-
PDF
- Split View
-
Views
-
Cite
Cite
Hongxuan Li, Cong Qiao, Lingyu Zhao, Qingxu Jing, Dongbo Xue, Yingmei Zhang, Epigallocatechin-3-gallate reduces neutrophil extracellular trap formation and tissue injury in severe acute pancreatitis, Journal of Leukocyte Biology, Volume 112, Issue 6, December 2022, Pages 1427–1443, https://doi.org/10.1002/JLB.3A0322-151R
- Share Icon Share
Abstract
Neutrophil extracellular traps (NETs) promote intra-acinar trypsin activation and tissue damage. Therefore, reducing NET formation can reduce tissue damage in severe acute pancreatitis (SAP). However, NET formation pathways may differ among disease models. In this study, we evaluated the role of the myeloperoxidase–neutrophil elastase (NE) pathway in NET formation in SAP. SAP was induced by intraperitoneal injection of cerulein and LPSs in mice, and NE activity was inhibited by GW311616. Pancreatic tissues were collected for multiplex immunofluorescence, scanning electron microscopy, and western blotting to detect NET formation and the effect of NE on citrullinated histone H3, followed by analyses of serum amylase and cytokine levels. Pretreatment with GW311616 significantly reduced NET formation, pancreatic tissue damage, and systemic inflammatory responses in SAP. Network pharmacology analyses using NE as the target revealed the monomeric compound epigallocatechin-3-gallate (EGCG). Binding between EGCG and NE was validated using molecular docking, and the ability of EGCG to inhibit NE activity was verified experimentally. NET formation by PMA-stimulated neutrophils was significantly reduced in vitro when the cells were pretreated with 40 μM EGCG. Pretreatment with EGCG significantly reduced NET formation, pancreatic tissue damage, and systemic inflammatory responses in vivo. These results reveal that NET formation requires the myeloperoxidase-NE pathway, and citrullination of histone H3 is affected by NE activity in SAP. EGCG shows therapeutic potential for affecting NE activity, NET formation, and systemic inflammation in SAP.
INTRODUCTION
Acute pancreatitis (AP) is characterized by local and systemic inflammatory reactions with varying clinical courses.1 Although most cases of AP are self-limiting, approximately 20% of patients develop moderate or severe AP with pancreatic or peripancreatic tissue necrosis, organ failure, or both, with a mortality rate of 20–40%.1,2 Despite a shift in the past decade toward multidisciplinary, individualized treatment of AP with minimal invasiveness, the mortality rate of severe acute pancreatitis (SAP) remains high.1 Therefore, studies of the underlying pathophysiology of SAP can provide a basis for the development of improved treatment plans.
Although AP is initially triggered by premature activation of trypsinogen in acinar cells, this event does not determine the disease severity. Severity is determined by immune system activation in response to damaged acinar cells through the release of damage-associated molecular patterns, resulting in the release of inflammatory factors and an inflammatory response; these events can further trigger systemic inflammatory response syndrome.3 During this process, neutrophils reach the tissue interstitium and contribute to pancreatic injury.4 In addition, neutrophil extracellular traps (NETs) produced by neutrophils infiltrating the pancreatic tissue can promote intra-acinar cell trypsin activation and tissue damage.5–7
NETs are formed via 2 known pathways. The first is a cell death pathway, known as NETosis, which begins with shedding of the nucleus and disassembly of the nuclear membrane and continues with the loss of cell polarization, depolymerization of chromatin, and rupture of the plasma membrane.8–10 The second is nonlytic NETosis, which can occur independently of cell death and involves secretory expulsion of nuclear chromatin accompanied by release of granule proteins through degranulation.8–10 These components assemble extracellularly and leave behind active anucleated cytoplasts, which continue to ingest microorganisms.8 Myeloperoxidase (MPO) and neutrophil elastase (NE) play key roles in the NETosis pathway. Reactive oxygen species (ROS) generated by reduced nicotinamide adenine dinucleotide phosphate oxidase stimulates MPO to trigger the activation and translocation of NE from azurophilic granules to the nucleus, where NE proteolytically processes histones to disrupt chromatin packaging. Subsequently, MPO binds to chromatin and acts synergistically with NE to decondense chromatin.11 However, NET formation pathways may differ among different disease models.12–14 Furthermore, it is unclear whether NET formation in AP also relies on the MPO-NE pathway.
Thus, we hypothesized that an NE deficiency in AP could reduce the production of NETs, thereby reducing pancreatic tissue damage and modulating local and systemic inflammatory responses. Using a network pharmacology approach, we found that monomeric epigallocatechin-3-gallate (EGCG), a green tea constituent with anti-inflammatory, anticancer, antibacterial, and antioxidant activities, is a candidate compound for reducing NET formation. Although the treatment of chronic inflammation-driven diseases, such as inflammatory bowel disease, fatty liver, and neurodegenerative diseases,15 has been extensively evaluated, few studies have focused on the mechanism by which EGCG influences AP and NET formation. Therefore, we examined the effects of EGCG on NET formation during AP, damage to pancreatic tissues, and local and systemic inflammatory responses.
MATERIALS AND METHODS
Animals
Animal procedures were approved by the Medicine Institutional Animal Care and Use Committee of the First Affiliated Hospital of Harbin Medical University. C57BL/6 mice (20–22 g, 6–8 weeks old) were purchased from the Second Affiliated Hospital of Harbin Medical University and housed under standard conditions at 22°C with a 12 h light–dark cycle, fed a laboratory diet, and provided water ad libitum.
Induction of AP and experimental design
AP was induced by intraperitoneal injection of cerulein (50 μg/kg, 7 times at 1 h intervals) and LPSs (10 mg/kg) immediately after the last injection of cerulein. The mice were sacrificed after 24 h. Control mice were administered the same dose of saline.16 Blood, pancreatic tissue, and lung tissue samples were collected from the mice for subsequent experiments. To investigate the effect of NE on NET formation during AP, relevant animal models were constructed by intraperitoneal injection of the NE inhibitor GW311616 and EGCG. The mice were divided into the following 6 groups: (1) control group, (2) control + GW311616 group, (3) control + EGCG group, (4) AP group, (5) AP + GW311616 group, and (6) AP + EGCG group. The NE inhibitor GW311616 (2.0 μg/g in 300 μl of PBS; MedChemExpress, Monmouth Junction, NJ, USA; HY-15891) was administered to the mice by intraperitoneal injection every 8 h at 1 day prior to AP model induction.17 EGCG (50 mg/kg; Sigma–Aldrich, St. Louis, MO, USA; E4143) was intraperitoneally injected once daily for 1 week prior to AP model induction.
Scanning electron microscopy
Freshly collected pancreatic tissues were washed with isotonic saline and placed in glutaraldehyde and osmium acid for double fixation. The samples were then dehydrated and dried using increasing concentrations of acetone, followed by treatment with a metal coating method to make the sample surfaces conductive for observations.
Immunohistochemistry and histologic examination
Freshly collected pancreatic and lung tissues were fixed overnight in 4% formaldehyde, dehydrated, and embedded in paraffin. Paraffin-embedded samples were then cut into 4 μm-thick sections. Antigen retrieval was performed in Tris–EDTA buffer (pH 9) for 2 min in a pressure cooker. Endogenous peroxidase activity was blocked with 3% hydrogen peroxide. Nonspecific binding was blocked by incubating the sections with 10% goat serum at 37°C for 30 min. Next, the samples were incubated with anti-IL-1β (1:200; Affinity Biosciences, Cincinnati, OH, USA; AF5103), anti-IL-6 (1:200; Affinity Biosciences; DF6087), anti-C-X-C motif chemokine ligand 1 (anti-CXCL1; 1:200; Affinity Biosciences; AF5403) antibodies overnight at 4°C, followed by incubation with HRP-labeled secondary antibodies for 30 min at 37°C. Finally, the color was developed by incubation in 3,3′-diaminobenzidine for 5–10 min. After immunohistochemistry (IHC), the tissue sections were stained with hematoxylin. Tissue samples from the pancreas and lungs were fixed overnight in 4% formaldehyde, dehydrated, and embedded in paraffin. The 4 μm-thick sections were stained with H&E and observed under a light microscope.
Amylase measurements and ELISA
Serum amylase activity was analyzed using a colorimetric assay with a commercial kit (Beijing Leagene Biotech Institute, Beijing, China; TE0203). MPO activity in mouse lung homogenates was determined using an ELISA kit (Cloud-Clone Corp., Houston, TX, USA; SEA601Mu) according to the manufacturer's instructions. Briefly, 100 μl of standard or sample to be tested was added to each well, the plate was sealed, and the samples were incubated for 120 min at 37°C. After incubation, the plate was washed 4 times with washing solution. Primary antibody working solution (100 μl) was added to each well, the plate was sealed, and the samples were incubated for 60 min at 37°C. The plate was washed again, 100 μl of enzyme-labeled antibody working solution was added to each well, and the plate was sealed and incubated for 30 min at 37°C. The plate was washed again, 100 μl of substrate working solution was added to each well, and the plate was sealed and incubated for 15 min at 37°C in the dark. After adding termination solution, absorbance was read at 450 nm using a luminescence microplate reader (ELX808; Bio-Tek Instruments, Winooski, VT, USA).
Measurement of trypsin activity
Trypsin activity was measured using Boc-Gln-Ala-Arg-AMC (Sigma–Aldrich; B4153) fluorogenic substrate as previously described.18 The trypsin substrate Boc-Gln-Ala-Arg-AMC was added to the assay buffer (50 mM Tris–HCl [pH 8.0]). Next, 285 μl trypsin substrate solution was mixed with 15 μl of the sample supernatant to prepare a final volume of 300 μl sample per well. Control and treated pancreatic tissue trypsin activity was measured at excitation and emission wavelengths 380 and 440 nm, respectively, on a Fluroskan Assent spectrofluorometer (Nanodrop 2000; Thermo Scientific, Waltham, MA, USA). Fluorometric values were analyzed and expressed as mg of protein.
Detection of serum MPO–DNA
We detected serum MPO–DNA using a previously described capture ELISA.19 We coated 96-well microtiter plates with 5 μg/ml anti-MPO monoclonal antibody (Abcam, Cambridge, UK; ab208670) as the capturing antibody overnight at 4°C. After blocking in 1% bovine serum albumin, mouse serum together with peroxidase-labeled anti-DNA monoclonal antibody was added (component No. 2 of the Cell Death Detection ELISA kit; Roche, Basel, Switzerland; 11774425001), incubated at room temperature for 2 h and washed with PBS 3 times, and the peroxidase substrate (Roche; 11774425001) was added. After incubation at 37°C for 20 min, the optical density was measured at 405 nm using a microplate reader (Berthold Technologies, Baden-Württemberg, Germany; LB941).
Western blotting
The mouse pancreas samples were washed twice with prechilled PBS according to the animal experimental groups, and 1 ml of radioimmunoprecipitation assay lysis buffer (Beyotime, Shanghai, China) spiked with PMSF (Solon, OH, USA) was added to a 100 mg aliquot of each tissue sample. After sufficient lysis, the samples were centrifuged at 4°C for 5 min at 12,000×g. The supernatant was immediately aspirated into a prechilled Eppendorf tube and used to extract cellular proteins. Protein was quantified using the bicinchoninic acid method. Protein was separated using sodium dodecyl sulfate–polyacrylamide gel electrophoresis and transferred onto polyvinylidene fluoride membranes (0.45 μm) (Millipore, Billerica, MA, USA). The membranes were blocked with 5% (w/v) skim milk and then incubated with rabbit anticitrullinated histone H3 (CitH3; 1 μg/ml; Abcam; ab5103) and rabbit anti-NE (1 μg/ml; Abcam; ab68672) overnight at 4°C. The membranes were incubated with HRP-conjugated secondary antibody (Abcam). Immunoreactive protein bands were detected using enhanced chemiluminescence and a Tanon 6600 luminescence imaging workstation (Tanon Science & Technology, Shanghai, China).
Network pharmacology-based analysis
Candidate compounds were predicted using the Traditional Chinese Medicine Systems Pharmacology (TCMSP) platform (http://tcmspw.com/tcmsp.php)20 with NE as the target. Screening criteria were oral bioavailability ≥30% and drug-like properties ≥0.18. Gene Ontology (GO) and Kyoto Encyclopedia of Genes and Genomes (KEGG) enrichment analyses of the possible targets of the target components were performed using R software (v.3.6.3; The R Project for Statistical Computing, Vienna, Austria). The structures of target proteins and ligands were obtained from the Protein Data Bank (http://www.rcsb.org/pdb). Molecular docking of the target proteins and ligands was performed using PyMoL (v.2.3) and AutoDock Vina (v.1.1.2) to evaluate the binding activity of EGCG to NE targets based on their binding energies.
Determination of antielastase activity
A fluorometric NE inhibitor screening kit (BioVision, San Francisco, CA, USA; K782-100) was used to assess the ability of EGCG to inhibit NE enzyme activity. The compound concentrations were 0, 0.01, 0.1, 1, 10, 100, 1000, and 10,000 μM. Samples were prepared in 96-well black plates (clear bottom) for fluorometric assays and analyzed according to the manufacturer's instructions. Briefly, NE solution, NE substrate, and inhibitor control (SPCK) were prepared according to the manufacturer's recommendations. The NE solution was prepared by adding 2 μl of NE stock solution and 48 μl of analytical buffer to each well. The sample, inhibitor control, and enzyme control wells were prepared separately. Next, 25 μl of sample was added to the sample wells, 25 μl of the inhibitor SPCK (diluted 1:25 in analytical buffer) was added to the inhibitor control wells, and 25 μl of analytical buffer was added to the enzyme control wells. The plates were incubated for 5 min at 37°C, after which 25 μl of reaction mixture (23 μl of analytical buffer and 2 μl of substrate) was added to each well, and the absorbance of the samples was measured immediately after mixing. Values at excitation/emission wavelengths of 400/505 nm (R1) and those at 400/505 nm (R2) were read after reacting the samples for 30 min at 37°C under protection from light, and ∆relative fluorescence units (RFU) were calculated as R2 − R1. The blank control was set to 100% relative activity. The following parameters were calculated:
Neutrophil isolation
Peripheral blood was collected from healthy volunteers after obtaining informed consent was obtained. Fresh peripheral blood was slowly collected in commercial EDTA-k2 anticoagulation tubes, from which neutrophils were isolated within 2 h of collection using a commercial kit (Tianjin Haoyang Biotechnology, Tianjin, China, LZS11131). Briefly, 5 ml of separation solution was added to a 15 ml centrifuge tube, and 5 ml of fresh whole blood was carefully placed above the separation solution and centrifuged at 500×g for 30 min at room temperature. The milky white cell layer containing neutrophils was collected, washed, resuspended in 5 ml of ammonium–chloride–potassium lysis buffer; washed twice in HBSS without Ca2+/Mg2+; and the neutrophils were counted after resuspension in serum-free RPMI-1640.
Neutrophil treatment and NET induction
The cells were seeded into 48-well plates at 2 × 105 cells/well, pretreated with different concentrations of EGCG, and incubated for 30 min at 5% CO2 and 37°C. The cells were then treated with 50 nM PMA (Sigma–Aldrich; P1585), and control cells were treated with an equal volume of RPMI-1640. After 4 h of incubation, the cell supernatants were collected for subsequent assays. The 48-well plates were preplated with sterile polylysine-treated glass slides and inoculated with 2 × 105 cells/well. After 4 h, the cell supernatant was removed and fixed with 4% paraformaldehyde for 20 min for immunofluorescence staining.
DNA detection with Quant-iT PicoGreen
To detect NET markers, Quant-iT PicoGreen dsDNA Reagent and Kits (Invitrogen, Carlsbad, CA, USA; P11496) were used to quantify free DNA in the cell supernatant. Experiments were performed as described previously with slight modifications.19,21 We rinsed the NETs attached to the bottom with supernatant, transferred the solution into 1.5 ml tubes, and centrifuged these samples at 370×g for 10 min. The supernatant was discarded, and the pellet was resuspended in 125 μl PBS. Micrococcal nuclease (0.5 U/ml) was added, the samples were incubated at 37°C and 5% CO2 for 10 min, and 5 mM EDTA was added to stop the reaction. Centrifugation was performed at 370×g for 5 min, and the cell supernatant containing NETs (NET-associated DNA) was collected for detection. The standard stock solution and samples were diluted according to the kit instructions, and signal intensity was detected using a fluorescence microplate reader at excitation and emission wavelengths of 480 and 520 nm, respectively.
Immunofluorescence staining
Cells used for immunofluorescence were fixed with 4% paraformaldehyde for 20 min at room temperature, washed with PBS, and permeabilized with 0.2% Triton X-100 for 5 min. Nonspecific binding was blocked using 10% donkey serum for 30 min at room temperature. The samples were incubated with rabbit anti-H3Cit (1:100; Abcam; ab5103) and goat anti-MPO (10 μg/ml; R&D Systems; AF3667) antibodies overnight at 4°C, followed by incubation with Alexa-Fluor-conjugated secondary antibody (Abcam) for 1 h at room temperature. The nuclei were counterstained with DAPI, and images were obtained using fluorescence microscopy. The tissues were stained using multicolor immunofluorescence. The tissues were fixed in 4% paraformaldehyde (Thermo Fisher Scientific) for 24 h at 4°C, washed with PBS, embedded in paraffin, and sectioned at a thickness of 4 μm. Antigen retrieval was performed using a target retrieval solution (Tris–EDTA, pH 9.0) in a pressure cooker for 2–3 min. Nonspecific binding was blocked by incubating the samples with 10% goat serum for 10 min at room temperature. The samples were then incubated with rabbit anti-NE (1:100; Abcam; ab68672), rabbit anti-H3Cit (1:100; Abcam; ab5103), and rabbit anti-MPO (1:100; Abcam; ab208670) antibodies overnight at 4°C. The tissues were incubated with HRP-labeled secondary antibodies for 30 min at 37°C. Next, the tissues were incubated with a fluorescent dye staining working solution for 10 min at 37°C to amplify the fluorescence signal. DAPI was used to counterstain the nuclei, and fluorescence signals were captured by multispectral imaging using a Vectra Polaris™ slide imager (Akoya Biosciences, Marlborough, MA, USA). The results of multiplex immunofluorescence analysis were quantified with HALO 3.3 next-generation digital pathology (Indica Labs, Albuquerque, NM, USA).
Statistical analysis
Data are presented as the means ± sem. Statistical analyses were performed using GraphPad Prism 8 software (GraphPad, Inc., La Jolla, CA, USA). Statistical differences between groups were determined using one-way analysis of variance and Tukey's tests. Statistical significance was set at p < 0.05, and n indicates the number of samples in each group.
RESULTS
GW311616 reduces pancreatic and lung tissue damage in AP
To evaluate the role of NE in NET formation and progression of AP, a specific inhibitor of NE (GW311616, NEi) was preinjected intraperitoneally. GW311616, (3aR,6S,6aS)−4-methylsulfonyl-1-[(E)−4-piperidin-1-ylbut-2-enoyl]−6-propan-2-yl-3,3a,6,6a-tetrahydro-2H-pyrrolo [3,2-b] pyrrol-5-one, is a high-activity, cell-permeable, orally biocompatible human NE inhibitor.22,23 GW311616 is a selective intracellular inhibitor of human NE and can rapidly eliminate NE circulating in dogs, with >90% inhibition maintained for 4 days.22 In addition, GW311616 has been used as an NE inhibitor in rats, hamsters, and mice.22,24,25 AP was induced in mice by intraperitoneal injection of cerulein combined with LPS.16 Histologic examination showed that the pancreatic structure of control mice was normal (Figure 1(A)). In contrast, the pancreatic tissue structure of AP mice was highly damaged, showing edema formation, acinar cell injury, and inflammatory cell infiltration (Figure 1(A)). Administration of GW311616 protected against tissue damage in AP mice (Figure 1(A)), resulting in a remarkable reduction in cerulein-induced edema formation and inflammatory cell infiltration (Figure 1(A)). Serum amylase levels, a valid indicator of pancreatic tissue injury, were significantly higher in AP mice than in control mice (p < 0.0001) (Figure 1(B)). Serum amylase levels were significantly lower in the GW311616 group than in the AP group (p < 0.01) (Figure 1(B)). Treatment with GW311616 alone had no effect on serum amylase levels in healthy mice (Figure 1(B)). Acute lung injury often occurs in SAP and is among the most serious complications of SAP.26,27 In addition, pulmonary lesions and functional changes of SAP often occur earlier than in other organs (such as the heart, liver, and kidney) and are closely related to early mortality in patients with SAP.28 Lung histology and lung MPO levels can be used to assess acute lung injury and inflammation.29 Histologic examination of the lung and elevated pulmonary MPO levels indicated acute lung injury during AP. Our findings revealed elevated MPO activity in the lungs of AP mice, with substantial pulmonary pathologic changes characterized by extensive alveolar wall thickening, interstitial lung edema, and inflammatory cell infiltration (Figures S1(A) and S1(B)). Administration of GW311616 resulted in significant reduction in MPO levels in the lungs of AP mice along with an improvement in histopathologic changes (p < 0.01) (Figures S1(A) and S1(B)).

GW311616 attenuates pancreatic tissue damage by targeting NE in SAP. (A) Representative H&E images of pancreatic tissue sections in the indicated groups. Scale bars: 200 μm. (B) Quantification of serum amylase levels (n = 6). (C and D) GW311616 inhibits NE expression in the AP mouse model; NE expression levels in the indicated groups were detected using western blotting (n = 3). (E) Representative immunohistochemistry images of NE-stained pancreatic tissue sections in the indicated groups. Scale bars: 100 μm. Data are presented as the means ± sem, ****p < 0.0001; ***p < 0.001; **p < 0.01. AP, acute pancreatitis; NE, neutrophil elastase
GW311616 reduces NET formation in AP
A recent study showed that NETs can increase the activity of trypsin in acinar cells, and activation of trypsin by NETs is comparable to that of cerulein in vitro.5 To evaluate whether inhibiting NET formation can effectively reduce damage to pancreatic tissues, we injected mice with the NE inhibitor GW311616 and evaluated NE expression and NET formation. Western blotting and IHC results showed that the protein level of NE was higher in AP pancreatic tissues than in control tissues, and NE was expressed in the nucleus, cytoplasm, and tissue interstitial space (Figures 1(C)–1(E)). The protein expression of NE in pancreatitis tissues was significantly lower in the group pretreated with GW311616 than in the AP group (p < 0.0001) (Figures 1(C)–1(E)). Normally, NE is stored in the azurophilic granules of neutrophils. During NET formation, NE can enter the nucleus, leading to chromatin decondensation, followed by release from the cell together with nuclear DNA to form extracellular reticular structures. This may explain why variable tissue localization of NE was observed using IHC. In addition, scanning electron microscopy revealed obvious reticular structures around the neutrophil infiltrate in the pancreatic tissue (Figure 2(A)). After pretreatment with GW311616, no reticular structures were observed around the neutrophil infiltrate, and NET formation was significantly reduced (Figure 2(A)). However, no reticular structure or inflammatory cell infiltration was observed in normal pancreatic tissues or in normal pancreatic tissues pretreated with GW311616 alone (Figure 2(A)).
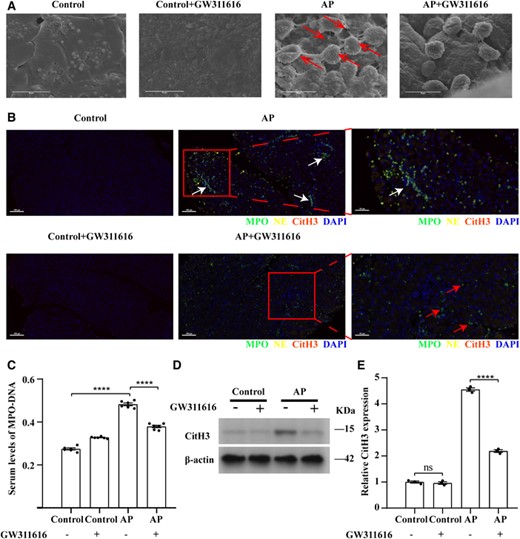
GW311616 reduces NET formation in AP. (A) Scanning electron microscopy showing extracellular web-like structures around the neutrophil infiltrate in AP mouse pancreatitis tissue (shown by red arrows). Scale bars: 10 μm. (B) Representative immunofluorescence images of NE, MPO, and CitH3 staining in the indicated groups. White arrows indicate NETs costained with NE, MPO, and DAPI; red arrows indicate intact neutrophils; and yellow arrows indicate CitH3. Nuclei were stained with DAPI (blue), whereas NE, MPO, and CitH3 were visualized in yellow, green, and red, respectively (scale bars = 100 μm). Images on the far right are magnified views (scale bar = 50 μm). (C) Serum levels of MPO–DNA in mice with AP (n = 6). (D and E) GW311616 inhibits the expression of CitH3 in the AP mouse model. CitH3 expression levels in the indicated groups were detected using western blotting (n = 3). Data are presented as the means ± sem, ****p < 0.0001; **p < 0.01; ns, not significant. AP, acute pancreatitis; CitH3, citrullinated histone 3, NET, neutrophil extracellular trap; NE, neutrophil elastase; MPO, myeloperoxidase; DAPI, 4′,6-diamidino-2-phenylindole
Considering that there is currently no uniform method for identifying NET formation in tissues, NETs are identified using CitH3, NE and DNA colocalization, or MPO and CitH3 colocalization.19,30–35 However, these indicators do not show uniform results in different research backgrounds. Accordingly, we simultaneously detected MPO, NE, and CitH3 using multiplex immunofluorescence staining to identify reliable markers for NET formation within 24 h of AP onset. NE and MPO were colocalized in the pancreatic tissue of AP mice, and CitH3 was present in small amounts and located close to MPO and NE. In the group pretreated with GW311616, NE and CitH3 were almost undetectable (Figure 2(B)). However, no significant positive signals for MPO, NE, or CitH3 were observed in normal pancreatic tissues or in normal pancreatic tissues pretreated with GW311616 alone (Figure 2(B)). NETs contain MPO and NE, whereas in resting neutrophils, they also coexist in granules. The difference is that their localization to the nucleus is relatively fixed in the resting state (Figure 2(B)), and clear nuclear morphology and protein localization can be observed. However, after NET formation, chromatin depolymerization and nuclear membrane rupture cause the positions of MPO, NE, and nuclear DNA to become unclear, disrupting the originally relatively fixed positions and resulting in residual mixing of the nucleus, cytoplasm, and granules; these conditions favor colocalization of these components. Notably, although the NET-enriched area can be quite large, NETs can be significantly more focused and contain only a few neutrophils in dense tissue, as observed in myocarditis.35,36 Therefore, it is crucial to determine the precise colocalization of nuclear and granular components using immunofluorescence staining. Notably, the serum NET levels, which were evaluated by detecting the MPO–DNA complex and showed good agreement with tissue NET levels, were significantly higher in mice with AP compared with in mice pretreated with GW311616 (p < 0.01) (Figure 2(C)). In addition, the expression level of CitH3 is regulated by protein arginine deiminase 4 (PAD4). Western blotting results showed that CitH3 protein levels were significantly higher in the pancreatic tissues of AP mice than in those of control mice (Figures 2(D) and 2(E)). CitH3 protein levels were significantly lower in the group pretreated with GW311616 than in the AP group (p < 0.0001) (Figures 2(D) and 2(E)). Pretreatment with GW311616 alone did not affect CitH3 protein levels in the pancreatic tissues of healthy mice (Figures 2(D) and 2(E)). These results demonstrate that citrullination of histone H3 is affected by NE activity.
Acquisition and verification of candidate compounds
After confirming that NE activity in AP significantly affects NET formation, we explored candidate compounds that could attenuate NE activity, reduce NET formation, and alleviate AP progression. We then used NE as the target protein and predicted related components using the TCMSP database. The database contains more than 29,000 compounds and provides comprehensive pharmacokinetic information for each compound. In addition, the database provides target and disease information for potentially active molecules, which can be used to form a corresponding drug–target–disease network for each herb and provide a platform for studying the mechanism of compounds at the system level.20 The database-related operation process is shown in Figure 3. We identified trans-2-nonenal, thymol, and EGCG (MOLID: MOL006821) as candidates. Only EGCG met the screening thresholds of oral bioavailability ≥30 and drug-like properties ≥0.18. Molecular docking results for EGCG with the target protein showed better binding activity, with the lowest binding energy of −5.14 kcal/mol (Figures 4(A) and S2). To verify these results, we assessed the effect of EGCG on NE activity in vitro and found that the substrate NE protein activity decreased significantly with increasing concentrations of EGCG. The half-maximal inhibitory concentration for inhibition of enzymatic activity of NE by EGCG was 51.68 nM (Figure 4(B)). In addition, according to GO and KEGG analyses, the target genes of EGCG were significantly enriched in various biologic processes, including the response to ROS and oxidative stress (Figures 4(C) and 4(D)). Given the antioxidant activity of EGCG and importance of ROS and NE in NET formation, these results suggest that EGCG can effectively reduce NET formation and pancreatic tissue damage.
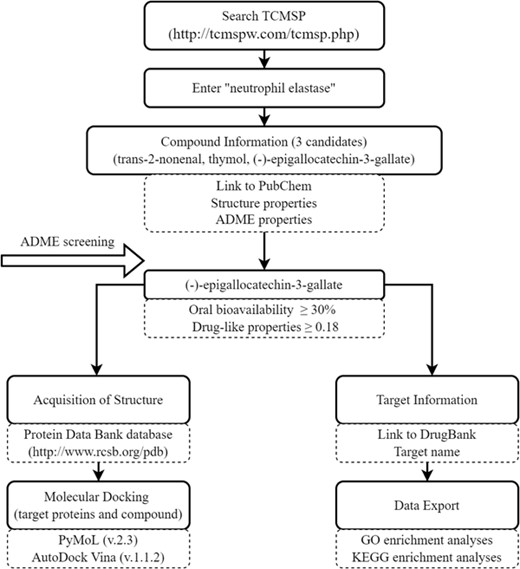
Schematic flowchart of database-related operations. TCMSP, Traditional Chinese Medicine Systems Pharmacology; ADME, absorption, distribution, metabolism, and excretion; GO, Gene Ontology; KEGG, Kyoto Encyclopedia of Genes and Genomes
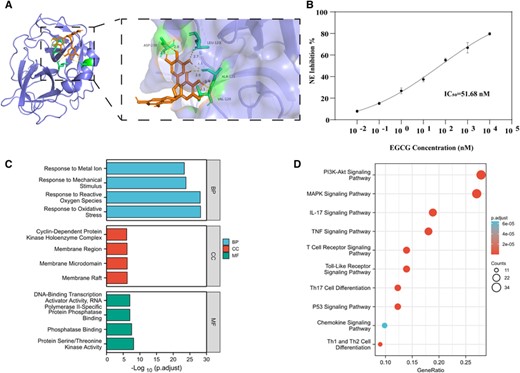
Acquisition and verification of candidate compounds. (A) Molecular docking of compounds to core targets; the protein structure of NE is shown in blue, structure of EGCG is shown in brown, and green shows the region where NE may bind to EGCG with the lowest binding energy of −5.41 kcal/mol. Only the lowest binding energy is shown, and other possible binding modes are described in the Supplementary Materials and methods section. (B) Half-maximal inhibitory concentration (IC50) of EGCG on NE activity was 51.68 nM. (C) GO enrichment analysis of EGCG target genes; blue shows biologic process (BP) terms, red shows cellular component (CC) terms, and green shows molecular function (MF) terms. (D) KEGG enrichment analysis of EGCG target genes. NE, neutrophil elastase; EGCG, epigallocatechin-3-gallate; GO, Gene Ontology; KEGG, Kyoto Encyclopedia of Genes and Genomes
EGCG reduces NET formation in vitro
We further investigated the effect of EGCG on NET formation. The effective concentration of EGCG for inhibiting NET formation was assessed by measuring the level of NET-DNA in the cell culture supernatant. The DNA content in the supernatant of PMA-stimulated neutrophils was significantly higher than that in the negative control group (RPMI-stimulated neutrophils) (p < 0.0001), and the DNA content in the supernatant of neutrophils stimulated with EGCG (40 μM) alone did not change significantly. Compared with levels in the positive control group (PMA-stimulated neutrophils), the DNA content was decreased in the supernatant of cells pretreated with 5, 10, and 160 μM EGCG; however, this trend was not significant. When EGCG was added to pretreated cells at concentrations of 20 μM (p < 0.01), 40 μM (p < 0.001), and 80 μM (p < 0.01), the DNA content in the cell supernatant decreased significantly with increasing drug concentrations. When the EGCG concentration was 80–160 μM, the DNA content in the cell supernatant increased with increasing drug concentrations (Figure 5(A)). Subsequently, colocalization of MPO and CitH3 was detected using immunofluorescence to identify NET formation. NET formation by PMA-stimulated neutrophils was significantly reduced when cells were pretreated with 40 μM EGCG (Figure 5(B)).
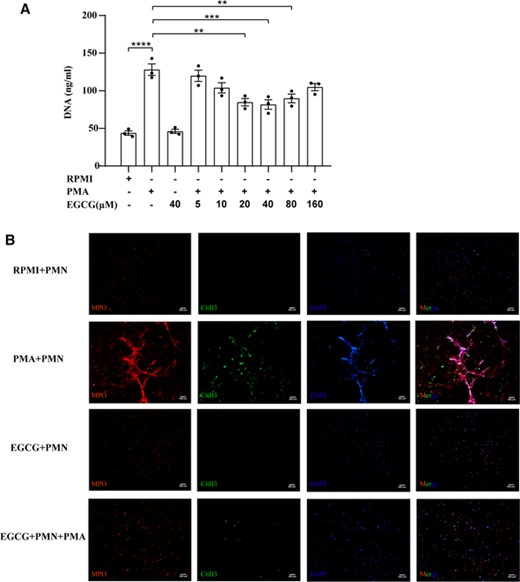
EGCG reduces NET formation in vitro. (A) Effect of EGCG on NET-DNA levels in the supernatant of neutrophils induced by PMA. Data are presented as the means ± sem for 3 individual experiments. ****p < 0.0001; ***p < 0.001; **p < 0.01. (B) Representative immunofluorescence micrographs of the indicated groups showing NETs, identified by colocalization of DNA (blue) with CitH3 (green) and the neutrophil granule marker MPO (red). Scale bars: 200 μm. RPMI, Roswell Park Memorial Institute; PMA, phorbol 12‑myristate 13‑acetate; EGCG, epigallocatechin-3-gallate; MPO, myeloperoxidase; CitH3, citrullinated histone 3; DAPI, 4′,6-diamidino-2-phenylindole
EGCG reduces pancreatic and lung tissue damage in AP
EGCG administration protected against tissue damage in AP mice (Figure 5(A)), resulting in a remarkable decrease in edema formation and inflammatory cell infiltration (Figure 6(A)). Serum amylase levels were significantly elevated in AP mice and significantly decreased in EGCG-treated AP mice (p < 0.001) (Figure 6(B)). Treatment with EGCG alone had no effect on serum amylase levels in healthy mice (Figure 6(B)). In addition, markedly elevated trypsin activity occurs as the first sign of AP and persists into later stages of the disease.37 Compared with the AP group, trypsin activity was significantly decreased in EGCG-treated AP mice (Figure 6(C)). Lung histologic examination and the MPO assay results revealed elevated MPO activity in the lungs of AP mice. Remarkable pulmonary pathologic changes were observed, characterized by extensive alveolar wall thickening, interstitial edema, and inflammatory cell infiltration (Figure S1). Administration of EGCG led to a significant reduction in MPO levels in the lungs along with improvement in histopathologic changes in AP mice (p < 0.01) (Figure S1).
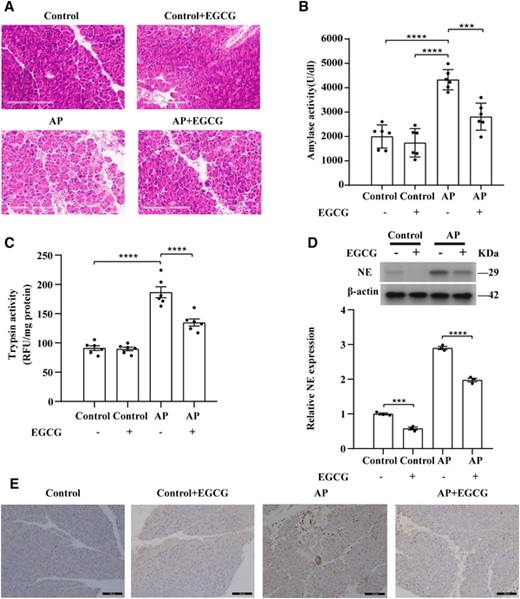
EGCG attenuates pancreatic tissue damage in SAP. (A) Representative H&E images of pancreatic tissue sections for the indicated groups. Scale bars: 200 μm. (B) Quantification of serum amylase levels (n = 6). (C) Quantification of trypsin activity levels (n = 6). (D) EGCG inhibits NE expression in the AP mouse model; NE expression in the indicated group was detected using western blotting (n = 3). (E) Representative immunohistochemistry images of NE-stained pancreatic tissue sections of the indicated groups. Scale bars: 100 μm. Data are presented as the means ± sem, ****p < 0.0001; ***p < 0.001. AP, acute pancreatitis; NE, neutrophil elastase; EGCG, epigallocatechin-3-gallate
EGCG reduces NET formation in AP
After confirming that EGCG reduced NET formation in vitro, we evaluated the expression of NE in pancreatic tissues and formation of NETs after intraperitoneal injection of EGCG in mice. Western blotting and IHC showed that the protein expression level of NE was significantly lower in normal pancreatic tissues treated with EGCG alone than in the control group (p < 0.001) (Figures 6(D) and 6(E)). The protein expression level of NE was significantly lower in pancreatitis tissues after pretreatment with EGCG than in the AP group (p < 0.0001) (Figures 6(D) and 6(E)). Scanning electron microscopy revealed obvious reticular structures around the neutrophil infiltrate in the AP pancreatic tissue, along with a large number of red blood cells, indicating hemorrhage in this tissue (Figure 7(A)). Unlike in the AP group, no reticular structure was observed around the neutrophil infiltrate in the pancreatitis tissue after pretreatment with EGCG, and NET formation was remarkably reduced (Figure 7(A)). However, no reticular structure or infiltration of inflammatory cells was observed in normal pancreatic tissues or in normal pancreatic tissues pretreated with EGCG alone (Figure 7(A)). As determined using multiplex immunofluorescence, NE and MPO were colocalized in the pancreatic tissue of AP mice, and CitH3 was present in small amounts and was in close proximity to MPO and NE. In the pretreatment group, EGCG, NE, and CitH3 were not expressed (Figure 7(B)). However, no significant positive MPO, NE, or CitH3 signals were observed in normal pancreatic tissues or in normal pancreatic tissues pretreated with EGCG alone (Figure 7(B)). Serum NET levels were significantly higher in mice with AP compared to in those pretreated with EGCG (p < 0.01) (Figure 7(C)). Furthermore, similar to in the GW311616-treated group, CitH3 protein levels decreased significantly in the pancreatitis tissues after EGCG pretreatment (p < 0.0001) (Figures 7(D) and 7(E)). Pretreatment with EGCG alone did not affect CitH3 protein levels in the pancreatic tissues of healthy mice (Figure 7(D) and 7(E)). However, NE is not the only target of EGCG, and the role of EGCG in the relationship between NE and histone citrullination requires further analysis. These results demonstrate that EGCG can reduce the expression levels of NE and CitH3 and inhibit the formation of NETs.
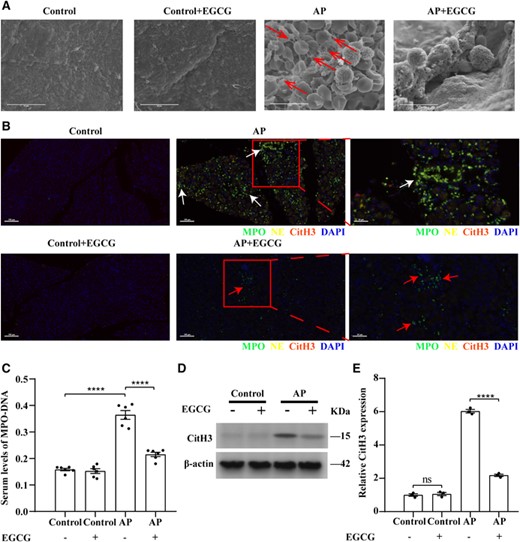
EGCG reduces NET formation in AP. (A) Scanning electron microscopy showing extracellular web-like structures around the neutrophil infiltrate in AP mouse pancreatitis tissues (red arrows). Scale bars: 10 μm. (B) Representative immunofluorescence images of staining for NE, MPO, and CitH3 in the indicated groups. White arrows indicate NETs costained with NE, MPO, and DAPI, and red arrows indicate intact neutrophils. Nuclei were stained with DAPI (blue), whereas NE, MPO, and CitH3 are shown in yellow, green, and red, respectively (scale bars = 100 μm). Images on the far right are magnified views of inserts (scale bar = 50 μm). (C) Serum levels of MPO–DNA in mice with AP (n = 6). (D and E) EGCG inhibits CitH3 expression in the AP mouse model; CitH3 expression in each group was detected using western blotting (n = 3). Data are presented as the means ± sem, ****p < 0.0001; **p < 0.01; ns, not significant. AP, acute pancreatitis; CitH3, citrullinated histone 3; EGCG, epigallocatechin-3-gallate; MPO, myeloperoxidase
GW311616 and EGCG regulate systemic inflammation
The initial immune response is characterized by the release of proinflammatory cytokines, which can trigger systemic inflammatory response syndrome in AP. However, recent studies revealed that systemic inflammatory response syndrome and compensatory anti-inflammatory response syndrome are parallel rather than consecutive processes in AP.29 We then examined the levels of pro- and anti-inflammatory cytokines in the circulation and local pancreatic tissues in different subgroups to assess the effects of GW311616 and EGCG on systemic and local inflammatory responses. The Bio-Plex Pro Mouse Cytokine Assay and IHC results showed that compared with the levels in healthy mice, the expression levels of IL-1β, IL-6, and CXCL1 in the peripheral blood and local pancreatic tissue were significantly increased within 24 h of AP (Figures 8(A), 8(B), 8(D), and 8(E)). Administration of GW311616 and EGCG significantly decreased the expression levels of IL-1β, IL-6, and CXCL1 in the peripheral blood and local pancreatic tissues compared to those in the AP group (Figures 8(A), 8(B), 8(D), and 8(E)). In addition, the expression levels of IL-10 significantly differed only in the peripheral blood of mice with AP but not in local pancreatic tissues (Figure 8(C)). Administration of GW311616 and EGCG significantly reduced IL-10 expression in the peripheral blood of AP mice (Figure 8(C)). GW311616 and EGCG alone had no effect on IL-1β, IL-6, CXCL1, and IL-10 levels in the peripheral blood and local tissues (Figure 8). These results demonstrate that GW311616 and EGCG effectively reduce systemic and local inflammatory responses in AP.
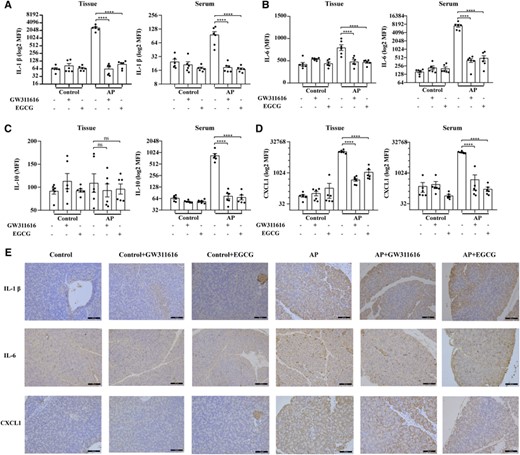
GW311616 and EGCG regulate systemic inflammation. (A) Quantification of IL-1β levels in the pancreatic tissue and serum. (B) Quantification of IL-6 levels in the pancreatic tissue and serum. (C) Quantification of IL-10 levels in the pancreatic tissue and serum. (D) Quantitative measurement of CXCL1 levels in the pancreatic tissues and serum. (E) Representative immunohistochemistry images of pancreatic tissue sections stained for IL-1β, IL-6, and CXCL1 in the indicated groups. Scale bars: 100 μm. Data are presented as the means ± sem (n = 6). ****p < 0.0001; ns, not significant. AP, acute pancreatitis; EGCG, epigallocatechin-3-gallate; MFI, median fluorescence intensity
DISCUSSION
Neutrophils infiltrating pancreatic tissues produce NETs, which can promote intra-acinar trypsin activation and tissue damage.5–7 In early studies, NET formation was eliminated by injection of DNase I into mice. NET inhibition was shown to reduce CXCL2 formation, neutrophil recruitment in the inflamed pancreas, and pancreatic tissue damage.5 In addition, in vitro experiments demonstrated that NETs can regulate STAT3 activity and trypsin activation in acinar cells.5 Thereafter, multiple research teams confirmed the important roles of c-Abl kinase, extracellular cold-inducible RNA-binding protein, and PAD4 in regulating NET formation in inflamed pancreas by using relevant specific inhibitors in mice.6,7,38,39 A recent study revealed that injection of protectin D1 into mice effectively inhibited the expression of PAD4, thus reducing NET formation and pancreatic tissue damage.40 Therefore, reducing NET formation can improve tissue damage in SAP. Most recent studies focused on the roles of histone citrullination in chromatin driven by PAD4 in the depolymerization of chromatin and NET formation.41 However, NE also plays a key role in NET formation, which can be translocated from azurophilic granules to the nucleus, hydrolyze histones in the nucleus to disrupt chromatin packaging, and act synergistically with MPO to promote chromatin decondensation and subsequent NET formation.11
Based on recent research, NET formation does not occur via the MPO-NE pathway in all diseases. Fungi and crystals stimulate NET formation via the MPO-NE pathway, the role of which has been confirmed in studies of neutrophils from patients with chronic granulomatous disease and complete MPO deficiency.42–45 In addition, in diseases such as sepsis, cancer, and lung infections, NETs were formed via the MPO-NE pathway in NE knockout or NE inhibitor mouse disease models.12–14 However, other studies demonstrated that NE is not required for NET formation under noninfectious stimuli and that NE deficiencies did not affect a mouse model of venous thrombosis induced by stenosis of the inferior vena cava.46 Therefore, we evaluated whether NE is required for NET formation during AP.
Our results demonstrated that the small-molecule compound GW311616, a known NE inhibitor and an inhibitor of NETs, can inhibit NE activity and reduce NET formation in the pancreatic tissues of AP mice. There is currently no uniform method for identifying NET formation in tissues. Using multiplex immunofluorescence staining for markers of NETosis and neutrophils,19 we found that in a specific AP mouse model, NET formation was more likely to occur via the MPO–NE pathway. We further found that CitH3 expression level was elevated, but citrullination of histone H3 was not the main pathway implicated for this effect. These results may be explained by the loss of NETs over time (i.e., in late stages) through their phagocytosis by phagocytes (such as macrophages) in the microenvironment. The exact mechanism of action requires further investigation. Therefore, the premise of using CitH3 as a signal for NET formation is that NETs are formed through a CitH3-dependent pathway. If NETs are formed through a CitH3-independent pathway during pathologic changes, or if the CitH3-related pathway is not the main pathway, then, the application of CitH3 may impact the results. Our comprehensive visual qualitative analysis of costaining for MPO, NE, and CitH3 using immunofluorescence provided a more complete assessment of NET formation, but further quantitative research is required. In addition, it is unclear whether histones can undergo citrullination and promote chromatin depolymerization in the absence of NE activity.8 One study showed that NE inhibitors blocked chromatin depolymerization during pulmonary fungal infection without interfering with the citrullination of histone H3, suggesting that histone citrullination occurs independently of NE activity.12 However, it is unclear whether histone citrullination is dependent on NE activity in AP. We found that CitH3 expression is affected by NE activity. Moreover, GW311616 significantly attenuated pancreatic and lung tissue damage and inflammatory cell infiltration in mice with AP.
We identified EGCG as a monomeric compound that may affect NE activity. A previous study showed that EGCG had an inhibitory effect on NE activity, which was consistent with our results; additionally, the effects were stronger than those of other inhibitors used to treat diseases characterized by neutrophil and leukocyte elastase involvement.47 In addition, EGCG has a strong antioxidant effect in healthy cells; it reduces oxidative damage and exhibits a variety of antitumor and immunomodulatory effects.48,49 However, EGCG has 8 phenolic groups, which can provide multiple electron acceptors and donors for proteins. This allows EGCG to bind to many different proteins with high affinity and inhibit their activity.50 Additionally, the inhibitory effect of EGCG on proteins is difficult to infer in vivo based on in vitro findings. Therefore, we showed in vivo that EGCG can significantly reduce the protein level of NE in pancreatic tissues. However, according to our previous prediction results, NE is not the only target protein of EGCG, and thus, it cannot be used as a specific inhibitor of NE. In addition, an indirect effect of EGCG on NE protein expression levels cannot be excluded. However, given the antioxidant activity of EGCG and importance of ROS and NE in NET formation, EGCG may be effective for reducing NET formation and pancreatic tissue damage. To date, the effects of EGCG on NETs have not been studied. We verified the effect of EGCG on NET formation in vitro. First, when stimulating isolated neutrophils in vitro, we applied PMA, which is commonly used and requires NE and CitH3 to stimulate NET formation.46,51 Second, based on the various methods for detecting NET formation, each of which have advantages and disadvantages, we measured NET-associated DNA levels, a widely used quantitative detection method for NET markers.21 In addition, CitH3 and MPO were detected using cellular immunofluorescence as a complementary method to visualize NET formation.19,31–33 Our results showed that NET formation was significantly inhibited by 40 μM EGCG. Our in vitro data were supported by in vivo experiments and scanning electron microscopy, showing that EGCG can effectively inhibit NE activity and reduce NET formation in the pancreatic tissues of AP mice. Immunofluorescence staining for specific markers of NET formation confirmed that EGCG significantly reduced NET formation in AP. Furthermore, EGCG significantly attenuated pancreatic and lung tissue damage and inflammatory cell infiltration in mice with AP. A recent study showed that EGCG significantly attenuates l-arginine-induced AP and subsequent lung injury in mice.15 These previous results are consistent with our findings, despite differences in construction of the AP model and mode of EGCG administration. Different methods for model construction can better illustrate the universal effects of EGCG in AP. Mechanism studies showed that EGCG can block NLRP3 inflammasome activation by inhibiting mitochondrial ROS production to reduce oxidized mitochondrial DNA production, thereby reducing mitochondrial DNA binding to NLRP3.15 However, the target genes of EGCG in inhibiting mitochondrial ROS production remain unknown. In addition, previous studies showed that EGCG can inhibit the activation of various protein kinases, including members of the MAPK family, particularly Akt kinase.52 EGCG can also regulate a variety of major pathways, including activator protein 1, NF-κB, and phosphatidylinositol-3-hydroxy kinase/protein kinase B pathways.48,53–55 For example, EGCG can inhibit activator protein 1-dependent transcriptional activity by inhibiting c-Jun NH2-terminal kinase-dependent56; EGCG also inhibits the degradation of IκB and activation of NF-κB.57 We identified numerous target genes involved in ROS and oxidative stress; exploration of these targets is time consuming and labor intensive. Although we cannot rule out that EGCG reduced the severity of AP through other pathways, we demonstrated the effect of EGCG on NE expression and NET formation. In terms of the administration method, Luo et al.15 administered EGCG via the intragastric route. However, because of the low oral bioavailability of EGCG and longer gavage time aggravating discomfort in mice, we used intraperitoneal injection and shortened the duration to 1 week. Additionally, intraperitoneal injection of 100 mg/kg/day EGCG was previously shown to result in liver injury in mice.58 Based on these previous results, we adjusted the intraperitoneal injection dose to 50 mg/kg/day. As related studies are limited, further investigations are needed to explore the relevant intrinsic mechanisms.
Systemic inflammation in patients with SAP is typically characterized by elevated levels of proinflammatory cytokines in the circulation.5 IL-6 is an important mediator of SAP-induced lung injury, and its effects are not species specific. In addition, IL-6 stimulates production of the neutrophil chemoattractant CXCL1.59 Therefore, we examined the expression levels of IL-6 and CXCL1 in the local pancreatic tissue and peripheral blood serum. IL-6 and CXCL1 were significantly elevated in SAP, and their expression levels were significantly reduced by administration of GW311616 and EGCG. These results suggest that GW311616 and EGCG can effectively control the systemic and local levels of IL-6 and CXCL1 while reducing NET formation. In addition, IL-6 expression can be reduced by inhibiting NET formation or NE expression.5,60 Although CXCL1 may be directly regulated by NETs or indirectly regulated by IL-6 levels, the mechanism remains to be clarified in-depth in future studies. Numerous studies reported that a large amount of IL-1β is produced in SAP and exacerbates the severity and mortality of pancreatitis,61–63 which is consistent with our results. In addition, we found that GW311616 and EGCG significantly reduce systemic and local IL-1β levels. Studies reported that NE can induce macrophages to produce IL-1β,64 and NET formation can increase IL-1β production by promoting the activation of NLRP3.65 In addition, EGCG can inhibit the expression of NE and NET formation as well as inhibit the activation of NLRP3.15 Therefore, both inhibiting the expression of NE and reducing NET formation can reduce the severity of AP to the greatest extent, but the mechanism underlying NET-regulated IL-1 β production should be further investigated. Notably, the expression level of the anti-inflammatory cytokine IL-10 in the peripheral blood serum was not correlated with that in local pancreatic tissues within 24 h of SAP onset. Previous studies showed that the early proinflammatory response in SAP is often accompanied by an anti-inflammatory response,29,66,67 with high expression of serum IL-10 as a marker of this response. However, within the initial 24 h of SAP, no significant expression of anti-inflammatory cytokines was found during the regulation of local inflammation in the pancreas. The high proinflammatory cytokine production indicated a bias in the inflammatory response towards a proinflammatory state in the local pancreatic tissue. Therefore, further studies should focus on the regulatory relationship between NETs and inflammatory responses.
Our study had some limitations. First, this was a preliminary exploration; we only used cerulein and LPS for model establishment, with a simple operation, short processing time, and good stability and repeatability. Second, invasive interventions are rarely performed in the early stages of SAP, and the optimal timing of invasive interventions is unclear,1 making it difficult to obtain human pancreatic tissue samples; accordingly, we did not use human tissues in this study. Despite these limitations, our results have important implications. Particularly, our results revealed that NET formation in AP requires the MPO-NE pathway, and citrullination of histone H3 is affected by NE activity. In addition, EGCG can effectively inhibit NE activity, reduce NET formation, and attenuate tissue damage and the systemic inflammatory response in AP. This study improves the understanding of the mechanism by which EGCG inhibits NET formation and provides diverse options for preventing and treating AP.
ACKNOWLEDGMENTS
We would like to thank Professor Yan He of Harbin Medical University for providing guidance on the experimental techniques. We would like to thank Ms. Wenjia Kou of the Second Affiliated Hospital of Harbin Medical University for help with blood collection. We would like to thank Editage (www.editage.cn) for English language editing.
AUTHORSHIP
H. L., C. Q., L. Z., and Q. J. performed experiments, interpreted results, and contributed to the writing. H. L., Y. Z., and D. X. conceived and designed the study and contributed to the writing. All authors approved the final manuscript.
FUNDING
This study was supported by grants from the National Natural Sciences Foundation of China (82170654).
DISCLOSURE
The authors declare that they have no conflicts of interest.
REFERENCES
Abbreviations
- AP
acute pancreatitis
- EGCG
epigallocatechin-3-gallate
- GO
Gene Ontology
- IHC
immunohistochemistry
- KEGG
Kyoto Encyclopedia of Genes and Genomes
- MPO
Myeloperoxidase
- NE
neutrophil elastase
- NET
neutrophil extracellular trap
- PAD4
protein arginine deiminase 4
- RFU
relative fluorescence units
- ROS
reactive oxygen species
- SAP
severe acute pancreatitis
- TCMSP
Traditional Chinese Medicine Systems Pharmacology GW311616 (3aR,6S,6aS)−4-methylsulfonyl-1-[(E)−4-piperidin-1-ylbut-2-enoyl]−6-propan-2-yl-3,3a,6,6a-tetrahydro-2H-pyrrolo [3,2-b] pyrrol-5-one
Author notes
Summary Sentence: We identified the functions by which epigallocatechin-3-gallate effectively inhibits neutrophil elastase activity, reduces neutrophil extracellular trap formation, and attenuates systemic inflammatory response in acute pancreatitis.