-
PDF
- Split View
-
Views
-
Cite
Cite
Katharina Wang, Laura Schober, Alessa Fischer, Nicole Bechmann, Julian Maurer, Lea Peischer, Astrid Reul, Constanze Hantel, Martin Reincke, Felix Beuschlein, Mercedes Robledo, Hermine Mohr, Natalia S Pellegata, Katharina Schilbach, Thomas Knösel, Matthias Ilmer, Martin Angele, Matthias Kroiss, Umberto Maccio, Martina Broglie-Däppen, Diana Vetter, Kuno Lehmann, Karel Pacak, Ashley B Grossman, Christoph J Auernhammer, Kathrin Zitzmann, Svenja Nölting, Opposing Effects of Cannabidiol in Patient-derived Neuroendocrine Tumor, Pheochromocytoma/Paraganglioma Primary Cultures, The Journal of Clinical Endocrinology & Metabolism, Volume 109, Issue 11, November 2024, Pages 2892–2904, https://doi.org/10.1210/clinem/dgae241
- Share Icon Share
Abstract
Treatment options for advanced neuroendocrine tumors (NETs), pheochromocytomas and paragangliomas (PPGLs) are still limited. In recent years, antitumor effects of cannabinoids have been reported; however, there are only very limited data available in NETs or PPGLs.
Investigation of the effects of cannabidiol (CBD) on patient-derived human NET/PPGL primary cultures and on NET/PPGL cell lines.
We established primary cultures derived from 46 different patients with PPGLs (n = 35) or NETs (n = 11) who underwent tumor resection at 2 centers. Treatment of patient primary cultures with clinically relevant doses (5 µM) and slightly higher doses (10 µM) of CBD was performed.
We found opposing effects of 5 µM CBD: significant antitumor effects in 5/35 (14%) and significant tumor-promoting effects in 6/35 (17%) of PPGL primary cultures. In terms of antitumor effects, cluster 2-related PPGLs showed significantly stronger responsivity to CBD compared to cluster 1-related PPGLs (P = .042). Of the cluster 2-related tumors, NF1 PPGLs showed the strongest responsivity (4/5 PPGL primary cultures with a significant decrease in cell viability were NF1-mutated). We also found opposing effects of 10 µM CBD in PPGLs and NETs: significant antitumor effects in 9/33 of PPGL (27%) and 3/11 of NET (27%) primary cultures and significant tumor-promoting effects in 6/33 of PPGL (18%) and 2/11 of NET (18%) primary cultures.
We suggest a potential novel treatment option for some NETs/PPGLs but also provide evidence for caution when applying cannabinoids as supportive therapy for pain or appetite management to cancer patients and possibly as health supplements.
Neuroendocrine neoplasms are a heterogeneous group of tumors that include neuroendocrine tumors (NETs) of the gastroenteropancreatic system (GEP-NETs) as well as tumors of the adrenal medulla [pheochromocytomas (PCCs)] or the sympathetic or parasympathetic paraganglia [paragangliomas (PGLs)], collectively referred to as PPGLs.
GEP-NETs are neoplasms with an increasing incidence of 7 per 100 000 population per year (1). Around 40% to 45% of GEP-NETs have already spread to other organs at the time of initial diagnosis, requiring systemic treatment modalities (2). The 5-year survival rates of patients with metastatic NETs are only 38% to 50% for pancreatic NETs and 43% to 69% for ileal NETs (1, 3, 4), and surgery is considered the only curative treatment option. The only established systemic therapy options for GEP-NETs include “cold” somatostatin analogs (for tumors with low proliferative potential, Ki-67 ≤ 10%) (5, 6), somatostatin receptor 2-guided radionuclide therapy (7), targeted therapies with the mTORC1-inhibitor everolimus (8, 9), the tyrosine kinase inhibitor sunitinib (10), or systemic chemotherapy protocols such as temozolomide/capecitabine or streptozotocin/5-fluorouracil (11).
In contrast to GEP-NETs, PPGLs are rare, with a combined annual incidence rate of 0.04 to 0.21 per 100 000 population (12). These tumors show the highest rate of heritability among all endocrine tumors. Around 70% of PPGLs can be assigned to 1 of the 3 main molecular clusters according to their somatic or germline pathogenic variants (PVs): pseudohypoxia-related cluster 1 (1A or 1B), kinase signaling-related cluster 2, and Wnt-signaling-related cluster 3 (13, 14). Cluster 1 PPGL show PVs in SDHA/B/C/D, HIF2A/EPAS1, and VHL genes, among others (13, 14), and are characterized by increased expression and stabilization of HIF-2α, which is associated with elevated disease aggressiveness (15). Cluster 2 PPGL show PVs in the NF1, RET, TMEM127, MAX, and HRAS genes, among others (13, 14). Cluster 3 PVs are associated with aggressive behavior and high metastatic risk (16, 17). Patients can receive personalized diagnostics and follow-up according to their genetic profile, since the clusters are associated with different biochemical and clinical presentations and prognostic differences (13).
Therapy, however, is not yet personalized for PPGL patients. This is problematic since each PPGL has the potential to spread to distant sites and approximately 10% to 15% of PCCs and 35% to 40% of PGLs metastasize (18-22). The median overall survival of patients with metastatic PPGLs has been reported to be 7 years (23), with a 5-year mortality rate of 37% in a large meta-analysis (24). Metastatic PPGLs are, moreover, difficult to treat, and the only Food and Drug Administration-approved therapy available, high-specific-activity [131I]-MIBG therapy, was withdrawn in the first quarter of 2024 due to high manufacturing costs.
There are, therefore, only limited treatment options available for both metastatic NETs and PPGLs, such that there is an urgent need for novel and innovative therapeutic approaches.
Cannabinoids are lipophilic substances that act as ligands for the cannabinoid receptors CB1 and/or CB2, which are part of the endocannabinoid system (ECS). The ECS includes endocannabinoids, as well as the enzymes responsible for their synthesis, transport, and degradation (25, 26). In addition to endocannabinoids, a multitude of plant-based and synthetically obtained compounds can interact with the ECS. Cannabis is the most prominent representative of cannabinoid-producing plants. More than 100 phytocannabinoids have been isolated from cannabis, including cannabidiol (CBD) and (−)-trans-Δ9-tetrahydrocannabinol (Δ9-THC), the latter being the primary psychoactive component. CBD and Δ9-THC constitute the active substances in nabiximols (Sativex®), which is approved for the treatment of multiple sclerosis-induced spasticity in over 25 countries (27). CBD as a single substance (Epidiolex®) is currently Food and Drug Administration and European Medicines Agency approved for the treatment of seizures in the rare cases of Lennox-Gastaut or Dravet syndromes (27).
In recent years, the direct antitumor effects of cannabinoids have been supported by numerous preclinical studies on several types of cancer, especially on cancers of ectodermal origin such as glioma, melanoma, and Ewing's sarcoma (28-34). These effects are mediated by different molecular pathways through cannabinoid receptors (Fig. 1). Recently, for the first time, positive results of a randomized, placebo-controlled clinical phase 1b trial on the treatment of glioblastoma multiforme with a CBD/THC combination have been published (NCT 01812616): 12 patients with glioblastoma multiforme were treated with the CBD/THC combination nabiximols in addition to the established chemotherapy with temozolomide and compared to a control group (n = 9 patients) who received only temozolomide. The 1-year survival rate was significantly higher (83%) in the nabiximols group compared to the control group (44%) (P = .042), while the median survival time in the nabiximols-treated group was 662 days vs 369 days in the control group (35).
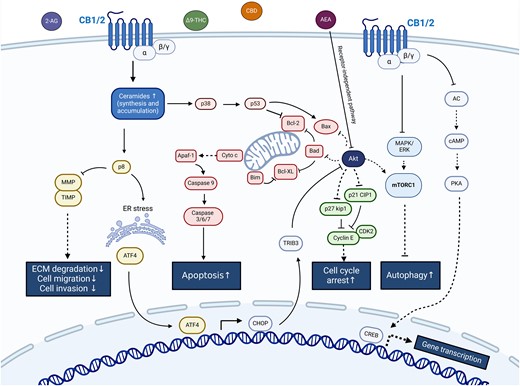
Simplified scheme of molecular anticancer pathways mediated by cannabinoids through cannabinoid receptors. ↑ activation; ⊥ inhibition. Created with Biorender.com.
Notably, due to their developmental history (arising from stem cells originating from neuroectodermal tissues), gliomas, PPGLs and NETs share a wide variety of molecular features, including germline and somatic PVs in critical tumor suppressor genes such as NF1 (36, 37). These alterations result in overlapping phenotypes associated with frequent upregulation of PI3K-Akt-mTOR- and Ras-Raf-Mek-Erk-signaling. It might therefore be expected that tumors displaying similar molecular profiles would be susceptible to similar treatment approaches.
Additionally, there is now a growing discussion concerning the legalization of recreational cannabis use in many countries involving some US states and parts of Europe, including Germany where cannabis was legalized in 2024. Moreover, nonpsychoactive cannabinoids, such as CBD oil, are being increasingly advised as health supplements. Furthermore, Δ9-THC (Dronabinol®) is officially approved for use in tumor patients with cancer pain, loss of appetite, or loss of weight (27), which are common tumor- or chemotherapy-associated symptoms and signs. This is why, in clinical practice, many PPGL or NET patients inquire about the use of cannabinoids. However, except for a limited number of in vitro studies in rat PCC cells (PC12) (38-40), the effects of cannabinoids in GEP-NETs and PPGLs have not as yet been investigated.
Therefore, we have now evaluated the therapeutic effects of the cannabinoid CBD in PPGL/NET cell lines (MPC, MTT, BON1, QGP-1, NCI-H727, GOT1 cells), MPC cell spheroids, and, most importantly, our previously established patient-derived PPGL primary culture model (36, 37) as well as in our newly established patient-derived NET primary cultures. We performed personalized drug testing in a comparatively large cohort of surgery-derived fresh primary cultures from a total of 35 PPGL patients and 11 NET patients.
Materials and Methods
NET and PPGL Cell Lines
We evaluated CBD in different NET and PPGL cell lines, including the pancreatic carcinoid cell line BON1 (Prof. R. Göke, Marburg, Germany), pancreatic islet cell line QGP-1 (JCRB Cell Banc, Osaka, Japan), human lung carcinoid cell line NCI-H727 (ATCC, Manassas, VA, USA), midgut carcinoid cell line GOT1 (41) (Prof. O. Nilsson, Sahlgrenska University Hospital Göteborg, Sweden), and murine pheochromocytoma cell lines MPC and MTT (Prof. K. Pacak, National Institutes of Health, Bethesda, MD, USA). The cell lines were cultured in DMEM/F12 (BON1 and QGP-1) or RPMI 1640 medium (NCI-H727, MPC, MTT, GOT1) supplemented with amphotericin B (0.4%), penicillin/streptomycin (1%), fetal bovine serum (10%), apotransferrin (5 mg/mL) (GOT1), and insulin (0.135 IE/mL) (GOT1).
MPC Spheroid Assay
MPC cells with stable expression of HIF-2α (MPCmCherry H2A) and the corresponding control cells without HIF-2α expression (MPCmCherry control) were used to generate 3D tumor cell spheroids as previously described (42, 43). For single treatment, spheroids were treated with 5 or 10 µM CBD or dimethyl sulfoxide (DMSO) as control at day 4 after seeding. For fractionated treatment, spheroids were treated at day 4, 8, 11, and 15 after seeding. Spheroid growth was investigated 18 days after seeding as previously described (42).
Human NET and PPGL Primary Cultures
Patients with PPGLs or NETs were included in this study based on participation in the European Network for the Study of Adrenal Tumors (ENS@T) or in the Exzellenz-Netzwerk für Neuroendokrine Tumoren und Hypophysen- und Nebennierenerkrankungen (NeoExNET), respectively, and written informed consent was obtained from all patients. The use of the PPGL primary cultures as part of ENS@T was approved by the Ethics Committee of LMU Munich (Projekt-Nr. 379-10) and by the Cantonal Ethics Committee Zurich under the reference number BASEC 2017-00771. The use of NET primary cultures for our purpose as part of NeoExNET was approved by the Ethics Committee of LMU Munich (Projekt-Nr. 152-10) and by the Cantonal Ethics Committee Zurich under the reference number BASEC 2017-00950.
We have previously established a pipeline for the isolation and processing of patient-derived PPGL primary cultures, and the methods have been described in detail (44, 45). For patient-derived NET primary cultures, we have now established a similar pipeline. In brief, fresh tumor tissue samples from patients undergoing resection of a PPGL or NET at the University Hospitals of Munich and Zurich were collected. The tumor tissues were then processed into small pieces, isolated, and suspended in culture medium (DMEM/F12 or RPMI 1640, both from Thermo Fisher Scientific, Waltham, MA, USA).
Cell Viability Assay
Primary cells or cell lines were counted, seeded into 96-well plates, and incubated for 72 hours so that adhesion was guaranteed. They were then treated with CBD for 72 hours while DMSO was used as control. Subsequently, cell viabilities were estimated by Cell Titer Blue® cell viability assay (Promega, Madison, WI, USA), as previously described (44). Each cell viability experiment was performed at least in triplicate. Additionally, the cell line results consist of 2 to 3 independently performed cell viability experiments.
Clinically Relevant Doses of CBD
We tested the naturally occurring cannabinoid CBD (Tocris Bioscience, Bristol, UK) in NET and PPGL cell lines, MPC cell spheroids, and patient primary cultures. For these trials, we used clinically relevant doses (5 µM) and doses close to clinically relevant doses (10 µM). These drug concentrations were chosen based on plasma concentrations measured in patients during therapy. For CBD, patient plasma concentrations were found to be between 0.9 µM and 2.5 µM after application of single ascending doses of CBD and up to 4.4 µM after application of multiple doses (46).
Genetic Testing
For patients with PPGLs, germline and somatic genetic testing was performed, and the variants found were classified, as previously described (45, 47-49). Germline PVs were identified in the respective centers of human genetics as part of routine clinical practice. Somatic testing was performed in 9/36 PPGLs: Sequencing of tumor DNA was carried out by the Spanish National Cancer Research Center (Madrid, Spain) by next-generation sequencing, as previously described (48). A custom-designed panel covered both known PPGL susceptibility genes and cancer-associated genes (48). Any PVs detected were then confirmed by Sanger sequencing.
Statistical Analysis
All cell viability results are displayed as the mean values ± SD of at least 3 samples. Student's t-test was used to compare the mean of each cell line or patient-derived primary culture treated with CBD with the mean of the respective control. To detect differences between clusters, comparisons between groups were performed by one-way ANOVA followed by the Bonferroni multiple comparisons test if parametric criteria were met after testing for normal distribution. In case of nonparametric data, the Kruskal–Wallis test followed by the Bonferroni correction was performed. A significance threshold of P-value < .05 was used. Statistical calculations were conducted with SPSS Version 29.0.1.1 (IBM Corp., Armonk, NY, USA, released 2022).
Results
Evaluation of CBD in PPGL and NET Cell Lines
We performed IC50 calculations for CBD in all cell lines and found IC50 concentrations between 11.7 µM and 23.2 µM after 72 hours of treatment using single ascending doses of 10 to 20 µM CBD and IC50 concentrations between 10.4 µM and 27.5 µM after 144 hours of treatment [Supplementary Table S1 (50)]. For further drug testing in cell lines and primary cultures, we therefore chose, on the one hand, a clinically relevant concentration of 5 µM CBD based on plasma concentrations measured in patients during therapy (46) and, on the other hand, slightly higher concentrations of 10 µM CBD based on the minimum IC50 concentrations identified.
First, we evaluated CBD in PPGL and NET cell lines (Fig. 2). In murine PPGL cell lines with PVs in NF1 (MTT and MPC), in a human pancreatic NET (BON1), and a human pulmonary NET (NCI-H727) cell line, cell viability was significantly suppressed by 10 µM CBD after incubation for 72 hours and/or 144 hours compared to the untreated control (Fig. 2A). However, while human midgut NET (GOT1) cells showed no significant changes in cell viability, human pancreatic NET (QGP-1) cell viability was significantly increased after CBD treatment.
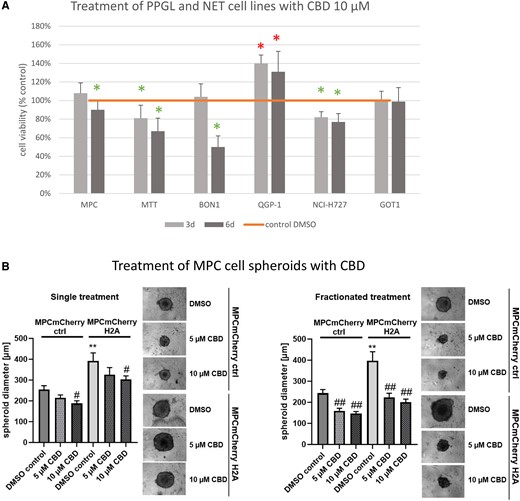
Cell viability results of different PPGL and NET cell lines and MPC cell spheroid data. (A) Cell viability of PPGL cell lines MPC, MTT, and NET cell lines BON1, QGP-1, NCI-H727, GOT1 after incubation with 10 µM CBD for 72 hours and 144 hours. (B) CBD reduced spheroid growth in a pseudohypoxic model. MPC cell spheroids generated from cells expressing HIF-2α (MPCmCherry H2A) or control cells (MPCmCherry EV) were treated with 5 µM or 10 µM CBD or DMSO as control (single treatment: day 4; fractionated treatment: day 4, 8, 11, 15). Spheroid diameter was determined 18 days after seeding. Four independent experiments (n = 12). Average ± SEM, one-way ANOVA with Bonferoni correction, vs MPCmCherry crtl, DMSO control **P < .01, vs DMSO control of the corresponding cell line #P < .05, ##P < .01.
Abbreviations: CBD, cannabidiol; DMSO, dimethyl sulphoxide; NET, neuroendocrine tumor; PPGL, pheochromocytoma and paraganglioma.
MPC Cell Spheroids (NF1 PV and HIF2α Expression)
Since the NF1 PV, which is present in the MTT/MPC cells, belongs to cluster 2, we also stably expressed HIF-2α in MPC cells, which is associated with aggressive cluster 1 disease (15). To investigate CBD under these conditions, we treated 3D tumor cell spheroids generated from MPC cells with stable expression of HIF-2α (MPCmCherry H2A) and the corresponding control cells (MPCmCherry control). Single treatment with 10 µM CBD diminished the spheroid diameter significantly, while 5 µM had no effect (Fig. 2B). In fractionated treatment, both 5 µM and 10 µM significantly reduced the diameter of the spheroids, with the spheroids expressing HIF-2α, even responding with a greater reduction in spheroid diameter than the control spheroids without HIF-2α expression.
Patient-derived NET/PPGL Primary Cultures: Patient Characteristics
Due to the cell line results showing opposing effects of CBD, with both stimulation and inhibition of cell viability depending on the cell line, we then investigated CBD in fresh, surgery-derived, patient NET and PPGL primary cultures, which we processed directly on the day of surgery. We established a total of 12 NET primary cultures from 11 NET patients and 36 PPGL primary cultures from a comparatively large cohort of 35 PPGL patients for this rare tumor entity. The PPGL and NET patient characteristics are listed in Table 1. The detailed characteristics of each patient are included in Supplementary Tables S2 and S3 (50).
Patients . | PPGL (n = 35) . | . | NET (n = 11) . |
---|---|---|---|
Primary cultures n | 36 | 12 | |
Median age in yrs. (range) | 52 (25-76) | 64 (38-81) | |
Sex | |||
Male, n | 17 | 6 | |
Female, n | 18 | 5 | |
Tumor type | Tumor type | ||
Pheochromocytoma, n (%) | 26 (72) | Pancreatic NET, n (%) | 6 (50) |
Paraganglioma, abdominal, n (%) | 4 (11) | Small intestinal NET, n (%) | 6 (50) |
Paraganglioma, head/neck, n (%) | 6 (17) | ||
Metastatic, n (%) | 2 (6) | 9 (82) | |
Germline testing, n (%) | 26 (74) | n/a | |
Somatic testing, n (%) | 9 (26) | n/a | |
Pathogenic variantsa | n/a | ||
NF1, n (%) | 5 (14) | ||
Germline NF1, n | 3 | ||
Somatic NF1, n | 2 | ||
RET, n (%) | 2 (6) | ||
MAX, n (%) | 1 (3) | ||
Somatic HRAS, n (%) | 1 (3) | ||
SDHB, n (%) | 3 (9) | ||
SDHC, n (%) | 1 (3) | ||
SDHD, n (%) | 2 (6) | ||
Germline SDHD, n | 1 | ||
Somatic SDHD, n | 1 | ||
VUS in VHL, n (%) | 1 (3) | ||
BRCA, n (%) | 1 (3) | ||
Somatic ATRX, n (%) | 1 (3) | ||
No pathogenic variants, n (%) | 3 (9) | ||
No germline, somatic unknown, n (%) | 5 (14) | ||
Unknown status, n (%) | 9 (26) | ||
Biochemical phenotype | Functionally active | ||
Adrenergic, n (%) | 15 (43) | Carcinoid syndrome, n (%) | 2 (18) |
Noradrenergic, n (%) | 14 (40) | Insulinoma, n (%) | 1 (9) |
Dopaminergic, n (%) | 1 (3) | Nonfunctional, n (%) | 8 (73) |
Silent, n (%) | 5 (14) |
Patients . | PPGL (n = 35) . | . | NET (n = 11) . |
---|---|---|---|
Primary cultures n | 36 | 12 | |
Median age in yrs. (range) | 52 (25-76) | 64 (38-81) | |
Sex | |||
Male, n | 17 | 6 | |
Female, n | 18 | 5 | |
Tumor type | Tumor type | ||
Pheochromocytoma, n (%) | 26 (72) | Pancreatic NET, n (%) | 6 (50) |
Paraganglioma, abdominal, n (%) | 4 (11) | Small intestinal NET, n (%) | 6 (50) |
Paraganglioma, head/neck, n (%) | 6 (17) | ||
Metastatic, n (%) | 2 (6) | 9 (82) | |
Germline testing, n (%) | 26 (74) | n/a | |
Somatic testing, n (%) | 9 (26) | n/a | |
Pathogenic variantsa | n/a | ||
NF1, n (%) | 5 (14) | ||
Germline NF1, n | 3 | ||
Somatic NF1, n | 2 | ||
RET, n (%) | 2 (6) | ||
MAX, n (%) | 1 (3) | ||
Somatic HRAS, n (%) | 1 (3) | ||
SDHB, n (%) | 3 (9) | ||
SDHC, n (%) | 1 (3) | ||
SDHD, n (%) | 2 (6) | ||
Germline SDHD, n | 1 | ||
Somatic SDHD, n | 1 | ||
VUS in VHL, n (%) | 1 (3) | ||
BRCA, n (%) | 1 (3) | ||
Somatic ATRX, n (%) | 1 (3) | ||
No pathogenic variants, n (%) | 3 (9) | ||
No germline, somatic unknown, n (%) | 5 (14) | ||
Unknown status, n (%) | 9 (26) | ||
Biochemical phenotype | Functionally active | ||
Adrenergic, n (%) | 15 (43) | Carcinoid syndrome, n (%) | 2 (18) |
Noradrenergic, n (%) | 14 (40) | Insulinoma, n (%) | 1 (9) |
Dopaminergic, n (%) | 1 (3) | Nonfunctional, n (%) | 8 (73) |
Silent, n (%) | 5 (14) |
Abbreviations: n/a, not available; NET, neuroendocrine tumor; PPGL, pheochromocytoma and paraganglioma; VUS, variant of unknown significance.
aGermline pathogenic variants if not further specified.
Patients . | PPGL (n = 35) . | . | NET (n = 11) . |
---|---|---|---|
Primary cultures n | 36 | 12 | |
Median age in yrs. (range) | 52 (25-76) | 64 (38-81) | |
Sex | |||
Male, n | 17 | 6 | |
Female, n | 18 | 5 | |
Tumor type | Tumor type | ||
Pheochromocytoma, n (%) | 26 (72) | Pancreatic NET, n (%) | 6 (50) |
Paraganglioma, abdominal, n (%) | 4 (11) | Small intestinal NET, n (%) | 6 (50) |
Paraganglioma, head/neck, n (%) | 6 (17) | ||
Metastatic, n (%) | 2 (6) | 9 (82) | |
Germline testing, n (%) | 26 (74) | n/a | |
Somatic testing, n (%) | 9 (26) | n/a | |
Pathogenic variantsa | n/a | ||
NF1, n (%) | 5 (14) | ||
Germline NF1, n | 3 | ||
Somatic NF1, n | 2 | ||
RET, n (%) | 2 (6) | ||
MAX, n (%) | 1 (3) | ||
Somatic HRAS, n (%) | 1 (3) | ||
SDHB, n (%) | 3 (9) | ||
SDHC, n (%) | 1 (3) | ||
SDHD, n (%) | 2 (6) | ||
Germline SDHD, n | 1 | ||
Somatic SDHD, n | 1 | ||
VUS in VHL, n (%) | 1 (3) | ||
BRCA, n (%) | 1 (3) | ||
Somatic ATRX, n (%) | 1 (3) | ||
No pathogenic variants, n (%) | 3 (9) | ||
No germline, somatic unknown, n (%) | 5 (14) | ||
Unknown status, n (%) | 9 (26) | ||
Biochemical phenotype | Functionally active | ||
Adrenergic, n (%) | 15 (43) | Carcinoid syndrome, n (%) | 2 (18) |
Noradrenergic, n (%) | 14 (40) | Insulinoma, n (%) | 1 (9) |
Dopaminergic, n (%) | 1 (3) | Nonfunctional, n (%) | 8 (73) |
Silent, n (%) | 5 (14) |
Patients . | PPGL (n = 35) . | . | NET (n = 11) . |
---|---|---|---|
Primary cultures n | 36 | 12 | |
Median age in yrs. (range) | 52 (25-76) | 64 (38-81) | |
Sex | |||
Male, n | 17 | 6 | |
Female, n | 18 | 5 | |
Tumor type | Tumor type | ||
Pheochromocytoma, n (%) | 26 (72) | Pancreatic NET, n (%) | 6 (50) |
Paraganglioma, abdominal, n (%) | 4 (11) | Small intestinal NET, n (%) | 6 (50) |
Paraganglioma, head/neck, n (%) | 6 (17) | ||
Metastatic, n (%) | 2 (6) | 9 (82) | |
Germline testing, n (%) | 26 (74) | n/a | |
Somatic testing, n (%) | 9 (26) | n/a | |
Pathogenic variantsa | n/a | ||
NF1, n (%) | 5 (14) | ||
Germline NF1, n | 3 | ||
Somatic NF1, n | 2 | ||
RET, n (%) | 2 (6) | ||
MAX, n (%) | 1 (3) | ||
Somatic HRAS, n (%) | 1 (3) | ||
SDHB, n (%) | 3 (9) | ||
SDHC, n (%) | 1 (3) | ||
SDHD, n (%) | 2 (6) | ||
Germline SDHD, n | 1 | ||
Somatic SDHD, n | 1 | ||
VUS in VHL, n (%) | 1 (3) | ||
BRCA, n (%) | 1 (3) | ||
Somatic ATRX, n (%) | 1 (3) | ||
No pathogenic variants, n (%) | 3 (9) | ||
No germline, somatic unknown, n (%) | 5 (14) | ||
Unknown status, n (%) | 9 (26) | ||
Biochemical phenotype | Functionally active | ||
Adrenergic, n (%) | 15 (43) | Carcinoid syndrome, n (%) | 2 (18) |
Noradrenergic, n (%) | 14 (40) | Insulinoma, n (%) | 1 (9) |
Dopaminergic, n (%) | 1 (3) | Nonfunctional, n (%) | 8 (73) |
Silent, n (%) | 5 (14) |
Abbreviations: n/a, not available; NET, neuroendocrine tumor; PPGL, pheochromocytoma and paraganglioma; VUS, variant of unknown significance.
aGermline pathogenic variants if not further specified.
We included 6 pancreatic NET (panNET) and 6 small intestinal NET (siNET) primary cultures from 11 different NET patients (n = 2 from the same patient, 1 siNET primary tumor and 1 metastasis). A majority of NET patients (9/11, 82%) presented with metastatic disease. In total, 3 NET patients presented with functionally active tumors, 2 of these with serotonin-producing carcinoid syndromes and 1 with an insulinoma [Table 1, Supplementary Table S2 (50)].
We included 26 PCCs, 4 abdominal PGLs, and 6 head and neck PGLs from 35 different patients (n = 2 PCCs from the same patient with bilateral PPCs). Two of the 35 patients with PPGLs presented with metastatic disease (n = 1 PCC, n = 1 head and neck PGL). The biochemical phenotypes of the PPGLs were adrenergic (15/35, 43%), noradrenergic (14/35, 40%), dopaminergic (1/35, 3%), or silent (5/35, 14%) [Table 1, Supplementary Table S3 (50)]. Germline sequencing was performed in 26 PPGL patients, and somatic sequencing was performed in 9 PPGLs. In total, we found germline PVs in 13/26 (50%) of the patients tested and somatic PVs in 5/9 (56%) of the PPGLs tested. PVs in cluster 1-associated genes (SDHB, SDHC, SDHD, variant of unknown significance in VHL) were found in 7 patients. Cluster 2-associated PVs (NF1, RET, MAX, HRAS) were found in 9 patients. One patient showed a PV in BRCA and 1 patient a PV in ATRX, which are not associated with any of the clusters. We found no germline and no somatic PVs in 3 PPGLs. Five PPGLs showed no germline PVs while somatic status was not available, and, in 9 PPGLs neither germline nor somatic status was available (unknown status). The detailed listing of the germline/somatic PVs of the PPGL patients can be found in Supplementary Table S3 (50).
Individual Drug Responsitivities of NET Primary Cultures to CBD
In NET primary cultures (n = 12, n = 2 from the same patient), clinically relevant and slightly higher doses of CBD (5 µM and 10 µM) were evaluated. Treatment with 5 µM CBD for 72 hours significantly increased cell viability compared to the untreated control in 1/12 (8%) tumor cultures and showed neutral effects in 11/12 tumor cultures with neither a significant increase nor significant decrease in cell viability (Fig. 3A). However, higher doses of 10 µM CBD (n = 11 primary cultures, n = 2 from the same patient) led to a significant decrease in cell viability in 3/11 (27%) primary cultures (n = 2 from the same patient, nonfunctional siNET primary plus metastasis, n = 1 nonfunctional panNET) (Fig. 3B). With 10 µM CBD, a significant increase in cell viability was found in 2/11 primary cultures (18%, n = 2 siNETs with carcinoid syndrome). All tumor cultures with significant effects (decrease or increase) were from metastatic NETs. Indeed, a majority of NET patients (9/11) presented with metastatic disease.
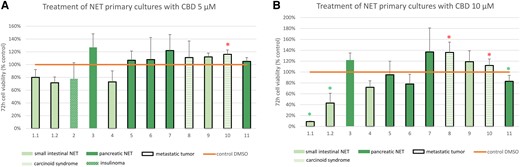
Cell viability of NET primary cultures after 72 hours incubation with (A) CBD 5 µM (n = 12) and (B) CBD 10 µM (n = 11). Primary cultures 1.1 and 1.2 are from the same patient. Small intestinal NETs in light green; pancreatic NETs in dark green. Functionally active NETs: Carcinoid syndromes in striped light green (tumors 8, 10); insulinoma in patterned dark green (tumor 2). Each cell viability experiment comprised 3 to 8 samples per drug concentration and patient. The mean values ± SD are shown. Green asterisk: significant decrease of cell viability P < .05. Red asterisk: significant increase of cell viability P < .05.
Abbreviations: CBD, cannabidiol; NET, neuroendocrine tumor.
Individual Drug Responsitivities of PPGL Primary Cultures to CBD and Correlation With Cluster Affiliation
Opposing effects of CBD treatment were similarly found in PPGL primary cultures: in 5/35 (14%) primary cultures, clinically relevant doses of 5 µM CBD significantly decreased cell viability after 72 hours compared to the untreated control, while in 6/35 (17%) primary cultures, 5 µM CBD significantly increased cell viability compared to the untreated control (Fig. 4). In the remaining tumor cultures (24/35), neutral effects with neither a significant decrease nor increase in cell viability were observed. Interestingly, 4/5 (80%) PPGL primary cultures with a significant decrease in cell viability in response to CBD showed cluster 2-associated PVs in NF1 (n = 3 germline, n = 1 somatic) (Fig. 4). In the 1 remaining tumor, germline testing was negative and somatic testing was not available. Of the 6 PPGLs with a significant increase in cell viability, 2/6 showed cluster 1-associated PVs (n = 1 germline SDHB, n = 1 somatic SDHD), 1/6 had no germline and no somatic PV, 1/6 showed no germline PV and somatic testing was not available, while for 2/6 no germline and no somatic testing was available [Supplementary Table S3 (50)].
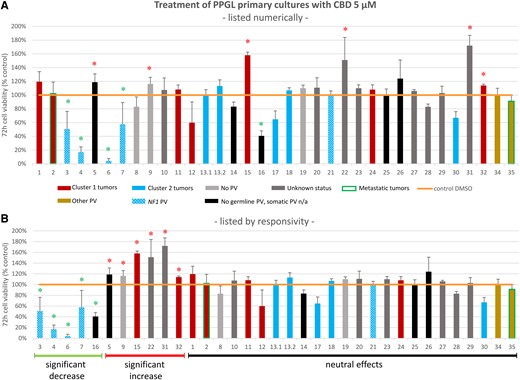
Cell viability of different human PPGL primary cultures (n = 35, n = 2 from the same patient) after incubation with 5 μM CBD for 72 hours (A) listed in numerical order and (B) listed in order of responsivity to CBD (significant decrease of cell viability, significant increase in cell viability, no significant decrease or increase of cell viability). Primary cultures 13.1 and 13.2 are from the same patient. Cluster 1-related PVs in red: SDHB (tumors 1, 32), SDHB and VUS in EPAS (tumor 2), SDHC (tumor 24), SDHD (tumors 11, 15), VUS in VHL (tumor 12). Cluster 2-related PVs in blue: NF1 (tumors 3, 4, 6, 7, 21; patterned blue), RET (tumors 13.1, 13.2, 17), HRAS (tumor 18), MAX (tumor 30). Other PVs in gold: BRCA (tumor 34), ATRX (tumor 35). Metastatic tumors 2, 35 framed in green. Germline and somatic testing was negative in tumors 8, 9, 19 (light grey). Tumors with negative germline testing and not available somatic status in black. Tumors without available germline and somatic status (unknown status) in dark grey. The detailed listing of the germline/somatic status of the individual PPGL patients can be found in Supplementary Table S3 (50). Each cell viability experiment comprised 3 to 8 samples per drug concentration and patient. The mean values ± SD are shown. Green asterisk: significant decrease of cell viability P < .05. Red asterisk: significant increase of cell viability P < .05.
Abbreviations: CBD, cannabidiol; PPGL, pheochromocytoma and paraganglioma; PV, pathogenic variant; VUS, variant of unknown significance.
Moreover, a comparison between cluster 1-related vs cluster 2-related PPGL primary cultures revealed significant differences: cluster 2 PPGL primary cultures showed a significantly stronger decrease in cell viability in response to 5 µM CBD compared to cluster 1 PPGL primary cultures (P = .042) (Fig. 5). CBD additionally demonstrated particular efficacy in PPGLs with PVs in NF1, with a significantly stronger reduction in cell viability in NF1-mutated PPGLs compared to cluster 1 PPGLs (P = .004) and compared to PPGLs without germline PV + no cluster 1/2 somatic PV or unknown somatic status (P = .015). This latter group includes all PPGLs with negative germline testing results plus either negative somatic testing results or unavailable somatic testing results or somatic PV in BRCA or ATRX (neither of which are associated with the PPGL clusters 1 or 2). No significant differences in the efficacy of CBD were found between cluster 1 PPGLs and PPGLs without germline PV + no cluster 1/2 somatic PV or unknown somatic status.
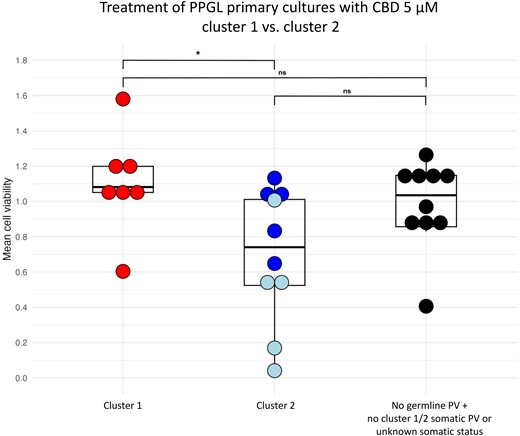
Boxplot of PPGL primary cultures after 72 hours incubation with 5 µM CBD: comparison between cluster 1-related (red) and cluster 2-related (blue) tumors. PPGLs with PVs in NF1 are shown in light blue. Additionally, there is a comparison of cluster 1-related and cluster 2-related tumors with tumors without germline PV (and without cluster 1/2 somatic PV or with unknown somatic status) (black). Each cell viability experiment comprised 3 to 8 samples per drug concentration and patient. The mean values are shown as 1 dot. This figure was created with the open-source statistics software R (Version 4.3.1, R Foundation for Statistical Computing, Vienna, Austria). *P < .05.
Abbreviations: CBD, cannabidiol; ns, nonsignificant; PPGL, pheochromocytoma and paraganglioma; PV, pathogenic variant.
After treatment with higher doses of 10 µM CBD, 9/33 (27%) primary cultures, including 1 metastatic PCC, showed a significant decrease in cell viability compared to the untreated control (Fig. 6). Of these primary cultures, 5/9 were from cluster 2 tumors [n = 3 NF1, n = 2 RET (of these n = 1 MEN2A, n = 1 MEN2B)], 1/9 (metastatic PCC) showed a somatic PV in ATRX, 1/9 showed no germline and no somatic PV, 1/9 showed no germline PV, while somatic status was not available; for 1/9 PPGLs with a significant decrease in cell viability, germline and somatic status was not available [Fig. 6, Supplementary Table S3 (50)]. The percentage of primary cultures with a significant increase in cell viability remained similar compared with lower doses of CBD (6/33, 18%). None of the 6 primary cultures with a significant increase in cell viability showed a cluster 2 PV. Moreover, neither of the 2 metastatic PPGL primary cultures showed a significant increase in cell viability in response to CBD, while the metastatic PCC primary culture with an ATRX PV showed a significant decrease in cell viability.
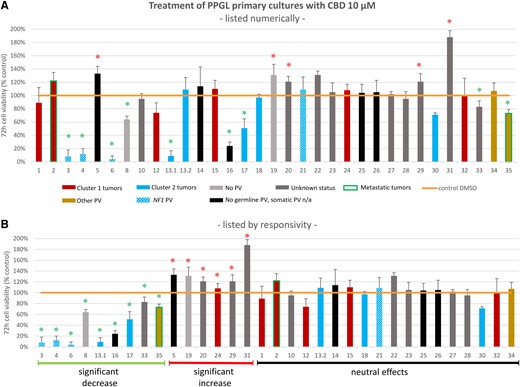
Cell viability of different human PPGL primary cultures (n = 33, n = 2 from the same patient) after incubation with 10 μM CBD for 72 hours (A) listed in numerical order and (B) listed in order of responsivity to CBD (significant decrease of cell viability, significant increase of cell viability, no significant increase or decrease of cell viability). Primary cultures 13.1 and 13.2 are from the same patient. Cluster 1-related PVs in red: SDHB (tumors 1, 32), SDHB and VUS in EPAS (tumor 2), SDHC (tumor 24), SDHD (tumor 15), VUS in VHL (tumor 12). Cluster 2-related PVs in blue: NF1 (tumors 3, 4, 6, 21; patterned blue), RET (tumors 13.1, 13.2, 17), HRAS (tumor 18), MAX (tumor 30). Other PVs in gold: BRCA (tumor 34), ATRX (tumor 35). Metastatic tumors 2, 35 framed in green. Germline and somatic testing was negative in tumors 8, 19 (light grey). Tumors with negative germline testing and not available somatic status in black. Tumors without available germline and somatic status (unknown status) in dark grey. The detailed listing of the germline/somatic status of the individual PPGL patients can be found in Supplementary Table S3 (50). Each cell viability experiment comprised 3 to 8 samples per drug concentration and patient. The mean values ± SD are shown. Green asterisk: significant decrease of cell viability P < .05. Red asterisk: significant increase of cell viability P < .05.
Abbreviations: CBD, cannabidiol; PPGL, pheochromocytoma and paraganglioma; PV, pathogenic variant.
Discussion
The medical use of cannabinoids, including CBD as single substance, is already established and approved for many indications, including multiple sclerosis-induced spasticity, cancer-associated pain, cancer-associated loss of weight or loss of appetite, as well as treatment of seizures in Lennox-Gastaut syndrome or Dravet syndrome (27). Since legalization of recreational cannabis use is now being increasingly discussed in many countries including those in Europe and some states of the United States, cannabinoids may be expected to receive even more widespread use. Therefore, the evaluation of cannabinoids not only in healthy subjects but also in cancer patients becomes increasingly clinically relevant.
While studies have shown anticancer properties of cannabinoids in some tumor entities in vitro (28-34), and recently also in patients with glioblastoma (35), the effects still remain largely unexplored in many tumor types including NETs and PPGLs. Glioblastomas, NETs and PPGLs are all of neuroectodermal origin (37), and therefore similar therapeutic approaches may be effective.
We thus evaluated the effects of CBD in PPGL and NET cell lines and found significantly decreased cell viability by CBD in the PPGL cell lines MPC and MTT (both with heterozygous NF1 gene knockout) as well as the pancreatic NET cell line BON1 and the human pulmonary NET cell line NCI-H727. While human midgut NET (GOT1) cells showed no significant changes in cell viability, human pancreatic NET (QGP-1) cell viability, in contrast, was even significantly increased after CBD treatment. These opposing effects, particularly in BON1 and QGP-1, which are both pancreatic NET cell lines, might be related to the genetic profiles of these cell lines, with BON1 showing, in contrast to QGP-1 cells, an additional PV in the mTOR/kinase signaling-related gene TSC2 (51), among others.
Consistent with the MPC cell line data, in PPGL (MPC) cell spheroids (with a heterozygous NF1 gene knockout), CBD similarly showed efficacy leading to a significant reduction in spheroid diameter. Interestingly, CBD led to an even stronger reduction in spheroid diameter of the HIF-2α-expressing MPC spheroids compared to the control spheroids without HIF-2α expression. Therefore, hypoxia-induced upregulation of HIF-2α (15, 49) in addition to the NF1 PV may even improve the anti-tumor effect of CBD. This might be due to increased aggressiveness with faster tumor growth and, simultaneously, pseudohypoxic conditions, both leading to a larger necrotic area in the spheroid tumor in response to therapy. Therefore, we speculate that a PV associated with an aggressive phenotype (eg, cluster 1 PV) in addition to a cluster 2 PV might be the reason for the improved CBD effect in NF1-mutated MPC cells expressing HIF-2α.
Due to these interesting results, we then investigated CBD in surgery-derived human NET and PPGL primary cultures and also found opposing effects of CBD on individual patient tumors. On the one hand, we found significant antitumor effects of CBD at clinically relevant doses, leading to tumor cell death in 14% of PPGLs and, at slightly higher doses, to tumor cell death in 27% of PPGLs and 27% of NETs. On the other hand, we found significant tumor-promoting effects of CBD at clinically relevant doses in 17% of PPGLs and 8% of NETs and, at higher doses, in 18% of PPGLs and 18% of NETs. In the remaining tumor primary cultures, neither a significant decrease nor a significant increase in cell viability was seen. A comparison of cluster 1 PPGLs with cluster 2 PPGLs revealed significantly stronger responsivity, in terms of a decrease in cell viability, of cluster 2-related PPGLs to CBD compared to cluster 1-related PPGLs. Interestingly, 4/5 PPGL primary cultures with a significant decrease in cell viability in response to 5 µM CBD showed a confirmed PV in the cluster 2-associated gene NF1. Their significant responsivity to CBD is consistent with our observations in the murine PCC cell lines and spheroids with heterozygous NF1 gene knockout.
Our preliminary results might be of some significance clinically, since NF1 is mutated or suppressed in a variety of neuroectodermal tumors. For instance, the frequency of somatic NF1 PVs is reported to be 14% to 23% in glioblastoma and 21% to 26% in PPGL (52), as well as up to 7% in NETs (53, 54). Loss of the NF1 gene product neurofibromin function due to inactivating PVs in NF1 results in enhanced and prolonged activation of Ras-Raf-Mek-Erk- and PI3K-Akt-mTOR-signaling (52, 55), and both pathways may play a role in the previously reported antitumor potential of cannabinoids (56, 57). Moreover, therapeutics that inhibit these kinase-signaling pathways, such as the mTORC1 inhibitor everolimus alone or in combination with the tyrosine kinase inhibitors cabozantinib or sunitinib, have shown significant antitumor effects in cluster 2-related PPGL patient primary cultures and cell lines, including those with NF1 PVs (44, 45, 58, 59).
Interestingly, 1 metastatic PCC with a somatic PV in ATRX, which is not associated with cluster 1 or cluster 2, also showed a significant decrease in cell viability in response to CBD. ATRX PVs can also be found in 17.6% of NETs (60) and a simultaneous occurrence of NF1 and ATRX PVs can be found in 1.3% of patients in the TCGA Pan Cancer dataset. In our study, significant antitumor effects of CBD were found in the NET cell line BON1 with an ATRX PV in addition to a TSC2 PV (51), among others. Therefore, we speculate that, in NETs, antitumor effects of CBD could be mediated by the simultaneous occurrence of an aggressive PV in addition to an mTOR/kinase signaling-related PV.
Antitumor and antiproliferative effects of CBD have previously been demonstrated in many cancer types (28-35, 61), but the tumor-promoting effects, which we now report in our PPGL and NET primary cultures, have to our knowledge not been reported previously. However, increased tumor growth induced by Δ9-THC, the second major component of cannabis and nabiximols (Sativex®), has been demonstrated in vivo in murine lung cancer models through suppression of the anticancer immune response (62). As a next step, it would be interesting to evaluate whether Δ9-THC and the combination of CBD/Δ9-THC also show opposing effects in PPGLs and NETs, depending on their clinical and genetic features.
Our study is the first to offer data on the responsivity of individual patient-derived PPGLs and NETs to CBD depending on different clinical and genetic characteristics. The only available preclinical studies evaluating cannabinoids in PPGLs were performed in PC12 rat PCC cells (38-40), and currently no data on NETs are available. Another strength of this present study is the inclusion of a relatively large number of patient-derived PPGL/NET primary cultures (n = 48), which generally are rare tumor entities.
The most important findings of our study are, on the one hand, the specific antitumor effects of CBD in cluster 2 PPGLs and, of these, particularly in NF1-mutated tumors. This demonstrates the potential implementation of personalized anticancer therapy depending on genetic background. It also cannot be excluded that the “neutral” effects of CBD in some tumor cultures in vitro might result in tumor-stabilizing effects in patients. On the other hand, we found significant CBD-induced tumor growth in 8% to 18% of tumor cultures, which is relevant not only with regards to the potential application of cannabinoids as anticancer treatment but also with regards to the already established application of cannabinoids as supportive drugs for cancer patients (for pain, weight and appetite management). While currently only Δ9-THC (Dronabinol®) is approved for this indication, a recent phase 3 clinical trial has shown benefits of nabiximols (CBD + Δ9-THC) as an adjunctive therapy in cancer patients with uncontrolled pain (63). Interestingly, in our study, specifically metastatic midgut siNETs with the carcinoid syndrome responded with tumor growth to CBD, a finding that needs to be confirmed in a larger cohort and further mechanistically explored. Especially in midgut NETs, the carcinoid syndrome can cause chronic diarrhea and mesenterial tumor-induced fibrosis with a desmoplastic reaction, which in turn can lead to stenosis and mesenteric angina with bowel ischemia (64). The effects of cannabinoids on diarrhea-predominant irritable bowel syndrome and on cancer-related pain have been investigated previously (65-71), and clinical guidelines with controversial comments regarding the use of cannabinoids in cancer-related pain have been published (72-74). At present, the use of CBD in patients with NETs/PPGLs, particularly with siNETs and the carcinoid syndrome or with PPGLs not related to cluster 2, should therefore be carefully considered, since it cannot be excluded that CBD may lead to tumor progression in vivo.
Thus, we established individualized drug testing of primary cultures from surgically removed PPGLs and NETs. This technique of prior drug testing in patient-derived primary cultures before the application of cannabinoids (even as supportive drugs for pain, weight, appetite management, or as health supplements) could be employed in clinical practice, since each individual tumor may react differently to CBD.
As previously described (45), limitations of our primary culture studies include the lack of the tumor microenvironment; moreover, disease stabilization, an important parameter of drug efficacy in vivo, cannot be explored in this model. This is because our primary culture data mostly represent tumor cell death (probably correlating with partial response in vivo) and tumor-promoting effects, with neutral effects possibly correlating with disease stabilization in vivo. This may result in discrepancies between primary culture and in vivo data.
Additionally, while we were able to emphasize the interesting aspect that in metastatic NETs, CBD shows both significant antitumor and significant tumor-promoting effects, our number of NET tumor samples included is too low to conclude that only metastatic patients respond to CBD (only 2/11 NETs in our cohort are nonmetastatic). It is, moreover, known that NETs show a higher metastatic risk compared to PPGLs, which might also influence the results. Moreover, germline or somatic testing of patients with NETs was not performed in our study (apart from only 1 NET patient with suspected bilateral renal cell carcinoma who received germline sequencing with negative results). This is because genetic sequencing of NETs is currently neither performed nor recommended as clinical standard practice. The reason for that is the high heterogeneity of NET PVs, which would require large panel diagnostics or whole-exome sequencing for reliable identification of driver PVs. Even though the incidence of NF1 PVs is lower in NETs in comparison to PPGLs (reported to be up to 7% in NETs) (53, 54), other genetic alterations in ATRX/DAXX/MEN1 and in the PI3K-Akt-mTOR signaling cascade can still be frequently detected in pancreatic NETs (55, 75, 76). In siNETs, loss of heterozygosity of chromosome 18 and CDKN1B PVs are frequent findings (77-79). In high-grade neuroendocrine neoplasms G3, a specific spectrum of PVs has been reported (54, 80). Moreover, comparative whole-exome sequencing of NET primary tumors and metastases has been previously reported, and specific alterations have been defined (81-84). Target actionability has been calculated to be approximately 50% in neuroendocrine neoplasms, highlighting the future potential of molecular profiling using large panel diagnostics or whole-exome sequencing (85, 86). Therefore, knowledge regarding the genetic status of NETs is both clinically and scientifically relevant and indeed should be further explored in the future.
Our findings of the specific individual antitumor potential of CBD in NF1 tumors should be cross-validated in vivo prior to translation to a clinical phase 2 study in patients with NF1 disease or other PPGLs/NETs with a similar molecular background, by analogy to glioblastoma multiforme.
Conclusions
We found significant opposing effects of CBD in patient-derived PPGL and NET primary cultures. This study offers novel data on the antitumor efficacy of CBD in individual patient tumors (with particular efficacy in NF1- and other cluster 2-associated PPGLs), as well as evidence for potential tumor-promoting effects of CBD in some PPGLs and NETs. Therefore, caution is needed when administering cannabinoids to patients with PPGLs/NETs as supportive therapies and possibly as health supplements. Overall, our data represent a further step towards true precision medicine in PPGL/NET patients.
Acknowledgments
We thank Daniela Stanke for her technical support. This paper contains parts of the unpublished doctoral thesis of Laura Schober.
Funding
This research was in part supported by the German Research Foundation [Deutsche Forschungsgemeinschaft (DFG)] within the CRC/Transregio 205/2, project number 314061271—TRR 205 “The Adrenal: Central Relay in Health and Disease” and the Immuno-TargET project under the umbrella of University Medicine Zurich.
Disclosures
The authors have nothing to disclose.
Data Availability
Original data generated and analyzed during this study are included in this published article or in the data repositories listed in References.
References
Author notes
K.W. and L.S. contributed equally to this work as first authors.
S.N. and K.Z. contributed equally to this work as senior authors.