt analysis. All reactions were performed with at least 3 replicates. Primers used for RT-qPCR are listed in Supplementary Data Set 13.
Small RNA northern hybridization
Approximately 20 μg total RNA was separated on 15% polyacrylamide denaturing gels, transferred to Hybond-NX membrane (GE Healthcare, Amersham, United Kingdom) and hybridized with biotinylated probes that were complementary to the target gene sequences. Finally, developing substrate was added, and an FLA-5000 phosphorimager (Fuji Medical Systems Inc., Stamford, CT) was used for imaging. U6 was used as an internal control. Each experiment was performed with at least 3 replicates. The probe sequences are listed in Supplementary Data Set 13.
RNA-seq
Ten-day-old seedlings of WT and STTM1432 transgenic lines treated with 150 mM d-mannitol for 48 h were collected for RNA isolation. RNA was extracted from seedlings using RNeasy Plant Mini-Kit (Qiagen). Libraries were constructed using a Next Multiplex Library Prep Set for Illumina kit (NEB) and then sequenced on the Illumina HiSeq 2000 platform. Each experiment was performed with at least 3 replicates.
Rice genome information for Nipponbare was acquired from the Ensembl Plant database (https://ftp.ensemblgenomes.ebi.ac.uk/pub/plants/release-58/fasta/oryza_sativa/dna/). DESeq2 analysis was performed to determine differential expression. The RNA-seq data were analyzed with TopHat and Cufflinks software. Genes with at least a 1.5-fold change in the drought stress samples compared with untreated controls were considered to be significantly up- or downregulated. Results were imported into Microsoft Excel for filtering (log2 ≥ 1.5 cutoff).
Analysis of GO and KEGG of DEGs
Genes that were up- or downregulated at least 1.5-fold in WT and STTM1432 samples subjected to drought stress were analyzed for GO term enrichment. We used BiNGO to analyze the item classification of DEGs (Maere et al. 2005) and calculated the P-value of each GO item classification using a hypergeometric test that was further checked as described (Benjamini and Hochberg 1995). GO item classification involved at least 5 genes, and we considered a checked P < 0.05 to be significantly enriched. KEGG pathway enrichment analysis was executed by using DAVID (https://david.ncifcrf.gov/) for the selected DEGs (Kanehisa and Goto 2000). A P < 0.05 was set as the cutoff criterion and regarded as statistically significant (Huang da et al. 2009).
Prediction of miR1432 targets
The psRNATarget program (https://www.zhaolab.org/psRNATarget/) and the TarHunter website (http://www.biosequencing.cn/TarHunter/osa.html) were used to predict potential targets of miR1432, and the O. sativa TIGR genome cDNA library (OSA1R5) was used as the reference when searching for targets.
Dual-luciferase reporter assay
To validate the interaction between miR1432 and the target gene OsCaML2 in vivo, we used the rice protoplast system for dual-luciferase reporter transient expression assay (Zhang et al. 2011). The miR1432 precursor and control sequences driven by the 35S promoter were constructed in the pGreen II 62-SK vector, and the predicted target gene, OsCaML2, driven by the 35S promoter was inserted into the pGreen II 0800-LUC vector. These 2 vectors were introduced into rice protoplasts using the PEG-mediated method (He et al. 2016). If there were an interaction between miR1432 and OsCaML2, the transcription level of OsCaML2 would be reduced compared to the putative target fused to the control vector. Changes in the ratio of firefly to Renilla luciferase activity (Fluc/Rluc) would show such differences. Each experiment was performed with at least 3 replicates.
RLM-RACE
Total RNA of WT rice was extracted and ligated to a synthesized RNA adaptor, which has a 5′-hydroxyl group at both ends and thus can only ligate to 5′-phosphorylated RNA, including the truncated products of miRNA-guided mRNA cleavage. The ligated product was directly reverse transcribed with an oligonucleotide primer (Lin et al. 2011), and the cDNA was amplified by nested PCR. The products from the 2nd round of PCR were purified by agarose gel electrophoresis, and the amplicons were cloned into the pMD19-T vector (Takara). Clones with the expected inserts were sequenced. Each experiment was performed with at least 3 replicates. Adaptor and primer sequences are shown in Supplementary Data Set 13.
Primers and probes
All primers used for RT-qPCR and probes used for northern blotting are listed in Supplementary Data Set 13.
Accession numbers
Sequence data from this article can be found in the GenBank/EMBL (https://www.ricedata.cn) data libraries under accession number Os03g0812400 (OsCaML2).
Acknowledgments
We thank the Instrumental Analysis Center of Shenzhen University for technical assistance.
Author contributions
X.C., L.Liu., and B.M. conceived the research. G.L., X.Y., and L.Li. performed the experiments. G.L., Y.Y., and L.G. analyzed the data. G.L., L.Liu., and X.C. wrote the manuscript. All authors read and approved the final manuscript.
Supplementary data
The following materials are available in the online version of this article.
Supplementary Figure S1. Spatiotemporal expression pattern of MIR1432 in different rice tissues.
Supplementary Figure S2. miR1432 expression in mir1432, STTM1432, and OEmiR1432 transgenic lines.
Supplementary Figure S3. Phenotype and physiological analysis of OEmiR1432, mir1432, and STTM1432 transgenic lines under drought stress.
Supplementary Figure S4. Phenotype of OEmiR1432, mir1432, and STTM1432 transgenic plants under water-deficit conditions.
Supplementary Figure S5. Determination of the expression levels of drought stress-related genes by RT-qPCR.
Supplementary Figure S6. Phenotype of other agronomic traits of OEmiR1432, STTM1432, and mir1432.
Supplementary Figure S7. Spatiotemporal expression pattern of OsCaML2 in different rice tissues determined by RT-qPCR.
Supplementary Figure S8. Illustration of mutated nucleotides in miR1432-resistant OEmCaML2 and diagram of the dual-luciferase reporter vector.
Supplementary Figure S9. Phenotype and physiological analysis of OEmCaML2 and caml2 plants under water-deficit conditions.
Supplementary Figure S10. Analyses of DEGs in STTM1432 and WT plants subjected to drought stress.
Supplementary Figure S11. Determination of the expression levels of OsACOT and OsEFH1 by RT-qPCR.
Supplementary Figure S12. Determination of the expression levels of OsPIP2, OsPR1a, and OsNADP-ME3 by RT-qPCR.
Supplementary Table S1. The germination rate of OEmiR1432, mir1432, and STTM1432 transgenic rice under 150 mM d-mannitol treatment.
Supplementary Table S2. The survival rate of OEmiR1432, mir1432, and STTM1432 transgenic lines under 150 mM d-mannitol treatment.
Supplementary Table S3. Measurements of grain length, grain width, and grain weight of OEmiR1432, mir1432, and STTM1432.
Supplementary Table S4. The survival rate of OEmCaML2 and caml2 mutant under 150 mM d-mannitol treatment.
Supplementary Data Set 1. Summary of predicted miR1432 target genes.
Supplementary Data Set 2. The mapping information of RNA libraries.
Supplementary Data Set 3. Correlation coefficients of mRNA-seq biological replicates.
Supplementary Data Set 4. List of DEGs in STTM1432 under drought stress (false discovery rate [FDR] < 0.05, fold change > 1.5).
Supplementary Data Set 5. List of DEGs in WT under drought stress (FDR < 0.05, fold change > 1.5).
Supplementary Data Set 6. List of differentially downregulated expressed genes under drought stress exclusively in STTM1432 (FDR < 0.05, fold change > 1.5).
Supplementary Data Set 7. List of differentially upregulated expressed genes under drought stress exclusively in STTM1432 (FDR < 0.05, fold change > 1.5).
Supplementary Data Set 8. List of the 11 DEGs upregulated in STTM1432 and downregulated in WT under drought stress (FDR < 0.05, fold change > 1.5).
Supplementary Data Set 9. List of GO enrichment in STTM1432 under drought stress (FDR < 0.05, fold change > 1.5).
Supplementary Data Set 10. List of GO enrichment in WT under drought stress (FDR < 0.05, fold change > 1.5).
Supplementary Data Set 11. List of GO enrichment of DEGs specific to STTM1432 under drought stress (FDR < 0.05, fold change > 1.5).
Supplementary Data Set 12. KEGG enrichment of DEGs specific to STTM1432 under drought stress (FDR < 0.05, fold change > 1.5).
Supplementary Data Set 13. Probes and primers used in this study.
Funding
This work was supported by the National Key Research and Development Program of China (2019YFA0903900), Natural Science Foundation of Guangdong Province (2021A1515010482), Shenzhen Basic Research General Project (JCYJ20220531101618038, JCYJ20230808105419038), China Postdoctoral Science Foundation (2019M653045), and Research Team Cultivation Program of Shenzhen University (2023DFT005).
Data availability
The data reported in this paper have been deposited in NCBI Sequence Read Archive (SRA) database (https://submit.ncbi.nlm.nih.gov/subs/sra/) with the accession numbers SRR19759304 to SRR19759315.
Dive Curated Terms
The following phenotypic, genotypic, and functional terms are of significance to the work described in this paper:
References
Author notes
The author responsible for distribution of materials integral to the findings presented in this article in accordance with the policy described in the Instructions for Authors (https://dbpia.nl.go.kr/plphys/pages/General-Instructions) is Lin Liu ([email protected]).
Conflict of interest statement. None declared.