-
PDF
- Split View
-
Views
-
Cite
Cite
Xiang-Jing Chen, Cheng Liu, Shan Zhang, Li-Feng Zhang, Wei Meng, Xin Zhang, Meng Sun, Yue Zhang, Ren-Zhong Wang, Cheng-Fang Yao, ILC3-like ILC2 subset increases in minimal persistent inflammation after acute type II inflammation of allergic rhinitis and inhibited by Biminkang: Plasticity of ILC2 in minimal persistent inflammation, Journal of Leukocyte Biology, Volume 112, Issue 6, December 2022, Pages 1445–1455, https://doi.org/10.1002/JLB.3MA0822-436RR
- Share Icon Share
Abstract
Minimal persistent inflammation (MPI), the local inflammation that occurs after an acute type II immune response in patients with allergic rhinitis (AR), is responsible for airway hyperreactivity and the recurrence of AR. Innate lymphoid cells (ILCs) play a crucial role in mucosal immune homeostasis, but the changes of ILC subsets in the MPI stage remain unclear. In this study, the levels of ILC-secreting cytokines in nasal lavages were analyzed from 19 AR patients and 8 healthy volunteers. AR and MPI model mice were established to study the ILC subsets. The results showed that IL-17A was significantly increased in nasal lavage of AR patients in the MPI stage by MSD technology. When compared with the AR model mice, the frequency of IL-13+ILC2 in the nasal mucosa and lungs decreased, while IL-5+ILC2 remain high in MPI model mice. A part of the IL-5+ILC2 subset displayed ILC3-like characteristics with elevated RORγt, IL-17A and IL-23R expression. Especially, these ILC3-like ILC2 exhibited up-regulation of GATA3+RORγt+ were increased in MPI model mice. After the treatment of Biminkang, the frequencies of IL-5+ILC2, IL-17A+ILC3, and GATA3+RORγt+ILC3-like ILC2 were significantly reduced, and IL-23R expression was also decreased on ILC3-like-ILC2 subset. These results suggested that the elevated IL-17A in the MPI stage has been related to or at least partly due to the increased of ILC3-like ILC2. Biminkang could effectively decrease IL-17A+ILC3 and inhibit ILC3-like ILC2 subset in the MPI stage. Biminkang is effective in administrating MPI by regulating airway ILC homeostasis.
INTRODUCTION
Environmental factors in industrialized cities have triggered an increase in the prevalence of allergic rhinitis (AR).1 AR, one of the most common airway allergic diseases, has affected 10–40% of the world's population.2 The AR and its impact on asthma (ARIA) working group of WHO emphasized that AR emerged as a global health issue. AR had an impact on the patients’ quality of life. Furthermore, a higher proportion of AR patients have nonspecific bronchial hyperreactivity. Asthma prevalence is higher in patients with AR than in subjects without AR. The concept “one airway one disease” raises the awareness of the treatment of AR.3 What makes the doctors and patients distressed during the treatment of AR is the recurrence, and the symptoms such as water rhinorrhea, sneezing, nasal obstruction, and pruritis are getting more and more serious.
“Minimal persistent inflammation (MPI)” is a subclinical inflammatory state that is caused by subthreshold doses of the allergen. MPI appears before and/or after the onset of the AR.4,5 It is mainly believed that subthreshold doses of the allergen can cause the eosinophil infiltration and the release of inflammatory factors, such as ECP, ICAM-1, and LTs in the nasal mucosa. According to the existing studies, the presence of MPI can be detected 4–6 weeks or more after the onset of the AR,6,7 and there was a persistent interaction between eosinophils and ILC2s.
Innate lymphoid cells (ILCs), a type of tissue-resident cells, is recognized for their crucial role in mucosal immunity, tissue repair, and remodeling. The cytokine and function of ILC subsets are similar to that of Th cell subsets. ILCs and Th cells are the sources of IFN-γ, IL-4, IL-5, IL-9, IL-13, and IL-17A. However, ILCs are tissue-resident cells that can respond to allergen attacks faster and play a larger role in the persistence of local inflammation than Th cells. In the patients, suffering from allergic airway disease, ILC2 seems to be a major component response to allergen challenges.8 The allergen attacks the epithelial cells, fibroblasts, and macrophages causing the produce of IL-25, IL-33, TSLP (thymic stromal lymphopoietin, TSLP), and TGF-β, all of which can activate ILC2. ILC2 activation can result in the production of Th2-type cytokines such as IL-4, IL-5, IL-9, and IL-13.9 IL-4 and IL-13 can activate dendritic cells, and then enhances Th2 maturation and activation. Th2 can amplify the activation, resulting in acute allergic reactions.9–11 However, with the research on ILCs, the plasticity of ILCs is reported. The plasticity allows the ILCs to bypass the differentiation of progenitor cells and produce a rapid immune response possible. Currently, a subset of ILC2 with IL-17A+ILC3-like characteristics has been identified and is thought to be pathologic in allergic airway disease.12,13 Thus, it is reasonable to speculate that these types of conversion might also trigger or contribute to certain inflammatory states, such as MPI.14
At present, the intranasal corticosteroids, antihistamines, and antileukotrienes are used for the treatment of MPI.15 However, considering the safety of long-term administration, the application of these drugs seems to be questioned. As a result, finding safe and effective therapeutic drugs for MPI remains a priority. Biminkang is a mixture of traditional Chinese herbal medicine, which contains Huangqi (Astragalus membranaceus), Mahuang (Ephedra), Guizhi (Cinnamomum cassia Presl), Baishao (Paeonia lactiflora Pall), Xixin (Asarum), Ganjiang (Zingiber officinale Rosc), Fangfeng (Saposhnikovia divaricate Schischk), Wumei (Prunus mume), Chantui (Cryptotympana pustulata Fabricius), Dilong (Pheretima vulgaris), and so on. Our previous study found that Biminkang could reduce the symptoms of AR, but it is unclear whether it can effectively regulate ILCs homeostasis in the MPI stage.
Here, we tested the nasal lavage of AR patients and found that the level of IL-17A in the MPI stage was increased compared with the early stage of MPI. Based on previous research, we suggested that there might be an imbalance of ILCs subsets in the local tissues of the MPI stage, and the imbalance was manifested as the advantage of IL-17A+ILC3. In this study, the ILC1, ILC2, and ILC3 subsets were detected and the mechanism for the imbalance of the ILC subsets were initially explored. Furthermore, Biminkang, an effective traditional Chinese medicine for AR, was used to intervene and treat the ILC imbalance in the MPI stage.
MATERIALS AND METHODS
Human nasal lavage and mice samples
Experiments using human nasal lavage from AR patients and normal volunteers were approved and guided by the Ethics Committee of Affiliated Hospital of Shandong University of Traditional Chinese Medicine. AR was defined according to the typical symptoms of water rhinorrhea, sneezing, nasal obstruction, and pruritis, as well as the positive skin prick test. Six to eight-week-old BALB/c female mice were purchased from Jinan Pengyue Experimental Animal Breeding Corporation. All mice were housed in a temperature-controlled room with 12 h light–dark cycles and were maintained under specific pathogen-free conditions. All mice were allowed 1 week of acclimatization before the experiment. All the experiments involving mice were approved by the Animal Ethics Committee of Affiliated Hospital of Shandong University of Traditional Chinese Medicine and under the guidance of the Institutional Animal Care and Use Committee of Affiliated Hospital of Shandong University of Traditional Chinese Medicine.
Human nasal lavage procedure and measurement of the cytokines level
Before and during the study, none of the AR patients and volunteers received intranasal corticosteroids or systemic corticosteroids therapy. A modified Greiff and Gruénberg method was performed using a 16FR dual-lumen silicone urinary catheter to achieve high-quality nasal lavage.16 Before performing nasal lavage, the tip of the urinary catheter was cut off at the distal end of the balloon, leaving the balloon intact. To better assess the dynamic changes of cytokines in AR, AR patients had been performed nasal lavage for 3 times, respectively at the onset of AR, the early stage of MPI (MPI-E, within 1 week after the symptoms disappeared, the transition stage from AR to MPI) and MPI stage (within 3 weeks after the symptoms disappeared). Healthy volunteers only performed once.
The patient was instructed to clear the nasal cavity of excess secretions. In the writing position, nearly 1.5 cm catheter was inserted into the frontal nasal cavity (toward the outside of the nasal cavity instead of the nasal septum). The balloon was inflated to hold it in place. Five milliliters of preheated isotonic saline was instilled from the other end of the catheter. The urinary catheter was closed with hemostatic forceps in the position close to the nasal cavity to prevent the lavage fluid from flowing out. After 5 min, the hemostatic forceps were removed, then the saline and mucus were recovered into a 15 ml centrifuge tube. And the process was repeated in the other nostril. The nasal lavage was filtered with double gauze to remove mucin. Next, the nasal lavage was centrifuged at 800×g for 15 min, and the supernatant was aliquoted (1.0 ml) and stored at −80°C until measured.17
The levels of IFN-γ, IL-4, IL-5, IL-13, and IL-17A were measured by the MSD electrochemiluminescence, with the Human U-PLEX Biomarker Group Assays from Meso Scale Discovery (MSD, USA). According to the standard manufacturer's protocol, the standard and sample were added to the MSD plate and then incubated for 2 h. After the washing process, the detection antibodies were added and incubated for another 2 h. The unbound antibodies were washed off, and the plate reading solution was added. Afterwards, MSD plates were analyzed on the MSD Meso QuickPlex SQ 120.
AR and MPI model mice and treatment
Establishing the AR and MPI model mice. The AR and MPI model mice were sensitized intraperitoneally on days 1, 8, and 15 with 200 μl sensitization solution. The sensitization solution was OVA 20 μg and 2.25 mg aluminum hydroxide (all from SIGMA, USA) emulsified in a total volume of 200 μl PBS. The AR and MPI model mice were challenged daily with OVA (1% in PBS) via the airways for 20 min from 22 to 28 days. Subsequently, the AR model mice were challenged daily with 1% OVA and the MPI model mice were challenged daily with 0.01% OVA from the 29 days. In the Control group, OVA was replaced with PBS.
The administration was performed from the 29 days (challenging with different OVA concentrations). Biminkang was given once a day by gavage at a dose of 9.1 ml/kg. Loratadine tablets were broken and suspended in distilled water before being gavaged once daily at a dose of 15.15 mg/kg. In the Control group, drug was replaced with distilled water. After 14 days, mice were sacrificed 24 h after the final challenge for the nasal mucosa and lungs. The AR and MPI model mice and treatment protocols was showed in Figure 1.
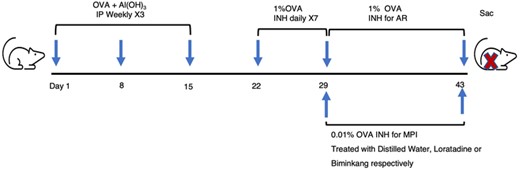
AR and MPI model mice and treatment protocols. BALB/c mice were sensitized weekly with OVA + Al(OH)3 for 3 times. One week later, mice were inhaled with 1% OVA for 7 days to establish AR model. Then, MPI model mice were inhaled with 0.01% OVA and AR model mice were inhaled with 1% OVA for 14 days. Meanwhile the MPI mice were treated with distilled water, Loratadine or Biminkang for 14 days respectively. IP, intraperitoneal injections. INH, inhaled
Quantitative real-time PC
The suspension of lungs from mice was prepared with a high-speed disperser. The supernatant was collected after centrifugation. Next, total mRNA was extracted with TaKaRa MiniBEST Universal RNA Extraction Kit, and mRNA was transcribed to cDNA with the use of PrimeScript™ RT Master Mix. QPCR was performed in a LightCycler 96 using TB Green® Premix Ex TaqTM II (all from TaKaRa Bio Incorporation, Japan). GAPDH gene was used as a housekeeping gene. The 2−ΔΔCT method was utilized to quantitatively investigate the data relative to Control group. ΔΔCT was determined as (CTsample – CTreference). Sequences of the primers used were as follows: GAPDH, forward: TGTGTCCGTCGTGGATCTGA, reverse: CCTGCTTCACCACCTTCTTGAT, IL-4, forward: TCACAGCAACGAAGAACACCA, reverse: CAGGCATCGAAAAGCCCGAA, IL-12, forward: TGTGGGAGAAGCAGACCCTT, reverse: CCCTGTTGATGGTCACGACG, IL-1β, forward: GTGTCTTTCCCGTGGACCTT, reverse: AATGGGAACGTCACACACCA, IL-23, forward: TGGAGCAACTTCACACCTCC, reverse: GGCAGCTATGGCCAAAAAGG, TGF-β, forward: AGCTGCGCTTGCAGAGATTA, reverse: AGCCCTTATTCCGTCTCCT.
ELISA
The lungs from all mice were detected the weight and homogenized with PBS. The supernatants were collected for detecting the expression of IL-4, IL-12, IL-1β, IL-23, and TGF-β. Supernatants were diluted 1:4 with sample dilution and measured by ELISA according to the manufacturer's instructions (MEIMIAN, China). The absorbance was read at 450 nm with a microplate spectrophotometer.
Cell isolation and flow cytometry
The nasal mucosa and lungs from all mice were diced and treated into single cell suspension by digestion solution with the gentleMACS Dissocitiator with Heater. The digestion solution contained 1 mg/ml collagenase D, 1 mg/ml Dispase II, and 0.35 μl DNase Ⅰ (all from SIGMA). The cells were washed, filtered with a 70-mm cell strainer, and purified with red blood cell lysis buffer.
Up to 2 × 106 cells/well were stimulated with 30 g/ml PMA, 100 ng/ml ionomycin, 10 μg/ml Brefeldin A (all from SIGMA), for 5 h in a cell incubator at 37°C. Fc block antibody was employed before the surface and intracellular staining. 7AAD (BD Biosciences, USA) was used to exclude dead cells, CD45 (clone 30-F11; Biolegend), CD127 (clone SB/199; BD Biosciences), and lineage (clone 145-2C11, RB6-8C5, RA3-6B2, Ter-119, M1/70; Biolegend) were used to identify ILCs. The anti-mouse lineage cocktail, which included anti-mouse CD3e, Ly-6G/Ly-6C, CD11b, CD45R/B220, TER-119/Erythroid cells, had been designed to exclude T lymphocytes, B lymphocytes, macrophages, granulocytes, NK cells, and erythrocytes.
The Fixation/Permeablization Kit (BD Biosciences) or eBioscience™ Foxp3/Transccription Factor Staining Buffer Set (Invitrogen, USA) was used for cell permeabilization, staining, and subsequent washing. The cells were stained with antibodies against IFN-γ (clone XMG1.2; Biolegend), IL-5(clone TRFK5; Biolegend), IL-13(clone eBio13A; Invitrogen), IL-17A (clone TC11-18H10.1; Biolegend), RORγt (clone Q31-378; BD Biosciences), and GATA3 (clone 16E10A23; Biolegend). The receptors of ILCs were stained with IL-23R (clone 12B2B64; Biolegend) and TGF-β RII (Catalog FAB532C; R&D Systems). 100,000 events were acquired using BD FACS Aria™ III. Collected data were analyzed using FlowJo software.
Statistical analysis
Statistical analysis was performed using GraphPad Prism 8. Statistical comparisons between 2 groups were performed using Mann–Whitney U test. Differences between 3 groups were performed using Kruskal–Wallis test (non-normal distribution) and followed by Dunn’s multiple comparisons test. A value of p < 0.05 was considered statistically significant.
RESULTS
High levels of IL-17A in nasal lavage of MPI stage in AR patients
We detected IFN-γ, IL-4, IL-5, IL-13, and IL-17A in the nasal lavage of AR patients and healthy volunteers. The detections were performed at the onset of AR, the early stage of MPI (MPI-E), and MPI stage. The results showed that the levels of IL-4, IL-5, and IL-13 in the nasal lavage of AR patients were significantly higher than that of the healthy volunteers, while the levels of IFN-γ and IL-17A were not different (Figure 2A). Along with the disappearance of allergic symptoms, expressions of IL-4, IL-5, and IL-13 in nasal lavage were decreased in MPI-E and MPI stage compared with AR. The expressions of IL-4 and IL-13 were down-regulated to the basal levels, while the IL-5 were still at a higher level at the MPI-E and MPI stage compared with healthy volunteers. The level of IFN-γ and IL-17A were decreased in the MPI-E stage and then increased during the MPI stage. The results were shown in Figures 2B and C.
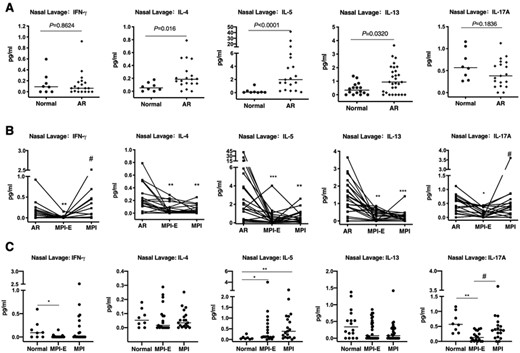
Cytokine levels of nasal lavage. (A) Cytokine levels of nasal lavage from AR patients (n = 19, n = 29) and healthy volunteers (n = 8, n = 16). (B) Cytokine levels of nasal lavage in different stages of AR patients. AR, at the onset of allergic rhinitis. MPI-E (the early stage of MPI, within 1 week after the symptoms disappeared). MPI (MPI stage, within 3 weeks after the symptoms disappeared). (C) Cytokine levels of nasal lavage in MPI stage and healthy volunteers. Horizontal bars represent median values. *Compared with AR, *p < 0.05, **p < 0.01, ***p < 0.001. # Compared with MPI-E, #p < 0.05
The imbalance of ILCs in AR and MPI model mice
We established the OVA-induced AR model mice and MPI model mice to evaluate the alteration of ILCs from airway. The nasal mucosa and lung cells from Control, AR, and MPI model mice were isolated, and 7AAD–CD45+CD127+Lineage–lymphocytes were identified as ILCs, the gating strategy was shown in Figure 2A. The frequencies of IFN-γ+ILC1, IL-5+ILC2, and IL-13+ILC2 were increased in the AR model mice when compared with the Control group. When compared with the AR model mice, the frequency of IL-13+ILC decreased in the MPI model mice, but not the frequency of IL-5+ILC2. Meanwhile, the frequency of IL-17A+ILC3 increased significantly in the MPI model mice (Figures 3B–E).
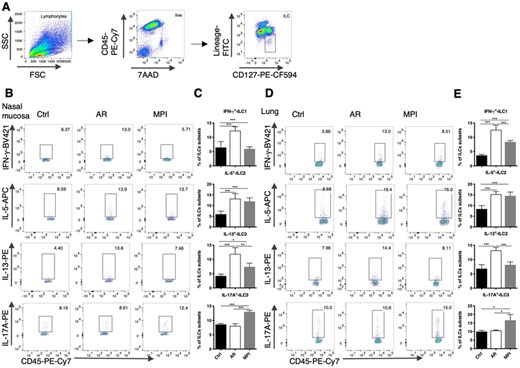
Altered ILCs in the nasal mucosa and lungs in model mice. (A) The gating strategy for the identification of ILCs. ILCs was identified as 7AAD–CD45+CD127+Lineage–. (B) Dot blots of ILC subsets in the nasal mucosa of Control, AR, and MPI model mice. (C) Frequencies of ILC subsets in the nasal mucosa of Control, AR, and MPI model mice. (D) Dot blots of ILC subsets in the lungs of Control, AR, and MPI model mice. (E) Frequencies of ILC subsets in the lungs of Control, AR, and MPI model mice. n = 6, data represent 2 independent experiments, mean ± sd, *p < 0.05, **p < 0.01, ***p < 0.001
The increased ILC3-like ILC2 in the MPI model mice
To determine whether the increase of IL-17A+ILC3 was the result of proliferation or circulating migration, we detected the frequency of total ILC, which was gated as 7AAD–CD45+CD127+Lineage– cells. There was no significant difference in the frequency of total ILC cells in the nasal mucosa or the lungs of the model mice (Figures 4A and B). The frequency of hybrid IL-5+IL-17A+ILC3-like ILC2 and GATA3+RORγt+ILC3-like ILC2 were increased compared with the Control group and the AR group (Figures 4D and E), while the frequency of IFN-γ+IL-17A+ILC did not change (Figure 4C). And we also found the change of IL-13+IL17A+ILC was not obvious (Figures S2 A, B). Both in the nasal mucosa and the lungs, the IL-5+IL-17A+ILC3-like ILC2 subsets took up nearly 30% of the IL-17A+ILC3.
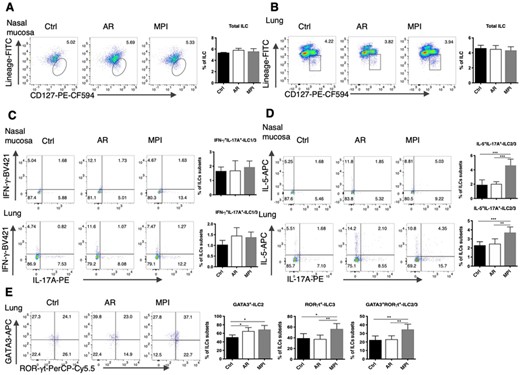
The increased ILC3-like ILC2 in MPI model mice. (A) Dot blots and frequencies of total ILCs in the nasal mucosa from Control, AR, and MPI model mice. (B) Dot blots and frequencies of total ILCs in the lungs from Control, AR, and MPI model mice. (C) Dot blots and frequencies of IFN-γ+IL-17A+ILC1/3 in the nasal mucosa and lungs. (D) Dot blots and frequencies of IL-5+IL-17A+ILC2/3 in the nasal mucosa and lungs. (E) Dot blots and frequencies of GATA3+ILC2, RORγt+ILC3, and GATA3+RORγt+ILC2/3 in the lungs. n = 6, data represent 2 independent experiments, mean ± sd, *p < 0.05, **p < 0.01, ***p < 0.01
The increased IL-23R in ILC3-like ILC2 in MPI
The gene transcription and protein production of IL-4 in the MPI model mice was reduced when compared with the AR model mice, according to this study. While there was no significant difference in IL-1β level. We discovered that IL-23 level was increased in MPI model mice compared with AR model mice. Meanwhile, the level of TGF-β was not changed, and the level of IL-12 was decreased (Figures 5A and B). The frequencies of IL-23R+IL-5+ILC2 and TGF-β RII+IL-5+ILC2 were tested. The frequency of IL-23R+IL-5+ILC2 was increased in the MPI model mice compared with the Control and AR model mice. The frequency of TGF-β RII+IL-5+ILC2 was reduced both in the AR mode mice and the MPI model mice compared with the Control group (Figures 5C and D). We further analyzed the frequencies of IL-5 and IL-17A expressed in the IL-23R+ILC subsets. And it was found that the IL-23R+IL-5+IL-17A+ILC3-like ILC2 was significantly higher in the MPI model (Figures 5E and F).
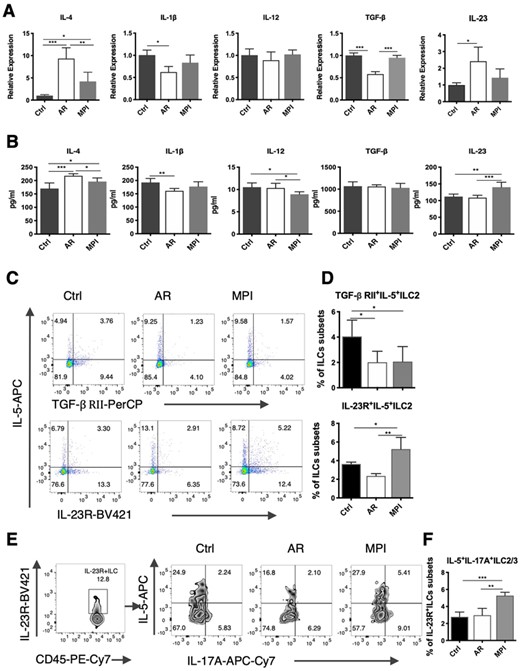
The increased IL-23R on ILC3-like ILC2 subsets in the lungs from MPI model mice. (A) mRNA Relative expression of IL-4, IL-1β, IL-12, TGF-β, and IL-23 in the lungs from Control, AR, and MPI model mice. (B) The level of IL-4, IL-1β, IL-12, TGF-β, and IL-23 in the lungs from Control, AR, and MPI model mice. (C) Dot blots of TGF-βRII+ILC2 and IL-23R+ILC2 subsets in the lungs. (D) Frequencies of TGF-βRII+IL-5+ILC2 and IL-23R+IL-5+ILC2 subsets in the lungs. (E) Zebra blots of IL-5+IL-17A+ILC3 after gated by IL-23R+ILC subsets in the lungs. (F) Frequencies of IL-5+IL-17A+ILC3 after gated by IL-23R+ILC subsets in the lungs. n = 6, data represent 2 independent experiments, mean ± sd, *p < 0.05, **p < 0.01, ***p < 0.001
Biminkang inhibited the ILC3-like ILC2 in the MPI model mice
Loratadine was treated as the positive control drug to observe the effects of Biminkang. After 2 weeks of the Biminkang or Loratadine treatment, the frequencies of IL-5+ILC2, IL-17A+ILC3, and IL-17A+ILC3-like ILC2 were reduced compared with the MPI model mice (Figures 6A and B). Both Biminkang and Loratadine could reduce the frequencies of GATA3+ILC2, RORγt+ILC3, and GATA3+RORγt+ILC3-like ILC2 in the lungs (Figure 6C). And there was no difference between the Biminkang and the Loratadine groups. Surprisingly, it was found that the frequencies of IL-23R+IL-5+ILC2 and IL-23R+IL-5+IL-17A+ILC3 were reduced after Biminkang and Loratadine treatment (Figures 6D and E).
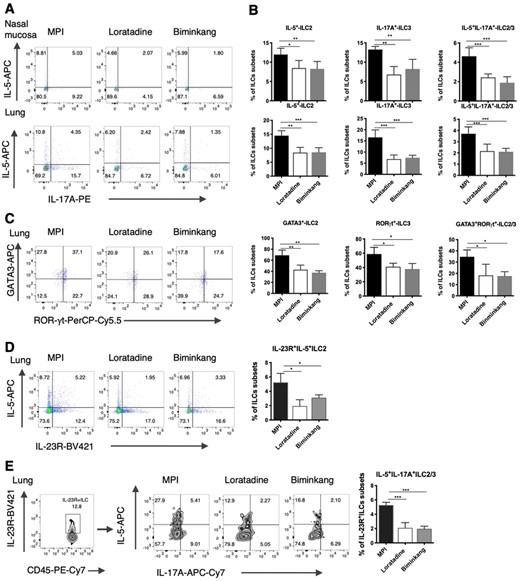
Biminkang inhibited the conversion of ILC3 from ILC2 in MPI model mice. (A) Dot blots of IL-5+ILC2, IL-17A+ILC3, and IL-5+IL-17A+ILC3 subsets in the nasal mucosa and lungs after treatment. (B) Frequencies of IL-5+ILC2, IL-17A+ILC3, and IL-5+IL-17A+ILC3 subsets in the nasal mucosa and lungs after treatment. (C) Dot blots and frequencies of GATA3+ILC2, RORγt+ILC3, and GATA3+RORγt+ILC2/3 in the lungs. (D) Dot blots and frequencies of IL-23R+IL-5+ILC2 subsets in the lungs. (E) Dot blots and frequencies of IL-5+IL-17A+ILC3 subsets after gated by IL-23R+ILC subsets in the lungs. n = 6, data represent 2 independent experiments, mean ± sd, *p < 0.05, **p < 0.01, ***p < 0.001
DISCUSSION
Although the pathogenesis of allergic airway disease was becoming more and more clear, the MPI stage remained unknown. Therefore, this study focused on the different stages in AR patients and found that some ILC2 persisted long after the symptoms disappearing and acquired ILC3-like phenotype. We believed that this might be a vital reason for the persistence of local inflammation in the MPI stage.
As local resident cells of mucosal tissue, ILCs had 100–200 times stronger secretion abilities than Th cells.18 The major producers of IL-17A in the lungs of mice were ILC3 cells, although some Lineage+ cells (such as CD4+Th17 cells and γδT cells) also produced IL-17A.13 To clarify the alteration of ILCs in airway tissue, ILC subsets were detected in the nasal mucosa and lungs from the AR and MPI model mice. It was an interesting phenomenon that IL-13+ILC2 decreased as expected in the MPI model mice, whereas the IL-5+ILC2 maintain a high level. Like Th2 cells, ILC2 could obtain memory-like characteristics when stimulated by allergens and persisted for a long time. Some ILC2 would even last longer than 4–6 weeks.10,19 Moreover, studies had found that this memory ILC2 showed a stronger capability than pure ILC2 to induce severe allergic inflammation.
Although ILC2 was critical to the onset of allergic airway disease, IL-17A+ILC3 caused the continuation of chronic inflammation in the later stage. Of course, γδ-T cells and NKT cells might also be involved in the chronic inflammation, the characteristics of the tissue residence permitted ILC3 to have a better advantage.20 In the absence of adaptive immunity, IL-17A produced ILC3 could induce airway hyperresponsiveness in lungs. Previous studies had found that compared with childhood asthma, adult asthma was characterized by more severe symptoms. The gene map of nasal brushing, sputum, and endobronchial brushings of adult patients showed more ILC3 gene signatures than in childhood.21 In other words, ILC3 was associated with chronic inflammation and high hyperresponsiveness, which was significantly consistent with the characteristics of MPI. So, we considered that the elevated IL-17A+ILC3 had been related to or at least partly accounted for MPI.
The increased ILC3 was thought to be the result of 3 factors: proliferation, migration, or conversion from ILC1 and ILC2. The frequencies of ILCs in the airway of the AR and MPI model mice changed insignificantly when compared with the Control group. It implied that the increased ILC3 could not come from proliferation or migration. It had been reported that ILCs were tissue-resident cells, and the ability of ILCs from the hematopoietic system to migrate into tissues was limited.22 Progenitors differentiated towards an ILC1, ILC2, or ILC3 subsets. To flexibly respond to the changes in the environment, as natural immune cells, ILCs were allowed to convert between subsets bypass the progenitor cells, and achieved the phenotype and secretory function of another subset.23 Studies had shown that ILC subsets transformed under certain conditions often have the phenotypic markers of the 2 subsets simultaneously and exist as a hybrid.24 This study indicated that the frequency of hybrid ILC3-like ILC2 (ILC2/3) of MPI model mice was increased, while the ILC3-like ILC1 (ILC1/3) was not, it meant that ILC3 was transformed from ILC2 rather than ILC1. Previous studies reported that ILC2 was functionally unstable, especially when they were stimulated, the cytokine production model was plasticity, as determined by the environmental.25 Compared with the Control group, the ILC3-like ILC2 was found to increase by 1.2–1.6 times, while IL-5–ILC3 only increased by 0.4–0.8 times in nasal mucosa and lungs.
This study speculated that in the MPI stage, the local inflammatory environment changed with Th2-type inflammation subsided, ILC2 transformed into ILC3-like subsets. The ILC3-like subsets might persist in local tissues for a long time, leading to chronic inflammation. Previous studies have found that ILC3 transformed from “ex-ILC2” had the potential to be transformed back into ILC2.26 When attacked by allergens, they could be rapidly transformed into ILC2 and secrete Th2-type cytokines. The ILC3-like ILC2 might be correlated with the increasing incidence of allergies and the increasing number of allergens that caused the reaction.
The plasticity of tissue-resident ILCs was most likely important in accelerating immune responses. However, these types of ILCs conversion might also lead to or contribute to certain tissue inflammation. It was not entirely clear how these conversions work. ILC2 could be divided into multiple sub-subgroups with various plasticity according to the expression of CD117, KLRG1, Bcl11b, and so on. ILC2, with these specific proteins, could shift to IL-17A+ILC3, depending on the presence or absence of inflammatory cytokines in the local tissue. IL-4 was critical for the maintenance of ILC2 phenotype and function IL-1β, IL-23, TGF-β can modulate the process of ILC2 shifting to ILC3-like cells, Notch signaling was also involved in the conversions process.25 ILC2 could also responds to IL-12 and IL-18 stimulation to produce IFN-γ.22,27 This study found that the expression of IL-4 in the lungs of the MPI model mice was significantly lower than that of the AR model mice. The high levels of IL-4 in AR model mice inhibited the plasticity conversion of ILC2, which explained why the conversion of ILC2 to ILC3 occurs in MPI model mice, and the reduced IL-4 levels in the lungs of MPI model mice relieved the inhibition of ILC2 plasticity. According to the results of this study, the level of IL-12 decreased in the lungs of the AR model mice and MPI model mice. This meat that the inflammatory environment in the lungs of the MPI model was unlikely to excite ILC2 subsets to transform into ILC1-like.
To clarify inflammatory environment in the lungs of the model mice, the levels of IL-23 and TGF-β were tested. And it was found that the protein level of IL-23 was increased in the lungs from MPI model mice. And the frequency of IL-23R+IL-5+ILC2 was increased. On the contrary, the level of TGF-β was not changed in the MPI model mice, and the frequency of TGF-β RII+IL-5+ILC2 decreased. Therefore, we believed that IL23R, but not TGF-βRII regulated the conversion of ILC2 to ILC3. ILCs and Th cells had a high degree of consistency. A study on Th17 cells found that both the TGF-β and IL-23 could induce the development and proliferation of Th17 cells that produce IL-17A, but the former induced nonpathogenic Th17, the latter induced pathogenic Th17, and drove T cells to promote neutrophil's infiltration.28,29 Whether ILC3-like ILC2 stimulated by IL-23 was pathogenic was a question worthy of further study.
Reversing the increase of IL-17A+ILC3 might have great significance in inhibiting the existence of MPI inflammation and its high responsiveness. Biminkang was a safe and effective Chinese medicine that is used for the treatment of AR in our department. And it could be taken for a long time safely. The mechanism of Biminkang inhibiting the conversion of ILC2 to ILC3-like in local tissues deserved our research. It was found that not only the frequency of IL-17A+ILC3 and ILC3-like ILC2 decreased but also the frequency of IL-23R+IL-5+ILC2 decreased in the model mice after the treatment of Biminkang. These results indicated that Biminkang could reduce the possibility of ILC2 conversion to ILC3 and reduce the ILC3-like ILC2 subsets, which might be related to the presence of MPI and airway hyperresponsiveness.
In conclusion, it was found that the IL-17A in the nasal lavage of AR patients increased during the MPI stage. More importantly, the frequency of IL-17A+ILC3 was significantly high in MPI model mice. Moreover, the increased IL-17A+ILC3 might be transformed from ILC2 because the frequency of ILC3-like ILC2 (IL-5+IL-17A+ILC and GATA3+RORγt+ILC) increased. And the IL-23R seemed to be critical in this process. These findings emphasized the importance of IL-23R on the surface of ILC2. And 23R+IL-5+IL-17A+ILC3 subsets might direct a new therapeutic strategy in MPI. And this study found that after the intervention of Biminkang, the MPI model mice could reduce the frequency of IL-17A+ILC3, especially, reduce the frequency of ILC3-like ILC2 subsets. This might be the result of changing the expression of IL-23R on the surface of ILC2 and inhibiting the conversion of ILC2 to ILC3. This might be a potential accurate method for us to treat the persistence of MPI.
ACKNOWLEDGMENTS
This study was supported by the following grants: the National Natural Science Foundation of China (No. 81974580, 82074088) and Academic Promotion Programme of Shandong First Medical University (2019QL007).
AUTHORSHIP
Conception and design: C. Y. and R. W.; Administrative support: R. W.; Provision of study materials or patients: X. C., S. Z., and C. L.; Collection and assembly of data: W. M. and L. Z.; Data analysis and interpretation: X. Z., M. S., and Y. Z.; Manuscript writing: X. C. and C. Y.; Final approval of manuscript: All authors. R.-Z. W. and C.-F. Y. contributed equally to this work.
DISCLOSURE
The authors declare no conflict of interest.