-
PDF
- Split View
-
Views
-
Cite
Cite
Carolina G Dolislager, Sean M Callahan, Dallas R Donohoe, Jeremiah G Johnson, Campylobacter jejuni induces differentiation of human neutrophils to the CD16hi/CD62Llo subtype, Journal of Leukocyte Biology, Volume 112, Issue 6, December 2022, Pages 1457–1470, https://doi.org/10.1002/JLB.4A0322-155RR
- Share Icon Share
Abstract
The discovery of neutrophil subtypes has expanded what is known about neutrophil functions, yet there is still much to learn about the role of these subtypes during bacterial infection. We investigated whether Campylobacter jejuni induced differentiation of human neutrophils into the hypersegmented, CD16hi/CD62Llo subtype. In addition, we investigated whether C. jejuni-dependent differentiation of this neutrophil subtype induced cancer-promoting activities of human T cells and colonocytes, which were observed in other studies of hypersegmented, CD16hi/CD62Llo neutrophils. We found that C. jejuni causes a significant shift in human neutrophil populations to the hypersegmented, CD16hi/CD62Llo subtype and that those populations exhibit delayed apoptosis, elevated arginase-1 expression, and increased reactive oxygen species production. Furthermore, incubation of C. jejuni-infected neutrophils with human T cells resulted in decreased expression of the ζ-chain of the TCR, which was restored upon supplementation with exogenous l-arginine. In addition, incubation of C. jejuni-infected neutrophils with human colonocytes resulted in increased HIF-1α stabilization and NF-κB activation in those colonocytes, which may result in the up-regulation of protumorigenic genes.
INTRODUCTION
Neutrophils are the most abundant leukocyte in the human body and are often the first immune cells recruited to the site of infection. At this site, neutrophils exert various antimicrobial functions such as phagocytosis, degranulation, reactive oxygen species (ROS) production, and the generation of neutrophil extracellular traps (NETs). Importantly, many of these activities are potent inducers of inflammation and can damage surrounding host tissue.1 Neutrophils were once thought to be homogeneous, short-lived, and transcriptionally inert; however, the discovery of neutrophil subtypes has shown that neutrophils are more diverse and complex than previously thought.2 Neutrophil subtypes with antitumorigenic or protumorigenic characteristics were discovered in tumor-associated neutrophil (TAN) populations, which led to the distinction of N1 and N2 TAN subtypes, respectively.3 Experimental administration of endotoxin to patients resulted in neutrophil subtypes that are characterized by their nuclear morphology (banded, segmented, or hypersegmented) and expression levels of the cell surface markers CD16 (Fc-gamma III receptor) and CD62L (l-selectin).4,5 Neutrophils expressing high levels of both CD16 and CD62L are typically associated with segmented nuclei with approximately 4 lobes and are most commonly isolated from the bloodstream under normal conditions.5 Neutrophils expressing low levels of CD16 and high levels of CD62L present with banded nuclei, tend to be immature, and are released from the bone marrow in instances of trauma and injury.6,7 Neutrophils expressing low levels of CD16 and CD62L are often associated with aging and apoptosis.5 Of particular interest in many studies are neutrophils that highly express CD16, express low levels of CD62L, and possess hypersegmented nuclei. These hypersegmented, CD16hi/CD62Llo neutrophils have been observed in response to several conditions such as sterile inflammation (endotoxemia), septic shock, cancer, viral infection with lymphocytic choriomeningitis virus, and bacterial infection with Helicobacter pylori.4–11 These neutrophils have been demonstrated to have a proteome distinct from banded and segmented neutrophils.12
Once differentiated, CD16hi/CD62Llo neutrophils can impact the adaptive immune system through elevated production of arginase-1 and ROS, both of which can reduce expression of the ζ-chain of the TCR, which negatively impacts the ability of T cells to become activated and proliferate.13 Arginase-1 is an enzyme possessed by granulocytes that converts l-arginine into l-ornithine and urea, which leads to l-arginine depletion. This depletion reduces expression of TCRζ since T cells must import adequate amounts of extracellular l-arginine to properly express TCRζ and proliferate.14,15 Moreover, T cells require large amounts of l-arginine during activation.16 ROS also reduce TCRζ expression, which has been reported to occur via a reduction in mRNA levels and/or direct alteration of proteins without affecting other TCR components like CD3ε (CD3).17 To our knowledge, the only bacteria that has been observed to promote CD16hi/CD62Llo subtype differentiation is H. pylori, where these neutrophils were also shown to have an extended lifespan.11 In our study, we investigated whether the closely related gastrointestinal pathogen Campylobacter jejuni induces similar effects to build the field's understanding of bacterially induced differentiation of neutrophil subtypes and their potential downstream effects on the host.
Campylobacter spp. are a leading cause of bacterially derived gastroenteritis worldwide, with infection often presenting as a self-limiting, moderate-to-severe inflammatory diarrhea.18,19 While H. pylori-associated gastric cancer has been well established, and despite the similarities and the prevalence of Campylobacter spp. infection, much less is known about the relationship between Campylobacter spp. and cancer.20–22
Recent studies have examined the microbiota of colonic polyps and healthy marginal tissues (HMTs) using 16S rRNA gene sequencing, finding that Campylobacter genus sequences are enriched in colonic polyps of Italian, Canadian, Chinese, and Spanish cohorts when compared with the HMTs.23–25 One study has provided molecular evidence for a link between C. jejuni and colorectal cancer by infecting germ-free ApcMin/+mice with both wild-type C. jejuni and a cytolethal distending toxin (CDT) mutant, followed by administration of 1% dextran sulfate sodium for 10 days. They found that infection with wild-type C. jejuni resulted in increased formation and size of tumors in the distal colon compared with both uninfected mice and mice infected with a CDT mutant, indicating that C. jejuni may promote colorectal tumors through the action of CDT. This group also demonstrated that DNA damage was increased in enteroids infected with wild-type C. jejuni compared with those infected with the CDT mutant, providing a potential explanation for the mechanism by which C. jejuni CDT causes tumorigenesis.26 Although some of these studies suggest there is an association between C. jejuni infection and colorectal cancer, there is still very little information as to whether this is truly causative and what the mechanism of tumorigenesis might be following infection in humans, which underscores the need for more studies in this area.
Important targets to investigate early in the development of colon cancer in response to bacterial infection are hypoxia-inducible factor-1 (HIF-1) and activation of the p65 (also called relA) subunit of the NF-κB family of transcription factors by phosphorylation, as both facilitate transcription of hundreds of protumorigenic and cancer progression genes.27 HIF-1 is a transcriptional regulator that results in the up-regulation of hundreds of genes, including those involved in angiogenesis, cell survival and proliferation, metabolism, and tumor metastasis. As a result, elevated HIF-1 is often observed in cancer cells. The HIF-1α subunit is rapidly degraded under normal oxygen conditions, but under hypoxic conditions, HIF-1α is stabilized and leads to HIF-1 promoting expression of its targets.28 Because hypoxia often occurs as a result of inflammation during bacterial infection, increased HIF-1 activation can occur in host tissues. Inflammation through the activation of NF-κB is also common during bacterial infection. The NF-κB family of transcription factors consists of 5 subunits, including p65 (also called RelA), whose activation by phosphorylation results in the up-regulation of many proinflammatory and protumorigenic targets, including genes involved in cell survival, cell proliferation, angiogenesis, tumor metabolism, and tumor metastasis.29,30 Like HIF-1, NF-κB is activated during tumor development and progression and is often elevated in cancer cells.31 Hypoxia and inflammation caused by bacterial infection could result in tumorigenesis in host tissues via the actions of HIF-1 and NF-κB, a process that, to our knowledge, has yet to be studied in the context of C. jejuni infection.32
In this study, we demonstrate that primary human neutrophils differentiate into discrete subtypes following interaction with C. jejuni in a dose- and time-dependent manner, including a majority that differentiated into the CD16hi/CD62Llo subtype. We visually determined that a significant number of primary human neutrophils exhibited nuclear hypersegmentation during interaction with C. jejuni. These neutrophil populations were also found to exhibit reduced apoptosis when compared with uninfected neutrophils. Because the hypersegmented, CD16hi/CD62Llo neutrophil subtype has been suggested to inhibit T cells through increased production of arginase-1 and ROS, we quantified these molecules following interaction of C. jejuni with primary human neutrophils and found both were significantly elevated.4,13,14 Such a result suggests that following interaction with C. jejuni, neutrophils may contribute to the creation of a protumorigenic environment through inhibition of T cells. To determine the impact of hypersegmented, CD16hi/CD62Llo neutrophils on T cells, we measured TCRζ expression in Jurkat T cells after coincubation with C. jejuni-infected neutrophils and observed significantly reduced expression of TCRζ. To determine whether arginase-1 or ROS from C. jejuni-infected neutrophils was impacting TCRζ expression, we supplemented the media with either l-arginine to counteract the action of arginase-1 or l-ascorbic acid to counteract the action of ROS. We found that supplementation with l-arginine but not l-ascorbic acid restored TCRζ in Jurkats incubated with C. jejuni-infected neutrophils. Last, because CD16hi/CD62Llo neutrophils may impact human colonocytes at the site of infection, we similarly incubated human HCT-116 colonocytes with C. jejuni-induced CD16hi/CD62Llo neutrophils and observed significantly increased stabilization of the HIF-1α subunit and phosphorylation of the p65 subunit of NF-κB in colonocytes.30 Taken together, these results suggest that neutrophil subtype differentiation in response to C. jejuni infection could facilitate protumorigenic conditions within colonocytes while simultaneously inhibiting the T cell response. This study is important in progressing our understanding of neutrophil subtypes and C. jejuni’s association with colorectal cancer, both of which are understudied and relatively new fields.
MATERIALS AND METHODS
Strains and culture conditions
C. jejuni strain 81–176 was grown for 48 h at 37°C in microaerobic conditions (85% N2, 10% CO2, and 5% O2) on Mueller–Hinton agar supplemented with 10% sheep's blood and 10 μg/ml trimethoprim. The invasion-deficient C. jejuni strain ΔflgE was grown for 48 h at 37°C in microaerobic conditions (85% N2, 10% CO2, and 5% O2) on Mueller–Hinton agar supplemented with 10% sheep's blood and 10 μg/ml trimethoprim. H. pylori strain 60190 was grown for 72 h at 37°C in microaerobic conditions on Mueller–Hinton agar supplemented with 10% sheep's blood.
Isolation of primary human neutrophils
Human neutrophils were isolated from venous blood from healthy adult volunteers as described previously and in accordance with the Institutional Review Board at the University of Tennessee (UTK IRB-18-04604-XP).33 Venous blood was drawn into EDTA-coated vacutainers before being added to 20 ml 1× PBS. Then, 10 ml lymphocyte separation medium was underlaid before centrifugation at 1400 rpm for 30 min with the brake off. Everything but the red blood cell and neutrophil pellet was aspirated off, and the pellet was resuspended in 20 ml Hank's balanced salt solution and 20 ml 3% dextran in 0.9% sodium chloride. After a 20-min incubation at room temperature, the upper layer containing neutrophils was added to a clean tube and centrifuged at 400×g for 5 min. The supernatant was decanted, and the cell pellet washed by resuspending in 20 ml 0.2% sodium chloride, then 20 ml 1.6% sodium chloride was added before centrifuging again at 400×g for 5 min. The supernatant was decanted, and the cell pellet was washed again as described in the previous step. After centrifugation at 400×g for 5 min, the cell pellet was resuspended in RPMI + 10% FBS and neutrophils were enumerated, and viability was determined using Trypan blue.
Infection of neutrophils with bacteria
Bacterial cells were suspended in RPMI 1640 + 10% FBS and normalized to an OD600 = 1.0 before being serially diluted and plated for enumeration of CFU. Unless otherwise specified, neutrophils were infected with the bacterial strains mentioned above in 200 μl RPMI 1640 + 10% FBS at a multiplicity of infection (MOI) of 1:1 (1 neutrophil to 1 bacterial cell) under microaerobic conditions at 37°C.
Flow cytometry analysis of neutrophil populations
Neutrophils were prepared for flow cytometry as previously described.33 Unless specified otherwise, 106 neutrophils were used for each replicate. After infection, neutrophils were centrifuged at 400×g for 5 min, washed 3 times in 1× PBS, then incubated with Live/Dead Near-IR stain (Thermo). Cells were then blocked in SuperBlock blocking buffer in PBS (Thermo) before incubation with antibodies against APC conjugated CD16 (Cat. no. 302012) and Pacific Blue™ conjugated CD62L (304826) (Biolegend). Cells were then fixed in fixation buffer (Biolegend) and stored in FACS buffer until analysis. 2 × 105 neutrophils were analyzed and data were processed using BD and FlowJo software.
Fluorescent microscopy of neutrophil nuclear morphology
Fluorescent microscopy was performed to assess nuclear morphology. Briefly, 106 neutrophils were added to 35 mm poly-d-lysine coated coverslip dishes (MatTek) in a total volume of 500 μl RPMI 1640 + 10% FBS and incubated under microaerobic conditions at 37°C for 1 h before 106C. jejuni cells were added in 100 μl RPMI 1640 + 10% FBS to a final MOI of 1:1. After incubation for 0, 1, 5, and 24 h under microaerobic conditions at 37°C, the cells were fixed in fixation buffer (Biolegend), washed 3 times in 1× PBS, permeabilized with 0.5% Triton X-100, washed again in 1× PBS, incubated in SuperBlock blocking buffer in PBS (Thermo) for 30 min in microaerobic conditions at 37°C, incubated in 5 μM SYTOX Green in blocking buffer (Invitrogen) for 1 h in microaerobic conditions at 37°C, washed in 1× PBS, and secured with a drop of Mowiol (Sigma) mounting medium. Coverslips were stored in the dark at 4°C until they were imaged using both fluorescent and brightfield channels. At least 150 individual neutrophils were evaluated for their nuclear morphology per coverslip and timepoint.
Detection of apoptotic neutrophils
After infection, neutrophils were collected by centrifugation and washed 3 times with 1× PBS before being resuspended in 150 μl Annexin binding buffer (Invitrogen). Five microliters of Annexin-V AlexaFluor 488 (Invitrogen) was added to each sample and incubated at room temperature for 15 min in the dark. Neutrophils were then fixed in fixation buffer (Biolegend) and stored in the dark at 4°C until flow cytometry analysis, where 2 × 104 cells were analyzed. Data were processed using BD and FlowJo software.
Measuring HIF-1α and NF-κB in colonocytes following coincubation with infected neutrophils
HCT-116 colonic epithelial cells were regularly maintained in DMEM + 10% FBS + 1% l-glutamine + 1% Pen-strep in 5% CO2 at 37°C. HCT-116s were seeded overnight in a 24-well tissue culture plate at a density of 106 cells per well in 1 ml DMEM + 10% FBS + 1% l-glutamine + 1% Pen-strep. Before coincubation with neutrophils, the cells were washed in 1× PBS and 200 μl RPMI 1640 + 10% FBS were added. Subsequently, C. jejuni alone, 100 μM cobalt (II) nitrate, or 105 neutrophils that had been uninfected or infected with C. jejuni for 5 h at an MOI of 1:1, were added to the HCT-116s and incubated for 1 h in 5% CO2 at 37°C. After 1 h, the supernatants were removed from the adherent HCT-116s, thoroughly washed 3 times with 1× PBS, and then HCT-116 proteins were collected and analyzed by Western blot as described below.
Western blot analysis of neutrophil and colonocyte proteins
After incubation alone or with C. jejuni, 106 neutrophils were pelleted at 400×g and collected in equal volumes 1× RIPA cell lysis buffer and 2× Laemmli sample buffer. After thoroughly washing away neutrophils, 106 HCT-116s were collected in 1× RIPA cell lysis buffer and 2× Laemmli sample buffer after thorough washing as described above. Proteins were separated on 10% SDS-PAGE gels and transferred at 250 mA for 2 h onto nitrocellulose membranes using transfer buffer consisting of glycine, SDS, and Tris base. The membranes were blocked in 5% nonfat dry milk in TBS-T, then incubated in one of the following antibodies (diluted 1:1000): anti-arginase-1 (Cat. no. 93668), anti-HIF-1α (Cat. no. 31716), anti-p65 (Cat. no. 3034), anti-phosphorylated p65 (Cat. no. 3033), or anti-β-actin (Cat. no. 4970S) (Cell Signaling Technology) before detection with the appropriate HRP-conjugated secondary antibody (diluted 1:2000) and Pico-Western luminol/enhancer solution. Densitometry data for Western blots were calculated using ImageJ software and normalized to β-actin.
Quantification of ROS from neutrophil populations
After infecting with C. jejuni for 5 or 24 h, 200 μl 1 mM nitroblue tetrazolium chloride was added to 106 primary human neutrophils and incubated for 1 h at 37°C under microaerobic conditions. Cells were then collected by centrifugation, resuspended, and lysed in 200 μl 2 M potassium hydroxide, and then solubilized in 200 μl DMSO. One hundred microliters of the resulting solution was then added to a 96 well plate, and the absorbance was measured at 620 nm.34,35 ROS were also measured using H2DCFDA dye. After infecting neutrophils with C. jejuni for 5 or 24 h, 2 μl of 1 mM H2DCFDA was added and allowed to incubate for 15 min. 2 × 105 cells were then processed for flow cytometry as described above and analyzed via flow cytometry immediately. Data were processed using BD and FlowJo software.
Measuring TCR ζ-chain expression following coincubation with infected neutrophils
Jurkat T cells were regularly maintained in RPMI 1640 + 10% FBS + 1% l-glutamine + 1% Pen-strep in 5% CO2 at 37°C. Before coincubation, 106 Jurkats were preincubated in 200 μl RPMI 1640 + 10% FBS that was unsupplemented or supplemented with 1.5 mM l-arginine, 30 μM l-ascorbic acid, or 1 mM l-ascorbic acid. These Jurkats were then incubated overnight with either C. jejuni alone or with 106 neutrophils that had either been uninfected or infected with C. jejuni for 5 h in 200 μl RPMI 1640 + 10% FBS supplemented with 1.5 mM l-arginine, 30 μM l-ascorbic acid, or 1 mM l-ascorbic acid. 2 × 105 cells were then analyzed via flow cytometry as described above using antibodies against CD247 (TCRζ) and CD3 (CD3ε) (Biolegend). Data were processed using BD and FlowJo software.
Statistical analysis
Unless otherwise stated, data were analyzed by one-way ANOVA with Tukey's post-hoc test. Flow cytometry data of CD16 and CD62L cell surface marker expression were analyzed by two-way multiple comparison testing performed using ANOVA with Tukey's post-hoc test. Arginase-1 Western blot data were analyzed by Mann–Whitney t-test. Statistical analysis was performed using Prism 7 software.
RESULTS
CD16hi/CD62Llo subtype differentiation of C. jejuni-infected neutrophils is MOI and time dependent
Neutrophil subtypes are often characterized by CD16 and CD62L cell surface marker expression and nuclear morphology. Neutrophils that highly express both CD16 and CD62L are most commonly isolated from the bloodstream and are associated with the archetypal 3–4 lobed nuclei. Neutrophils that express low levels of CD16 but high levels of CD62L are associated with immature neutrophils with banded nuclei. Apoptotic neutrophils often display low levels of CD16 and CD62L.5 Neutrophils that express high levels of CD16 and low levels of CD62L display hypersegmented nuclei and have been shown to have immunosuppressive effects in other systems, like in sepsis and cancer.8,9 As persistent Campylobacter infections and reinfection with Campylobacter are observed, we suspect that the adaptive immune system may be suppressed in Campylobacter infection; however, very little is known about this.36,37 Furthermore, hypersegmented, CD16hi/CD62Llo neutrophils have also been observed in H. pylori infection, a close relative of C. jejuni, which can also present as a persistent infection; however, it has not been elucidated whether hypersegmented, CD16hi/CD62Llo neutrophils as a result of H. pylori infection have immunomodulatory effects.11
To determine whether subtypes are observed during C. jejuni infection of primary human neutrophils, we used flow cytometry to characterize the neutrophil populations based on the abundance of CD16 and CD62L on individual cells. Initially, we used various MOIs, including 50:1 (bacterial cells-to-neutrophils), 20:1, 5:1, 1:1, and 1:5 (Figures 1(A) and S1). After a 5-h incubation, we observed that the majority (61.5%) of uninfected neutrophils displayed CD16hi/CD62Lhi cell surface marker expression, which are archetypal neutrophils. At 50:1, the majority (65.6%) of neutrophils displayed CD16lo/CD62Lhi cell surface markers, whereas at 20:1, 48.3% of neutrophils were CD16lo/CD62Lhi and 48.1% of neutrophils were CD16lo/CD62Llo. In contrast, at an MOI of 5:1 45.6% were CD16hi/CD62Llo. At 1:1, CD16hi/CD62Llo neutrophils increased to 64.5%. At an MOI of 1:5, 70.5% of neutrophils were CD16hi/CD62Llo. This shows a trend that lower MOIs result in the largest population of CD16hi/CD62Llo neutrophils. Overall, these data indicate that the neutrophil response to C. jejuni is dependent on bacterial concentrations, which lends remarkable evidence to the plasticity of neutrophils, a concept only recently demonstrated.38 In addition, since C. jejuni infection can be established at low doses, this MOI is likely physiologically relevant to C. jejuni infection.38 Because an MOI of 1:1 induced differentiation of a majority of the neutrophil population to the CD16hi/CD62Llo phenotype, the remainder of the experiments were performed at an MOI of 1:1.
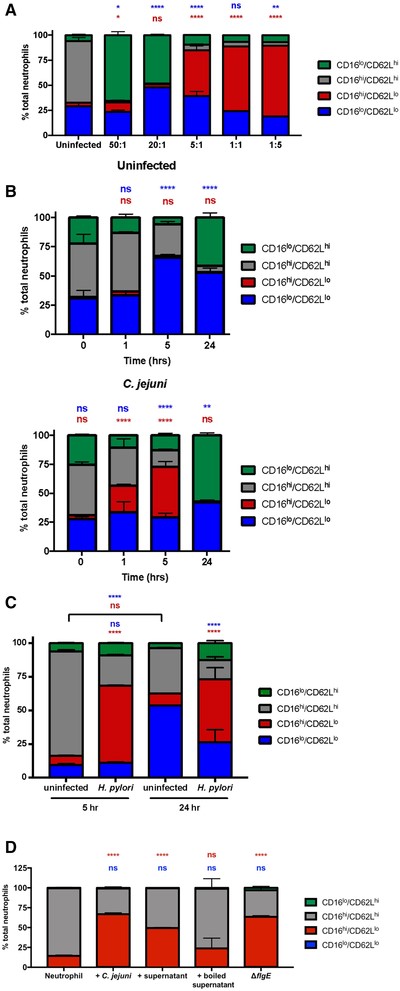
C. jejuni induction of CD16hi/CD62Llo neutrophils is multiplicity of infection (MOI) and time dependent. (A) Neutrophils were left uninfected or infected with C. jejuni at the indicated varying MOIs for 5 h. Expression of CD16 and CD62L was measured after 5 h. (B) Neutrophils were left uninfected or infected with C. jejuni at an MOI of 1:1. Expression of CD16 and CD62L was measured at 0, 1, 5, and 24 h. (C) Neutrophils were left uninfected or infected with H. pylori at an MOI of 1:1. Expression of CD16 and CD62L was measured at 5 and 24 h. (D) Neutrophils were left uninfected or infected with C. jejuni at an MOI of 1:1, coincubated with C. jejuni supernatant, coincubated with boiled C. jejuni supernatant, or infected with an invasion-deficient mutant strain of C. jejuni at an MOI of 1:1. Expression of CD16 and CD62L was measured at 5 h. Representative graphs, n = 3, separate donors. Percentage of neutrophils are displayed as mean + sem. Multiple comparison testing was performed using ANOVA with Tukey's post-hoc test. *p < 0.05, **p < 0.01, ***p < 0.001, ****p < 0.0001 compared with (A) uninfected, (B) 0 h uninfected, (C) uninfected at corresponding timepoints, and (D) uninfected
To determine how time impacts differentiation to the CD16hi/CD62Llo subtype, neutrophils were infected with C. jejuni at an MOI of 1:1 for 0, 1, 5, and 24 h (Figure 1(B)). CD16hi/CD62Llo neutrophils represented 8.1% of the uninfected population at the 0-h timepoint, and 11.8% of the C. jejuni-infected neutrophil population. At 1 h, 5.7% of uninfected neutrophils were CD16hi/CD62Llo, and 23.1% of C. jejuni-infected neutrophils were CD16hi/CD62Llo. By 5 h, 5.2% of uninfected neutrophils were CD16hi/CD62Llo, whereas C. jejuni-infected neutrophils exhibited a significant increase in the CD16hi/CD62Llo population at 35.5%. By 24 h of infection with C. jejuni, very little of the neutrophil population exhibited the CD16hi/CD62Llo phenotype with 1.9% of uninfected neutrophils and 0.4% of C. jejuni-infected neutrophils.
To test this assay using a bacterial strain that has already been found to induce CD16hi/CD62Llo neutrophil differentiation, H. pylori 60190 was used to infect primary human neutrophils and these cells were similarly analyzed by flow cytometry (Figure 1(C)). We observed that H. pylori-infected neutrophils exhibited a significant increase in CD16hi/CD62Llo neutrophils at 5 h (57.4%) and 24 h (46.8%) when compared with uninfected neutrophils at the same timepoints (6.8 and 8.9%, respectively). These results confirm those of previous studies and support the methodology used to generate the observations that C. jejuni infection leads to significant differences in CD16hi/CD62Llo neutrophil populations, albeit for less time, since this cell surface marker expression is absent by 24 h postinfection.
We then wanted to see if C. jejuni conditioned media could also induce CD16hi/CD62Llo subtype differentiation (Figure 1(D)). To test this, we added filtered or filtered and boiled cell-free supernatants from C. jejuni to neutrophils and allowed them to incubate for 5 h. Neutrophils incubated unboiled C. jejuni supernatant showed a significant increase in the CD16hi/CD62Llo subtype; however, no significant change in CD16hi/CD62Llo neutrophils was observed for neutrophils incubated with boiled C. jejuni supernatant. This suggests that a C. jejuni secreted factor may be responsible for neutrophil subtype differentiation. Further, when neutrophils were incubated with an invasion-deficient strain of C. jejuni for 5 h, we observed a significant increase in CD16hi/CD62Llo neutrophils, suggesting that C. jejuni does not need to be intracellular to induce neutrophil subtype differentiation.
C. jejuni-infected neutrophil populations exhibit higher rates of hypersegmented nuclear morphology
Previous studies demonstrated that CD16hi/CD62Llo neutrophils possess hypersegmented nuclei, with the number of nuclear lobes often exceeding 4.5 To determine whether the subtype differentiation observed above also leads to changes in nuclear morphology of C. jejuni-infected neutrophils, we stained the neutrophil nuclei with Sytox and examined them by fluorescent microscopy (Figure 2). The incidence of hypersegmentation of the neutrophil nucleus, defined as 5 or greater nuclear lobes, was determined for uninfected and C. jejuni-infected neutrophils at 0, 1, 5, and 24 h postinfection (Figure 3(A)). At 0 h, 6.4% of uninfected neutrophils and 8.0% of C. jejuni-infected neutrophils exhibited hypersegmented nuclei. At 1 h, 5.3% of uninfected neutrophils and 7.1% of C. jejuni-infected neutrophils had hypersegmented nuclei. The incidence of hypersegmented nuclei in C. jejuni-infected neutrophils was not significantly different when compared with uninfected neutrophils until the 5 h timepoint, which saw an increase in the incidence of hypersegmentation in C. jejuni-infected neutrophils (20.3%) over uninfected (3.1%). At 24 h, a significant increase in the percentage of hypersegmented nuclei was also observed in C. jejuni-infected neutrophils (10.9%) when compared with uninfected (1.5%) cells. Importantly, most of the uninfected neutrophils at 24 h had condensed, unlobed nuclei (an average of 1.1 lobes per neutrophil), which is indicative of apoptosis; however, C. jejuni-infected neutrophil populations exhibited a significantly increased incidence of hypersegmentation at 24 h, as well as significantly more nuclear lobes on average (2.7 lobes per neutrophil), which indicates prolonged viability (Figure S2). When this microscopy data were compared with the expression of CD16 and CD62L in similar neutrophil populations, a discrepancy emerged at 24 h since nuclear hypersegmentation was present for C. jejuni-infected neutrophils despite only 0.4% of the neutrophil population exhibiting the CD16hi/CD62Llo phenotype. We hypothesize that shedding of the CD16 marker occurs between 5- and 24-h postinfection with C. jejuni without altering the appearance of the nuclei or the other properties described below; however, further studies should be done to investigate the mechanisms behind this and the differences in CD16 expression at 24 h during C. jejuni and H. pylori infection.
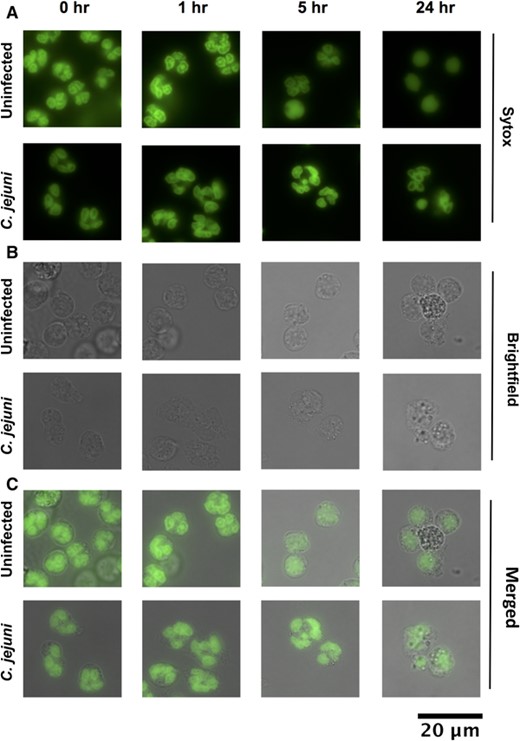
Morphology of neutrophil nuclei upon C. jejuni infection. (A) Green fluorescence, DNA stained with Sytox. (B) Brightfield. (C) Merge. Hypersegmentation is defined as > 4 nuclear lobes. Condensed, 1 lobed nuclei are indicative of apoptosis. 63×. Representative images, n = 3, separate donors, > 150 neutrophils scored per timepoint
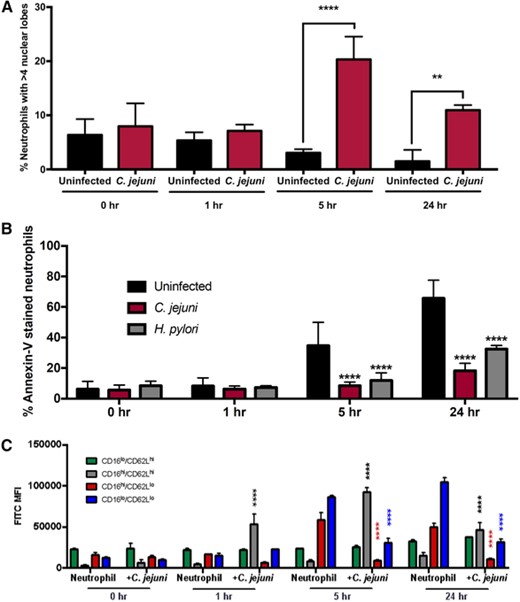
Incidence of hypersegmentation increases and apoptosis decreases at 5 and 24 h in C. jejuni-infected neutrophils. (A) Occurrence of hypersegmentation of neutrophil nuclei as measured at 0, 1, 5, and 24 h in uninfected and C. jejuni-infected neutrophils (MOI 1:1). Hypersegmentation is defined as > 4 nuclear lobes. (B) Occurrence of apoptosis as measured by Annexin-V staining at 0, 1, 5, and 24 h in uninfected, C. jejuni- and H. pylori-infected neutrophils (MOI 1:1). (C) Occurrence of apoptosis per neutrophil subtype as measured by Annexin-V staining and expression of CD16 and CD62L at 0, 1, 5, and 24 h in uninfected and C. jejuni-infected neutrophils (MOI 1:1). Percentage of neutrophils are displayed as mean + sem. One-way ANOVA with Tukey's post-hoc test. *p < 0.05, **p < 0.01, ***p < 0.001, ****p < 0.0001 compared with uninfected at corresponding timepoints. (A) n = 3, separate donors, > 150 neutrophils scored per timepoint, (B) n = 3, separate donors, representative graph, and (C) n = 2, separate donors, representative graph
C. jejuni-infected neutrophils exhibit reduced apoptosis
Because delayed apoptosis was observed for hypersegmented neutrophils that resulted from infection with H. pylori,11 we examined whether C. jejuni-infected neutrophils exhibited reduced apoptosis at 0, 1, 5, and 24 h postinfection (Figure 3(B)). No significant changes in apoptosis were observed at the 0 and 1 h timepoints. In contrast, apoptosis was significantly decreased at 5 and 24 h postinfection for neutrophils infected with C. jejuni or H. pylori when compared to uninfected neutrophils. Specifically, at 5 h, 34.7% of uninfected neutrophils were apoptotic, whereas 8.5 and 11.9% of C. jejuni- or H. pylori-infected neutrophils, respectively, were apoptotic. At 24 h, the majority of uninfected neutrophils (65.7%) were apoptotic, whereas only 18.3% of C. jejuni-infected neutrophils and 32.4% of H. pylori-infected neutrophils were apoptotic. These results from C. jejuni- and H. pylori-infected neutrophil populations were significantly different when compared with uninfected neutrophil populations. Significantly delayed neutrophil apoptosis upon infection with C. jejuni is important because it not only further supports that hypersegmented, CD16hi/CD62Llo neutrophils are induced during C. jejuni infection, but also that extending the life of neutrophils may be responsible for the immunopathologic effects that occur during human C. jejuni infection.
To confirm that CD16hi/CD62Llo neutrophils were specifically displaying delayed apoptosis, we looked at apoptosis via Annexin-V staining within each neutrophil subtype. Indeed, we observed significantly delayed apoptosis in CD16hi/CD62Llo neutrophils at both 5 and 24 h in C. jejuni-infected neutrophils compared with uninfected neutrophils at both timepoints (Figure 3(C)).
C. jejuni-infected neutrophils exhibit increased arginase-1 expression and ROS production
Hypersegmented, CD16hi/CD62Llo neutrophils have been previously shown to negatively impact T cell activation and proliferation by reducing expression of the ζ-chain of the TCR through either the production of arginase-1 or the generation of ROS.13 To determine whether the hypersegmented, CD16hi/CD62Llo neutrophils induced by C. jejuni infection could impact T cells, we first examined the production of arginase-1 and ROS within infected neutrophil populations.
To examine arginase-1 production, C. jejuni was used to infect primary human neutrophils under the same conditions where a majority of the population exhibits the CD16hi/CD62Llo phenotype. As expected, a significant, approximately 2-fold increase of arginase-1 was observed for C. jejuni-infected neutrophil (density relative to β-actin = 0.9) whole cell lysates when compared with those from uninfected neutrophils (density relative to β-actin = 0.4) (Figures 4(A) and 4(B)). As hypersegmented, CD16hi/CD62Llo neutrophils have also been shown to reduce TCRζ expression by elevated production of ROS, we used nitroblue tetrazolium chloride to examine the ability of C. jejuni-infected neutrophils to produce ROS. Nitroblue tetrazolium chloride is one of the most common methods to measure the respiratory burst and intracellular ROS production in neutrophils. Reduction of nitroblue tetrazolium chloride by ROS forms an indigo-colored, insoluble formazan precipitate, which is then solubilized and whose absorbance is measured.35 Using the same conditions where a majority of the infected neutrophil population exhibits the CD16hi/CD62Llo phenotype, a significant, 3-fold increase in ROS production was observed when compared with uninfected neutrophils (Figure 4(C)). To further support our hypothesis that the CD16hi/CD62Llo neutrophil subtype is specifically responsible for the increase in ROS and not a general response to C. jejuni infection of neutrophils, neutrophils were infected with C. jejuni at an MOI of 20:1 for 5 h and ROS was similarly measured. This is due to the above observation that infection of neutrophils with C. jejuni for 5 h at an MOI of 20:1 results in predominantly CD16lo/CD62Lhi and CD16lo/CD62Llo neutrophil populations. As a result, neutrophils infected at an MOI of 20:1 produced approximately 70% of the ROS produced by neutrophils infected with an MOI of 1:1, which supports other studies demonstrating the unique ability of CD16hi/CD62Llo neutrophils to produce elevated amounts of ROS (Figure S3). We then used H2DCFDA dye to quantify relative intracellular ROS production per subtype at 5 and 24 h. At 5 h of C. jejuni infection, there was a significant increase in ROS production by CD16hi/CD62Llo and CD16hi/CD62Lhi neutrophils compared with uninfected neutrophils at 5 h. At 24 h of C. jejuni infection, there was a significant increase in ROS production by CD16hi/CD62Lhi neutrophils and an increase in ROS production by CD16hi/CD62Llo neutrophils compared with uninfected neutrophils at 24 h, although this increase was not significant. CD16hi/CD62Lhi neutrophils produced the most ROS at both 5 and 24 h, followed by the CD16hi/CD62Llo subtype; however, because there is a greater percentage of CD16hi/CD62Llo neutrophils at both 5 and 24 h, CD16hi/CD62Llo neutrophils may be greater contributors to ROS generation than CD16hi/CD62Lhi neutrophils (Figure 4(D)). As we have shown the CD16hi/CD62Llo subtype is induced by a heat-labile factor and not phagocytosis, we hypothesize that the elevated ROS is not due to bacterial internalization. Furthermore, while ROS generation by neutrophils is classically a rapid process, it is interesting how prolonged the production of ROS is by CD16hi/CD62Llo neutrophils and what kind of bystander effects this may have on regional colonocytes.
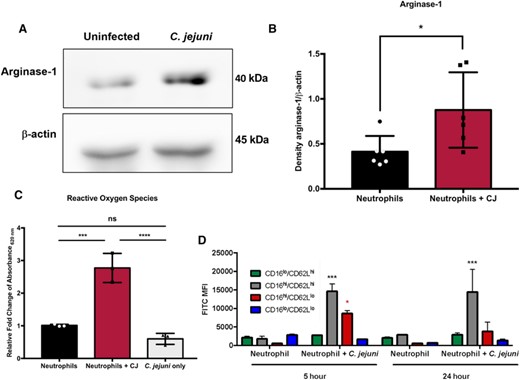
Arginase-1 expression and reactive oxygen species production are increased in neutrophils infected with C. jejuni (MOI 1:1) for 5 h when compared to uninfected neutrophils. (A) Western blot of arginase-1 and β-actin of uninfected and C. jejuni-infected neutrophil lysates. (B) Densitometry of arginase-1 relative to β-actin. (C) Relative fold-change of reactive oxygen species production as measured using NBT compared with uninfected neutrophils from absorbance at 620 nm values. (D) Abundance of reactive oxygen species per neutrophil subtype as measured by H2DCFDA staining and expression of CD16 and CD62L at 5 and 24 h in uninfected and C. jejuni-infected neutrophils (MOI 1:1). Densitometry and fold change displayed as mean + SEM. (B) Mann–Whitney test and (C) one-way ANOVA. *p < 0.05, **p < 0.01, ***p < 0.001, ****p < 0.0001 compared with uninfected. (A) Representative image, n = 3, separate donors, (B) n = 3, separate donors, (C) n = 3, separate donors, and (D) n = 2, separate donors
Coincubation of C. jejuni-infected neutrophils with human T cells leads to reduced TCRζ expression
To determine whether hypersegmented, CD16hi/CD62Llo neutrophils induced by C. jejuni infection impact the TCR, we incubated neutrophils with C. jejuni under conditions where a majority differentiate to the CD16hi/CD62Llo phenotype. We then incubated these neutrophils with human T cells overnight and examined for TCRζ expression by flow cytometry (Figures 5 and S4). From this analysis, we observed that 73.5% of T cells possessed detectable TCRζ following coincubation with C. jejuni-infected neutrophils when compared with T cells alone (100%). This is a significant reduction in the TCRζ expressing population, including when compared to T cells incubated with uninfected human neutrophils (99.0%) or with C. jejuni alone (96.0%) (Figure 5(A)). To determine whether increased arginase-1 production by C. jejuni-infected neutrophils was responsible for the reduction in TCRζ expression, T cells were supplemented with 1.5 mM l-arginine for 24 h before and during coincubation to counteract the effects of arginase-1. This concentration of l-arginine was chosen as it has been shown that 1–2 mM l-arginine maximally activates CD3+ T cells.39,40 In the presence of l-arginine, TCRζ expression of T cells incubated with uninfected neutrophils (105.1%), with C. jejuni-infected neutrophils (89.5%), or with C. jejuni alone (101.1%) was not significantly decreased when compared with T cells alone supplemented with l-arginine (100%) (Figure 5(B)).
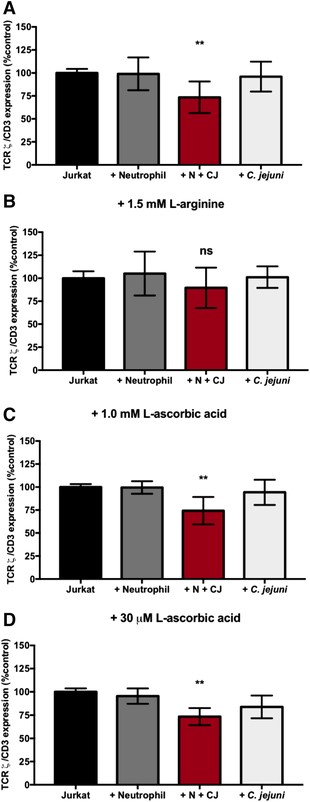
TCRζ chain reduction in Jurkats incubated with C. jejuni-infected neutrophils is restored in the presence of 1.5 mM l-arginine, not 1.0 mM or 30 μM l-ascorbic acid. (A) TCRζ expression relative to CD3 normalized to Jurkats alone in RPMI 1640 + 10% FBS. (B) TCRζ expression relative to CD3 normalized to Jurkats alone in RPMI 1640 + 10% FBS + 1.5 mM l-arginine. (C) TCRζ expression relative to CD3 normalized to Jurkats alone in RPMI 1640 + 10% FBS + 1.0 mM l-ascorbic acid. (D) TCRζ expression relative to CD3 normalized to Jurkats alone in RPMI 1640 + 10% FBS + 30 μM l-ascorbic acid. %TCRζ expression expressed as mean + SEM. One-way ANOVA with Tukey's post-hoc test. *p < 0.05, **p < 0.01, ***p < 0.001, ****p < 0.0001 compared with uninfected (A) n = 5, separate donors, (B) n = 3, separate donors, (C) n = 3, separate donors, and (D) n = 3, separate donors
Because ROS produced by hypersegmented, CD16hi/CD62Llo neutrophils is elevated at 24 h when TCRζ expression is reduced and because ROS has been shown in previous studies to inhibit TCRζ expression, T cells were supplemented with either 1 mM or 30 μM l-ascorbic acid, an antioxidant, before and during coincubation to counteract ROS produced by C. jejuni-infected neutrophils. These concentrations were chosen, as 1 mM is a supraphysiologic concentration of l-ascorbic acid in T cells, whereas 30 μM is a physiologically normal concentration of l-ascorbic acid in T cells and serum.41 The addition of either concentration of l-ascorbic acid did not affect TCRζ expression when compared with T cells incubated without supplementation. Specifically, upon addition of 1 mM l-ascorbic acid, we observed no significant changes to the T cell population expressing TCRζ when incubated with uninfected neutrophils (99.5%) or incubated with C. jejuni alone (94.3%) when compared with T cells alone (100%). However, like our unsupplemented group, we still observed a significant decrease in TCRζ expression in T cells when incubated with C. jejuni-infected neutrophils (74.3%) despite the addition of 1 mM l-ascorbic acid (Figure 5(C)). This result was also observed upon supplementation with 30 μM l-ascorbic acid. For example, a significant decrease in the T cell population expressing TCRζ was observed following incubation with C. jejuni-infected neutrophils (73.4%) and no significant differences were detected following incubation of T cells with uninfected neutrophils (95.5%) or C. jejuni alone (83.9%) when compared with T cells alone supplemented with 30 μM l-ascorbic acid (Figure 5(D)). This suggests that elevated ROS production by C. jejuni-infected neutrophils is not responsible for reduced TCRζ production. Although we cannot definitely state that the hypersegmented neutrophil subtype is directly responsible for the various phenotypes within T cells, we hypothesize the correlated functions of this neutrophil subtype (increased arginase-1 and ROS), could likely be responsible.
Coincubation of C. jejuni-infected neutrophils with human colonocytes induces HIF-1α stabilization and phosphorylation of NF-κB
Beyond impacts to T cells, the significantly increased production of ROS and inflammation from hypersegmented, CD16hi/CD62Llo neutrophils may also affect adjacent colonocytes. To determine the potential effects of CD16hi/CD62Llo neutrophil-derived ROS production on the colonic epithelium, we first examined HIF-1α stabilization in colonocytes. HIF-1 is a transcription factor that promotes the expression of several systems involved in tumorigenesis, and ROS has been shown to increase HIF-1α subunit stabilization.42 We incubated predominantly CD16hi/CD62Llo neutrophil populations or uninfected neutrophils with colonocytes for 1 h and examined for HIF-1α stabilization by Western blot. In addition, we treated colonocytes with cobalt (II) as a positive control since it has been shown to stabilize HIF-1α independent of hypoxia.43 Colonocytes incubated with C. jejuni-infected neutrophils, which are predominantly the CD16hi/CD62Llo subtype, exhibited an approximately 2-fold increase in HIF-1α stabilization (density relative to β-actin = 1.8), whereas incubation of colonocytes with C. jejuni alone (density relative to β-actin = 0.90) or uninfected neutrophils (density relative to β-actin = 0.95) failed to produce any significant changes in HIF-1α stabilization when compared with colonocytes alone (density relative to β-actin = 0.96). In contrast, colonocytes incubated with cobalt (II) nitrate exhibited a 3.5-fold increase in HIF-1α (density relative to β-actin = 3.4) (Figures 6(A) and 6(B)). We hypothesize that increased production of ROS by hypersegmented, CD16hi/CD62Llo neutrophils that are induced in response to C. jejuni may be responsible for increased stabilization of HIF-1α in these colonocytes, which may lead to transcription of protumorigenic genes targets of HIF-1.
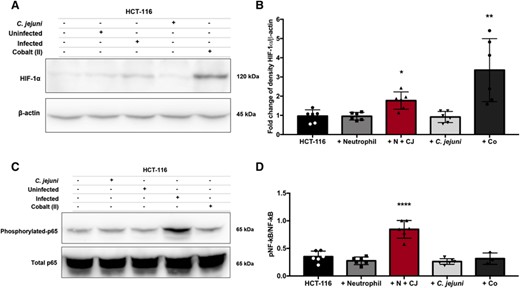
HIF-1α stabilization and p65 phosphorylation of NF-κB are increased in HCT-116 colonocytes coincubated with C. jejuni-infected neutrophils. (A) Western blot of HIF-1α and β-actin of HCT-116 colonocyte cell lysates alone or after 1-h coincubation with uninfected neutrophils, neutrophils infected with C. jejuni (MOI 1:1) for 5 h, C. jejuni alone, or cobalt (II) nitrate as a positive control. (B) Densitometry of HIF-1α relative to β-actin. (C) Western blot of phosphorylated p65 subunit of NF-κB relative to the total p65 subunit of NF-κB of HCT-116 colonocyte cell lysates alone or after 1-h coincubation with uninfected neutrophils, C. jejuni alone, neutrophils infected with C. jejuni (MOI 1:1) for 5 h, or cobalt (II) nitrate. (D) Densitometry of the phosphorylated p65 subunit of NF-κB relative to the total p65 subunit of NF-κB. Densitometry displayed as mean + sem. (A) Nonparametric test and (B) one-way ANOVA. *p < 0.05, **p < 0.01, ***p < 0.001, ****p < 0.0001 compared with uninfected. (A) Representative image, n = 3, separate donors, (B) n = 3, separate donors, (C) representative image, n = 3, separate donors, and (D) n = 3, separate donors
HIF-1 and NF-κB have been shown to interact during infection and inflammation, as NF-κB is often activated when HIF-1α is stabilized.44 Because we have demonstrated that HIF-1α is stabilized in colonocytes incubated with C. jejuni-infected neutrophils, we also examined NF-κB activation through phosphorylation of p65 in these colonocytes (Figures 6(C) and 6(D)). As expected, phosphorylation of p65 was significantly increased (2.4-fold) in colonocytes incubated with neutrophil populations where the CD16hi/CD62Llo subtype is the majority. In contrast, colonocytes incubated with uninfected neutrophils or C. jejuni alone showed no significant change in phosphorylation of p65. This was somewhat surprising since other groups have demonstrated C. jejuni alone induces NF-κB activation in colonocytes.45 Instead, our results suggest that neutrophil activity, including the induction of the hypersegmented, CD16hi/CD62Llo subtype, may be responsible for increased inflammation and resulting pathology of host intestinal tissue. Colonocytes incubated with cobalt (II) nitrate showed no significant change in phosphorylation of p65.
DISCUSSION
Infection by Campylobacter species is a significant cause of gastrointestinal disease worldwide that has both acute and long-term implications to human health, including the development of postinfectious inflammatory disorders and possibly colorectal cancer.18,19,23–26 Importantly, the bacterial and host factors that are responsible for these various outcomes are mostly unknown. For the field to advance in understanding the processes that contribute to Campylobacter-induced diseases, it is important that we begin to thoroughly characterize the host responses to these important pathogens and how those processes impact the host. We have demonstrated in this study that the majority of human neutrophils differentiate into a hypersegmented, CD16hi/CD62Llo subtype after being exposed to low doses of C. jejuni and that the neutrophil population exhibits delayed apoptosis, increased expression of arginase-1, and elevated production of ROS. Not only do these neutrophil activities support our initial observations that C. jejuni infection induces differentiation of the hypersegmented, CD16hi/CD62Llo subtype, but it also suggests how immunopathology during campylobacteriosis might occur. For example, the decreased apoptosis of neutrophil populations we observed during infection likely leads to prolonged exposure of the surrounding gastrointestinal tissues to the activities of neutrophils.1,46 This includes several processes and products we previously examined that may directly damage host cells or are exceedingly proinflammatory, including the release of S100A12, lipocalin-2, myeloperoxidase, and neutrophil elastase.47,48 While CD16hi/CD62Llo subtype neutrophils have been shown to occur in normal, nonhypersegmented, activated neutrophils, we demonstrate that this subtype could lead to established effects on other cell types. However, more research must be done on the effect of various C. jejuni-induced neutrophil subtypes and the role these cell types could play during infection, along with isolation of specific subtypes to examine their role of other cellular processes. Beyond these more classical responses, our current work suggests that a portion of the neutrophil population may be inappropriately immunosuppressive and counteract specific components of adaptive immunity (e.g., T cells). In addition, it is particularly interesting to note that induction of the hypersegmented, CD16hi/CD62Llo neutrophil subtype is greatest when there are 1 or fewer bacteria per neutrophil, since we previously demonstrated that NET formation occurs when there are ten or greater C. jejuni cells to one neutrophil.48 Interestingly, we find that induction of these hypersegmented neutrophils are dependent on a heat-labile factor from C. jejuni. Unlike other Gram-negative pathogens, C. jejuni solely encodes a flagellar type III secretion system (T3SS).49,50 While some effector proteins have been identified in C. jejuni, the role of the T3SS and the identification of other effector proteins remain largely understudied. These results indicate that neutrophils dynamically respond to stimuli at the site of infection, which is in stark contrast to early impressions of neutrophils as terminally differentiated and transcriptionally limited cells and suggests that host responses and disease outcomes may be dependent on Campylobacter burden.
Further, hypersegmented, CD16hi/CD62Llo neutrophils as well as myeloid-derived suppressor cells (MDSCs) produce elevated levels of arginase-1 and ROS.13 In order to further classify the hypersegmented, CD16hi/CD62Llo neutrophil differentiation we observed in response to C. jejuni infection and to investigate what effects the neutrophil subtype may have on the host during campylobacteriosis, we examined arginase-1 expression and ROS production. As predicted, we found that both were elevated in neutrophils that had been incubated with C. jejuni under conditions where a majority develop into the hypersegmented, CD16hi/CD62Llo subtype. The ability of this neutrophil subtype and MDSCs to suppress T cell function has been attributed to decreased TCRζ-chain expression, which is caused by either l-arginine depletion via arginase-1 or ROS directly impacting the TCR.14,15,17 Because of this, we subsequently demonstrated that human T cells incubated with these differentiated neutrophil populations exhibited a significant decrease in TCRζ expression, which is important for TCR signaling and proliferation. To preliminarily determine whether arginine depletion or ROS production were responsible for this reduction in TCRζ expression, we supplemented T cells with exogenous l-arginine or an antioxidant (l-ascorbic acid), finding that l-arginine partially restored TCRζ expression in the T cell population while l-ascorbic acid did not. Determining whether arginase-dependent suppression of T cells occurs during C. jejuni infection could provide valuable targets for aiding the adaptive immune system's response to C. jejuni infection. This could help reduce persistent infection or reinfection, aid in vaccine development through the elicitation of an adequate memory response or provide protection against cancer formation by strengthening the adaptive immune response.16 Indeed, dietary supplementation with l-arginine has been shown to increase bacterial clearance and decrease susceptibility to bacterial infections and sepsis.51,52 Better nutrition and increased bacterial clearing as a result of dietary l-arginine in developed countries may explain why persistent Campylobacter infections occur less frequently in developed countries than in developing countries.53,18 The interactions between C. jejuni infection and nutrition, especially arginine, and their global trends are areas that need further investigation.
Beyond their impacts to inflammation and T cell activation, hypersegmented, CD16hi/CD62Llo neutrophils could have direct implications to colonocytes, including colorectal tumorigenesis. Although the links between C. jejuni infection and colorectal cancer is severely understudied, C. jejuni has been proposed to cause colorectal tumors through damage to colonocyte DNA by CDT.26 Furthermore, 16S rRNA gene sequencing studies have shown members of the Campylobacter genus are associated with colorectal polyps and tumors but not HMTs.23–25 These studies suggest that sample type may have impacted the field's ability to associate Campylobacter infection with colorectal tumorigenesis since an initial study that established a correlation does not exist relied on fecal samples for detection.54 If Campylobacter remains restricted to polyp or tumor tissues, as the tissue microbiome studies suggest, culture or PCR of fecal samples may not be sufficient to detect these associations. Further supporting a possible association with colorectal cancer, we found that incubation of colonocytes with neutrophil populations where hypersegmented, CD16hi/CD62Llo cells predominate, HIF-1α stabilization and NF-κB activation occurred, a combination that could lead to up-regulation of hundreds of tumor promoting genes. While increased expression of HIF-1α could occur, we hypothesize increased stabilization of HIF-1α is occurring within colonocytes due to the short incubation time with neutrophils. An unexpected observation from this work was that, despite repeated attempts using various cell lines, C. jejuni strains, and MOIs, we were unable to observe NF-κB activation in the absence of neutrophils (data not shown). In the end, damage to colonocytes at the site of infection directly through C. jejuni effectors like CDT or through the activities of the innate immune response (e.g., NETosis) combined with stabilization of HIF-1α, activation of NF-κB, and decreased T cell function through TCRζ down-regulation, could allow for tumorigenesis to occur and go unchecked. As T cells serve an important role in surveillance of host tissue for cancer, further studies need to be conducted to determine whether immunosuppressive neutrophil subtypes, as well as direct effects of C. jejuni on colonocytes, could be responsible for the development of colorectal cancer.
ACKNOWLEDGMENTS
This work was supported by UTK start-up funds to J. G. J. and USDA NIFA (2019-67017-29261) awarded to D. R. D. The authors would like to thank Trevor J. Hancock, Engin Berber, Jenny K. Heppert, Sarah J. Kauffman, Jaydeep Kolape, Bohye Park, Rachel Patton McCord, Tim Sparer, and Benjamin J. Parker. We would also like to thank Eleanor Mancini and Caroline Parker for their assistance. Graphical abstract was created with BioRender software.
AUTHORSHIP
Conceptualization: C. G. D., S. M. C., D. R. D., and J. G. J. Investigation: C. G. D. Data curation—formal analysis: C. G. D. Writing—original draft: C. G. D. and J. G. J. Writing—review, editing, and revision: C. G. D., D. R. D., and J. G. J.
DISCLOSURE
The authors declare no conflict of interest.
REFERENCES
Abbreviations
- CD
cluster of differentiation
- CDT
cytolethal distending toxin
- HIF-1
hypoxia-inducible factor-1
- HMTs
healthy marginal tissues
- MDSC
myeloid-derived suppressor cell
- MOI
multiplicity of infection
- NET
neutrophil extracellular trap
- ROS
reactive oxygen species
Author notes
Summary sentence: Campylobacter jejuni infection of neutrophils results in differentiation of a subtype that activates HIF-1 and NF-κB in colonocytes and decreases TCRζ in T cells.