-
PDF
- Split View
-
Views
-
Cite
Cite
Vincenzo Condello, Anello M Poma, Elisabetta Macerola, Paola Vignali, Johan O Paulsson, Jan Zedenius, Fulvio Basolo, C Christofer Juhlin, Prevalence, Molecular Landscape, and Clinical Impact of DICER1 and DGCR8 Mutated Follicular-Patterned Thyroid Nodules, The Journal of Clinical Endocrinology & Metabolism, Volume 109, Issue 7, July 2024, Pages 1733–1744, https://doi.org/10.1210/clinem/dgae034
- Share Icon Share
Abstract
Mutations in micro-RNA (miRNA) regulators DICER1 and DGCR8 have recently been uncovered, revealing a potential novel mechanism driving thyroid tumor development. However, the true frequency of these hotspot mutations in follicular-patterned thyroid tumors (FTs) and their relation to established driver gene events remain elusive.
A total of 440 FTs from 2 institutions were interrogated for DICER1, DGCR8, and RAS family hotspot mutations using Sanger sequencing. Whole-exome sequencing was also performed to identify additional driver gene aberrations in DICER1/DGCR8-mutant cases. Subsets of cases were further analyzed using miRNA expression profiling, and key dysregulated miRNAs were validated as markers of DICER1 mutations using quantitative RT-PCR analysis. The Cancer Genome Atlas (TCGA) database was also probed for DICER1/DGCR8 mutations and miRNA dysregulation.
Fourteen (3.2%) and 4 (1%) FTs harbored DICER1 and DGCR8 hotspot mutations, respectively, in the combined cohort, and no cases with normal tissue available were found to exhibit a constitutional variant. Two DGCR8-mutant cases also harbored oncogenic RAS mutations. Whole-exome sequencing analysis did not identify additional driver gene events in DICER1/DGCR8-positive cases. Comprehensive miRNA expression profiling revealed a unique pattern of dysregulated miRNAs in DICER1/DGCR8-mutant cases compared with wild-type lesions. Moreover, DICER1-mutant cases showed a remarkable reduction of 5′ arm miRNAs, findings corroborated in the TCGA cohort.
DICER1 and DGCR8 hotspot mutations are rare in unselected cohorts of FTs, and mutated cases exhibit a specific miRNA profile. Although DGCR8 mutations may coexist with established RAS gene alterations, FTs with DICER1 variants were devoid of other driver gene events.
In the past several decades, the relevance of micro-RNA (miRNA) processing machinery genes has taken center stage in molecular pathology and oncology. Specific gene mutations have been identified in different human cancers, suggesting that aberrations in miRNA expression driven by these mutations might be an important hallmark of tumor development and progression (1). In this respect, thyroid neoplasms may epitomize an attractive prototype to explore because they incorporate different morphological and histopathological subtypes originating mostly from the same cell type, albeit with different grades of differentiation (2).
Germline mutations in DICER1 and DGCR8, two of the main miRNA processing genes regulating miRNA maturation, are responsible for the DICER1 and familial multinodular goiter with schwannomatosis syndromes, respectively (3, 4). Patients with DICER1 syndrome usually exhibit truncating or frameshift DICER1 alterations and may develop a plethora of tumors, including thyroid follicular nodular disease (FND) and well-differentiated thyroid carcinoma (WDTC) (5-9). DGCR8 mutational carriers, on the other hand, often present with FND, whereas the true incidence of thyroid cancer within this syndrome is unknown (4, 10). In addition to germline alterations, subsets of sporadic thyroid tumors display recurrent hotspot mutations in these genes (11-17).
Somatic DICER1 mutations have been reported across the entire spectrum of RAS-driven FTs, such as follicular thyroid adenoma (FTA), noninvasive follicular thyroid neoplasm with papillary-like nuclear features (NIFTP), invasive encapsulated follicular variant papillary thyroid carcinoma (IEFVPTC), follicular thyroid carcinoma (FTC), and rare cases of poorly differentiated thyroid carcinoma (PDTC) arising in pediatric/adolescent patients (8, 13, 18-21). DGCR8 mutations on the other hand seem to aggregate in clinically troublesome cases and have been identified in subsets of FTC and PDTC with a particularly aggressive behavior (16, 17).
In all, DICER1 mutations are believed to be more prevalent than DGCR8 mutations in the sporadic setting. Both DICER1 and DGCR8 are postulated to exhibit tumor-suppressive functions, which is supported by the biallelic inactivation pattern observed in syndromic and sporadic cases. Specifically, biallelic DICER1 mutations are common, and DGCR8 mutations with loss of the remaining allele have been reported (6, 16, 22). For DICER1 specifically, combinations of truncating mutations throughout the gene and hotspot mutations in the functionally important RNAse III region are commonly observed together. Interestingly, although truncating mutations may occur as both germline and somatic events, hotspot alterations are typically exclusively somatic (8).
In terms of functional connotations, analyses of global miRNA expression seem to support an aberrant profile for both DICER1 and DGCR8 mutants, which in turn may have consequences for gene regulation at the posttranscriptional level (16, 22, 23). Also, knock-down of DICER1 mRNA in FTC cell lines promotes proliferation in vitro, thereby further strengthening the hypothesis of these miRNA regulators as tumor suppressor genes (15).
Despite these advances, most of what we know regarding the prevalence of DICER1 and DGCR8 mutations are data amassed from smaller, monoinstitutional characterizations, and other driver gene aberrations are not always accounted for. To counter this, FTs from two European high-volume thyroid cancer centers have been interrogated for DICER1 and DGCR8 hotspot mutations to establish the frequency of these genetic alterations. Additionally, to investigate the impact of these mutations, subsets of DICER1 and DGCR8 mutants were further analyzed for global miRNA output and exome sequenced to establish a potential relationship to other genetic drivers.
Materials and Methods
The workflow of the study is summarized in Fig. 1.
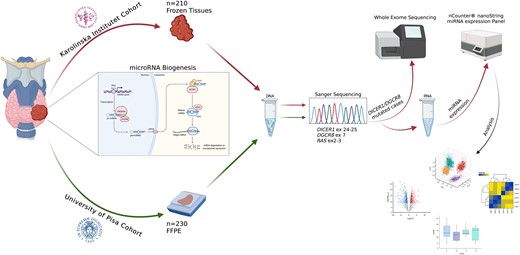
Summary of the tissue material and overall workflow applied in this study. A total of 440 thyroid lesions were included and Sanger sequenced for hotspot mutations in DICER1, DGCR8, and RAS genes. Karolinska Institutet cohort was solely based on fresh-frozen samples; thus, these samples were selected for subsequent whole-exome sequencing and global miRNA profiling. The figure was created with Biorender.com.
Patient Cohort
Tumor samples were obtained from the Karolinska University Hospital, Stockholm, Sweden, and from the Unit of Surgical Pathology of the University of Pisa, Italy. Following approval of the institutional review boards, both institutions were queried for the diagnosis of FTs. This study included archived fresh-frozen tissues (Karolinska) and formalin-fixed and paraffin-embedded tissues (Pisa), totaling 40 FNDs, 127 FTAs, 48 NIFTPs, 1 follicular tumor of uncertain malignant potential (FT-UMP), 80 IEFVPTCs, 126 FTCs, 15 oncocytic thyroid carcinomas (OTC), and 3 differentiated high-grade thyroid carcinomas (DHGTCs). All cases were retrieved and reviewed to confirm the histological diagnosis by two different pathologists (C.C.J. and F.B.). Demographic information including age, sex, and nodule size were collected from each patient at the time of diagnosis, in addition to histopathologic results and tumor staging. In total, 440 FTs with these diagnoses were identified and collected. Single cases with previously established DICER1 (n = 1) and DGCR8 (n = 1) mutations were included for internal control of the Sanger sequencing procedure, in which the laboratory investigator was blinded of the genotype (15, 16). Constitutional DNA from normal thyroid tissue was later acquired for all cases with subsequently verified DICER1 or DGCR8 mutations, except for 2 pediatric cases in the Karolinska cohort for which normal tissue samples were not available.
Nucleic Acids Isolation
For all included samples for which fresh-frozen tissues and formalin-fixed and paraffin-embedded were available, genomic DNA was extracted using DNeasy Blood and Tissue kit (Qiagen, Hilden, Germany) according to the manufacturer’s instructions, and concentration was evaluated using a spectrophotometer (NanoDrop 2000; Thermo Fisher Scientific, Waltham, MA).
Total RNA, including miRNAs, was isolated using miRNeasy Mini Kit (Qiagen) according to the manufacturer's instructions. The RNA concentration was assessed using a spectrophotometer (NanoDrop 2000; Thermo Fisher Scientific), and the quality control was assessed using the RNA 6000 nano kit (Bioanalyzer, Agilent, Santa Clara, CA). Only samples with a concentration ≥30 ng/μL, a ratio between the value of the absorbance at 260 nm and the absorbance at 280 nm was ≥1.8, and a ratio between the value of absorbance at 260 nm and that at 230 nm was ≥2 and with an RNA integrity number ≥ 7 were included for the analysis.
Mutational Analysis
The hotspot mutational status of DICER1 (exons 24-25), DGCR8 (exon 7), and N-H-KRAS (exons 2-3) genes was investigated by direct sequencing (ABI 3730 DNA Analyzer, Applied Biosystems, Foster City, CA, USA) according to standard procedures in all 440 cases. Only DICER1/DGCR8-positive cases were further interrogated for the PAX8::PPARG fusion. All the primers used for sequencing are reported in Supplementary Table S1 (24). The paired normal tissues available from the contralateral lobe or the adjacent normal parenchyma of lesions harboring mutations in DICER1 and DGCR8 were used to confirm the somatic or germline nature of the variants according to current guidelines (25). All chromatograms were read in full, so amplified nucleotide sequences adjacent to hotspot regions were also assessed.
Whole Exome Sequencing
Thirteen samples (8 DICER1 or DGCR8 mutated tumors and 5 corresponding normal tissues) were submitted for whole exome sequencing (Novogene, Cambridge, UK). For 2 DICER1 mutant cases (T4 and T5), normal DNA was unavailable. For 1 DGCR8 mutated tumor (T8), constitutional DNA was not submitted because this case was previously screened for germline involvement in a separate study (16). Briefly, genomic DNA of 8 tumor samples and 5 corresponding normal tissue samples was physically fragmented and prepared as libraries containing dual-indexed sequencing barcodes. The precapture libraries were enriched using Exome 2.0 Panel (Twist Bioscience) for coding regions and splice junction sites of 20 000 human genes. The postcapture libraries were sequenced using NovaSeq 6000 (Illumina). The sequence data were analyzed using a custom-developed bioinformatics pipeline that aligns sequence data to human genome (GRCh37/GRCh38) to perform variant calls and annotations. The pipeline quality control of the sequence data was performed to ensure the quality of the data and variant annotations were performed with Variant Effect Predictor tool.
MicroRNA Profiling
A total of 24 samples were submitted for miRNA expression analysis, including 5 DICER1 mutants, 2 DGCR8 mutants, 15 wild-type tumors, and 2 normal thyroid samples. We also incorporated global miRNA profiling data acquired from a third DGCR8 mutant case from an earlier publication using the same platform, bringing the total number of analyzed samples to 25. All wild-type samples were selected based on similar clinicopathologic features as the mutated cases.
The nCounter miRNA Expression Panel (nanoString Technologies, Seattle, WA, USA) was used for the analysis. The panel includes specific probes for 827 human miRNAs, 6 positive mRNA controls, 8 negative mRNA controls, 3 ligation-positive miRNA controls, 3 ligation-negative miRNA controls, 5 mRNA reference controls, and 5 spike-in controls.
Briefly, approximately 100 ng of total RNA from each fresh-frozen tissue sample were hybridized with probes containing unique barcodes and counted using the nCounter platform according to the manufacturer's instructions. Initial data quality control and data analysis were performed using nSolver v.4 (nanoString Technologies) and Rosalind (www.rosalind.bio). Data were normalized using the mean expression of the 100 most expressed miRNAs in the dataset. To select miRNA highly expressed among samples, the mean plus 2 SDs of negative control probes was used as threshold, and targets detected in less than 50% of samples were filtered out, as performed previously (26).
Real-time RT-PCR
cDNA was synthesized using TaqMan MicroRNA Reverse Transcription Kit (Applied Biosystems) with assay-specific TaqMan primers, miR-135a-5p (hsa-miR-135a, 000460), miR-135b-5p (hsa-miR-135b, 002261), miR-181a-3p (hsa-miR-213, 000516), and the control assay RNU6B (001093) (Applied Biosystems) according to the manufacturer's instructions. Samples were run in QuantStudio1 Real-Time PCR System (Applied Biosystems) in triplicate with assay-specific TaqMan primers stated previously, and expression levels were calculated using the 2−ΔΔCt method. Validation was only performed on DICER1 mutated cases because they constituted the majority of the samples submitted for global miRNA analyses.
Comparative Analyses in The Cancer Genome Atlas
Level 3 miRNA expression and clinical data of The Cancer Genome Atlas (TCGA) (27) on thyroid cancer were downloaded from Firehose (https://gdac.broadinstitute.org/). For downstream analyses, RPM log2 data of mature miRNA expression was used. Unsupervised hierarchical clustering was carried out using Euclidean distance, Ward clustering method, and following the procedures of heatmap3 R package v.1.1.9. The proportion of 5p and 3p miRNA strands were defined as the cumulative 5p and 3p miRNA levels in total. Differentially expressed miRNA (DEM) were computed by the Bayesian moderated t-statistics and following the procedures of the limma Bioconductor package v.3.56.2. P values were adjusted with the Benjamini-Hochberg method. A false discovery rate (FDR) below 0.05 was deemed significant. Venn diagrams were drawn to highlight overlapping DEM between DICER1 and DGCR8 mutant cases. Analyses and plots were generated in R environment (v.4.1.2, https://www.r-project.org/, last accessed June 22, 2023).
Statistical Analyses
Differential expression analysis was calculated using nSolver v.4 and Rosalind softwares (nanoString Technologies). P values were adjusted with the Benjamini-Hochberg method. An FDR <0.05 was considered significant. Chi-square and Mann-Whitney U tests were used to compare clinicopathological features and a P value <.05 was considered significant. Statistical computations were performed using RStudio v12.0 and GraphPad Prism 9.5.1 (GraphPad Software, San Diego, CA, USA).
Results
Baseline Patient Characteristics
Patient and nodule characteristics are shown in detail in Table 1. The combined cohort from both institutions included a total of 440 patients with FTs, of which 38% were classified as benign (40 FND, 127 FTA), 11% low-risk (48 NIFTP, 1 follicular tumor of uncertain malignant potential), and 51% malignant (80 EFVPTC, 126 FTC, 15 oncocytic thyroid carcinomas, and 3 DHGTC). The mean age was 48.8 ± 16.7 years, and 72.3% (n = 319) were female. Only 6 cases (1.3%) were considered pediatric/adolescent patients (ranged in age from 10 to 18 years).
Clinicopathological characteristics of 440 follicular-patterned thyroid nodules included in the cohort
. | Benign, n = 167 (38%) . | Low-Risk, n = 49 (11%) . | Malignant, n = 224 (51%) . | . | |||||
---|---|---|---|---|---|---|---|---|---|
. | FND n = 40 . | FTA n = 127 . | NIFTP n = 48 . | FT-UMP n = 1 . | EFVPTC n = 80 . | FTC n = 126 . | OTC n = 15 . | DHGTC n = 3 . | All samples n = 440 . |
Sex | |||||||||
Female | 32 | 88 | 37 | 1 | 56 | 94 | 8 | 3 | 319 |
Male | 8 | 39 | 11 | / | 23 | 33 | 7 | / | 121 |
Age, y, mean ± SD | 58.2 ± 15 | 48.5 ± 15 | 47.3 ± 12.7 | 49 | 48.1 ± 15.7 | 53.4 ± 19.3 | 50.2 ± 17.3 | 51 ± 22.6 | 48.8 ± 16.7 |
Nodule size, cm, mean ± SD | NA | 3.2 ± 1.5 | 2.8 ± 1.5 | 7 | 2.6 ± 1.3 | 4 ± 2.2 | 3.9 ± 1.9 | 8.4 ± 7 | 3.3 ± 1.8 |
Tumor invasiveness | |||||||||
Minimally invasive | / | / | / | / | 50 | 57 | 8 | n.a. | 115 |
Widely invasive | / | / | / | / | 19 | 34 | 5 | n.a. | 58 |
Angioinvasive | / | / | / | / | 4 | 36 | 2 | n.a. | 42 |
Infiltrative | / | / | / | / | 7 | / | / | n.a. | 7 |
. | Benign, n = 167 (38%) . | Low-Risk, n = 49 (11%) . | Malignant, n = 224 (51%) . | . | |||||
---|---|---|---|---|---|---|---|---|---|
. | FND n = 40 . | FTA n = 127 . | NIFTP n = 48 . | FT-UMP n = 1 . | EFVPTC n = 80 . | FTC n = 126 . | OTC n = 15 . | DHGTC n = 3 . | All samples n = 440 . |
Sex | |||||||||
Female | 32 | 88 | 37 | 1 | 56 | 94 | 8 | 3 | 319 |
Male | 8 | 39 | 11 | / | 23 | 33 | 7 | / | 121 |
Age, y, mean ± SD | 58.2 ± 15 | 48.5 ± 15 | 47.3 ± 12.7 | 49 | 48.1 ± 15.7 | 53.4 ± 19.3 | 50.2 ± 17.3 | 51 ± 22.6 | 48.8 ± 16.7 |
Nodule size, cm, mean ± SD | NA | 3.2 ± 1.5 | 2.8 ± 1.5 | 7 | 2.6 ± 1.3 | 4 ± 2.2 | 3.9 ± 1.9 | 8.4 ± 7 | 3.3 ± 1.8 |
Tumor invasiveness | |||||||||
Minimally invasive | / | / | / | / | 50 | 57 | 8 | n.a. | 115 |
Widely invasive | / | / | / | / | 19 | 34 | 5 | n.a. | 58 |
Angioinvasive | / | / | / | / | 4 | 36 | 2 | n.a. | 42 |
Infiltrative | / | / | / | / | 7 | / | / | n.a. | 7 |
Abbreviations: DHGTC, differentiated high-grade thyroid carcinoma; EFVPTC, encapsulated follicular variant papillary thyroid carcinoma; FND, follicular nodular disease; FTA, follicular thyroid adenoma; FTC, follicular thyroid carcinoma; FT-UMP, follicular tumor of uncertain malignant potential; NA, not available; NIFTP, noninvasive follicular thyroid neoplasm with papillary-like nuclear features; OTC, oncocytic thyroid carcinoma.
Clinicopathological characteristics of 440 follicular-patterned thyroid nodules included in the cohort
. | Benign, n = 167 (38%) . | Low-Risk, n = 49 (11%) . | Malignant, n = 224 (51%) . | . | |||||
---|---|---|---|---|---|---|---|---|---|
. | FND n = 40 . | FTA n = 127 . | NIFTP n = 48 . | FT-UMP n = 1 . | EFVPTC n = 80 . | FTC n = 126 . | OTC n = 15 . | DHGTC n = 3 . | All samples n = 440 . |
Sex | |||||||||
Female | 32 | 88 | 37 | 1 | 56 | 94 | 8 | 3 | 319 |
Male | 8 | 39 | 11 | / | 23 | 33 | 7 | / | 121 |
Age, y, mean ± SD | 58.2 ± 15 | 48.5 ± 15 | 47.3 ± 12.7 | 49 | 48.1 ± 15.7 | 53.4 ± 19.3 | 50.2 ± 17.3 | 51 ± 22.6 | 48.8 ± 16.7 |
Nodule size, cm, mean ± SD | NA | 3.2 ± 1.5 | 2.8 ± 1.5 | 7 | 2.6 ± 1.3 | 4 ± 2.2 | 3.9 ± 1.9 | 8.4 ± 7 | 3.3 ± 1.8 |
Tumor invasiveness | |||||||||
Minimally invasive | / | / | / | / | 50 | 57 | 8 | n.a. | 115 |
Widely invasive | / | / | / | / | 19 | 34 | 5 | n.a. | 58 |
Angioinvasive | / | / | / | / | 4 | 36 | 2 | n.a. | 42 |
Infiltrative | / | / | / | / | 7 | / | / | n.a. | 7 |
. | Benign, n = 167 (38%) . | Low-Risk, n = 49 (11%) . | Malignant, n = 224 (51%) . | . | |||||
---|---|---|---|---|---|---|---|---|---|
. | FND n = 40 . | FTA n = 127 . | NIFTP n = 48 . | FT-UMP n = 1 . | EFVPTC n = 80 . | FTC n = 126 . | OTC n = 15 . | DHGTC n = 3 . | All samples n = 440 . |
Sex | |||||||||
Female | 32 | 88 | 37 | 1 | 56 | 94 | 8 | 3 | 319 |
Male | 8 | 39 | 11 | / | 23 | 33 | 7 | / | 121 |
Age, y, mean ± SD | 58.2 ± 15 | 48.5 ± 15 | 47.3 ± 12.7 | 49 | 48.1 ± 15.7 | 53.4 ± 19.3 | 50.2 ± 17.3 | 51 ± 22.6 | 48.8 ± 16.7 |
Nodule size, cm, mean ± SD | NA | 3.2 ± 1.5 | 2.8 ± 1.5 | 7 | 2.6 ± 1.3 | 4 ± 2.2 | 3.9 ± 1.9 | 8.4 ± 7 | 3.3 ± 1.8 |
Tumor invasiveness | |||||||||
Minimally invasive | / | / | / | / | 50 | 57 | 8 | n.a. | 115 |
Widely invasive | / | / | / | / | 19 | 34 | 5 | n.a. | 58 |
Angioinvasive | / | / | / | / | 4 | 36 | 2 | n.a. | 42 |
Infiltrative | / | / | / | / | 7 | / | / | n.a. | 7 |
Abbreviations: DHGTC, differentiated high-grade thyroid carcinoma; EFVPTC, encapsulated follicular variant papillary thyroid carcinoma; FND, follicular nodular disease; FTA, follicular thyroid adenoma; FTC, follicular thyroid carcinoma; FT-UMP, follicular tumor of uncertain malignant potential; NA, not available; NIFTP, noninvasive follicular thyroid neoplasm with papillary-like nuclear features; OTC, oncocytic thyroid carcinoma.
DICER1 and DGCR8 Mutation Frequencies in Follicular-patterned Thyroid Lesions
To establish an overall mutational frequency, a total of 440 consecutive FTs from the two institutions were interrogated for hotspot mutations in DICER1 and DGCR8 genes using Sanger sequencing. We identified 18 (4.1%) cases carrying hotspot mutations in either DICER1 or DGCR8, and these events were mutually exclusive. Fourteen (3.2%) cases showed hotspot mutations within the RNase IIIb domain of the DICER1 gene, including E1705K (n = 2), and D1709N (n = 1) (exon 24); D1810Y (n = 1), D1810V (n = 4), E1813K (n = 1), E1813G (n = 1), and E1813Q (n = 4) (exon 25). Four (1%) cases showed E518K mutations of the DGCR8 gene, whereas 1 case carried an L516P mutation. The latter alteration is not a previously reported hotspot mutation but was identified when reading through the entire chromatogram. For all the positive cases, including the DGCR8 L516P mutated case, in which constitutional DNA was available (n = 16), the germline/somatic status was tested, showing a somatic origin of all mutations. Point mutations within the RAS gene family were also interrogated by examining codons 12, 13, and 61 for N-H-KRAS. Collectively, 119 (27%) cases showed RAS hotspot mutations, including NRAS (n = 71, 16.1%), HRAS (n = 33, 7.5%), and KRAS (n = 15, 3.4%). Two cases, both DGCR8 mutated, showed N- and HRAS mutations, respectively, whereas the remaining cases did not show any overlap. Results are detailed in Fig. 2.
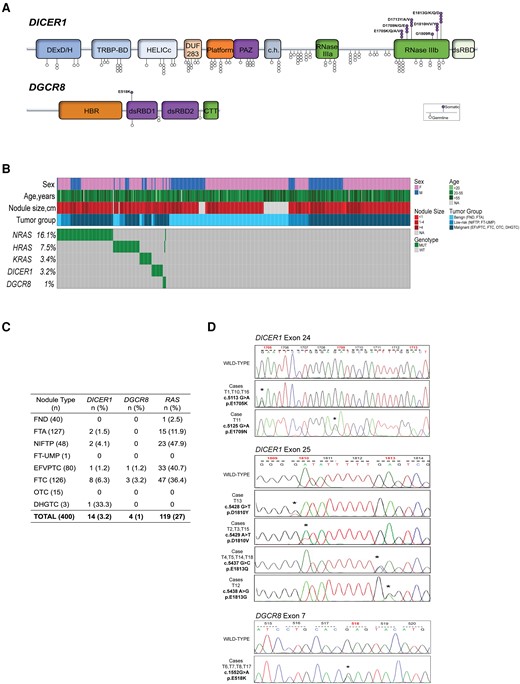
Breakdown of DICER1 and DGCR8 mutational analysis. (A) Lollipop plot highlighting the 6 known somatic hotspot mutations in DICER1 and 1 in DGCR8. Somatic hotspots are illustrated at the top, whereas common germline variants are shown at the bottom of the figure. (B) Oncoprint plot depicting Sanger sequencing results from 440 follicular-patterned thyroid nodules from the bi-institutional cohort. Each column represents 1 sample, and each row represents a gene. (C) Summary of the mutations discovered in the cohort stratified into unique diagnostic entities. (D) Representative chromatograms obtained by Sanger sequencing analysis showing somatic missense mutations in DICER1 and DGCR8 (chromatogram for T17 carrying DICER1 E1813K was not available).
Clinical Characteristics of DICER1 and DGCR8 Mutated Cases
The 18 DICER1/DGCR8 mutated cases included 15 females (83%) with a mean age of 41 ± 19 years (median, 36) and a mean nodule size of 4.3 ± 3.4 cm (median, 3.5). Histopathological data were available for all these patients, but only 8 had clinical follow-up information available of >10 years. The whole spectrum of benign, low-risk, and malignant lesions harbored DICER1 mutations, including 2 FTAs, 2 NIFTPs, 1 angioinvasive EFVPTC, 4 minimally invasive FTCs, 4 angioinvasive FTCs, and 1 DHGTC, whereas 1 minimally invasive EFVPTC and 3 widely invasive FTCs harbored a DGCR8 mutation (Fig. 2C). Cases harboring DICER1 mutations (T1-T5) with a follow-up of >10 years were all alive without recurrent disease at the time of this study. Among all 14 DICER1 mutated cases, 12 (86%) were female and only 2 were pediatric patients (aged 10 and 17 years, respectively), whereas the remaining 12 patients harboring DICER1 mutations had an age ranging from 24 to 77 years. A significant association between DICER1 mutations and younger age at surgery (P = .004) was found.
Of 4 cases harboring DGCR8 mutations, 2 (T6 and T7) showed recurrent disease with metastatic spread to lungs and bone, and both died of the disease 10 and 12 years after the diagnosis, respectively. The third case (T8) presented elevated thyroglobulin levels at the last examination but no structural evidence of relapse or metastases. For the fourth case (T17), no follow-up information was available. Among the 4 DGCR8 mutated cases, 3 (75%) were female and showed a significant association with the larger nodule size (P = .009) compared with the wild-type cases. Histopathologic characteristics of the positive cases are summarized in Table 2.
Case ID . | Sex . | Age at surgery, y . | Nodule size, cm . | Tumor type . | pTNM . | Gene mutation . | RAS . | PAX8::PPARG . |
---|---|---|---|---|---|---|---|---|
T1 | F | 24 | 3.5 | MIFTC | pT2Nx | DICER1 c.5113 G > A p.E1705K | WT | WT |
T2 | F | 29 | 2.4 | FTA | NA | DICER1 c.5429 A > T p.D1810V | WT | WT |
T3 | F | 47 | 3.5 | MIFTC | pT2Nx | DICER1 c.5429 A > T p.D1810V | WT | WT |
T4 | F | 10 | 4.7 | EAIFTC | pT3aNx | DICER1 c.5437 G > C p.E1813Q | WT | WT |
T5 | F | 17 | 5 | EAIFTC | pT3aNx | DICER1 c.5437 G > C p.E1813Q | WT | WT |
T6 | M | 66 | 8 | WIFTC | pT3aNx | DGCR8 c.1552 G > A p.E518K | WT | WT |
T7 | F | 74 | NA | WIFTC | NA | DGCR8 c.1552 G > A p.E518K | WT | WT |
T8 | F | 31 | 5 | WIFTC | pT3 | DGCR8 c.1552 G > A p.E518K | HRAS c.182 A > G p.Q61R | WT |
T9 | F | 50 | 1.5 | NIFTP | / | DICER1 c.5125 G > A p.D1709N | WT | WT |
T10 | F | 35 | 3.5 | NIFTP | / | DICER1 c.5113 G > A p.E1705K | WT | WT |
T11 | F | 18 | 1.6 | EAIFTC | pT1bNxMx | DICER1 c.5125 G > A p.E1709N | WT | WT |
T12 | M | 77 | 16 | DHGTC | pT3aNxM1 | DICER1 c.5438 A > G p.E1813G | WT | WT |
T13 | F | 33 | 1.2 | MIFTC | pT1bNxMx | DICER1 c.5428 G > T p.D1810Y | WT | WT |
T14 | F | 47 | 1.6 | MIFTC | pT1bNxMx | DICER1 c.5437 G > C p.E1813Q | WT | WT |
T15 | F | 54 | 3 | EAIFTC | pT2N0Mx | DICER1 c.5429 A > T p.D1810V | WT | WT |
T16 | F | 37 | 3.9 | AIEFVPTC | pT2N0Mx | DICER1 c.5113 G > A p.E1705K | WT | WT |
T17 | F | 54 | 5 | MIEFVPTC | pT3aNxMx | DGCR8 c.1552 G > A p.E518K | NRAS c.182 A > C p.Q61R | WT |
T18 | M | 35 | 3.4 | FTA | / | DICER1 c.5437 G > A p.E1813Q | WT | WT |
Case ID . | Sex . | Age at surgery, y . | Nodule size, cm . | Tumor type . | pTNM . | Gene mutation . | RAS . | PAX8::PPARG . |
---|---|---|---|---|---|---|---|---|
T1 | F | 24 | 3.5 | MIFTC | pT2Nx | DICER1 c.5113 G > A p.E1705K | WT | WT |
T2 | F | 29 | 2.4 | FTA | NA | DICER1 c.5429 A > T p.D1810V | WT | WT |
T3 | F | 47 | 3.5 | MIFTC | pT2Nx | DICER1 c.5429 A > T p.D1810V | WT | WT |
T4 | F | 10 | 4.7 | EAIFTC | pT3aNx | DICER1 c.5437 G > C p.E1813Q | WT | WT |
T5 | F | 17 | 5 | EAIFTC | pT3aNx | DICER1 c.5437 G > C p.E1813Q | WT | WT |
T6 | M | 66 | 8 | WIFTC | pT3aNx | DGCR8 c.1552 G > A p.E518K | WT | WT |
T7 | F | 74 | NA | WIFTC | NA | DGCR8 c.1552 G > A p.E518K | WT | WT |
T8 | F | 31 | 5 | WIFTC | pT3 | DGCR8 c.1552 G > A p.E518K | HRAS c.182 A > G p.Q61R | WT |
T9 | F | 50 | 1.5 | NIFTP | / | DICER1 c.5125 G > A p.D1709N | WT | WT |
T10 | F | 35 | 3.5 | NIFTP | / | DICER1 c.5113 G > A p.E1705K | WT | WT |
T11 | F | 18 | 1.6 | EAIFTC | pT1bNxMx | DICER1 c.5125 G > A p.E1709N | WT | WT |
T12 | M | 77 | 16 | DHGTC | pT3aNxM1 | DICER1 c.5438 A > G p.E1813G | WT | WT |
T13 | F | 33 | 1.2 | MIFTC | pT1bNxMx | DICER1 c.5428 G > T p.D1810Y | WT | WT |
T14 | F | 47 | 1.6 | MIFTC | pT1bNxMx | DICER1 c.5437 G > C p.E1813Q | WT | WT |
T15 | F | 54 | 3 | EAIFTC | pT2N0Mx | DICER1 c.5429 A > T p.D1810V | WT | WT |
T16 | F | 37 | 3.9 | AIEFVPTC | pT2N0Mx | DICER1 c.5113 G > A p.E1705K | WT | WT |
T17 | F | 54 | 5 | MIEFVPTC | pT3aNxMx | DGCR8 c.1552 G > A p.E518K | NRAS c.182 A > C p.Q61R | WT |
T18 | M | 35 | 3.4 | FTA | / | DICER1 c.5437 G > A p.E1813Q | WT | WT |
Abbreviations: AEIFVPTC, angioinvasive encapsulated follicular variant papillary thyroid carcinoma; AIFTC, angioinvasive follicular thyroid carcinoma; DHGTC, differentiated high-grade thyroid carcinoma; EAIFVPTC, encapsulated angioinvasive follicular variant papillary thyroid carcinoma; MIEFVPTC, minimally invasive encapsulated follicular variant papillary thyroid carcinoma; MIFTC, minimally invasive follicular thyroid carcinoma; NA, not available; WIFTC, widely invasive follicular thyroid carcinoma; WT, wild-type.
Case ID . | Sex . | Age at surgery, y . | Nodule size, cm . | Tumor type . | pTNM . | Gene mutation . | RAS . | PAX8::PPARG . |
---|---|---|---|---|---|---|---|---|
T1 | F | 24 | 3.5 | MIFTC | pT2Nx | DICER1 c.5113 G > A p.E1705K | WT | WT |
T2 | F | 29 | 2.4 | FTA | NA | DICER1 c.5429 A > T p.D1810V | WT | WT |
T3 | F | 47 | 3.5 | MIFTC | pT2Nx | DICER1 c.5429 A > T p.D1810V | WT | WT |
T4 | F | 10 | 4.7 | EAIFTC | pT3aNx | DICER1 c.5437 G > C p.E1813Q | WT | WT |
T5 | F | 17 | 5 | EAIFTC | pT3aNx | DICER1 c.5437 G > C p.E1813Q | WT | WT |
T6 | M | 66 | 8 | WIFTC | pT3aNx | DGCR8 c.1552 G > A p.E518K | WT | WT |
T7 | F | 74 | NA | WIFTC | NA | DGCR8 c.1552 G > A p.E518K | WT | WT |
T8 | F | 31 | 5 | WIFTC | pT3 | DGCR8 c.1552 G > A p.E518K | HRAS c.182 A > G p.Q61R | WT |
T9 | F | 50 | 1.5 | NIFTP | / | DICER1 c.5125 G > A p.D1709N | WT | WT |
T10 | F | 35 | 3.5 | NIFTP | / | DICER1 c.5113 G > A p.E1705K | WT | WT |
T11 | F | 18 | 1.6 | EAIFTC | pT1bNxMx | DICER1 c.5125 G > A p.E1709N | WT | WT |
T12 | M | 77 | 16 | DHGTC | pT3aNxM1 | DICER1 c.5438 A > G p.E1813G | WT | WT |
T13 | F | 33 | 1.2 | MIFTC | pT1bNxMx | DICER1 c.5428 G > T p.D1810Y | WT | WT |
T14 | F | 47 | 1.6 | MIFTC | pT1bNxMx | DICER1 c.5437 G > C p.E1813Q | WT | WT |
T15 | F | 54 | 3 | EAIFTC | pT2N0Mx | DICER1 c.5429 A > T p.D1810V | WT | WT |
T16 | F | 37 | 3.9 | AIEFVPTC | pT2N0Mx | DICER1 c.5113 G > A p.E1705K | WT | WT |
T17 | F | 54 | 5 | MIEFVPTC | pT3aNxMx | DGCR8 c.1552 G > A p.E518K | NRAS c.182 A > C p.Q61R | WT |
T18 | M | 35 | 3.4 | FTA | / | DICER1 c.5437 G > A p.E1813Q | WT | WT |
Case ID . | Sex . | Age at surgery, y . | Nodule size, cm . | Tumor type . | pTNM . | Gene mutation . | RAS . | PAX8::PPARG . |
---|---|---|---|---|---|---|---|---|
T1 | F | 24 | 3.5 | MIFTC | pT2Nx | DICER1 c.5113 G > A p.E1705K | WT | WT |
T2 | F | 29 | 2.4 | FTA | NA | DICER1 c.5429 A > T p.D1810V | WT | WT |
T3 | F | 47 | 3.5 | MIFTC | pT2Nx | DICER1 c.5429 A > T p.D1810V | WT | WT |
T4 | F | 10 | 4.7 | EAIFTC | pT3aNx | DICER1 c.5437 G > C p.E1813Q | WT | WT |
T5 | F | 17 | 5 | EAIFTC | pT3aNx | DICER1 c.5437 G > C p.E1813Q | WT | WT |
T6 | M | 66 | 8 | WIFTC | pT3aNx | DGCR8 c.1552 G > A p.E518K | WT | WT |
T7 | F | 74 | NA | WIFTC | NA | DGCR8 c.1552 G > A p.E518K | WT | WT |
T8 | F | 31 | 5 | WIFTC | pT3 | DGCR8 c.1552 G > A p.E518K | HRAS c.182 A > G p.Q61R | WT |
T9 | F | 50 | 1.5 | NIFTP | / | DICER1 c.5125 G > A p.D1709N | WT | WT |
T10 | F | 35 | 3.5 | NIFTP | / | DICER1 c.5113 G > A p.E1705K | WT | WT |
T11 | F | 18 | 1.6 | EAIFTC | pT1bNxMx | DICER1 c.5125 G > A p.E1709N | WT | WT |
T12 | M | 77 | 16 | DHGTC | pT3aNxM1 | DICER1 c.5438 A > G p.E1813G | WT | WT |
T13 | F | 33 | 1.2 | MIFTC | pT1bNxMx | DICER1 c.5428 G > T p.D1810Y | WT | WT |
T14 | F | 47 | 1.6 | MIFTC | pT1bNxMx | DICER1 c.5437 G > C p.E1813Q | WT | WT |
T15 | F | 54 | 3 | EAIFTC | pT2N0Mx | DICER1 c.5429 A > T p.D1810V | WT | WT |
T16 | F | 37 | 3.9 | AIEFVPTC | pT2N0Mx | DICER1 c.5113 G > A p.E1705K | WT | WT |
T17 | F | 54 | 5 | MIEFVPTC | pT3aNxMx | DGCR8 c.1552 G > A p.E518K | NRAS c.182 A > C p.Q61R | WT |
T18 | M | 35 | 3.4 | FTA | / | DICER1 c.5437 G > A p.E1813Q | WT | WT |
Abbreviations: AEIFVPTC, angioinvasive encapsulated follicular variant papillary thyroid carcinoma; AIFTC, angioinvasive follicular thyroid carcinoma; DHGTC, differentiated high-grade thyroid carcinoma; EAIFVPTC, encapsulated angioinvasive follicular variant papillary thyroid carcinoma; MIEFVPTC, minimally invasive encapsulated follicular variant papillary thyroid carcinoma; MIFTC, minimally invasive follicular thyroid carcinoma; NA, not available; WIFTC, widely invasive follicular thyroid carcinoma; WT, wild-type.
DICER1 Mutated Thyroid Lesions Lack Mutations in Established Thyroid Cancer Drivers, Whereas DGCR8 Mutations May Coexist With RAS Aberrations
All the sequencing quality parameters are listed in Supplementary Table S2 (24).
The mutational landscape of all DICER1 and DGCR8 mutated found in the Karolinska cohort (T1-T8) was performed using whole-exome sequencing to identify additional driver mutations and verify the somatic origin of the alterations whenever possible.
First, to determine whether DICER1 and DGCR8 mutations were truly somatic or germline, the paired normal tissues of 5 cases were also submitted for analysis, the results of which are consistent with a somatic origin of the mutations for the informative cases.
All positive cases yielded the same DICER1 and DGCR8 missense mutation variants detected by Sanger sequencing. All 5 DICER1 mutated cases (T1-T5) also exhibited a second, non-hotspot DICER1 variant (truncating or splicing) in addition to the missense known variant.
Next, we mainly focused on the genetic mutations of established thyroid cancer driver genes or thyroid cancer progression. None of the 8 tumors showed alterations in known hotspots of thyroid-related genes such as BRAF, EIF1AX, PIK3CA, PTEN, TP53, CDKNA2, and RBM10 genes (Supplementary Table S2) (24). An HRAS Q61R mutation was confirmed in the case harboring the DGCR8 E518K mutation (T8). Moreover, no additional mutations were found in the other genes involved in the miRNA processing machinery, such as TARBP2, DROSHA, XPO5, or AGO2 (Fig. 3).

Oncoprint plot showing the molecular landscape obtained by whole-exome sequencing analysis of 5 DICER1 and 3 DGCR8 mutated cases found in the Karolinska cohort highlighting well-known thyroid-related genes and other genes involved in the miRNA biogenesis machinery. Besides a single HRAS mutation found in T8, all other samples were wild-type for additional thyroid-related genes as well as additional genes involved in the miRNA machinery.
To further investigate the real impact of DICER1 and DGCR8 mutations on these tumors, all positive samples from both institutions were also interrogated for PAX8::PPARG fusions using RT-PCR analysis; none harbored this alteration (Table 2).
Impact of DICER1 and DGCR8 Mutations on Global miRNA Expression
We next sought to investigate whether DICER1 and DGCR8 mutations might have an influence on miRNA biogenesis and expression. We first used a principal component analysis (PCA) to carry out an unsupervised investigation of the relationship between tumor genotype and global miRNA expression. As seen in Fig. 4A, PCA revealed that all the DICER1/DGCR8 positive cases clustered together, showing a common and unique miRNA profile driven by mutations and independent of histology. One widely invasive FTC wild-type for DICER1 and DGCR8 hotspot mutations clearly clustered together with the mutated samples on both PCA plot and miRNA heatmap (Fig. 4A and 4B, respectively). This sample was submitted for further molecular analysis, using a next-generation sequencing panel used in clinical routine that covers the whole DICER1 gene (22). No other mutations were found in DICER1 (data not shown). Furthermore, we interrogated the same case for the most common hotspot mutations in DROSHA gene, but no alterations were found. The clustering of this single sample thus remains elusive but may involve epigenetic dysregulation of miRNA-related genes, previously shown for FTCs (15). Moreover, a second DICER1/DGCR8 wild-type case appears to cluster with the mutated group in Fig. 4B, but this was not clearly reflected in the PCA plot. Given the ambiguity in its association with the mutated cluster in terms of miRNA dysregulations, we opted not to screen this tumor for additional miRNA-regulating gene mutations.
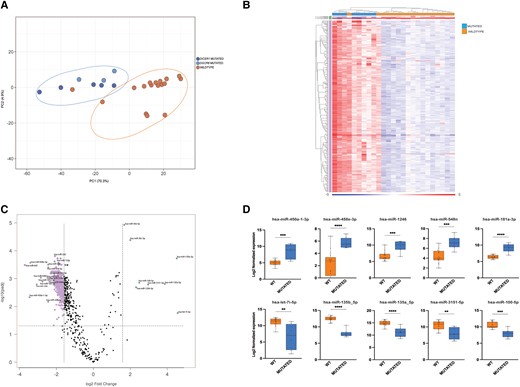
miRNA dysregulation in thyroid nodules harboring somatic hotspot mutations in DICER1 and DGCR8 genes. (A) Principal component analysis (PCA) based on DICER1 (n = 5) and DGCR8 (n = 3) mutated cases found in the Karolinska cohort and 17 wild-type cases (matched for age, sex, and diagnoses) (B) Heatmap of the 427 differentially expressed miRNAs between wild-type and mutated cases. All DICER1/DGCR8 mutated cases clustered together in a separate branch (left part of the heatmap) (C) Volcano plot showing the most dysregulated miRNAs between mutated and wild-type cases. (D) Top 5 up- and top 5 down-regulated miRNAs in mutated compared with wild-type cases. The first row shows 5 miRNAs up-regulated in mutated cases compared with wild-type; the second row shows 5 miRNAs down-regulated in mutated compared with wild-type.
Next, a differential miRNA expression analysis was performed to explore differences between DICER1/DGCR8 mutated and wild-type cases. Setting a cutoff FDR of 0.05 with a fold change ≥3, a total of 427 DEMs were found between wild-type and mutated cases, as shown in Fig. 4B and 4C. Among these, we searched for individual miRNAs that had the highest and lowest levels of expression in wild-type versus mutated. The top 5 upregulated and downregulated miRNAs were selected and are shown in Fig. 4D. Five miRNAs (miR-450a-1-3p, miR-548e-3p, miR-1246, miR-548n, and miR-181a-3p) were overexpressed in mutated cases, whereas 5 additional miRNAs (let-7i-5p, miR-135b-5p, miR135a-5p, miR3151-5p, and miR-100-5p) were most consistently downregulated in mutated cases. Most have been previously reported to be deregulated in thyroid cancer (18, 28, 29). Using the same cutoff, no significant differences were found when we compared DICER1 with DGCR8 mutated cases only.
Predictably, all the DICER1 mutated cases displayed a reduced expression of 5p miRNAs and an increased level of 3p miRNAs compared with wild-type cases, supporting the hypothesis that these mutations determine an evident imbalance 5p:3p miRNAs (Fig. 5B-C). These results are clearly consistent with other studies (18, 23).
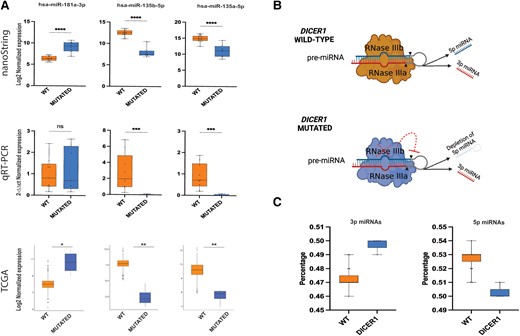
Extended miRNA expression validation in thyroid nodules with DICER1/DGCR8 mutations. (A) Top: expression levels of 3 selected miRNAs using global miRNA profiling via nanoString; middle: relative expression levels of the same 3 selected miRNAs validated experimentally by quantitative RT-PCR; bottom: expression levels of the same 3 selected miRNAs extracted from TCGA data. (B) Disabling mutations in RNase III domains of DICER1 lead to 5p miRNA depletion. Schematic representation of the mechanism by which DICER1 cleaves pre-miRNA in wild-type and mutated conditions; hotspot mutations in the RNase IIIb domain lead to a significant reduction of 5p strand miRNAs in DICER1 mutants compared with wild-type cases. The figure was created with Biorender.com. (C) Remarkable difference in 5p:3p strand ratio between DICER1 mutants and wild-type cases was observed in the Karolinska cohort.
Furthermore, the DEMs in wild-type vs mutated cases have been used for pathway enrichment analysis on the DIANA-miRPath v3.0 tool, and more than 50 pathways resulted significantly enriched. In Table 3, the top 20 pathways enriched, ranked by ascending P values, are detailed.
List of the top 20 significantly enriched pathways controlled by the miRNAs deregulated in DICER1/DGCR8 mutant vs wild-type
. | Pathways (KEGG) . | Adjusted P . | Number of target genes . | Number of miRNAs . |
---|---|---|---|---|
1 | Protein processing in endoplasmic reticulum | 8.66e-14 | 125 | 53 |
2 | Proteoglycans in cancer | 1.13e-12 | 134 | 54 |
3 | Viral carcinogenesis | 7.29e-11 | 126 | 58 |
4 | Adherens junction | 4.06e-09 | 58 | 48 |
5 | Hippo signaling pathway | 4.06e-09 | 98 | 55 |
6 | Prostate cancer | 3.30e-07 | 68 | 49 |
7 | Pancreatic cancer | 4.23e-07 | 49 | 48 |
8 | Colorectal cancer | 8.67e-07 | 49 | 47 |
9 | Bacterial invasion of epithelial cells | 2.23e-06 | 57 | 50 |
10 | Ubiquitin mediated proteolysis | 2.23e-06 | 95 | 52 |
11 | Cell cycle | 2.67e-06 | 88 | 56 |
12 | Central carbon metabolism in cancer | 3.83e-06 | 50 | 43 |
13 | Renal cell carcinoma | 5.61e-06 | 49 | 42 |
14 | Lysine degradation | 6.24e-06 | 32 | 46 |
15 | Oocyte meiosis | 6.68e-06 | 76 | 50 |
16 | TGF-β signaling pathway | 9.58e-06 | 53 | 48 |
17 | Endocytosis | 9.58e-06 | 134 | 50 |
18 | Small cell lung cancer | 1.23e-05 | 63 | 47 |
19 | p53 signaling pathway | 1.23e-05 | 52 | 52 |
20 | HIF-1 signaling pathway | 1.68e-05 | 74 | 47 |
. | Pathways (KEGG) . | Adjusted P . | Number of target genes . | Number of miRNAs . |
---|---|---|---|---|
1 | Protein processing in endoplasmic reticulum | 8.66e-14 | 125 | 53 |
2 | Proteoglycans in cancer | 1.13e-12 | 134 | 54 |
3 | Viral carcinogenesis | 7.29e-11 | 126 | 58 |
4 | Adherens junction | 4.06e-09 | 58 | 48 |
5 | Hippo signaling pathway | 4.06e-09 | 98 | 55 |
6 | Prostate cancer | 3.30e-07 | 68 | 49 |
7 | Pancreatic cancer | 4.23e-07 | 49 | 48 |
8 | Colorectal cancer | 8.67e-07 | 49 | 47 |
9 | Bacterial invasion of epithelial cells | 2.23e-06 | 57 | 50 |
10 | Ubiquitin mediated proteolysis | 2.23e-06 | 95 | 52 |
11 | Cell cycle | 2.67e-06 | 88 | 56 |
12 | Central carbon metabolism in cancer | 3.83e-06 | 50 | 43 |
13 | Renal cell carcinoma | 5.61e-06 | 49 | 42 |
14 | Lysine degradation | 6.24e-06 | 32 | 46 |
15 | Oocyte meiosis | 6.68e-06 | 76 | 50 |
16 | TGF-β signaling pathway | 9.58e-06 | 53 | 48 |
17 | Endocytosis | 9.58e-06 | 134 | 50 |
18 | Small cell lung cancer | 1.23e-05 | 63 | 47 |
19 | p53 signaling pathway | 1.23e-05 | 52 | 52 |
20 | HIF-1 signaling pathway | 1.68e-05 | 74 | 47 |
Abbreviations: KEGG, Kyoto Encyclopedia of Genes and Genomes; miRNA, micro-RNA.
List of the top 20 significantly enriched pathways controlled by the miRNAs deregulated in DICER1/DGCR8 mutant vs wild-type
. | Pathways (KEGG) . | Adjusted P . | Number of target genes . | Number of miRNAs . |
---|---|---|---|---|
1 | Protein processing in endoplasmic reticulum | 8.66e-14 | 125 | 53 |
2 | Proteoglycans in cancer | 1.13e-12 | 134 | 54 |
3 | Viral carcinogenesis | 7.29e-11 | 126 | 58 |
4 | Adherens junction | 4.06e-09 | 58 | 48 |
5 | Hippo signaling pathway | 4.06e-09 | 98 | 55 |
6 | Prostate cancer | 3.30e-07 | 68 | 49 |
7 | Pancreatic cancer | 4.23e-07 | 49 | 48 |
8 | Colorectal cancer | 8.67e-07 | 49 | 47 |
9 | Bacterial invasion of epithelial cells | 2.23e-06 | 57 | 50 |
10 | Ubiquitin mediated proteolysis | 2.23e-06 | 95 | 52 |
11 | Cell cycle | 2.67e-06 | 88 | 56 |
12 | Central carbon metabolism in cancer | 3.83e-06 | 50 | 43 |
13 | Renal cell carcinoma | 5.61e-06 | 49 | 42 |
14 | Lysine degradation | 6.24e-06 | 32 | 46 |
15 | Oocyte meiosis | 6.68e-06 | 76 | 50 |
16 | TGF-β signaling pathway | 9.58e-06 | 53 | 48 |
17 | Endocytosis | 9.58e-06 | 134 | 50 |
18 | Small cell lung cancer | 1.23e-05 | 63 | 47 |
19 | p53 signaling pathway | 1.23e-05 | 52 | 52 |
20 | HIF-1 signaling pathway | 1.68e-05 | 74 | 47 |
. | Pathways (KEGG) . | Adjusted P . | Number of target genes . | Number of miRNAs . |
---|---|---|---|---|
1 | Protein processing in endoplasmic reticulum | 8.66e-14 | 125 | 53 |
2 | Proteoglycans in cancer | 1.13e-12 | 134 | 54 |
3 | Viral carcinogenesis | 7.29e-11 | 126 | 58 |
4 | Adherens junction | 4.06e-09 | 58 | 48 |
5 | Hippo signaling pathway | 4.06e-09 | 98 | 55 |
6 | Prostate cancer | 3.30e-07 | 68 | 49 |
7 | Pancreatic cancer | 4.23e-07 | 49 | 48 |
8 | Colorectal cancer | 8.67e-07 | 49 | 47 |
9 | Bacterial invasion of epithelial cells | 2.23e-06 | 57 | 50 |
10 | Ubiquitin mediated proteolysis | 2.23e-06 | 95 | 52 |
11 | Cell cycle | 2.67e-06 | 88 | 56 |
12 | Central carbon metabolism in cancer | 3.83e-06 | 50 | 43 |
13 | Renal cell carcinoma | 5.61e-06 | 49 | 42 |
14 | Lysine degradation | 6.24e-06 | 32 | 46 |
15 | Oocyte meiosis | 6.68e-06 | 76 | 50 |
16 | TGF-β signaling pathway | 9.58e-06 | 53 | 48 |
17 | Endocytosis | 9.58e-06 | 134 | 50 |
18 | Small cell lung cancer | 1.23e-05 | 63 | 47 |
19 | p53 signaling pathway | 1.23e-05 | 52 | 52 |
20 | HIF-1 signaling pathway | 1.68e-05 | 74 | 47 |
Abbreviations: KEGG, Kyoto Encyclopedia of Genes and Genomes; miRNA, micro-RNA.
Data Validation Using the TCGA Database
To further validate our results, all DICER1 and DGCR8 mutant cases with clinical parameters were retrieved from the TCGA database of PTCs (Supplementary Table S3) (24). First, a clustering analysis was performed to detect differences in terms of miRNA expression profiles. All mutated cases clustered together on a separate branch. One exception was the only case with a DICER1 mutation that was not located in the RNase IIIb domain (Supplementary Fig. S1) (24).
As expected, the cumulative proportion of 3p strand miRNAs was higher in the 2 tumors harboring DICER1 RNase IIIb mutations (Supplementary Fig. S2) (24); hence, only these 2 cases were considered as DICER1 mutated in the following analyses. Cases harboring DGCR8 E518K showed no consistent loss of 5p miRNAs.
Differential expression analysis highlighted 106 and 72 DEMs in DICER1 and DGCR8 mutant tumors, respectively. Thirty-seven miRNAs were commonly deregulated (Supplementary Fig. S3A) (24). A remarkable effect of miRNA strand was observed in DICER1 mutant only, whereas 3p miRNAs were upregulated; the expression of 5p miRNA was suppressed (Supplementary Fig. S3B and 3C) (24). Looking at DEMs in DGCR8-mutant tumors, 10 miRNA pairs (ie, 3p and 5p miRNAs originating from the same precursor) were deregulated in the same direction (ie, either both up- or downregulated). In detail, 4 miRNA pairs were suppressed (ie, miR-141, miR-29c, miR-708, miR-30d), whereas the expression levels of 6 miRNA pairs were enhanced (ie, miR-145, miR-143, miR-378a, miR-126, miR-140, miR-29b-2).
Among the top 5 up- and downregulated DEMs of the nanoString analysis performed in our institutional cases, 3 miRNAs (miR-135b-5p, miR135a-5p, and miR-181a-3p) were consistently deregulated also in the TCGA series. These miRNAs were further investigated by quantitative RT-PCR in 5 DICER1-positive cases and 10 wild-type cases with similar clinicopathologic features as the mutated cases. A reliable concordance between results using different techniques was found (Fig. 5A). Only the miRNA miR-181a-3p did not reach statistical significance using this method.
Discussion
With the recent identification of recurrent DICER1 and DGCR8 hotspot mutations in subsets of WDTCs (16, 20), the interest in genes involved in miRNA processing machinery has grown among researchers. However, the question arises whether these alterations are independent driver gene alterations or late events unrelated to tumorigenesis per se. Our integrated analysis reports the prevalence and spectrum of DICER1 and DGCR8 mutations in an unselected, bi-institutional cohort of FTs and shows how these alterations clearly impact the global miRNA expression status. Furthermore, we strengthened the robustness of our data by comparing our findings with data from the TCGA cases in terms of clinical parameters and influence on miRNA expression profile.
In the past few years, several studies reported DICER1 biallelic inactivation at the somatic level, predominantly in WDTC, lacking genetic alterations of established thyroid cancer driver genes, strongly suggesting that DICER1 mutations may trigger the onset of specific subgroups of thyroid tumors (20). Somatic mutations in DICER1 RNase IIIb hotspots have been shown in a wide variety of RAS-like lesions, ranging from benign FTA (23), NIFTP (30), to malignant FTC (22) and FVPTCs (12), highlighting an association to early- and adult-onset thyroid lesions with a favorable prognosis (31, 32). By contrast, Chernock and collaborators recently demonstrated how childhood- and adolescent-onset PDTC are genetically distinct from adult-onset PDTC in that they were strongly associated with DICER1 mutations, suggesting that the clinically aggressive behavior of PDTC contrasts sharply with the indolent nature of most thyroid lesions driven by DICER1 mutations reported to date (21).
In the present study, 3.2% of the cohort cases harbored DICER1 hotspot mutations, occurring in FTA (2/127), NIFTP (2/48), EFVPTC (1/80), FTC (8/126), and DHGTC (1/3). Of these, 5 cases were found to harbor a second, non-hotspot DICER1 variant in addition to the primary missense mutation when analyzed via WES. Among the 14 mutated cases, the p.E1813Q missense mutation occurs in 2 pediatric patients (aged 10 and 17 years, respectively), whereas the remaining patients ranged in age from 24 to 77 years. Interestingly, a recent study reported that the frequency of DICER1 hotspot mutations in thyroid nodules with indeterminate cytology was 1.4%, occurring in a proportion of adult patients and appearing mutually exclusive with alterations in other thyroid cancer-related genes (20). However, it is worth mentioning that these data, including the current study, include hotspot alterations only, and therefore the true prevalence of DICER1 mutated cases (including non-RNase IIIb region mutations) remains to be established.
In our cohort, patients with DICER1 mutated lesions were younger than DICER1 wild-type cases, corroborating previous observations (13, 14, 22). Although the DICER1-mutated patient group as a whole skewed toward a younger demographic, it is crucial to note that all mutations identified in this study that could be tested in constitutional DNA were of somatic origin. However, T4 and T5 originate from pediatric patients (10-year-old and 17-year-old females, respectively) with DICER1-mutated encapsulated angioinvasive FTC. For these cases, constitutional tissue was unavailable for sequencing, leaving the germline DICER1 status unknown. Despite this, we chose to include them in the whole-exome sequencing analysis because potential biallelic inactivation of DICER1 would support its role as a driving force behind tumor development in these patients. Both cases exhibit no recurrences or indications of other tumor manifestations and exhibit negative family history concerning lesions related to DICER1 syndrome.
A pertinent question emerges: Should every patient with DICER1 mutated thyroid nodules, identified through molecular workup, undergo clinical genetics evaluation to rule out DICER1 syndrome? At present, neither of our institutions authoring this study perform clinical routine reflex sequencing of DICER1 and DGCR8. Our data, when considered alongside previous observations, suggest that the majority of DICER1 mutations in thyroid tumors are indeed somatic, even in cases of biallelic mutations. However, it is imperative to factor in elements such as family medical history, the presence of other tumor manifestations, and the overall disease presentation when making this determination. Certain studies have identified subsets of patients with germline DICER1 involvement, even in cases lacking a positive family history and other manifestations beyond thyroid follicular nodular disease (33). Consequently, considering the potential clinical benefit, it might be worthwhile to screen patients with identified DICER1 variants in tumor tissue for constitutional involvement. Given the infrequency of these mutations in routine clinical practice, the overall cost associated with clinical genetics counseling and germline DNA sequencing is likely to be minimal in comparison to the potential benefits of identifying a patient with a syndromic predisposition. Therefore, the threshold for initiating germline DNA investigations should be set relatively low. Nevertheless, our study is underpowered in terms of cases analyzed for germline involvement, which prevents us from providing comprehensive advice on genetic counseling. Future studies on this topic could eventually provide clarity regarding the overall frequency of these genetic alterations in thyroid nodules and might be helpful in constructing guidelines for clinical practice. It must also be stressed that we focused on hotspot alterations only, meaning that the entire genes were not fully interrogated in this study.
For DGCR8 mutations, the number of published cases is limited. Although there may be an association with aggressive biological behavior, certain patients with this mutation occurring in the germline develop thyroid follicular nodular disease rather than thyroid cancer (4). Consequently, the established value of identifying this mutation in somatic screening remains uncertain.
Regarding the DGCR8 gene, the specific E518K variant causes multinodular goiter with schwannomatosis (4), and somatic loss of heterozygosity has been shown as a second event in tumor tissues from these patients. The same combination has also been shown to act as driver event in Wilms tumor, thus strongly advocating for a tumor suppressor function (34). Recently, our group reported somatic E518K mutations with concurrent loss of heterozygosity in FTCs, showing how this mutation potentially influences the aggressive behavior of these tumors (16). Another study reported the same mutation in a single PDTC (17). We identified 4 cases harboring the E518K mutation (including 1 previously published case), of which 3 were detected in aggressive FTCs and 1 in an IEFVPCT, suggesting that E518K variant could be specific for high-risk malignancies. However, because of the limited number of published cases with this alteration, further investigations are needed before a true causality between this genotype and clinical attributes can be verified. This is particularly important because subsets of cases with germline DGRC8 mutations only seem to develop thyroid follicular nodular disease.
Furthermore, we provided evidence of how miRNA expression profiles vary in DICER1/DGCR8 mutated compared with wild-type tumors. The impact of these mutations at the miRNA expression level is explained by a common and unique molecular signature on mutated cases, pointing toward the possible variation in miRNA signatures depending on the mutational status of these 2 genes independently of histology. However, the mechanistic reason for the altered miRNA expression may vary between DICER1 and DGCR8 mutants, as 3p:5p miRNA imbalance is typical of DICER1 mutations, whereas dysregulated processing of specific precursors is the hallmark of DCGR8 mutations. Among the DEMs, we searched for individual miRNAs that had the highest and lowest levels of expression in wild-type vs mutated. We particularly focused on those that were already described to have a key role in thyroid lesions. In particular, miR-181a-3p was previously described as deregulated in NIFTP and IFVPTC and associated with adverse outcomes in patients with EFVPTC (28, 29). miR-135a-5p and miR-135b-5p were recently described as downregulated in DICER1 mutated pediatric thyroid cancer cases (18). These 2 miRNAs have also been associated with tumor suppressor function in PTC (35). Finally, the present investigation supports the currently held dogma: DICER1 mutations strongly impair miRNA biogenesis. Predictably, the imbalance 5p:3p in all those cases carrying DICER1 RNase IIIb hotspot mutations was found, in line with previous studies (18, 23, 36).
The findings of miRNA dysregulation in DICER1 and DGCR8 mutants were consistently supported by the results obtained by analyzing TCGA data from PTCs, suggesting that the mutational effect on miRNA profiles is apparent irrespective of tumor type. This strongly supports our hypothesis that the mutational status of DICER1 and DGCR8 rather than tumor histotype determines the global change in miRNA expression. Moreover, a sharp detection of imbalance 5p:3p miRNAs between DICER1 mutated cases compared with wild-type cases was demonstrated, suggesting a similar modus operandi also for PTCs.
In conclusion, we provide an overall mutational frequency of DICER1 and DGCR8 mutations in a bi-institutional cohort of thyroid nodules, showing that these mutations are rare events in FTs. Furthermore, we corroborated that DICER1 mutations may have independent abilities to trigger the onset of specific subgroups of thyroid lesions, whereas DGCR8 mutations may coexist with bona fide thyroid drivers but may stimulate progression because this genetic event was congregated in tumors with aggressive behavior and poor outcome. Mutations in these 2 genes have a clear impact on global miRNA expression, strongly implying that the mutations cause dysregulation of the miRNA machinery. Future investigations of dysregulated miRNAs in DICER1 and DGCR8 mutated tumors may provide important clues for thyroid tumor initiation and progression.
Acknowledgments
We thank the KI Gene core facility at Karolinska Institutet for aiding with the nanoString analysis. The authors also acknowledge the Novogene Company for the whole-exome sequencing analysis.
Funding
This work was supported by The Swedish Cancer Society (Cancerfonden CAN 2020/426) and the Cancer Research Funds of Radiumhemmet (Radiumhemmets Forskningsfonder 224053).
Author Contributions
V.C. contributed to conceptualization, methodology, investigation, data curation, formal analysis, visualization, writing of original draft, and project administration. A.M.P. performed TCGA data analysis. E.M., P.V., and J.O.P. were involved in the main investigation and provided experimental expertise. J.Z. and F.B. collected clinical data and played a crucial role in the revision of the manuscript. C.C.J. conceived and supervised the project, contributed to writing, reviewing, and editing the manuscript, project administration, and funding acquisition.
Disclosures
The authors have nothing to disclose and have no conflicts of interest to declare.
Data Availability
Some or all datasets generated during and/or analyzed during the current study are not publicly available but are available from the corresponding author on reasonable request.
Ethical Approval
This study was approved by the Swedish Ethical Review Authority (EPN 2015_959-31) and by the Institutional Ethical Committee (CEAVNO, protocol number 9989/2019)
References
Abbreviations
- DEM
differentially expressed micro-RNA
- DHGTC
differentiated high-grade thyroid carcinoma
- FDR
false discovery rate
- FND
follicular nodular disease
- FTA
follicular thyroid adenoma
- FTC
follicular thyroid carcinoma
- IEFVPTC
invasive encapsulated follicular variant papillary thyroid carcinoma
- miRNA
micro-RNA
- NIFTP
noninvasive follicular thyroid neoplasm with papillary-like nuclear features
- PCA
principal component analysis
- PDTC
poorly differentiated thyroid carcinoma
- TCGA
The Cancer Genome Atlas
- WDTC
well-differentiated thyroid carcinoma