-
PDF
- Split View
-
Views
-
Cite
Cite
Daniela Flores Gomez, Siroon Bekkering, Rob ter Horst, Benjamin Cossins, Inge C L van den Munckhof, Joost H W Rutten, Leo A B Joosten, Mihai G Netea, Niels P Riksen, The effect of leptin on trained innate immunity and on systemic inflammation in subjects with obesity, Journal of Leukocyte Biology, Volume 115, Issue 2, February 2024, Pages 374–384, https://doi.org/10.1093/jleuko/qiad118
- Share Icon Share
Abstract
Leptin is associated with cardiometabolic complications of obesity, such as metabolic syndrome and atherosclerosis. In obese men, the presence of metabolic syndrome is associated with higher circulating leptin and interleukin (IL)-6 concentrations and increased monocyte cytokine production capacity. Here, we investigated the effects of leptin on monocyte function and systemic inflammatory markers in obese individuals. We specifically explored whether leptin can induce long-term changes in innate immune function by inducing innate immune memory (also called trained immunity). We exposed human primary monocytes for 24 h to relevant leptin concentrations in vitro and measured cytokine production. In addition, after removing leptin, we incubated monocytes for 5 d in culture medium, and we restimulated them on day 6 to assess cytokine production capacity, phagocytosis, and foam cell formation. Direct stimulation with leptin did not induce cytokine production, but exposure to 50 ng/mL leptin augmented lipopolysaccharide- and R848-induced tumor necrosis factor α (TNF-α) production after 1 wk. In a separate in vivo study in a cohort of 302 obese subjects (body mass index [BMI] >27 kg/m2, 55 to 81 yr), we measured circulating leptin, inflammatory markers, and cytokine production upon ex vivo stimulation of isolated peripheral blood mononuclear cells. Circulating leptin concentrations positively correlated with circulating IL-1β and IL-6, which was more pronounced in men than in women. Four single nucleotide polymorphisms in the leptin gene influenced circulating IL-6 concentrations in men, suggesting a direct effect of leptin on IL-6. In conclusion, in vitro, leptin does not directly stimulate monocytes to produce cytokines, yet induces long-term monocyte hyperresponsiveness, i.e. trained immunity. In obese subjects, leptin is associated with circulating IL-6 in a sex-dependent manner. The underlying mechanisms of the sex-specific effect of leptin on innate immune cells remain to be further investigated.
1. Introduction
During the last decades, a large proportion of the world's population became overweight or obese leading to an increase in related cardiometabolic complications.1 Obesity is associated with low-grade systemic inflammation, and this contributes to its metabolic and atherosclerotic consequences.1,2 However, the mechanisms driving this association have not yet been fully elucidated.3
Atherosclerotic cardiovascular disease is a chronic, low-grade inflammatory disorder of the vascular wall, and its pathophysiology is strongly mediated by innate immune cells such as monocytes.4 Circulating monocytes are recruited to the vascular wall, where they differentiate into macrophages playing an important role in the formation and destabilization of atherosclerotic plaques.5 Obesity is associated with the activation of innate immune cells such as monocytes and macrophages.6 It has recently been described that monocytes can build a long-term hyperresponsive phenotype after brief exposure to endogenous atherogenic stimuli, including oxidized low-density lipoprotein (oxLDL),7 lipoprotein(a),8 and adrenal hormones.9,10 This de facto innate immune memory has been termed trained immunity and probably contributes to atherosclerotic cardiovascular disease development.11 Importantly, recent experimental studies have shown that obesity can also induce trained immunity, which persists after weight loss.12–14
Obesity is associated with an increased production of adipose tissue-related proinflammatory molecules, such as the adipokine leptin.15 While its main function is to regulate appetite, leptin is also involved in other physiological processes such as energy homeostasis, and metabolic and immune regulation.16,17
The concentrations of circulating leptin in the blood are directly proportional to the amount of adipose tissue and, generally, women have higher levels of circulating leptin than men.18 We recently explored the association between circulating leptin and the presence of metabolic syndrome (MetS) in individuals with overweight or obesity.19 We observed that in men, circulating leptin and interleukin (IL)-6 concentrations are higher in the presence of MetS, whereas in women, the presence of MetS is associated with lower anti-inflammatory adiponectin levels.19 Additionally, the production capacity of isolated peripheral blood mononuclear cells (PBMCs) for IL-6 and IL-1β was higher in men with MetS compared to overweight men without MetS.19
Based on these observations, we hypothesized that leptin contributes to the adverse metabolic and cardiovascular complications of obesity by inducing trained immunity in circulating innate immune cells.
In the present study, we performed a series of in vitro studies in isolated primary human monocytes using physiologically relevant concentrations of leptin. We subsequently explored the association between circulating leptin concentrations and markers of inflammation and cytokine production capacity of PBMCs in a cohort of obese individuals. These results help to understand the role of leptin in the regulation of inflammation in obesity.
2. Methods
2.1 Reagents
Human recombinant leptin was dissolved in 20 mM of Tris-HCl pH 8, according to the instructions of the manufacturer (R&D Systems). Toll-like receptor (TLR) agonists include lipopolysaccharide (LPS) for TLR4 (Sigma-Aldrich; Escherichia coli serotype 055:B5, further purified as described)20 and Pam3Cys for TLR2 (EMC micro-collections; L2000). Cells were cultured in RPMI 1640 Dutch modified culture medium supplemented with 50 µg/mL gentamycin (Centrafarm), 1 mM pyruvate (Invitrogen), 2 mM glutamine (Invitrogen), and 10% human pooled serum.
2.2 Preparation of oxidized low-density lipoprotein
oxLDL was prepared from LDL, which was previously isolated by density-grade ultra-centrifugation from EDTA blood from healthy volunteers. LDL was dialyzed in a Slide-A-Lyzer Dialysis Cassette, 10 K MWCO, 3 mL (Thermo Fisher Scientific) for 7 h in phosphate-buffered saline (PBS), refreshing the buffer after 1 and 3 h. LDL was then oxidized by incubation with 20 μM CuSO4, for 16 h in a heat block at 37 °C, 600 rpm followed by 1 h of dialysis in PBS as previously described.21 The protein concentration was measured with BCA Protein Assay Kit (Thermo Fisher Scientific).
2.3 Cell isolation
Human PBMCs and monocytes were isolated from the blood of healthy volunteers after written informed consent (Sanquin Bloodbank) as described previously.22 Briefly, PBMCs were isolated by density gradient centrifugation with Ficoll-Plaque PLUS (GE Healthcare Biosciences) followed by 3 washes with cold PBS. Monocytes were isolated from PBMCs by differential centrifugation using hyperosmotic Percoll solution (Sigma-Aldrich) and washed with cold PBS 1 time as described previously.22 Cells were resuspended in supplemented RPMI 1640 and counted using a Sysmex Hematoanalyzer XE5000.
2.4 Direct stimulation and in vitro training experiments
PBMCs were diluted to a concentration of 5 × 106/mL in supplemented RPMI. A total of 5 × 105 cells were plated per well in round-bottom 96-well plates and were stimulated for 24 h with RPMI only as the negative control, and 1, 50, and 100 ng/mL of human recombinant leptin. After 24 h, the plates were centrifuged and supernatants were collected and stored at −20 °C until further assessment.
Trained immunity was assessed using the well-established training protocol as described before.23,24 In brief, monocytes were diluted to a concentration of 1 × 106/mL in supplemented RPMI medium. Cells were plated on flat-bottom 96- or 6-well culture plates for 1 h, followed by a warm PBS wash to remove the nonadherent cells. This step allows Percoll-isolated monocytes to reach a purity of >95%, as previously reported.24 The monocytes were incubated with 10% human pooled serum and oxLDL (10 µg/mL), human recombinant leptin (1, 5, and 50 ng/mL), or both for 24 h, after which the cells were washed with warm PBS. The cells were then incubated for 6 d in medium containing RPMI + 10% human pooled serum. After this period, they were restimulated for 24 h with RPMI only, LPS (10 ng/mL), R848 (5 µg/mL), or Pam3Cys (10 µg/mL) to assess IL-6 and TNF-α; and LPS + Nigericin (10 ng/mL and 1 μM, respectively) to assess intra- and extracellular IL-1β. Following this incubation period, the plate was centrifuged and supernatants were collected. Additionally, 100 µL of 0.5% Triton X (Sigma-Aldrich) were added to the cells for intracellular measurements. All the material was stored at −20 °C until further assessment.
2.5 Cytokine measurements
IL-6 (DY201), TNF-α (DY210), and IL-1β (DY206) were measured in supernatants by enzyme-linked immunosorbent assay following the manufacturer's instructions (R&D Systems).
2.6 Flow cytometry
Monocytes were isolated using magnetic-activated cell sorting using a pan-monocyte isolation kit according to the manufacturer's instructions (purity >95%, data not shown) (Miltenyi Biotec). A total of 1 × 106 cells were plated per well in a 6-well culture plate following the trained immunity protocol described previously. Cells were stimulated with RPMI only, oxLDL (10 µg/mL), leptin (1 or 50 ng/mL), or both. After 6 d the cells were detached using Accutase solution (400 to 600 units/mL; Sigma-Aldrich), counted in a CASY cell counter (Omni Life Science), and used for flow cytometry analysis.
TLR2 and TLR4 expression was determined by flow cytometry. Trained macrophages were stained with monoclonal antibodies CD45, CD284, CD282 (TLR2), and live/dead stain FVS620 (Table 1) in buffer containing 1% bovine serum albumin (Sigma-Aldrich) and PBS for 30 min. After the incubation time, the cells were washed and resuspended in the same buffer to be measured with a CytoFlex flow cytometer (Beckman Coulter; RRID: SCR_017217) that underwent daily quality control. The gating strategy is shown in Supplementary Fig. 1, and gates were determined by fluorescence minus one method.25 In short, macrophages were gated based on CD45+ and side-scatter properties, then the selection continued based on TLR2+ or TLR4+. Median fluorescence intensity was assessed on TLR2- or TLR4-positive cells. Data were analyzed with Kaluza 2.1 software (Beckman Coulter; RRID:SCR_016182).
Flow cytometry panel used to measure the expression of TLR2 and TLR4 in trained macrophages at day 6.
Marker . | Fluorochrome . | Clone . | Manufacturer . | Cat# . | RRID# . |
---|---|---|---|---|---|
CD45 | BV510 | 30-F11 | BioLegend | 103137 | AB_2561392 |
CD284 (TLR4) | PE | HTA125 | BioLegend | 312806 | AB_2205002 |
CD282 (TLR2) | APC | W15145C | BioLegend | 392304 | AB_2721443 |
FVS620 (live/dead) | ECD | — | BD | 564996 | AB_2869636 |
Marker . | Fluorochrome . | Clone . | Manufacturer . | Cat# . | RRID# . |
---|---|---|---|---|---|
CD45 | BV510 | 30-F11 | BioLegend | 103137 | AB_2561392 |
CD284 (TLR4) | PE | HTA125 | BioLegend | 312806 | AB_2205002 |
CD282 (TLR2) | APC | W15145C | BioLegend | 392304 | AB_2721443 |
FVS620 (live/dead) | ECD | — | BD | 564996 | AB_2869636 |
Abbreviations: APC = allophycocyanin; BV = brilliant violet; ECD = phycoerythrin-Texas Red conjugate/electron coupled dye.
Flow cytometry panel used to measure the expression of TLR2 and TLR4 in trained macrophages at day 6.
Marker . | Fluorochrome . | Clone . | Manufacturer . | Cat# . | RRID# . |
---|---|---|---|---|---|
CD45 | BV510 | 30-F11 | BioLegend | 103137 | AB_2561392 |
CD284 (TLR4) | PE | HTA125 | BioLegend | 312806 | AB_2205002 |
CD282 (TLR2) | APC | W15145C | BioLegend | 392304 | AB_2721443 |
FVS620 (live/dead) | ECD | — | BD | 564996 | AB_2869636 |
Marker . | Fluorochrome . | Clone . | Manufacturer . | Cat# . | RRID# . |
---|---|---|---|---|---|
CD45 | BV510 | 30-F11 | BioLegend | 103137 | AB_2561392 |
CD284 (TLR4) | PE | HTA125 | BioLegend | 312806 | AB_2205002 |
CD282 (TLR2) | APC | W15145C | BioLegend | 392304 | AB_2721443 |
FVS620 (live/dead) | ECD | — | BD | 564996 | AB_2869636 |
Abbreviations: APC = allophycocyanin; BV = brilliant violet; ECD = phycoerythrin-Texas Red conjugate/electron coupled dye.
2.7 Phagocytosis assay
To assess changes in macrophage phagocytosis capacity, we used the trained immunity model and exposed monocytes to leptin (1 and 50 ng/mL) and oxLDL 10 µg/mL for 24 h. Monocytes were differentiated into macrophages for 6 d and subsequently, a phagocytosis assay was performed according to the instructions of the manufacturer (Cayman Chemical; 500209). In short, latex beads (rabbit IgC FITC) were added to the macrophages for 3 h. The cell nucleus was then stained with Hoechst blue and dead cells were stained with 4 μM ethidium homodimer and wheat germ agglutinin. The phagocytosis rate was quantified using R version 4.2.2 (R Foundation for Statistical Computing). Cell clusters were separated from the background in a greyscale image by blurring and brightening and adaptive thresholding. Cluster size was then measured in number of pixels allowing filtering of clusters to identify single cells. Identified clusters were converted to masks in the blue, green, and red channels to then measure median fluorescence intensity. The phagocytic rate in the macrophages trained with leptin or oxLDL was expressed as the fold increase compared to the uptake by the untrained RPMI control cells.
2.8 Foam cell formation
To determine the effect of leptin training on macrophage lipoprotein uptake, a foam cell formation assay was performed as described previously.7 Monocytes were trained for 24 h with leptin (1 and 50 ng/mL) and oxLDL 10 µg/mL and differentiated into macrophages. To induce foam cell formation, at day 6, macrophages were incubated with serum-free RPMI medium for 4 h. Then, cells were incubated with medium containing RPMI only or oxLDL 50 µg/mL for 24 h. The accumulation of lipids was then visualized by Oil Red O staining. We quantified the intracellular lipid droplets using the R package EBImage. In brief, cells were identified and isolated from the images, before re-analysis to identify droplets within the cells. Cell identification was performed by inverting the bright-field image, converting it to grayscale, brightening and blurring, and applying an adaptive threshold to identify foreground components. To maximize the area of the cells covered, and account for gaps, the foreground area was dilated, and all enclosed areas were filled to make a continuous cell. Each cell was then reprocessed in turn to identify droplets within, starting by reverting to bright field, brightening, adaptive thresholding, and finally quantification. To differentiate oil droplets from the bright halo (resulting from dilation of the cells) and nonstained areas in the cells, light transmittance was reduced to a 93% to identify the droplets with red stain in them. A schematic representation of the quantification can be found in Supplementary Fig. 2.
2.9 300-Obesity cohort description
To validate our in vitro data, we used data from the 300-Obesity (300-OB) cohort. This cohort consists of 302 obese individuals with BMI ≥27 kg/m2 between 55 and 82 yr of age, mostly of Western European background, and has been reported previously.19 Circulating leptin (R&D Systems), IL-6 (Sanquin; M9316), and IL-1β (R&D Systems; DY201) were measured in EDTA plasma using enzyme-linked immunosorbent assay according to the manufacturer's instructions. PBMCs were isolated from EDTA blood with density gradient centrifugation with Ficoll-Plaque PLUS (GE Healthcare Biosciences). A total of 5 × 105 PBMCs were stimulated for 24 h in 96-well round-bottom plates at 37 °C and 5% CO2 with RPMI as negative control and LPS in a low dose (1 ng/mL) and a high dose (100 ng/mL) as well as Pam3Cys (1 µg/mL). After the 24 h incubation period, supernatants were collected and stored at −20 °C until cytokine assessment. TNF-α (R&D Systems; DY210), IL-6 (Sanquin; M9316), and IL-1β (R&D Systems; DY201) cytokine production after stimulation was measured according to the instructions of the manufacturer.
2.10 300-OB cohort correlation analysis
Spearman correlation was calculated between leptin plasma concentration, circulatory cytokines, and cytokine production capacity of PMBCs in all the participants, and in groups stratified according to sex and MetS.
We corrected for age as a cofactor and for multiple testing using the Benjamini-Hochberg false discovery rate method. Adjusted P values ≤0.05 were considered statistically significant.
2.11 300-OB cohort single nucleotide polymorphism analysis
2.11.1 Genotyping
About half of the samples (n = 134) were genotyped using the Illumina HumanCoreExome-24 BeadChip Kit (later renamed to Infinium CoreExome-24 Kit). The other half of the samples (n = 168) were previously genotyped using the Illumina Infinium Omni-express chip because the volunteers had participated in another study,26 and the genotype data from this study were used. The 2 sets were merged, keeping only single nucleotide polymorphisms (SNPs) in common between the 2 datasets. After imputation (described below), principal component analysis was used to verify that there were no differences between the 2 batches. As an additional precaution, in all genetic analyses “chip origin” was used as a covariate. Out of the 302 total participants, 24 had to be excluded from genetic analyses due to data quality, familial relationships, or ethnicity. To prevent false positives, the final data were filtered for minor allele frequency (MAF) > 0.10, and all genotypes that are present should have at least 3 samples.
2.11.2 Data processing
The genotypes from the Illumina HumanCoreExome-24 BeadChip Kit were called using optiCall.27 A sex check was performed using the software package plink.28 Using plink, for both sets the minimum MAF was set to 0.001, and we excluded markers that failed the Hardy-Weinberg equilibrium test at a specified significance threshold of P value <1×10−4. Only SNPs with a 99% genotyping rate were included. The data from the Illumina Infinium Omni-express were then lifted from GrCh38 to GrCh37 using a variation of liftOver (https://github.com/sritchie73/liftOverPlink), the original liftOver being provided by University of California, Santa Cruz.29 The data were then aligned to the 1000 Genomes dataset (phase3_shapeit2_mvncall_integrated_v5a.20130502) using Genotype Harmonizer,30 set to consider 500 flanking variants. Finally, the 2 sets of genotypes were combined keeping only SNPs present in both sets and imputed using the Michigan Imputation Server selecting the Haplotype Reference Consortium reference panel hrc.r1.1.201631 and phasing using Eagle.32 The data were filtered for having a minimum MAF of 0.1, a minimum imputation R2 score of 0.3, and minimum output empirical R2 of 0.3 (as provided in the imputation results). Data were annotated using bcftools. By overlapping the data with the 1000 Genomes dataset and applying multidimensional scaling, we identified 5 participants that had non–Western European ancestry, and they were removed from further genetic analyses. Additionally, we checked for family relationships. If 2 or more individuals were related (PI_HAT threshold >0.6), we excluded all but 1 of these. In total, 9 people were excluded on this basis. We sequenced several genes for all 302 participants using an independent method. For 10 participants, all from the 168 volunteers that were sequenced in a previous study, their genetic data did not match between the SNP chip data and the second sequencing run. Therefore, these individuals were excluded from genetic analyses. This means that out of the 302 total participants, 24 had to be excluded from genetic analyses. To prevent false positives, the final data were filtered for MAF > 0.10 and all genotypes that are present should have at least 3 samples.
2.11.3 QTL analysis
All QTL analyses were performed using the MatrixEQTL package in the R programming language. This package constructs linear regression models for each combination of SNP (independent variable) and quantitative variable (dependent variable) separately. The quantitative variables (cytokine production capacity and plasma markers) were log transformed, and MetS, National Cholesterol Education Program criteria, chip origin, and sex were used as covariates. The National Cholesterol Education Program criteria define MetS as the presence of 3 or more of the following criteria: abdominal obesity, high blood pressure, high glucose levels, high triglyceride levels, and low high-density lipoprotein cholesterol.33 QTL results were filtered for correlated SNPs. For all SNPs with an R2 ≥0.8, only the most significant result was kept. Specifically, R2 values were calculated based on the SNP dosage values, and filtering always kept the most significant SNP out of any correlated set. Samples with missing values were excluded from each analysis independently.
2.12 Statistics
The Shapiro-Wilk test was performed to assess the normality of the data. Data did not follow a normal distribution, therefore only nonparametric tests were executed. For the QTL in vivo data, values were log-transformed for normalization and reduction of variability as described previously. In vitro data are presented as mean ± SEM and experiments were performed with at least 6 donors. Statistical significance was determined with Wilcoxon signed rank test using GraphPad Prism 9 (GraphPad Software). The 300-OB cohort data analysis was performed with IBM SPSS Statistics 25 and RStudio version 4.0.2. Two-sided P values below 0.05 were considered to be statistically significant. For the phagocytosis and foam cell formation experiments, we did not perform any statistical testing, as this was only done in monocytes from 3 donors.
3. Results
3.1 Short and long-term effects of leptin on monocytes
To study the direct stimulatory effect of leptin on monocytes, we exposed PBMCs to physiologically relevant leptin concentrations (1, 50, and 100 ng/mL) and measured the IL-6 and TNF-α concentrations in the supernatant. None of the leptin concentrations induced any cytokine production in PBMCs (data not shown).
Next, using the well-established trained immunity protocol,24 we explored whether a brief exposure of monocytes to leptin induces trained immunity (Fig. 1A). Therefore, we exposed monocytes for 24 h to leptin and assessed cytokine production capacity in response to other stimuli in the monocyte-derived macrophages 6 d later. Twenty-four-hour exposure to leptin dose-dependently augmented TNF-α production following LPS exposure on day 6, which was statistically significant for 50 ng/mL of leptin (Fig. 1C). There was no effect of leptin pre-exposure on LPS-induced IL-6 production (Fig. 1B), nor on Pam3Cys-induced cytokine production. In addition, we restimulated the cells with the TLR7/8 agonist R848. We found that 24 h exposure to leptin 50 ng/mL significantly augmented R848-induced TNF-α production (Fig. 1E). Finally, we also investigated whether leptin-trained macrophages produced more IL-1β by restimulating the cells with nigericin and LPS. Fig. 1F–H shows that total IL-1β production tends to increase after training with leptin (1 and 50 ng/mL) and upon restimulation with LPS + Nigericin; however, this increase was not statistically significant because of the large interindividual variability.
![Exposure of human primary monocytes during 24 h to human recombinant leptin and oxLDL induces trained immunity. (A) Schematic representation of the experimental setup. Human primary monocytes from healthy volunteers were exposed for 24 h to medium alone, human recombinant leptin (1 and 50 ng/mL), oxLDL (10 µg/mL), or leptin + oxLDL. After 6 d of resting, cells were restimulated with LPS, Pam3Cys, and R848, and we measured (B, D) IL-6 and (C, E) TNF-α (n = 11 [A, B], n = 6 [C, D]; *P < 0.05, ***P < 0.001). To induce the secretion of IL-1β, at day 6 cells were also restimulated with LPS + Nigericin. (F) Extracellular, (G) intracellular, and (H) total IL-1β were measured (n = 3). Created with BioRender.com.](https://oup.silverchair-cdn.com/oup/backfile/Content_public/Journal/jleukbio/115/2/10.1093_jleuko_qiad118/1/m_qiad118f1.jpeg?Expires=1748807957&Signature=krImr3AFqlxWOuzMhsnczKN4qes0RBV70wcBY24IdC-fUXyzM-Iqr7fifwghuZMC9bbCkbT2glstXrIFMaa7qvXg8GN-vke5OefSd53lQjZUxCv1DZRUjC8ZaaJY~555ZkGmEDsMOg6Rjxx9YPp3e5-ZpjV3CPSfqxSZEXpsGjQqQnul8oz0Z9cNqbn0upArDn4m5NFGdWjGjQV6zzAi-lJ2dts8ndq7kHxM2-ijSTFMKZWILamtmVoQi0ywkPIY3gf8hsYcXMe1jSFItbojMkK2mBjnQb9zDNAs8zxY4aWthPHD1yyQAeY91e0giXTjURJfYPPGAxcMe2EWZPyUmA__&Key-Pair-Id=APKAIE5G5CRDK6RD3PGA)
Exposure of human primary monocytes during 24 h to human recombinant leptin and oxLDL induces trained immunity. (A) Schematic representation of the experimental setup. Human primary monocytes from healthy volunteers were exposed for 24 h to medium alone, human recombinant leptin (1 and 50 ng/mL), oxLDL (10 µg/mL), or leptin + oxLDL. After 6 d of resting, cells were restimulated with LPS, Pam3Cys, and R848, and we measured (B, D) IL-6 and (C, E) TNF-α (n = 11 [A, B], n = 6 [C, D]; *P < 0.05, ***P < 0.001). To induce the secretion of IL-1β, at day 6 cells were also restimulated with LPS + Nigericin. (F) Extracellular, (G) intracellular, and (H) total IL-1β were measured (n = 3). Created with BioRender.com.
We subsequently determined whether the increased TNF-α production upon restimulation was due to an upregulation of TLR4/2 in the leptin-exposed macrophages. Flow cytometry analysis of monocyte-derived macrophages on day 6 after training showed no significant changes in the percentage of cells positive for TLR2 or TLR4, and the level of expression of the receptors on TLR4/2-positive cells did not change either (Supplementary Fig. 3), ruling out TLR upregulation as an explanation for the enhanced cytokine response.
3.2 Leptin exposure augments phagocytosis and foam cell formation in macrophages
To assess whether brief leptin exposure also affects other macrophage functions, we performed phagocytosis and foam cell formation assay in the macrophages. We observed that both oxLDL- and leptin-trained monocyte-derived macrophages showed a higher uptake of latex-beads after 3 hours, compared with the untrained control (Fig. 2A). To determine if there was higher lipoprotein uptake in leptin-trained macrophages, we incubated the cells for 24 h with 50 µg/mL of oxLDL or RPMI without oxLDL and performed an Oil Red O staining to identify the intracellular lipid droplets. Morphologically, the cells exposed to oxLDL and leptin are bigger with more lipid droplets compared to the untrained control (Fig. 2B, representative pictures). Automated quantification of the lipid droplets in each cell showed that for all 3 donors, the average number of lipid droplets per cell were higher in the leptin-trained cells than in the untrained macrophages (Fig. 2C).
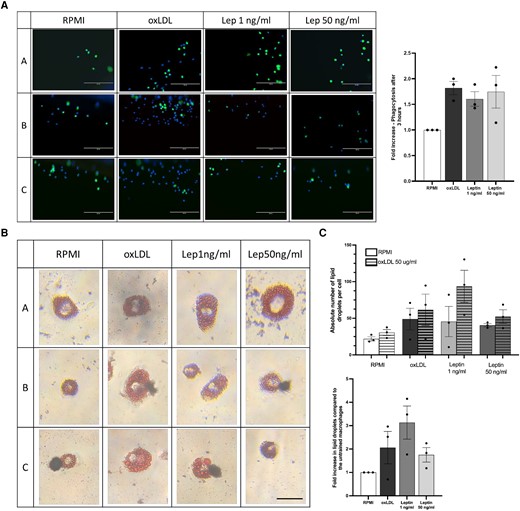
Training human primary monocytes with human recombinant leptin and oxLDL augments phagocytosis and foam cell formation. Human primary monocytes were exposed during 24 h to human recombinant leptin (1 and 50 ng/mL) or oxLDL 10 µg/mL. (A) After 6 d, the macrophages were exposed to fluorescent latex beads for 3 h and after washing images with the EVOS microscope were made to measure fluorescent beads uptake (10× magnification, scale bar = 200 μm). The uptake of beads in oxLDL and leptin-trained cells is expressed as fold change compared to the untrained control cells (n = 3). (B) Foam cell formation was induced by incubating the trained macrophages for 24 h with RPMI only or oxLDL 50 µg/mL followed by Oil Red O staining. (C) Foam cell morphology and lipid uptake quantification of trained macrophages was compared with untrained control (n = 3; scale bar = 25 μm).
3.3 Leptin does not modulate oxLDL-induced training in monocytes
It has been shown previously that brief exposure of monocytes to oxLDL induces a trained macrophage phenotype characterized by increased cytokine production capacity.7 Some endogenous stimuli such as glucose can enhance this effect,34 indicating a synergistic effects of specific metabolic stimuli. We studied whether coexposure of cells to leptin also modulates oxLDL-induced trained immunity. We stimulated human primary monocytes for 24 h with oxLDL (10 µg/mL) with or without co-incubation with leptin (1 and 50 ng/mL) (Fig. 1A). We confirmed that 24-hour oxLDL-exposure augments LPS-induced TNF-α and IL-6 production, and Pam3Cys-induced TNF-α production at day 6 compared to RPMI-exposed cells, but co-incubation with leptin did not potentiate this effect (Fig. 1B and C).
3.4 Circulating IL-6 and IL-1β are correlated to circulating leptin in obese patients in vivo
We subsequently aimed to explore whether the immunomodulatory effects of leptin we observed in vitro translate into differences in immune cell function in vivo. Therefore, we assessed the correlation between the leptin concentration and circulating proinflammatory cytokine and PBMC cytokine production after 24 h stimulation with TLR2/4 agonists in a cohort of 300 obese individuals (300-OB) (age and false discovery rate corrected) (Fig. 3). In this analysis, we also explored whether associations were influenced by sex and by the absence or presence of MetS. In our cohort, there was a significant correlation between the leptin concentration and circulating IL-1β concentrations in men but not in women and IL-6 in men and women. Moreover, correlations were only observed in individuals without MetS. There was no significant correlation between circulating leptin concentrations and cytokine production of isolated PBMCs.
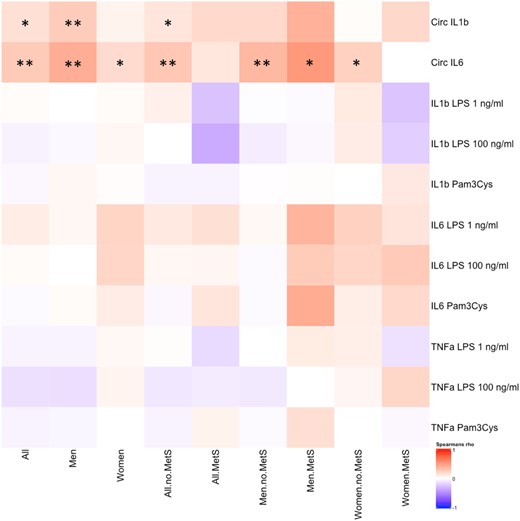
Heatmap showing Spearman correlations between circulating leptin and various circulating cytokines and cytokines produced by ex vivo stimulation of PBMCs in 302 obese individuals, either in the entire cohort or in subgroups, indicated on the horizontal axis. Spearman correlation was performed, and data are presented divided in columns for all individuals, men, and women with and without MetS. Cells colored in red indicate a positive correlation and in blue indicate a negative correlation. Age- and false discovery rate–corrected data, *P < 0.05, **P < 0.01.
3.5 Genetic variants in the leptin gene modulate circulating IL-6 levels in men
The strong association between leptin and IL-6 in the male participants of the 300-OB cohort could be driven by a direct effect of leptin and leptin receptor stimulation on IL-6 production, by an effect of IL-6 on leptin production, or by an independent factor that influences both leptin and IL-6 concentrations. To explore the causality of this association, we made use of genetic variants in the genes encoding for leptin (LEP), the leptin receptor (LEPR), and IL-6. We proposed that if leptin receptor stimulation directly affects the IL-6 concentration, functional SNPs in the leptin gene or its receptor gene would also affect the circulating IL-6 concentration. Similarly, we explored the effect of SNPs in the IL-6 gene on the circulating leptin concentration. Given the fact that the correlation between circulating leptin and IL-6 is restricted to males (Fig. 3), we performed the analysis in males and females separately.
We tested for associations between SNPs in the leptin gene (170 SNPs) and leptin receptor gene (182 SNPs) and the circulatory IL-6 concentration. We also tested for associations between SNPs in the IL-6 gene (153 SNPs) and circulatory leptin in obese males and females or both. The complete list of SNPs can be found in Supplementary Table 1. We identified 4 SNPs in the leptin gene that were associated at a suggestive threshold with circulating IL-6: variants rs896183 (located in LEP [P = 0.0041, b = −0.1117]), rs11772985 (located in LEP [P = 0.0086, b = −0.1048]), rs13237683 (located in LEP [P = 0.0138, b = −0.0974]) and rs12706830 (located in LEP [P = 0.0457, b = 0.1106]) (Fig. 4A). Interestingly, these associations were only present in men, echoing the male-specific associations between the circulating concentrations of leptin and IL-6 (Fig. 3). All variants were located in exonic regions of the gene as shown in Fig. 4B and C. Genotyped SNPs in the leptin receptor were not associated with circulating IL-6, and SNPs in IL-6 were not associated with circulating leptin.
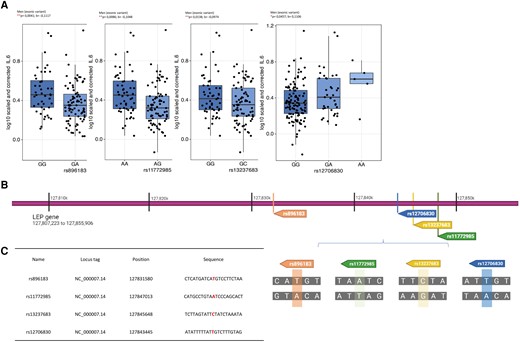
Four identified SNPs in the leptin gene have a significant effect on circulating IL-6 levels in obese men. (A) Boxplots of the exonic genetic variants in the leptin loci rs896183, rs11772985, rs13237683, and rs12706830 indicating the circulating IL-6 levels per genotype (300-OB cohort, n = 302). (B) Position and location of the SNPs in the leptin gene as well as (C) identification of affected nucleotide in the sequence. False discovery rate–corrected data, *P < 0.05, **P < 0.01. For the full list of identified SNPs, see Supplementary Table 1. GeneCards—the human gene database.35,36 Created with BioRender.com.
4. Discussion
In this study, we aimed to assess the effects of leptin on innate immune cell function and systemic inflammation in the context of obesity. We observed that, in vitro, relevant concentrations of leptin do not have a direct stimulatory effect on cytokine production of human primary PBMCs and monocytes. However, 24-hour exposure to leptin did augment TNF-α and IL-1β production after 6-day differentiation of monocytes into macrophages, which point to trained innate immunity. In addition, leptin-trained macrophages showed increased foam cell formation and increased phagocytic capacity. In vivo, in a cohort of 300 overweight and obese individuals, there was no association between circulating leptin and cytokine production of isolated PBMCs. Nonetheless, there was a strong positive association between circulating leptin and IL-6 and IL-1β concentrations. Genetic analyses suggest that the direction of this association is from leptin to IL-6. Importantly, this effect was mainly seen in men and not in women.
Leptin is an adipokine mainly produced in the adipose tissue and normal leptin levels in the circulation range from 1 to 100 ng/mL in obese individuals.37,38 Leptin has been suggested to contribute to the low-grade inflammation that accompanies obesity.17 Additionally, the proinflammatory effects of leptin have been proposed to contribute to the increased risk of autoimmune diseases in patients with obesity, including type 1 diabetes mellitus, multiple sclerosis, and rheumatoid arthritis.39,40 We recently reported profound sex differences in the association between leptin and metabolic dysregulation in obesity.19 In men, the presence of MetS was associated with higher circulating leptin and IL-6 concentrations and with higher PBMC cytokine production capacity, whereas in women, MetS was associated with lower adiponectin concentrations.19,41
Our in vitro studies showed that leptin, in physiological concentrations, does not directly activate PBMCs. This is in contrast to previous studies that reported that a brief exposure of PBMCs to high concentrations of leptin (1,000 ng/mL) can induce a higher expression of TNF-α and IL-6,42–44 although these concentrations are not in the physiological range. Our findings were corroborated in vivo, as there was no association between circulating leptin concentrations and PBMC cytokine production capacity. This suggests that a direct effect of leptin on monocytes cannot explain the previous observation that men with MetS have higher circulating leptin and increased PBMC cytokine production capacity.
In contrast, 24-h exposure of monocytes to leptin induced long-term changes in the function of monocyte-derived macrophages (also termed trained immunity). We have previously reported that various atherogenic endogenous molecules can induce trained immunity, including oxLDL and lipoprotein(a), glucose, and the adrenal hormones aldosterone and catecholamines.7–10,45 The hallmark of trained immunity is an augmented cytokine production capacity that persists even when the stimulus has long been removed.46 Indeed, we observed that 24 h exposure of monocytes to 50 ng/mL augmented the production of TNF-α in the monocyte-derived macrophages after restimulation with TLR4 and TLR7/8 agonists. We previously reported that oxLDL-trained macrophages showed increased foam cell formation,7 and we could now confirm that also for leptin-trained macrophages. It has previously been reported that exposure to leptin during the 7 days of monocyte-to-macrophage differentiation increased foam cell formation,47,48 and our study now demonstrates that this also holds true when only the monocytes are briefly exposed to leptin. Finally, we assessed phagocytosis capacity in the trained macrophages, since this is a crucial process involved in the development of atherosclerotic plaques49 We observed that leptin exposure appeared to increase the uptake of latex beads, as a measure for phagocytosis. In summary, we found that leptin-trained macrophages have an atherogenic phenotype, with increased cytokine production capacity, foam cell formation, and phagocytosis. In murine models of atherosclerosis, trained immunity can contribute to the development of atherosclerosis in the context of hyperglycemia50 and hyperlipidemia.51 This remains to be established for leptin-induced trained immunity. Interestingly, in mice, a limited period of obesity also induces trained immunity by reprogramming of hematopoietic myeloid progenitor cells, which results in persistent immune cell hyperresponsiveness also after returning to normal body weight.14 Stearic acid was identified as a possible factor triggering this trained immunity. Our results suggest that leptin could also contribute to this obesity-induced innate immune memory, although its effects are relatively small.
In our cohort of overweight and obese individuals, although there was no correlation between circulating leptin and PBMC cytokine production capacity, we did identify a strong correlation between circulating leptin concentrations and circulating IL-6 and IL-1β. A potential explanation is that leptin-trained monocyte-derived macrophages play a role in heightened production of IL-1β and IL-6 in vivo because these cytokines are mainly derived from tissue-resident macrophages, such as Kupffer cells.52,53 In addition, direct effects of leptin on macrophages have been previously described. Leptin can augment the phagocytic function of macrophages and their cytokine production capacity.54 More recently, Monteiro et al.55 reported that leptin augments LPS-induced proinflammatory cytokine production in murine macrophages.
The interrelation between leptin and inflammatory cytokines is complex, with leptin affecting cytokine production of immune cells and adipocytes, which can subsequently modulate leptin production by adipocytes.17 To gain insight into the causal directionality of the association between circulating leptin and IL-6, we performed a detailed analysis of SNPs in the genes encoding leptin and the leptin receptor, and IL-6. We identified 4 SNPs in the leptin gene that were significantly associated with IL-6 concentrations exclusively in men. This suggests that, in men, the association between leptin and IL-6 is driven by a direct effect of leptin on IL-6 production. More research is needed to elucidate the strong sex differences in the relation between leptin and inflammatory cytokines.
There are some limitations in our study. First, all our in vitro experiments were performed with PBMCs and monocytes from healthy volunteers, but we are not informed about the sex, weight, circulating leptin levels, or BMI of these individuals. Second, while we showed that leptin induced trained immunity in terms of augmented cytokine production capacity, we did not investigate the underlying epigenetic and metabolic processes that characterize trained immunity. Also, we did not investigate other time points than the 6 days after removal of the leptin. Third, the stratification of the cohort according to sex and MetS reduced the power of the cohort study This could have caused the lack of association in the subgroup of obese individuals with MetS. Finally, we have not confirmed that leptin-induced trained immunity occurs in vivo; this would allow a prospective follow-up of our cohort to compare subjects who lost weight to subjects with persistent obesity. Therefore, more research is needed to investigate the relevance and importance of leptin-induced trained immunity in mediating the obesogenic memory recently described.
In conclusion, these results increase our understanding of the inflammatory effects of leptin and highlight a crucial sex-specific mechanism; however, more research is needed to be able to understand how leptin mediates the inflammatory response in the tissue. Additionally, it is essential to study the intracellular mechanisms involved in this response.
Acknowledgments
The figures were created with BioRender.com.
Author contributions
D.F.G., S.B., J.H.W.R., L.A.B.J., M.G.N., and N.P.R. were responsible for conceptualization of the study. D.F.G., S.B., and I.C.L.M. performed the investigation (experiments). Data curation and analysis was performed by D.F.G., S.B., R.H., and B.C. Project oversight was done by S.B. and N.R. D.F.G. and S.B. wrote the draft manuscript, which was afterward reviewed and edited by all coauthors.
Supplementary material
Supplementary materials are available at Journal of Leukocyte Biology online.
Funding
J.H.W.R., L.A.B.J., M.G.N., and N.P.R were supported by a CVON grant from the Dutch Heart Foundation and Dutch Cardiovascular Alliance (DCVA; CVON2018-27). N.P.R. was further supported by a grant of the ERA-CVD Joint Transnational Call 2018, which is supported by the Dutch Heart Foundation in the Hague (JTC2018, project MEMORY; 2018T093). S.B. was supported by the Dutch Heart Foundation in the Hague (Dekker grant 2018-T028). M.G.N. was further supported by a European Research Council Advanced Grant (FP/2007-2013/ERC grant 2012-322698) and a Spinoza Prize by NWO (NWO SPI 92-266).
References
Author notes
Conflict of interest statement. None declared.