-
PDF
- Split View
-
Views
-
Cite
Cite
Johannes Leierer, Michael Rudnicki, Susie-Jane Braniff, Paul Perco, Christian Koppelstaetter, Irmgard Mühlberger, Susanne Eder, Julia Kerschbaum, Christoph Schwarzer, Andrea Schroll, Günter Weiss, Stefan Schneeberger, Silvia Wagner, Alfred Königsrainer, Georg A. Böhmig, Gert Mayer, Metallothioneins and renal ageing, Nephrology Dialysis Transplantation, Volume 31, Issue 9, September 2016, Pages 1444–1452, https://doi.org/10.1093/ndt/gfv451
- Share Icon Share
Abstract
Human lifespan is increasing continuously and about one-third of the population >70 years of age suffers from chronic kidney disease. The pathophysiology of the loss of renal function with ageing is unclear.
We determined age-associated gene expression changes in zero-hour biopsies of deceased donor kidneys without laboratory signs of impaired renal function, defined as a last serum creatinine >0.96 mg/dL in females and >1.18 mg/dL in males, using microarray technology and the Significance Analysis of Microarrays routine. Expression changes of selected genes were confirmed by quantitative polymerase chain reaction and in situ hybridization and immunohistochemistry for localization of respective mRNA and protein. Functional aspects were examined in vitro.
Donors were classified into three age groups (<40, 40–59 and >59 years; Groups 1, 2 and 3, respectively). In Group 3 especially, genes encoding for metallothionein (MT) isoforms were more significantly expressed when compared with Group 1; localization studies revealed predominant staining in renal proximal tubular cells. RPTEC/TERT1 cells overexpressing MT2A were less susceptible towards cadmium chloride–induced cytotoxicity and hypoxia-induced apoptosis, both models for increased generation of reactive oxygen species.
Increased expression of MTs in the kidney with ageing might be a protective mechanism against increased oxidative stress, which is closely related to the ageing process. Our findings indicate that MTs are functionally involved in the pathophysiology of ageing-related processes.
INTRODUCTION
During the last decades, the human lifespan has increased continuously in developed countries [1]. During the third US National Health and Nutrition Examination Survey, glomerular filtration rate (GFR) was estimated using the Modification of Diet in Renal Disease formula, and almost 35% of the population ≥70 years of age were reported to have at least Stage 3 chronic kidney disease (CKD) [2]. Similar data have since been reported worldwide [3]. In addition to these cross-sectional studies, longitudinal analyses have also shown that in addition to cortical renal blood flow, GFR declines after the fourth decade by 0.4–1.02 mL/min/year, but a large interindividual range has been reported [1]. Given the socio-economic impact of these findings, it is important to decipher the contribution of ‘physiological ageing’ processes and accumulation of disease-associated factors to renal functional loss and especially the observed interindividual variability [4].
On a glomerular level, ageing and progression of CKD seem to resemble different phenomena. In an autopsy study, the gradual loss of glomerular number after the age of 60 years was accompanied by a decrease in glomerular volume and thickening of the glomerular basement membrane [5]. When combined with afferent and efferent arteriolar atrophy, this leads to global glomerular sclerosis, a finding that is supposed to be characteristic for renal ageing [6]. The reduction of glomerular volume, however, is in striking contrast to the hypertrophy usually noticed in the setting of glomerular loss during the progression of CKD. On the contrary, in the tubulointerstitial compartment, a loss of peritubular capillary density is observed with ageing, but also progression of CKD. The loss of microvasculature creates a hypoxic environment triggering a pro-fibrotic response, which in turn extends the regions of hypoxia by affecting previously unaffected areas [7].
On a molecular level, pathways associated with ageing include the insulin-like growth factor 1 axis, the mammalian target of rapamycin (mTOR) pathway, the sirtuin family [8], the autophagy pathway [9] and genes associated with progerias like Klotho [10]. Another molecular marker for biological age is telomere length [11, 12], and the cell cycle inhibitors CDKN2A (p16INK4a) and CDKN1A (p21WAF1) have been associated with telomere shortening in donor kidneys and even functional outcome after transplantation [13–15].
The aim of this project was to expand our knowledge of gene expression changes in ‘healthy’ ageing kidneys by studying renal biopsy specimens from deceased donor kidneys. Additionally, we studied in vitro functional aspects of genes of interest and looked for possible associations with renal function after transplantation.
MATERIALS AND METHODS
Kidney biopsy samples
Wedge biopsies from 37 deceased donor kidneys ages 20–80 years were obtained prior to reperfusion. Biopsy tissue was bisected and immersed in RNAlater stabilization solution (Ambion, Austin, TX, USA) or formalin fixed and paraffin embedded. The study was approved by the Ethics Board of the Medical University Innsbruck.
RNA extraction and quality control
Total RNA was isolated according to the manufacturer's protocol for the RNeasy Mini Kit (Qiagen, Valencia, CA, USA). RNA yield was determined using a Nanodrop 1000 (Thermo Fisher Scientific, Wilmington, DE, USA). RNA quality was determined by the RNA Integrity Number (RIN) [49] with an Agilent 2100 Bioanalyser and RNA Pico Chips (Agilent Technologies, Palo Alto, CA, USA). To align mRNA quality, only samples with a RIN value of 5–8 were used, with 1 being the most degraded and 10 being the most intact.
Microarray hybridization
For each biopsy, at least 200 ng total RNA was used to generate cyanine 3-labelled cRNA for hybridization to Agilent oligonucleotide microarrays according to the Agilent One-Color Microarray protocol (Agilent Technologies). Arrays were scanned at 5 µm using an Axon Gene Pix 4000B scanner (Molecular Devices, Sunnyvale, CA, USA) and signal intensity data extracted using Agilent Feature Extraction software (version 10.5.1).
Data analysis and statistics
To create a homogeneous sample population and to eliminate confounders, kidney donors with laboratory signs of impaired renal function, defined as a last serum creatinine >0.96 mg/dL in females and >1.18 mg/dL in males, were excluded, as were samples from living donors, resulting in a group of n= 37 samples. Donor age was between 18 and 82 years with 14 female and 23 male donors. Twenty-five donors died of cerebrovascular accidents, 11 of trauma and 1 of other reason. The donor creatinine values were between 0.46 and 0.88 for women and between 0.56 and 1.13 for men. Thirty donors received catecholamines, while seven did not. Clinical parameters are given in Supplementary data, Table S1. In accordance with other studies [50], the 37 samples were divided into three age groups: Group 1: <40 years, n= 7; Group 2: 40–59 years, n= 17 and Group 3: >59 years, n= 13.
The Agi4x44PreProcess R module was used to generate a gene expression datafile for 41 091 features. Quantile–quantile normalization was used to normalize raw mean intensity values. Features were additionally filtered to focus on those having a coefficient of variation that was 1 SD above the mean coefficient of variation of all 41 091 features across all arrays of the data file, leaving 4164 features in the dataset. Raw data are available at NCBI Gene Expression Omnibus with the accession number GSE60807.
Differences in gene expression between the three age groups were analysed by the Significance Analysis of Microarrays method [51], as implemented in the TIGR MeV software (http://www.tm4.org/mev.html), setting the false discovery rate to 5% using 200 permutations per comparison. Age groups were compared by Kruskal–Wallis test. Spearman's rank correlation coefficient was used to test statistical dependence between the age-related gene set and creatinine follow-up as well as between microarray and qPCR data.
Comparisons of clinical parameters sex, cause of death, catecholamine administration used to stabilize haemodynamics prior to explantation and last donor creatinine across the three age groups were done by χ2 tests and t-tests. Associations of age-related gene set expression to clinical parameters were assessed via t-test and correlation analysis to exclude confounding factors that might influence gene expression besides age.
Differences in CdCl2 cytotoxicity were analysed by Mann–Whitney test and differences in hypoxia treatment by t-test for independent samples.
Reverse transcription and preamplification for qPCR
Reverse transcription of RNA was done with the High Capacity cDNA Reverse Transcription kit (Applied Biosystems, Foster City, CA, USA) in a 50-μL reaction according to the manufacturers' instructions. Preamplification was performed using TaqMan Gene Expression Assays (vide infra) and the TaqMan PreAmp Master Mix according to the manufacturer's protocols. All gene expression assays used had been previously tested to ensure uniform preamplification as recommended by the manufacturer (data not shown).
Quantitative real-time PCR
The preamplified cDNA was analysed on a 7500 Fast Real-Time PCR System (Applied Biosystems) using inventoried TaqMan Gene Expression Assays for PPIA; primers and probes for MT isoforms were designed (Supplementary data, Table S1).
Each reaction contained 5 μL cDNA. The mean fractional cycle number (CT) for each gene was determined and the relative amount of transcript for each gene was normalized to the reference gene PPIA as follows: ΔCT= CT (gene of interest) – CT (PPIA). The ΔCT was linearized according to the formula to determine the relative expression of each gene of interest.
In situ hybridization
In situ hybridization of MT2A mRNA was done according to Illig et al. [52, 53]. 3′ and 5′ FAM-labelled probes of 40–45 bases in length were synthesized on an automated system (Microsynth, Balgach, Switzerland). Unlabelled probes were used as controls. Probes and sequences used (end numbers indicate position on mRNA) were as follows:
MT2A—149: 5′-CAGCTTTTCTTGCAGGAGGTGCATTTGCACTCTTTGCATT-3′
MT2A—290: 5′-AAAATCCAGGTTTGTGGAAGTCGCGTTCTTTACATCTGGGAGCGG-3′
Sheep anti-FITC Fab fragments conjugated to horseradish peroxidase (HRP, Roche, catalogue no. 11426346910; dilution 1:50) were used for detection of FAM-labelled probes.
Immunohistochemical staining
Four-micrometre sections of paraffin-embedded tissues were deparaffinated and rehydrated. After blocking in 0.3% H2O2, antigen retrieval was done with 2 M HCl and proteinase K. Sections were blocked with 20% normal goat serum and incubated with primary antibody directed against a single and highly conserved epitope of MT1 and MT2 (monoclonal mouse anti-metallothionein clone E9, M0639, Dako; dilution 1:100) overnight at 4°C. Secondary antibody (goat anti-mouse Ig labelled with HRP, P0447, Dako; dilution 1:1000) was applied for 1 h at room temperature and visualized with DAB substrate (Sigma-Aldrich, Vienna, Austria) followed by counterstaining in Meyer's Hämalaun.
Cell culture
Human proximal tubule cells RPTEC/TERT1 (Evercyte, Vienna, Austria) [16] were cultured as described previously [54]. Cell culture reagents were obtained from Life Technologies (Lofer, Austria) and Sigma-Aldrich.
Generation of cell lines stably overexpressing MT2A
A cell line (RPTEC/TERT1) stably overexpressing MT2A was generated using a gamma retroviral vector with an MP91 backbone (kindly provided by the Section for Virology, Medical University Innsbruck). The MT2A gene sequence (including a NotI and a BstXI restriction) was amplified from RPTEC/TERT1 cDNA by PCR using the following primers:
forward: 5′-CGAAGCGGCCGCATGGATCCCAACTGCTCCTGCG-3′;
reverse: 5′-CGAACCACCGCGGTGGTCAGGCGCAGCAGCTGCAC-3′.
The DNA fragment and gamma retroviral vector were digested with NotI and BstXI restriction enzymes and ligated overnight at 16°C. Amplification of ligated DNA was done via chemocompetent bacterial cells. The correct position of the inserted MT2A was confirmed by control digests and sequencing of the amplified vector DNA. Generation of gamma retroviral particles and infection of cells was performed as described previously [55].
Western blot analysis
Cultured cells were lysed in radio-Immunoprecipitation assay buffer containing proteinase inhibitor. Thirty milligrams of protein per lane were resolved using 4–12% Bis–Tris gels and transferred onto a nitrocellulose membrane (Life Technologies). Primary MT antibody (vide supra) diluted 1:100 was incubated overnight at 4°C. After washing, membranes were incubated with secondary antibody (goat anti-mouse Ig labelled with HRP, P0447, Dako). Enzymatic reaction was induced with enhanced chemiluminescence solutions and membranes subsequently scanned using the LAS 4000 system (GE Healthcare).
Cell treatment
CdCl2 stock (10 mM) was prepared in distilled water and stored at −20°C in aliquots. Subsequent dilutions were prepared freshly in medium. For all experiments, RPTEC/TERT1 cells were used at confluence. Cells were cultured on 96-well plates and incubated with 0, 2, 4, 6, 8, 10 or 15 µM CdCl2 for 72 h. Ten microlitres of supernatant of each well were analysed with the LDH-Cytotoxicity Assay Kit II (ab65393; Abcam, Cambridge, UK) according to the manufacturer's protocol. For hypoxia experiments, cells were grown to confluence in 96-well plates and inserted into a hypoxia chamber (Billups-Rothenberg, Del Mar, CA, USA), which was flushed with a custom gas mixture containing 5% CO2 and 95% N2 for 15 min. The partial pressure of oxygen (pO2) was determined in the culture medium by a gas analyser (Bayer/Ciba-Corning 278, Diamond Diagnostics, Holliston, MA, USA). Six hours after CO2/N2 gas incubation, pO2 was 35 mmHg, which persisted for 36 h.
RESULTS
Gene expression profiling of zero-hour kidney biopsies
Comparing three chronological donor age groups (<40, 40–59 and >59 years; Groups 1, 2 and 3, respectively), no significant differences with respect to the clinical and laboratory parameters (gender, cause of death, catecholamine administration and last donor creatinine) could be found. Microarray analyses of differences in gene expression between the three age groups revealed 16 features encoding 13 genes with significantly increased expression in Group 3 when compared with Group 1: metallothionein (MT) 1 isoforms A, B, E, H, L, M; two probes of MT 1G, 1X and 2A, respectively; immunoglobulin lambda-like polypeptide 5 and immunoglobulin heavy constant gamma 1 and two unknown transcripts. Thus, 9 of the 16 features in this cluster are isoforms of the MT gene family (Table 1). In order to check whether our results were due to high-sequence similarity, a multiple alignment of the given MTs was created using clustalw (http://www.ebi.ac.at/) (Supplementary data, Figure S1). Although aligned sequences could be detected, completely identically overlapping sequence fragments could be found only in a few cases. Thus, the age-associated transcriptional changes for the named features are not due to overlapping sequences but rather reflect differential expression of each single gene.
Microarray analysis revealed the following 16 transcripts to be significantly upregulated in age group 3 (>59 years) when compared with age group 1 (<40 years)
Probe . | Accnum . | Symbol . | Description . | Gr1 mean expr. . | Gr2 mean expr. . | Gr3 mean expr. . | P-value of Group 1 versus Group 3 . |
---|---|---|---|---|---|---|---|
A_32_P200144 | AK130614 | IGHG1 | Immunoglobulin heavy constant gamma 1 | 6.76 | 8.60 | 8.97 | 0.024 |
A_23_P43979 | M87790 | IGLL5 | Immunoglobulin lambda-like polypeptide 5 | 7.13 | 8.80 | 9.18 | 0.023 |
A_23_P54840 | NM_005946 | MT1A | MT1A | 10.83 | 11.85 | 12.30 | 0.003 |
A_23_P37983 | NM_005947 | MT1B | MT1B | 10.55 | 11.76 | 12.19 | 0.005 |
A_23_P206724 | NM_175617 | MT1E | MT1E | 10.67 | 11.93 | 12.42 | 0.005 |
A_23_P206707 | NM_005950 | MT1G | MT1G | 9.31 | 10.34 | 10.80 | 0.009 |
A_23_P60933 | NM_005950 | MT1G | MT1G | 11.39 | 12.63 | 13.04 | 0.013 |
A_23_P414343 | NM_005951 | MT1H | MT1H | 10.80 | 11.98 | 12.50 | 0.006 |
A_23_P427703 | X97261 | MT1L | MT1L (gene/pseudogene) | 10.52 | 11.80 | 12.26 | 0.009 |
A_23_P66241 | NM_176870 | MT1M | MT1M | 9.14 | 10.21 | 10.69 | 0.038 |
A_23_P303242 | NM_005952 | MT1X | MT1X | 10.82 | 11.96 | 12.48 | 0.002 |
A_24_P125096 | NM_005952 | MT1X | MT1X | 10.27 | 11.38 | 11.86 | 0.006 |
A_23_P106844 | NM_005953 | MT2A | MT2A | 11.96 | 13.01 | 13.48 | 0.024 |
A_24_P361896 | NM_005953 | MT2A | MT2A | 11.65 | 12.81 | 13.33 | 0.022 |
A_23_P163782 | NM_001039954 | NA | NA | 10.33 | 11.45 | 11.91 | 0.016 |
A_23_P252413 | NA | NA | NA | 11.46 | 12.54 | 13.03 | 0.005 |
Probe . | Accnum . | Symbol . | Description . | Gr1 mean expr. . | Gr2 mean expr. . | Gr3 mean expr. . | P-value of Group 1 versus Group 3 . |
---|---|---|---|---|---|---|---|
A_32_P200144 | AK130614 | IGHG1 | Immunoglobulin heavy constant gamma 1 | 6.76 | 8.60 | 8.97 | 0.024 |
A_23_P43979 | M87790 | IGLL5 | Immunoglobulin lambda-like polypeptide 5 | 7.13 | 8.80 | 9.18 | 0.023 |
A_23_P54840 | NM_005946 | MT1A | MT1A | 10.83 | 11.85 | 12.30 | 0.003 |
A_23_P37983 | NM_005947 | MT1B | MT1B | 10.55 | 11.76 | 12.19 | 0.005 |
A_23_P206724 | NM_175617 | MT1E | MT1E | 10.67 | 11.93 | 12.42 | 0.005 |
A_23_P206707 | NM_005950 | MT1G | MT1G | 9.31 | 10.34 | 10.80 | 0.009 |
A_23_P60933 | NM_005950 | MT1G | MT1G | 11.39 | 12.63 | 13.04 | 0.013 |
A_23_P414343 | NM_005951 | MT1H | MT1H | 10.80 | 11.98 | 12.50 | 0.006 |
A_23_P427703 | X97261 | MT1L | MT1L (gene/pseudogene) | 10.52 | 11.80 | 12.26 | 0.009 |
A_23_P66241 | NM_176870 | MT1M | MT1M | 9.14 | 10.21 | 10.69 | 0.038 |
A_23_P303242 | NM_005952 | MT1X | MT1X | 10.82 | 11.96 | 12.48 | 0.002 |
A_24_P125096 | NM_005952 | MT1X | MT1X | 10.27 | 11.38 | 11.86 | 0.006 |
A_23_P106844 | NM_005953 | MT2A | MT2A | 11.96 | 13.01 | 13.48 | 0.024 |
A_24_P361896 | NM_005953 | MT2A | MT2A | 11.65 | 12.81 | 13.33 | 0.022 |
A_23_P163782 | NM_001039954 | NA | NA | 10.33 | 11.45 | 11.91 | 0.016 |
A_23_P252413 | NA | NA | NA | 11.46 | 12.54 | 13.03 | 0.005 |
Data of the three groups indicate normalized intensity values in log2 scale. Accnum, accession number; Gr, group; expr, expression.
Microarray analysis revealed the following 16 transcripts to be significantly upregulated in age group 3 (>59 years) when compared with age group 1 (<40 years)
Probe . | Accnum . | Symbol . | Description . | Gr1 mean expr. . | Gr2 mean expr. . | Gr3 mean expr. . | P-value of Group 1 versus Group 3 . |
---|---|---|---|---|---|---|---|
A_32_P200144 | AK130614 | IGHG1 | Immunoglobulin heavy constant gamma 1 | 6.76 | 8.60 | 8.97 | 0.024 |
A_23_P43979 | M87790 | IGLL5 | Immunoglobulin lambda-like polypeptide 5 | 7.13 | 8.80 | 9.18 | 0.023 |
A_23_P54840 | NM_005946 | MT1A | MT1A | 10.83 | 11.85 | 12.30 | 0.003 |
A_23_P37983 | NM_005947 | MT1B | MT1B | 10.55 | 11.76 | 12.19 | 0.005 |
A_23_P206724 | NM_175617 | MT1E | MT1E | 10.67 | 11.93 | 12.42 | 0.005 |
A_23_P206707 | NM_005950 | MT1G | MT1G | 9.31 | 10.34 | 10.80 | 0.009 |
A_23_P60933 | NM_005950 | MT1G | MT1G | 11.39 | 12.63 | 13.04 | 0.013 |
A_23_P414343 | NM_005951 | MT1H | MT1H | 10.80 | 11.98 | 12.50 | 0.006 |
A_23_P427703 | X97261 | MT1L | MT1L (gene/pseudogene) | 10.52 | 11.80 | 12.26 | 0.009 |
A_23_P66241 | NM_176870 | MT1M | MT1M | 9.14 | 10.21 | 10.69 | 0.038 |
A_23_P303242 | NM_005952 | MT1X | MT1X | 10.82 | 11.96 | 12.48 | 0.002 |
A_24_P125096 | NM_005952 | MT1X | MT1X | 10.27 | 11.38 | 11.86 | 0.006 |
A_23_P106844 | NM_005953 | MT2A | MT2A | 11.96 | 13.01 | 13.48 | 0.024 |
A_24_P361896 | NM_005953 | MT2A | MT2A | 11.65 | 12.81 | 13.33 | 0.022 |
A_23_P163782 | NM_001039954 | NA | NA | 10.33 | 11.45 | 11.91 | 0.016 |
A_23_P252413 | NA | NA | NA | 11.46 | 12.54 | 13.03 | 0.005 |
Probe . | Accnum . | Symbol . | Description . | Gr1 mean expr. . | Gr2 mean expr. . | Gr3 mean expr. . | P-value of Group 1 versus Group 3 . |
---|---|---|---|---|---|---|---|
A_32_P200144 | AK130614 | IGHG1 | Immunoglobulin heavy constant gamma 1 | 6.76 | 8.60 | 8.97 | 0.024 |
A_23_P43979 | M87790 | IGLL5 | Immunoglobulin lambda-like polypeptide 5 | 7.13 | 8.80 | 9.18 | 0.023 |
A_23_P54840 | NM_005946 | MT1A | MT1A | 10.83 | 11.85 | 12.30 | 0.003 |
A_23_P37983 | NM_005947 | MT1B | MT1B | 10.55 | 11.76 | 12.19 | 0.005 |
A_23_P206724 | NM_175617 | MT1E | MT1E | 10.67 | 11.93 | 12.42 | 0.005 |
A_23_P206707 | NM_005950 | MT1G | MT1G | 9.31 | 10.34 | 10.80 | 0.009 |
A_23_P60933 | NM_005950 | MT1G | MT1G | 11.39 | 12.63 | 13.04 | 0.013 |
A_23_P414343 | NM_005951 | MT1H | MT1H | 10.80 | 11.98 | 12.50 | 0.006 |
A_23_P427703 | X97261 | MT1L | MT1L (gene/pseudogene) | 10.52 | 11.80 | 12.26 | 0.009 |
A_23_P66241 | NM_176870 | MT1M | MT1M | 9.14 | 10.21 | 10.69 | 0.038 |
A_23_P303242 | NM_005952 | MT1X | MT1X | 10.82 | 11.96 | 12.48 | 0.002 |
A_24_P125096 | NM_005952 | MT1X | MT1X | 10.27 | 11.38 | 11.86 | 0.006 |
A_23_P106844 | NM_005953 | MT2A | MT2A | 11.96 | 13.01 | 13.48 | 0.024 |
A_24_P361896 | NM_005953 | MT2A | MT2A | 11.65 | 12.81 | 13.33 | 0.022 |
A_23_P163782 | NM_001039954 | NA | NA | 10.33 | 11.45 | 11.91 | 0.016 |
A_23_P252413 | NA | NA | NA | 11.46 | 12.54 | 13.03 | 0.005 |
Data of the three groups indicate normalized intensity values in log2 scale. Accnum, accession number; Gr, group; expr, expression.
No other genes could be found that showed significant differential expression. This might be due to the rather strict rules applied for statistical data analysis (false discovery rate to 5% using 200 permutations per comparison), which were applied to minimize false-positive results.
To validate the microarray data, quantitative polymerase chain reaction (qPCR) primers and probes were designed for the functional MT1 isoforms and MT2A and expression levels were determined in the same zero-hour biopsies as used for microarray experiments (due to the limited access to RNA, only 30 samples could be analysed). qPCR analysis showed that MT mRNA correlated significantly with microarray data (Supplementary data, Table S4). When comparing qPCR expression levels in the three age groups, a clear tendency towards of higher expression of MTs in Group 3 could be seen, but due to the reduced sample size, only the levels for MT1X and MT2A in Group 3 reached statistical significance (Figure 1). Correlations between MT1X as well as MT2A and age were also significant (r = 0.373, P = 0.038; r = 0.462, P = 0.009).
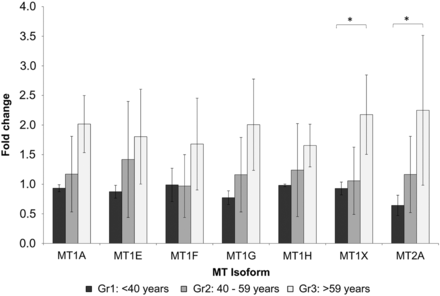
mRNA levels of MT isoforms 1A, 1E, 1F, 1G, 1H, 1X and 2A compared in the three age groups (<40 years: black bars; 40–59 years: grey bars; >59 years: light grey bars) analysed by qPCR. MT1X and MT2A are significantly higher in Group 3 compared with Group 1 (*P < 0.05). Data are presented as the fold change normalized to the reference gene PPIA.
Associations of age-related gene set expression to the clinical parameters
There was no significant association of one of the differentially expressed genes to sex, cause of death, catecholamine administration and last donor creatinine; additionally, no correlation was found with cold ischaemia time (Supplementary data, Table S3). However, all MT isoforms found to be upregulated with age correlated significantly and positively with serum creatinine levels at 3 months after transplantation (Table 2); later, a positive trend was observed. MT2A qPCR levels also showed a strong trend for correlation with 3-month postoperative renal function (r = 0.432, P = 0.057), and thus we focussed on this isoform in our in vitro functional experiments.
Spearman correlation analysis of microarray data revealed the MT isoforms correlate significantly with age (except for MT1M) and serum creatinine levels at the 3-month follow-up
Probe . | Accnum . | Symbol . | Description . | Mean expr. . | Spearman correlation . | |||
---|---|---|---|---|---|---|---|---|
r for age . | P-value . | r for crea3m . | P-value . | |||||
A_32_P200144 | AK130614 | IGHG1 | Immunoglobulin heavy constant gamma 1 | 8.38 | 0.397 | 0.015 | 0.118 | 0.575 |
A_23_P43979 | M87790 | IGLL5 | Immunoglobulin lambda-like polypeptide 5 | 8.62 | 0.377 | 0.022 | 0.103 | 0.625 |
A_23_P54840 | NM_005946 | MT1A | MT1A | 10.83 | 0.455 | 0.005 | 0.459 | 0.021 |
A_23_P37983 | NM_005947 | MT1B | MT1B | 10.55 | 0.405 | 0.013 | 0.412 | 0.041 |
A_23_P206724 | NM_175617 | MT1E | MT1E | 10.67 | 0.390 | 0.017 | 0.473 | 0.017 |
A_23_P206707 | NM_005950 | MT1G | MT1G | 9.31 | 0.360 | 0.029 | 0.447 | 0.025 |
A_23_P60933 | NM_005950 | MT1G | MT1G | 11.39 | 0.340 | 0.039 | 0.416 | 0.038 |
A_23_P414343 | NM_005951 | MT1H | MT1H | 10.80 | 0.408 | 0.012 | 0.465 | 0.019 |
A_23_P427703 | X97261 | MT1L | MT1L (gene/pseudogene) | 10.52 | 0.376 | 0.022 | 0.440 | 0.028 |
A_23_P66241 | NM_176870 | MT1M | MT1M | 9.14 | 0.304 | 0.068 | 0.444 | 0.026 |
A_23_P303242 | NM_005952 | MT1X | MT1X | 10.82 | 0.465 | 0.004 | 0.457 | 0.022 |
A_24_P125096 | NM_005952 | MT1X | MT1X | 10.27 | 0.406 | 0.013 | 0.450 | 0.024 |
A_23_P106844 | NM_005953 | MT2A | MT2A | 11.96 | 0.334 | 0.043 | 0.397 | 0.049 |
A_24_P361896 | NM_005953 | MT2A | MT2A | 11.65 | 0.348 | 0.035 | 0.440 | 0.028 |
A_23_P163782 | NM_001039954 | NA | NA | 10.33 | 0.367 | 0.025 | 0.414 | 0.040 |
A_23_P252413 | NA | NA | NA | 11.46 | 0.436 | 0.007 | 0.449 | 0.024 |
Probe . | Accnum . | Symbol . | Description . | Mean expr. . | Spearman correlation . | |||
---|---|---|---|---|---|---|---|---|
r for age . | P-value . | r for crea3m . | P-value . | |||||
A_32_P200144 | AK130614 | IGHG1 | Immunoglobulin heavy constant gamma 1 | 8.38 | 0.397 | 0.015 | 0.118 | 0.575 |
A_23_P43979 | M87790 | IGLL5 | Immunoglobulin lambda-like polypeptide 5 | 8.62 | 0.377 | 0.022 | 0.103 | 0.625 |
A_23_P54840 | NM_005946 | MT1A | MT1A | 10.83 | 0.455 | 0.005 | 0.459 | 0.021 |
A_23_P37983 | NM_005947 | MT1B | MT1B | 10.55 | 0.405 | 0.013 | 0.412 | 0.041 |
A_23_P206724 | NM_175617 | MT1E | MT1E | 10.67 | 0.390 | 0.017 | 0.473 | 0.017 |
A_23_P206707 | NM_005950 | MT1G | MT1G | 9.31 | 0.360 | 0.029 | 0.447 | 0.025 |
A_23_P60933 | NM_005950 | MT1G | MT1G | 11.39 | 0.340 | 0.039 | 0.416 | 0.038 |
A_23_P414343 | NM_005951 | MT1H | MT1H | 10.80 | 0.408 | 0.012 | 0.465 | 0.019 |
A_23_P427703 | X97261 | MT1L | MT1L (gene/pseudogene) | 10.52 | 0.376 | 0.022 | 0.440 | 0.028 |
A_23_P66241 | NM_176870 | MT1M | MT1M | 9.14 | 0.304 | 0.068 | 0.444 | 0.026 |
A_23_P303242 | NM_005952 | MT1X | MT1X | 10.82 | 0.465 | 0.004 | 0.457 | 0.022 |
A_24_P125096 | NM_005952 | MT1X | MT1X | 10.27 | 0.406 | 0.013 | 0.450 | 0.024 |
A_23_P106844 | NM_005953 | MT2A | MT2A | 11.96 | 0.334 | 0.043 | 0.397 | 0.049 |
A_24_P361896 | NM_005953 | MT2A | MT2A | 11.65 | 0.348 | 0.035 | 0.440 | 0.028 |
A_23_P163782 | NM_001039954 | NA | NA | 10.33 | 0.367 | 0.025 | 0.414 | 0.040 |
A_23_P252413 | NA | NA | NA | 11.46 | 0.436 | 0.007 | 0.449 | 0.024 |
Mean expression (mean expr.) indicates normalized intensity values in log2 scale of given genes for n= 37. Mean expression of serum creatinine levels for n= 25 patients at the 3-month follow-up was 1.8. Accnum, accession number; crea3m, serum creatinine at 3 months.
Spearman correlation analysis of microarray data revealed the MT isoforms correlate significantly with age (except for MT1M) and serum creatinine levels at the 3-month follow-up
Probe . | Accnum . | Symbol . | Description . | Mean expr. . | Spearman correlation . | |||
---|---|---|---|---|---|---|---|---|
r for age . | P-value . | r for crea3m . | P-value . | |||||
A_32_P200144 | AK130614 | IGHG1 | Immunoglobulin heavy constant gamma 1 | 8.38 | 0.397 | 0.015 | 0.118 | 0.575 |
A_23_P43979 | M87790 | IGLL5 | Immunoglobulin lambda-like polypeptide 5 | 8.62 | 0.377 | 0.022 | 0.103 | 0.625 |
A_23_P54840 | NM_005946 | MT1A | MT1A | 10.83 | 0.455 | 0.005 | 0.459 | 0.021 |
A_23_P37983 | NM_005947 | MT1B | MT1B | 10.55 | 0.405 | 0.013 | 0.412 | 0.041 |
A_23_P206724 | NM_175617 | MT1E | MT1E | 10.67 | 0.390 | 0.017 | 0.473 | 0.017 |
A_23_P206707 | NM_005950 | MT1G | MT1G | 9.31 | 0.360 | 0.029 | 0.447 | 0.025 |
A_23_P60933 | NM_005950 | MT1G | MT1G | 11.39 | 0.340 | 0.039 | 0.416 | 0.038 |
A_23_P414343 | NM_005951 | MT1H | MT1H | 10.80 | 0.408 | 0.012 | 0.465 | 0.019 |
A_23_P427703 | X97261 | MT1L | MT1L (gene/pseudogene) | 10.52 | 0.376 | 0.022 | 0.440 | 0.028 |
A_23_P66241 | NM_176870 | MT1M | MT1M | 9.14 | 0.304 | 0.068 | 0.444 | 0.026 |
A_23_P303242 | NM_005952 | MT1X | MT1X | 10.82 | 0.465 | 0.004 | 0.457 | 0.022 |
A_24_P125096 | NM_005952 | MT1X | MT1X | 10.27 | 0.406 | 0.013 | 0.450 | 0.024 |
A_23_P106844 | NM_005953 | MT2A | MT2A | 11.96 | 0.334 | 0.043 | 0.397 | 0.049 |
A_24_P361896 | NM_005953 | MT2A | MT2A | 11.65 | 0.348 | 0.035 | 0.440 | 0.028 |
A_23_P163782 | NM_001039954 | NA | NA | 10.33 | 0.367 | 0.025 | 0.414 | 0.040 |
A_23_P252413 | NA | NA | NA | 11.46 | 0.436 | 0.007 | 0.449 | 0.024 |
Probe . | Accnum . | Symbol . | Description . | Mean expr. . | Spearman correlation . | |||
---|---|---|---|---|---|---|---|---|
r for age . | P-value . | r for crea3m . | P-value . | |||||
A_32_P200144 | AK130614 | IGHG1 | Immunoglobulin heavy constant gamma 1 | 8.38 | 0.397 | 0.015 | 0.118 | 0.575 |
A_23_P43979 | M87790 | IGLL5 | Immunoglobulin lambda-like polypeptide 5 | 8.62 | 0.377 | 0.022 | 0.103 | 0.625 |
A_23_P54840 | NM_005946 | MT1A | MT1A | 10.83 | 0.455 | 0.005 | 0.459 | 0.021 |
A_23_P37983 | NM_005947 | MT1B | MT1B | 10.55 | 0.405 | 0.013 | 0.412 | 0.041 |
A_23_P206724 | NM_175617 | MT1E | MT1E | 10.67 | 0.390 | 0.017 | 0.473 | 0.017 |
A_23_P206707 | NM_005950 | MT1G | MT1G | 9.31 | 0.360 | 0.029 | 0.447 | 0.025 |
A_23_P60933 | NM_005950 | MT1G | MT1G | 11.39 | 0.340 | 0.039 | 0.416 | 0.038 |
A_23_P414343 | NM_005951 | MT1H | MT1H | 10.80 | 0.408 | 0.012 | 0.465 | 0.019 |
A_23_P427703 | X97261 | MT1L | MT1L (gene/pseudogene) | 10.52 | 0.376 | 0.022 | 0.440 | 0.028 |
A_23_P66241 | NM_176870 | MT1M | MT1M | 9.14 | 0.304 | 0.068 | 0.444 | 0.026 |
A_23_P303242 | NM_005952 | MT1X | MT1X | 10.82 | 0.465 | 0.004 | 0.457 | 0.022 |
A_24_P125096 | NM_005952 | MT1X | MT1X | 10.27 | 0.406 | 0.013 | 0.450 | 0.024 |
A_23_P106844 | NM_005953 | MT2A | MT2A | 11.96 | 0.334 | 0.043 | 0.397 | 0.049 |
A_24_P361896 | NM_005953 | MT2A | MT2A | 11.65 | 0.348 | 0.035 | 0.440 | 0.028 |
A_23_P163782 | NM_001039954 | NA | NA | 10.33 | 0.367 | 0.025 | 0.414 | 0.040 |
A_23_P252413 | NA | NA | NA | 11.46 | 0.436 | 0.007 | 0.449 | 0.024 |
Mean expression (mean expr.) indicates normalized intensity values in log2 scale of given genes for n= 37. Mean expression of serum creatinine levels for n= 25 patients at the 3-month follow-up was 1.8. Accnum, accession number; crea3m, serum creatinine at 3 months.
Cellular localization of MTs
To investigate the functional aspects of MTs, it was necessary to first assess their localization within the kidney. Renal localization of MT2A mRNA and MT protein was analysed by in situ hybridization and immunohistochemistry, respectively. The signal of MT mRNA could be seen almost exclusively in the brush border of proximal tubuli of the kidney, while glomeruli were nearly completely spared. The findings were replicated on a protein level again showing predominant staining in the same location (Figure 2).
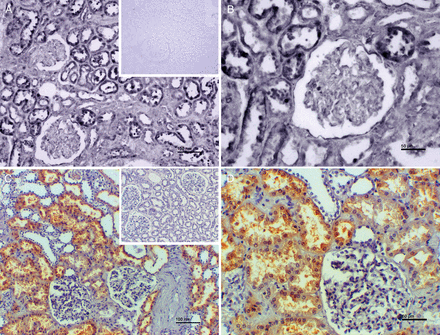
Representative images of in situ hybridization for MT2A mRNA (A and B) and immunohistochemistry with an antibody against MT1 and MT2 isoforms (C and D). MT2A on the mRNA level (dark blue/black labels in A and B) as well as MTs on the protein level (brown coloured in C and D) are located in (epithelial cells of) proximal tubuli. Glomeruli show sparse MT labelling. Inserts show negative controls. Scale bars indicate 100 µm (A and C) and 50 µm (B and D).
Functional analysis
After localization of MT in the human kidney to the proximal tubular system, we used an RPTEC/TERT1 cell line, which is a recently developed human renal proximal tubule cell line that shares many characteristics with primary renal cells [16], for in vitro experiments with the intention of showing the effects of overexpressed MT under stress conditions that are typical for aged kidneys.
Using a gamma retroviral system, the MT2A gene was introduced into the RPTEC/TERT1 cells, thereby forcing the cells to overexpress MT2A protein. This was confirmed by western blot (Supplementary data, Figure S2). Functional tests were done by exposing control and MT2A-overexpressing cells to cadmium chloride (CdCl2) in concentrations of 0–20 µM. Although no differences between the two cell lines could be found following short-time incubation with CdCl2, after 72 h incubation control cells showed a dose-dependent increase in cytotoxicity and at 4, 6 and 8 µM CdCl2, while MT2A-overexpressing cells were significantly less affected (Figure 3). In another test, control and MT2A-overexpressing cells were incubated for 36 h under hypoxic conditions (5% CO2). MT2A-overexpressing cells were significantly less affected by apoptosis than control cells, as assessed by terminal deoxynucleotidyl transferase dUTP nick end labeling (TUNEL) assay (Figures 4 and 5).
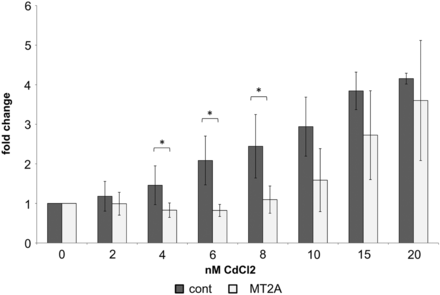
Fold change of LDH cytotoxicity of RPTEC/TERT1 cells (cont) compared with RPTEC/TERT1 overexpressing MT2A after incubation with various concentrations of CdCl2 for 72 h. MT2A-overexpressing cells were significantly less sensitive to 4, 6 and 8 µM CdCl2 than control cells (n= 5; *P < 0.05).
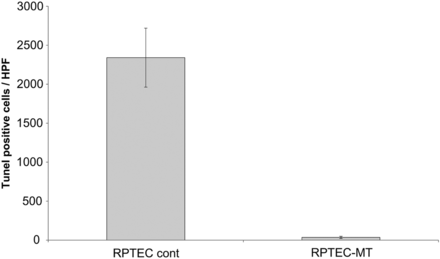
Effect of 36 h of hypoxia on RPTEC control and RPTEC MT cells assessed by TUNEL assay. RPTEC MT cells were significantly (P < 0.0001) less susceptible to hypoxia than control cells. Representative experiment of three with n= 5 for each is shown.
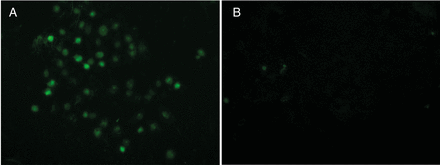
RPTEC control (A) and RPTEC MT (B) cells after 36-h incubation under hypoxic conditions stained by TUNEL assay.
DISCUSSION
In this project, we used biopsies from kidneys of deceased donors obtained immediately prior to transplantation to describe age-related changes in renal genome-wide gene expression. In accordance with other studies [17, 18], we excluded donors with laboratory signs of acute kidney injury to study apparently ‘healthy’ organs. Hitherto most studies analysing transcriptional changes of the ageing kidney [17, 18] have shown multiple, yet small differential gene expression between chronologically ‘younger’ and ‘older’ organs, suggesting that the functional decline associated with ageing may be the result of subtle changes in multiple pathways. In contrast, our analyses revealed most prominently an augmented expression of isoforms of the MT gene family with increasing chronological donor age. A reason for this discrepancy might be the use of different specimens (‘healthy donor kidneys’ versus ‘normal tissue’ removed at nephrectomy due to cancer), as we could not identify other confounding factors such as cause of donor death, cold ischaemia time, gender, catecholamine administration prior to organ procurement or last donor creatinine.
It could be argued that our results are affected by a selection bias by including extremely ‘poor’ or ‘excellent’ donors. However, we do argue that the pre-transplant donor characteristics (e.g. use of catecholamines) and post-transplant outcome (e.g. postoperative acute renal failure) observed are close to what is expected in our unit and thus selection bias seems unlikely to have happened. Nonetheless, it would be very interesting to include kidneys of living donors. However, the frequency of living donor transplantation is low in our centre and the age of the donors does not span the spectrum covered by the deceased population in our article.
In our study, MT2A mRNA as well as MT1 and MT2 protein abundance, which showed the most significant changes associated with age, were mostly localized to the proximal renal tubules and their brush borders. To our knowledge, only a few groups have been able to localize MTs to the renal proximal tubule [19–21] and we wanted to demonstrate the important role of MTs, especially in those cells.
It has been described previously that ageing-related kidney damage is associated with an increase in superoxide production [22, 23], and the reactive oxygen species (ROS) scavenging properties of MTs are well described [24, 25]. Therefore, we hypothesize that the overexpression of MTs might be an intrinsic protective mechanism. When MT2A was genetically overexpressed in the proximal tubular cell line RPTEC/TERT1, CdCl2- and hypoxia-induced cytotoxicity after 72 h of incubation was markedly reduced when compared with control cells. This may indicate a role of MT2A in the scavenging of ROS, as CdCl2 induces excessive amounts of ROS, eliciting oxidative stress damage such as destruction of DNA, proteins and lipid function and activates pro-apoptotic pathways by mitochondrial damage in proximal tubular cells [26]. In addition, incubation of cells under hypoxic conditions induced significantly more apoptosis in control cells when compared with MT2A-overexpressing cells. These data are in accordance with studies performed in MT knockout mouse models, which show that depletion of MT exacerbates intermittent hypoxia-induced renal injury [27] and worsens diabetic nephropathy in streptozotocin-induced diabetes [28]. In addition, podocyte-specific overexpression of MT in a mouse model reduced diabetic nephropathy [29], and effective delivery of endogenous antioxidants MT and superoxide dismutase can ameliorate diabetic nephropathy [30, 31].
Increased renal expression of MTs during ageing might be an attempt by the kidney to scavenge ROS and thus counteract pro-apoptotic actions of oxidative stress, which has been shown to increase during renal ageing [32]. However, we also showed that MT expression in renal tissue before transplantation positively correlates with serum creatinine after 3 months of follow-up. This finding could indicate that MTs are an indicator of oxidative stress in the donor organ reflecting a (subclinical) vulnerable status of the kidney. This status might characterize kidneys that perform poorly whenever an additional burden, such as ischaemia/reperfusion injury, hemodynamic changes, calcineurin inhibitor toxicity and allogeneic immune responses, is put on the organ [33, 34].
MTs are a family of cysteine-rich, low molecular weight proteins. One of their functions is to interact via their thiols with divalent heavy metal cations [35], contributing to their detoxification (e.g. mercury and copper) or homeostasis (e.g. copper and zinc) [36]. However, understanding the various other roles of MTs is complicated by the fact that until today, 11 functional MT genes, 7 pseudogenes and 4 MT-like genes have been identified and the corresponding proteins mostly contain only small differences in amino acid sequence [37]. MTs are classified, based on factors such as molecular weight, metal-binding specificity, encoding gene and chromosomal location, among others, into four groups. The major isoforms are MT1 (with isoforms A, B, E, F, G, H and X) and MT2, which are present in the majority of cells throughout the body. MT3 is found primarily in the brain and central nervous system and MT4 in the skin and upper gastrointestinal tract [36, 38, 39]. As mentioned above, MTs also have protective effects against free radicals, especially ROS [24], and are capable of regulating cellular redox potential [25]. It seems likely that MT expression is also increased by cellular stress such as, for example, hypoxia [23]. Increased MT levels were detected in tumour cells of many tissues, including breast and kidney, and MT levels are directly connected with the grade and prognosis of these diseases [40]. Induction of MT is also reported with hyperglycaemia [41, 42], furosemide use [43] and vitamin D [44] application. Each of these factors may be applicable for deceased donors >60 years of age. Thus, we cannot completely exclude that MT expression changes might also be partially induced by these factors.
On the other hand, MTs have been associated with the ageing process previously. When transgenetically overexpressed in cardiac tissue of FVB mice, lifespan was increased by 14% and a reduction in superoxide generation was observed [45]. Caloric restriction has been shown to prolong lifespan in several species and MTs are upregulated by this intervention in a variety of tissues [46]. In particular, zinc-bound MTs could play a key role under increased oxidative stress because of their intrinsic antioxidant properties as well as their ability to release zinc ions necessary for several proteins and enzymes also involved in antioxidant and DNA-repair responses. This assumption is supported by the fact that during ageing, but also inflammation, zinc release from MTs is impaired [47]. MT-1-overexpressing mice had significantly increased survival compared with their respective controls when fed a standard or zinc-supplemented diet [48].
In summary, excessive production of ROS, as observed during ageing, might lead to an increased expression of MTs in the kidney as a counterregulatory protective mechanism. Therefore, we conclude that our findings are more in line with the theory that MTs are not merely ‘markers’ of the ageing process, but rather are functionally involved in the pathophysiology of ageing-related processes.
AUTHORS’ CONTRIBUTIONS
G.M., M.R., C.K. and J.L. were responsible for the conception and design. S.-J.B., S.E. and M.R. did microarray and qPCR setup and analysis. P.P., I.M. and J.L. did the statistical analysis and interpretation of data. M.R. and J.K. collected follow-up data from patients. C.S. and J.L. were responsible for in situ hybridization and immunohistochemistry experiments; the latter did cell culture experiments. A.S. and G.W. conducted hypoxia experiments and TUNEL staining. S.S. conducted biopsy collection in Innsbruck, S.W. and A.K. in Tübingen and G.A.B. in Vienna. S.W. and G.A.B. collected follow-up data. M.R. and J.L. wrote the report.
CONFLICT OF INTEREST STATEMENT
None declared. The results presented in this article have not been published previously in whole or part, except in abstract format.
ACKNOWLEDGEMENTS
We would like to thank Dr Lisa Egerer and Dr Janine Kimpel from the Division of Virology, Department of Hygiene, Microbiology and Social Medicine, Medical University of Innsbruck for their help in generating the MT-overexpressing hTERT/RPTEC cell line. This study was supported by the Austrian Science Foundation (grant FWF-P-19876-B11). The funding source of this study had no role in study design, data collection, data analysis, data interpretation or writing of the report.
REFERENCES
Author notes
These authors contributed equally to this manuscript.
- aging
- apoptosis
- oxidative stress
- polymerase chain reaction
- gene expression
- in situ hybridization
- immunohistochemistry
- renal function
- kidney failure, chronic
- hypoxia
- biopsy
- cadmium chloride
- genes
- metallothionein
- protein isoforms
- reactive oxygen species
- rna, messenger
- kidney
- cytotoxicity
- renal impairment
- creatinine tests, serum
- life span
- donors
Comments