-
PDF
- Split View
-
Views
-
Cite
Cite
Anira Iqbal, Keren Zhou, Sangeeta R Kashyap, M Cecilia Lansang, Early Post-Renal Transplant Hyperglycemia, The Journal of Clinical Endocrinology & Metabolism, Volume 107, Issue 2, February 2022, Pages 549–562, https://doi.org/10.1210/clinem/dgab697
- Share Icon Share
Abstract
Though posttransplant diabetes mellitus (PTDM, occurring > 45 days after transplantation) and its complications are well described, early post–renal transplant hyperglycemia (EPTH) (< 45 days) similarly puts kidney transplant recipients at risk of infections, rehospitalizations, and graft failure and is not emphasized much in the literature. Proactive screening and management of EPTH is required given these consequences.
The aim of this article is to promote recognition of early post-renal transplant hyperglycemia, and to summarize available information on its pathophysiology, adverse effects, and management.
A PubMed search was conducted for “early post–renal transplant hyperglycemia,” “immediate posttransplant hyperglycemia,” “post–renal transplant diabetes,” “renal transplant,” “diabetes,” and combinations of these terms. EPTH is associated with significant complications including acute graft failure, rehospitalizations, cardiovascular events, PTDM, and infections.
Patients with diabetes experience better glycemic control in end-stage renal disease (ESRD), with resurgence of hyperglycemia after kidney transplant. Patients with and without known diabetes are at risk of EPTH. Risk factors include elevated pretransplant fasting glucose, diabetes, glucocorticoids, chronic infections, and posttransplant infections. We find that EPTH increases risk of re-hospitalizations from infections (cytomegalovirus, possibly COVID-19), acute graft rejections, cardiovascular events, and PTDM. It is essential, therefore, to provide diabetes education to patients before discharge. Insulin remains the standard of care while inpatient. Close follow-up after discharge is recommended for insulin adjustment. Some agents like dipeptidyl peptidase-4 inhibitors and glucagon-like peptide-1 receptor agonists have shown promise. The tenuous kidney function in the early posttransplant period and lack of data limit the use of sodium-glucose cotransporter 2 inhibitors. There is a need for studies assessing noninsulin agents for EPTH to decrease risk of hypoglycemia associated with insulin and long-term complications of EPTH.
Hyperglycemia is frequently observed after kidney transplantation and is a risk factor for increased readmission, infection, and acute graft rejection rates. However, hyperglycemia within the first 45 days after transplant (which we will refer to as early post–renal transplant hyperglycemia or EPTH) has not been given as much attention as the later period, perhaps because of less clarity in its definition and clinical implications. Conceivably, the term EPTH might also refer to other organ transplants in the future, but since there are unique pathophysiologic mechanisms and specific immunosuppressive treatment timelines in kidney transplantation, our review is focused on this organ.
The nomenclature for hyperglycemia or diabetes after transplantation had led to such confusion in the literature that an international consensus meeting was held in Vienna in 2013 to discuss challenges surrounding diabetes after renal transplantation at which the term posttransplant diabetes mellitus (PTDM) was proposed (1). PTDM includes newly diagnosed diabetes approximately more than 45 days after transplant, irrespective of whether it was present but undetected before the transplant. However, EPTH (stated as ≤ 45 days after transplant in the meeting) was excluded from the term PTDM. Before PTDM, the term new-onset diabetes after transplant (NODAT) was used and included patients without known pretransplant diabetes who had a new diagnosis of diabetes any time after transplant (2). The term NODAT had fallen out of favor because it implied exclusion of patients with diabetes before transplant but included patients who might have had unrecognized diabetes before transplant. The term transplant-associated hyperglycemia has also been infrequently used to include patients with hyperglycemia at any point after transplant (3) (Fig. 1). Most published studies and guidelines focus on PTDM/NODAT because these are known risk factors for posttransplant complications such as infections, readmissions, graft failure, and cardiovascular events (4-8). However, EPTH is important to highlight. For our article, we define hyperglycemia as any episode of fasting glucose (FG) greater than or equal to 140 mg/dL in the inpatient setting or FG greater than or equal to 126 mg/dL in the outpatient setting or random glucose (RG) greater than or equal to 200 mg/dL or requirement of insulin at any time after surgery. In the inpatient setting immediately after transplant, RG greater than or equal to 200 mg/dL and requirement of insulin is more commonly used as a measure of hyperglycemia.
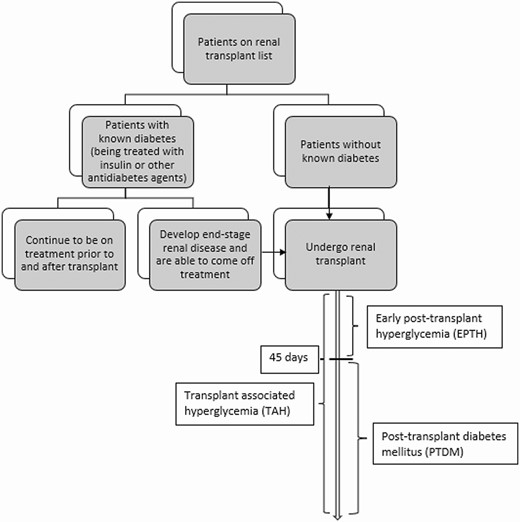
Terminologies used for post–renal transplant hyperglycemia and timing.
Among patients who have undergone renal transplant, diabetes remains the most frequent clinical condition listed, making up to 30% of patients in 2018 (9). Many patients with preexisting diabetes experience better glycemic control while in the end-stage renal disease (ESRD) state, with a resurgence of hyperglycemia after kidney transplant. Maintaining adequate glucose control after transplant in these patients is necessary to prevent recurrent diabetic nephropathy and transplant failure in the long term (10). Even in patients without known pretransplant diabetes, monitoring for hyperglycemia posttransplant is important. EPTH occurs in 75% to 92% of patients within the first week (11-13). In a retrospective study conducted by Chakkera et al (11) of 424 patients who underwent renal transplant, 100% of patients with and 87% without pretransplant diabetes had evidence of inpatient hyperglycemia (RG ≥ 200 mg/dL or physician-instituted insulin therapy) that was sustained throughout hospitalization (72 hours of hospital stay). EPTH is a risk factor for readmissions and infections and can affect morbidity and mortality in the transplant patient. We herein present data on the recognition, pathophysiology, adverse effects, and management of EPTH.
Patient Cases
Case 1
A 64-year-old man with ESRD secondary to diabetes presented to our institution for kidney transplant. He had been on oral diabetes medications for a few years, and then was transitioned to basal-bolus insulin. He was started on hemodialysis in 2013, after which his insulin requirement decreased until he was able to completely stop using insulin in 2017. In 2020, he received a deceased donor kidney. He received methylprednisolone the day of surgery, and on postoperative day (POD) 1, followed by prednisone 60 mg twice a day on POD 2, tapering until the dose was down to 7.5 mg on POD 56 onward. Tacrolimus was also initiated during admission. The inpatient endocrine consult team was notified immediately after transplant.
He developed hyperglycemia on POD 1 and was given insulin lispro based on correction scale, given his lean habitus, lack of appetite, and low insulin requirement. He was discharged on insulin glargine U100 10 units in the morning, and an insulin lispro correction scale with meals. He was seen by an endocrinologist at the renal transplant clinic 2 days after discharge, when scheduled insulin lispro 2 units with meals was added. At the next clinic visit after 4 days, insulin was further uptitrated. At 2 weeks after transplantation, the patient contracted COVID-19, was readmitted, and was kept on home doses of insulin. After discharge, he continued to be followed by the endocrinology team closely and was eventually transitioned to an oral agent (glimepiride 2 mg daily) approximately 8 weeks after transplant.
Case 2
A 56-year-old man with autosomal dominant polycystic kidney disease and stage 5 chronic kidney disease presented to our institute for kidney transplant. He had no prior history of diabetes and there was no pretransplant glycated hemoglobin A1c (HbA1c) on file. He underwent living donor kidney transplant in February 2020. Immunosuppression was initiated with tacrolimus, glucocorticoid, and mycophenolate mofetil. He developed hyperglycemia while an inpatient. He was started on scheduled neutral protamine Hagedorn (NPH) insulin 10 units with breakfast along with insulin glulisine correction scale with meals. Prior to discharge on POD 2, he was seen by a registered dietician, pharmacist, and diabetes educator to teach him about new insulin use and signs of hypoglycemia. Two days after discharge, he was seen by the endocrinology team and his NPH insulin was decreased to 8 units. Correctional insulin was discontinued given tapering dose of glucocorticoids and lower blood glucose (BG) values. At a follow-up visit 1 week later, he reported increased appetite and higher glucose levels, so NPH insulin was increased and scheduled insulin glulisine with dinner was added. One month later, prednisone was titrated down to 7.5 mg daily at which time insulin was stopped and he was transitioned to alogliptin 25 mg daily with good BG control.
Case 1 demonstrates the return of hyperglycemia in a patient with known diabetes after transplant, whereas case 2 shows the onset of hyperglycemia requiring treatment in a patient without prior known diagnosis of diabetes.
Pathophysiology of Post–Renal Transplant Hyperglycemia
Post–renal transplant hyperglycemia is a result of insulin resistance and relative insulin deficiency, like type 2 diabetes or glucocorticoid-induced diabetes. However, several specific considerations need to be highlighted. Endogenous insulin enters the bloodstream via the portal vein and is mainly cleared by the liver, with the kidney playing a secondary role. Exogenous insulin, on the other hand, bypasses first-pass hepatic excretion, and is cleared proportionately more by the kidneys (14). Therefore, renal function directly affects the metabolism of injected insulin for treatment of diabetes leading to increased insulin susceptibility and predisposition to hypoglycemia. As renal disease progresses, insulin requirement decreases because decreased clearance by the kidney. In addition, uremia from renal failure causes nausea and loss of appetite, thus reducing hepatic glycogen stores, causing fasting hypoglycemia. Accumulation of uremic toxins also impairs the degradation of insulin in the liver, prolonging the half-life of endogenous insulin (15). Approximately 20% of gluconeogenesis occurs in the kidneys, which is further decreased in renal dysfunction (14). It is, thus, not uncommon for patients with type 2 diabetes, who previously needed several oral antidiabetic agents and/or insulin, to no longer need these while awaiting kidney transplantation. After successful kidney transplant, insulin clearance improves remarkably, which unmasks the preexisting glucose intolerance. Appetite improves because of the resolution of uremia, and with the addition of oral glucocorticoids. This results in repletion of hepatic glycogen stores, glycogenolysis, and renal gluconeogenesis resumption, adding to hyperglycemia (16, 17). The use of oral glucocorticoids as posttransplant immunosuppression increases insulin resistance at the skeletal muscle and adipose tissue level, contributing to hyperglycemia (18-20). Other immunosuppressants, such as cyclosporine and tacrolimus, are reported to decrease insulin secretion, although this has recently been challenged as further discussed later. Surgical stress and posttransplant infections can further increase the risk of EPTH.
In case 1, the patient ceased to have exogenous insulin requirement before transplant. He developed hyperglycemia postoperatively requiring insulin, which was sustained throughout his hospitalization as well on discharge. This demonstrates the known phenomenon of a reduced-to-nil insulin requirement during ESRD and resurgence of hyperglycemia after renal transplant. In case 2, there was no exogenous insulin requirement before transplant but he still developed hyperglycemia postoperatively. The possible reasons for this are discussed later.
Risk Factors for Early Post–Renal Transplant Hyperglycemia
Impaired Pretransplant Glycemic Control
The level of FG pretransplant is the strongest risk factor for EPTH (Table 1). In a retrospective study of 490 kidney transplant recipients with pretransplant FG less than 126 mg/dL, Cosio et al (12) observed that increased FG levels before transplantation were related to higher glucose levels 1 week post transplant (FG ≥ 126 mg/dL or requiring insulin/oral antidiabetic agents). The risk of post–renal transplant hyperglycemia also increased as the fasting pretransplant glucose levels increased. Sheu et al (21) included patients with preexisting diabetes in their retrospective analysis and found that this population was at higher risk of developing hyperglycemia in the early posttransplant period compared to those without preexisting diabetes. Preexisting diabetes was also associated with increased rejection rates, possibly due to increased ischemic-reperfusion injury.
Risk factors for early post–renal transplant hyperglycemia and supporting evidence
Risk factor . | Pathophysiology . | Reference studies . |
---|---|---|
Impaired pretransplant glycemic control | Loss of β-cell function and mass predisposing to hyperglycemic effects of glucocorticoids, underlying insulin resistance | (12, 21) |
Glucocorticoids | Increased insulin resistance at skeletal muscle and liver, hepatic gluconeogenesis | (1, 18-20) |
Calcineurin inhibitors, mTOR inhibitors | Possible impaired insulin secretion, insulin resistance | (22-30) |
Surgical stress, post-operative pain, increased appetite | Increased cytokines and stress hormones | (31) |
Pretransplant and posttransplant infections (chronic hepatitis C, CMV) | Increased cytokines and stress hormones | (32-34) |
Increasing age, obesity, and elevated triglyceride levels | Developing insulin resistance, relative insulin deficiency | (12, 35-37) |
Risk factor . | Pathophysiology . | Reference studies . |
---|---|---|
Impaired pretransplant glycemic control | Loss of β-cell function and mass predisposing to hyperglycemic effects of glucocorticoids, underlying insulin resistance | (12, 21) |
Glucocorticoids | Increased insulin resistance at skeletal muscle and liver, hepatic gluconeogenesis | (1, 18-20) |
Calcineurin inhibitors, mTOR inhibitors | Possible impaired insulin secretion, insulin resistance | (22-30) |
Surgical stress, post-operative pain, increased appetite | Increased cytokines and stress hormones | (31) |
Pretransplant and posttransplant infections (chronic hepatitis C, CMV) | Increased cytokines and stress hormones | (32-34) |
Increasing age, obesity, and elevated triglyceride levels | Developing insulin resistance, relative insulin deficiency | (12, 35-37) |
Abbreviations: CMV, cytomegalovirus; mTOR, mechanistic target of rapamycin.
Risk factors for early post–renal transplant hyperglycemia and supporting evidence
Risk factor . | Pathophysiology . | Reference studies . |
---|---|---|
Impaired pretransplant glycemic control | Loss of β-cell function and mass predisposing to hyperglycemic effects of glucocorticoids, underlying insulin resistance | (12, 21) |
Glucocorticoids | Increased insulin resistance at skeletal muscle and liver, hepatic gluconeogenesis | (1, 18-20) |
Calcineurin inhibitors, mTOR inhibitors | Possible impaired insulin secretion, insulin resistance | (22-30) |
Surgical stress, post-operative pain, increased appetite | Increased cytokines and stress hormones | (31) |
Pretransplant and posttransplant infections (chronic hepatitis C, CMV) | Increased cytokines and stress hormones | (32-34) |
Increasing age, obesity, and elevated triglyceride levels | Developing insulin resistance, relative insulin deficiency | (12, 35-37) |
Risk factor . | Pathophysiology . | Reference studies . |
---|---|---|
Impaired pretransplant glycemic control | Loss of β-cell function and mass predisposing to hyperglycemic effects of glucocorticoids, underlying insulin resistance | (12, 21) |
Glucocorticoids | Increased insulin resistance at skeletal muscle and liver, hepatic gluconeogenesis | (1, 18-20) |
Calcineurin inhibitors, mTOR inhibitors | Possible impaired insulin secretion, insulin resistance | (22-30) |
Surgical stress, post-operative pain, increased appetite | Increased cytokines and stress hormones | (31) |
Pretransplant and posttransplant infections (chronic hepatitis C, CMV) | Increased cytokines and stress hormones | (32-34) |
Increasing age, obesity, and elevated triglyceride levels | Developing insulin resistance, relative insulin deficiency | (12, 35-37) |
Abbreviations: CMV, cytomegalovirus; mTOR, mechanistic target of rapamycin.
Before the development of overt diabetes, there is loss of β-cell function and mass that causes elevated FG (38). Based on cross-sectional studies, normoglycemia can be maintained until approximately 50% of β-cell mass is lost (39). In patients with impaired FG before transplant, there is an underlying loss of more than 50% β-cell mass, which predisposes them to hyperglycemic effects of glucocorticoids. There might also be underlying insulin resistance in these patients that is unmasked with the use of glucocorticoids intra-transplant and post transplant predisposing to EPTH.
Immunosuppression Regimen
Oral glucocorticoids are an integral part of posttransplant immunosuppression and act as one of the biggest risk factors for early hyperglycemia. Suggested mechanisms include increased insulin resistance in skeletal muscle and adipose tissue and hepatic gluconeogenesis (18-20). The incidence of hyperglycemia increases both with the dose and duration of glucocorticoid therapy, although a plateau effect might be seen at a certain dose of glucocorticoids after which there is no worsening hyperglycemia. High-dose glucocorticoids are commonly prescribed with induction and to treat rejection or graft-vs-host disease in the early posttransplant period. Unfortunately, dose reduction in glucocorticoids can also increase the risk for graft rejection. The consensus is that immunosuppression regimens should have the best outcome for the patient and graft survival, irrespective of risk for hyperglycemia (1) (Table 2).
Agent . | Proposed mechanisms of hyperglycemia . |
---|---|
Glucocorticoids (19–21) | Increased insulin resistance at the level of skeletal muscle and liver |
Increased hepatic gluconeogenesis | |
Calcineurin inhibitors (22-25) | Dose-dependent β-cell toxicity, which reduces insulin secretion |
Tacrolimus | Exacerbate insulin resistance |
Cyclosporine | Removal of GLUT4 transporter from cell surface |
Tacrolimus associated with more PTDM than cyclosporine | |
mTOR inhibitors (26) | Impairment of insulin signaling pathway |
Sirolimus | Possibly reduces β-cell function |
Everolimus | Possibly reduces islet mass |
Agent . | Proposed mechanisms of hyperglycemia . |
---|---|
Glucocorticoids (19–21) | Increased insulin resistance at the level of skeletal muscle and liver |
Increased hepatic gluconeogenesis | |
Calcineurin inhibitors (22-25) | Dose-dependent β-cell toxicity, which reduces insulin secretion |
Tacrolimus | Exacerbate insulin resistance |
Cyclosporine | Removal of GLUT4 transporter from cell surface |
Tacrolimus associated with more PTDM than cyclosporine | |
mTOR inhibitors (26) | Impairment of insulin signaling pathway |
Sirolimus | Possibly reduces β-cell function |
Everolimus | Possibly reduces islet mass |
Abbreviations: GLUT4, glucose transporter protein type-4; mTOR, mechanistic target of rapamycin; PTDM, posttransplant diabetes mellitus.
Agent . | Proposed mechanisms of hyperglycemia . |
---|---|
Glucocorticoids (19–21) | Increased insulin resistance at the level of skeletal muscle and liver |
Increased hepatic gluconeogenesis | |
Calcineurin inhibitors (22-25) | Dose-dependent β-cell toxicity, which reduces insulin secretion |
Tacrolimus | Exacerbate insulin resistance |
Cyclosporine | Removal of GLUT4 transporter from cell surface |
Tacrolimus associated with more PTDM than cyclosporine | |
mTOR inhibitors (26) | Impairment of insulin signaling pathway |
Sirolimus | Possibly reduces β-cell function |
Everolimus | Possibly reduces islet mass |
Agent . | Proposed mechanisms of hyperglycemia . |
---|---|
Glucocorticoids (19–21) | Increased insulin resistance at the level of skeletal muscle and liver |
Increased hepatic gluconeogenesis | |
Calcineurin inhibitors (22-25) | Dose-dependent β-cell toxicity, which reduces insulin secretion |
Tacrolimus | Exacerbate insulin resistance |
Cyclosporine | Removal of GLUT4 transporter from cell surface |
Tacrolimus associated with more PTDM than cyclosporine | |
mTOR inhibitors (26) | Impairment of insulin signaling pathway |
Sirolimus | Possibly reduces β-cell function |
Everolimus | Possibly reduces islet mass |
Abbreviations: GLUT4, glucose transporter protein type-4; mTOR, mechanistic target of rapamycin; PTDM, posttransplant diabetes mellitus.
Other immunosuppressants could also contribute to hyperglycemia, including the calcineurin inhibitors (tacrolimus more than cyclosporine) and inhibitors of the mechanistic target of rapamycin (mTOR), such as sirolimus and everolimus. Calcineurin inhibitors (CNIs) are reported to reduce insulin secretion, increase islet cell destruction, and exacerbate insulin resistance (22-25). CNIs inhibit calcineurin-dependent gene transcription that leads to dose-dependent reductions in insulin messenger RNA, cellular insulin content, and glucose-stimulated insulin secretion in β-cell lines and rodent islets (26). In human adipocytes, both cyclosporine and tacrolimus inhibit glucose uptake independent of insulin signaling by removing glucose transporter protein type-4 from the cell surface through an increased rate of endocytosis (29). Tacrolimus has been associated with a higher risk of PTDM compared to cyclosporine, but this trend is unclear in the early posttransplant period. The hyperglycemic effects of these medications are observable as early as 2 months after the initiation of immunosuppression (27). Sirolimus disrupts insulin action through multiple effects on the insulin signal transduction pathway and can reduce β-cell function and islet mass, although its use has decreased recently because of adverse effects (23). However, another line of evidence suggests that insulin secretion may be maintained in the presence of tacrolimus, as demonstrated in a study comparing pancreas-kidney and kidney-alone transplant recipients to islet cell recipients (29). While β-cell secretory capacity was reduced in islet transplant recipients, it was normal in the other 2 groups while they were on the same tacrolimus-based immunosuppression. This was attributed to islet recipients having lower engrafted β-cell mass. None of the groups showed increased insulin resistance, suggesting that tacrolimus might not markedly contribute. This was also noticed in a study using mTOR inhibitors such as sirolimus (30).
Notably, the kidney-alone transplant recipients in the study by Rickels et al (29) did not have diabetes before transplant and the average body mass index of all the participants was 20 to 25. This suggests that CNIs and mTOR inhibitors by themselves might not contribute as much to impaired posttransplant glycemic control as pretransplant risk factors and posttransplant weight gain. Modern lower-dose tacrolimus and sirolimus regimens seem to have a lesser effect on insulin secretion and resistance (29, 30). Further studies are necessary to state this conclusively.
Mycophenolate mofetil, azathioprine, and the new agent belatacept have not been shown to have a large effect on insulin action or glucose metabolism and do not appear to have a major role in early hyperglycemia (27, 40, 41).
Surgical Stress, Nutrition, Physical Activity, Postoperative Pain, and Infections
Surgery and postoperative pain are known to increase risk of hyperglycemia by triggering cytokines and stress hormones. Surgical stress has a deleterious effect on pancreatic β-cell function due to the release of catabolic hormones causing inhibition of insulin secretion resulting in hyperglycemia (29). This hyperglycemia is usually transient and resolves after discharge. In addition, especially in the first few weeks after surgery, patients’ appetite increases compared to when they were in ESRD. High-dose glucocorticoids also increase appetite, and postoperative recovery limits the amount of exercise that can be performed.
The immunosuppressed state post transplant increases the risk of infections. Severe infection or sepsis can precipitate hyperglycemia even in patients with normal glucose tolerance (30, 40). Preexisting chronic infections such as chronic hepatitis C or cytomegalovirus (CMV) can also increase risk of posttransplant hyperglycemia (41).
Early hyperglycemia has also been seen to be associated with increasing age, obesity, and elevated triglyceride levels (12, 31-33).
For the patient described in case 1, his major risk factors for development of EPTH included preexisting diabetes (probably his biggest risk factor), surgical stress, postoperative pain, high-dose glucocorticoids, possibly tacrolimus use, and COVID infection.
For the patient described in case 2, although he did not have known preexisting diabetes, the absence of screening before transplant restricts us in saying that he did not have diabetes. His other risk factors included surgical stress, postoperative pain, glucocorticoids, and possible tacrolimus use.
Complications of Early Post–Renal Transplant Hyperglycemia: Rehospitalizations, Infections, Graft Survival, and Cardiovascular Risk
Inpatient hyperglycemia in general is known to be associated with adverse patient outcomes, higher mortality, and greater costs (42-44) (Table 3).
Complications of early post–renal transplant hyperglycemia and supporting evidence
Complications . | Pathophysiology . | Reference studies . |
---|---|---|
Infections/Rehospitalization | Hyperglycemia affects the immune system by causing changes in leukocyte function, cytokine response, and microvascular response and results in a proinflammatory state causing oxidative stress. | (10, 45-47) |
COVID-19 infection | (48-51) | |
Acute graft rejection, return to dialysis | Hyperglycemia causes attenuation of endothelium-dependent vasodilation causing ischemic injury to graft. The generation of lactate and reactive oxygen species is augmented by hyperglycemia, which could lead to reperfusion injury. | (10, 47, 52-55) |
Increased cardiovascular risk | Hyperglycemia causes endothelial dysfunction through oxidative stress, which is the first step to atherogenesis. Attenuation of endothelium-mediated vasodilation also reduces myocardial perfusion. | (6, 56-59) |
Posttransplant diabetes mellitus | Hyperglycemia might be due to underlying insulin resistance and relative insulin deficiency which can progress to diabetes mellitus if not controlled well. | (6, 12, 13, 18, 47, 60, 61) |
Complications . | Pathophysiology . | Reference studies . |
---|---|---|
Infections/Rehospitalization | Hyperglycemia affects the immune system by causing changes in leukocyte function, cytokine response, and microvascular response and results in a proinflammatory state causing oxidative stress. | (10, 45-47) |
COVID-19 infection | (48-51) | |
Acute graft rejection, return to dialysis | Hyperglycemia causes attenuation of endothelium-dependent vasodilation causing ischemic injury to graft. The generation of lactate and reactive oxygen species is augmented by hyperglycemia, which could lead to reperfusion injury. | (10, 47, 52-55) |
Increased cardiovascular risk | Hyperglycemia causes endothelial dysfunction through oxidative stress, which is the first step to atherogenesis. Attenuation of endothelium-mediated vasodilation also reduces myocardial perfusion. | (6, 56-59) |
Posttransplant diabetes mellitus | Hyperglycemia might be due to underlying insulin resistance and relative insulin deficiency which can progress to diabetes mellitus if not controlled well. | (6, 12, 13, 18, 47, 60, 61) |
Complications of early post–renal transplant hyperglycemia and supporting evidence
Complications . | Pathophysiology . | Reference studies . |
---|---|---|
Infections/Rehospitalization | Hyperglycemia affects the immune system by causing changes in leukocyte function, cytokine response, and microvascular response and results in a proinflammatory state causing oxidative stress. | (10, 45-47) |
COVID-19 infection | (48-51) | |
Acute graft rejection, return to dialysis | Hyperglycemia causes attenuation of endothelium-dependent vasodilation causing ischemic injury to graft. The generation of lactate and reactive oxygen species is augmented by hyperglycemia, which could lead to reperfusion injury. | (10, 47, 52-55) |
Increased cardiovascular risk | Hyperglycemia causes endothelial dysfunction through oxidative stress, which is the first step to atherogenesis. Attenuation of endothelium-mediated vasodilation also reduces myocardial perfusion. | (6, 56-59) |
Posttransplant diabetes mellitus | Hyperglycemia might be due to underlying insulin resistance and relative insulin deficiency which can progress to diabetes mellitus if not controlled well. | (6, 12, 13, 18, 47, 60, 61) |
Complications . | Pathophysiology . | Reference studies . |
---|---|---|
Infections/Rehospitalization | Hyperglycemia affects the immune system by causing changes in leukocyte function, cytokine response, and microvascular response and results in a proinflammatory state causing oxidative stress. | (10, 45-47) |
COVID-19 infection | (48-51) | |
Acute graft rejection, return to dialysis | Hyperglycemia causes attenuation of endothelium-dependent vasodilation causing ischemic injury to graft. The generation of lactate and reactive oxygen species is augmented by hyperglycemia, which could lead to reperfusion injury. | (10, 47, 52-55) |
Increased cardiovascular risk | Hyperglycemia causes endothelial dysfunction through oxidative stress, which is the first step to atherogenesis. Attenuation of endothelium-mediated vasodilation also reduces myocardial perfusion. | (6, 56-59) |
Posttransplant diabetes mellitus | Hyperglycemia might be due to underlying insulin resistance and relative insulin deficiency which can progress to diabetes mellitus if not controlled well. | (6, 12, 13, 18, 47, 60, 61) |
Infections and Rehospitalizations
Hyperglycemia in the immediate period after renal transplant has been associated with increased risk of rehospitalization for infections while being on the same immunosuppressive regimen as patients without hyperglycemia (45). This can be explained by the complex deleterious effects of hyperglycemia on the immune system including changes in leukocyte function, cytokine response, altered microvascular response, and the proinflammatory state caused by hyperglycemia leading to oxidative stress (62). In a study by Thomas et al (10), all patients with poor postoperative glycemic control (mean glucose levels > 200 mg/dL) in the first 100 hours after transplant developed infections. Studies have reported a higher incidence of CMV infection, which is one of the most common postrenal transplant infections, in patients with EPTH (46, 47).
The recent COVID-19 pandemic deserves mentioning. Kidney transplant recipients may be at high risk of severe COVID-19 infection because of long-term immunosuppression, residual chronic kidney disease, and other comorbidities, in particular diabetes and hypertension, which are now recognized as significant factors that influence outcomes in patients with COVID-19 infection (62). A study conducted in the epicenter of the pandemic (Bronx, New York) suggested a prevalence of 23.4% (including asymptomatic patients) of COVID-19 infection in renal transplant recipients (48). Kidney transplant recipients infected with COVID-19 have been seen to have a higher rate of graft rejection and death in a few reports (49, 50). However, in a case report of a patient who contracted COVID-19 and was maintained on conventional-dose immunosuppression during inpatient treatment, it was proposed that the immunosuppressed state could have protected the patient from the cytokine storm and severe immune injury (51). There are not enough data to suggest a modified hyperglycemia management approach for kidney transplant recipients admitted for COVID-19. Following guidelines, these patients are managed with insulin, like other patients with inpatient hyperglycemia as specified further later.
The patient described in case 1 was managed for COVID-19 at home without oxygen requirement or further treatment specific for COVID-19. He continued to require 12 units of glargine in the morning with mealtime lispro increased to 6 units (from 4 units) along with correctional scale. He did not develop diabetic ketoacidosis and was seen in clinic 2 weeks after detection of COVID-19. We now know that, per COVID-19 treatment guidelines laid out by the National Institutes of Health, anti-SARS-CoV-2 monoclonal antibodies are indicated to treat nonhospitalized patients with mild to moderate COVID-19 who are at high risk of clinical progression, such as transplant recipients on immunosuppression (63). Monoclonal antibodies have been shown to reduce the risk of severe disease requiring hospitalization (64). A transplant specialist should also be consulted before adjusting an immunosuppressive regimen and clinicians should pay careful attention to drug-drug interactions and potential toxicities when treating these patients.
Renal Graft Dysfunction and Acute Graft Rejection
Acute rejection is believed to be initiated in the early postoperative period by antigen presentation, possibly in response to allograft inflammation and injury. Early hyperglycemia has been associated with acute graft rejection, which is defined as biopsy-proven or clinical rejection occurring within 20 days of transplantation (10, 47, 52). This was shown in a retrospective study conducted by Thomas et al in 2001 (53) wherein 58% of patients (n = 50) with poor perioperative glycemic control (mean glucose levels ≥ 200 mg/dL) developed acute rejection. High glucose levels in the immediate period after surgery potentiate ischemic injury and can predispose to acute graft rejection (65-67). These patients have increased rates of return to dialysis after transplant, which was found to be related to BG control during transplant admission (54). This suggests that hyperglycemia immediately after transplant may represent a modifiable risk factor for donor graft failure.
While the exact mechanism has not been elucidated, it can be hypothesized that the inflammatory response associated with hyperglycemia contributes to rejection. Hyperglycemia has deleterious effects on the endothelium by attenuation of endothelium-dependent vasodilation resulting in vasoconstriction potentially causing ischemic injury to graft (68). The generation of lactate and reactive oxygen species is augmented by hyperglycemia, which could lead to reperfusion injury (69). The inflammatory response to ischemia/reperfusion and rejection is also exaggerated by hyperglycemia worsening the rejection episode (70).
Wyzgal et al (47) observed that early hyperglycemia was associated with acute graft rejection, worse renal graft function, and higher proteinuria. It is important that there was still a high incidence of acute rejection (37%) in patients who had immediate hyperglycemia but did not end up developing PTDM in the follow-up period. In a study conducted by Schiel et al (55), the prevalence of acute transplant rejections was 16.3% in patients with diabetes and 7.1% in patients without diabetes, although this was not clinically significant (P = .11). In multivariate analysis though, the most important parameter associated with the incidence of transplant rejections was high FG at 6 to 8 weeks, which was significant (P = .009). All other parameters (body mass index, time since transplantation, diabetes duration, immunosuppressive therapy, HbA1c, and human leukocyte antigen mismatch) revealed no associations.
Cardiovascular Complications and Mortality
Renal transplant recipients have an increased risk of premature death, with cardiovascular disease, malignancy, and infectious disease being the predominant causes of mortality (56). Diabetes as a risk factor for increased cardiovascular risk is now widely accepted. Multiple studies have shown hyperglycemia and fluctuating glucose levels to be an independent risk factor for cardiovascular risk (58, 71). This could be attributable to the endothelial dysfunction, caused by hyperglycemia through oxidative stress, which is the first step to atherogenesis. Acute hyperglycemia attenuates endothelium-mediated vasodilation and reduces myocardial perfusion causing increased cardiovascular risk (72).
High FG levels (≥ 100 mg/dL) at 1 month (as well as at 4 and 12 months) post transplant have been significantly related to cardiovascular events such as myocardial infarction, cerebrovascular accidents, and peripheral vascular disease (6). These relationships were independent of other cardiovascular risk factors including older age, pretransplant cardiovascular events, male sex, and dyslipidemia. In a prospective study by of 1410 patients without known diabetes who underwent renal transplant, Valderhaug et al (59) showed that posttransplant hyperglycemia (defined as 2-hour serum glucose after oral glucose tolerance test ≥ 140 mg/dL) at 10 weeks was associated with an approximately 2-fold increased risk of death from cardiovascular disease, malignancy, and infectious disease as compared to patients with normoglycemia. However, data regarding effect of hyperglycemia occurring within 45 days of transplant on cardiovascular risk is scant.
Posttransplant Diabetes Mellitus
In patients without preexisting diabetes before transplant, not everyone who develops early hyperglycemia will progress to PTDM. Of those who do, development of fasting hyperglycemia during the first week post transplant has been shown to be the strongest predictor of PTDM at 1 year (6, 12). Multiple retrospective studies in patients with PTDM have reported a high frequency of early hyperglycemia compared to those who did not develop PTDM (18, 60, 73). Early initiation of basal insulin to achieve glycemic control after kidney transplant may reduce the risk of developing PTDM (13). Wyzgal et al (47) demonstrated in their prospective 3-year study that 75% of people with hyperglycemia during the first week of renal transplantation developed diabetes mellitus in the follow-up period of 3 years.
It is well documented that PTDM is associated with poor graft outcomes, increased infections, cardiovascular disease, and recurrent hospitalizations following renal transplants (4-8). Thus, an increased risk of PTDM in this population calls for early intervention.
Table 3 consolidates and summarizes the available evidence regarding complications of EPTH. Further studies are needed to predict the long-term effects of EPTH on morbidity and mortality. The authors recommend studies looking at long-term cardiovascular effects of EPTH. It would also be beneficial to study the rates of COVID-19 infection in patients undergoing renal transplant during the pandemic (2020-2021) and whether patients with EPTH have been more predisposed to contracting the infection.
Clinical Considerations and Management
Most of the recommendations published on hyperglycemia management after renal transplant are focused on PTDM occurring months or years after surgery and not during the early postoperative period. Management is currently performed on an institutional basis and requires coordinated interdisciplinary care while patients are hospitalized and on discharge.
Inpatient Diabetes Education
Patients with preexisting diabetes may have experienced a decrease in diabetes medication requirements, including insulin, in the setting of ESRD or might have been controlled on diet alone before transplant. After kidney transplantation, there is a resurgence of hyperglycemia as explained earlier. Even for patients without a pretransplant diagnosis of diabetes, most patients develop hyperglycemia while in the hospital (11). Therefore, a significant percentage of patients will need education or reeducation on checking capillary BG via self-monitoring of BG or a continuous glucose monitor. Though nutrition and exercise are also integral components of diabetes management, patients have poor appetite and limited physical activity during this early postoperative period.
At our institute, patients are often discharged 2 days after renal transplantation, unless with complications such as our patient in case 1. Most patients with hyperglycemia are discharged on insulin. To facilitate this, the inpatient endocrine consult team is notified after surgery, and the diabetes educators initiate insulin and glucose meter teaching. We have found that many patients are too tired or overwhelmed after surgery, so we have started the insulin and glucose meter teaching before transplantation for patients not currently on insulin as they approach the top of the transplant list. So far, we have initiated this for patients on the list for deceased kidney donation since there is time to schedule the training. We are evaluating the feasibility of doing this in a timely manner for live donor recipients but the time from evaluation to surgery in this scenario is a bit tighter.
Whereas the patient in case 1 had diabetes before transplant and was well educated about insulin use, our case 2 patient had no prior experience with insulin administration and side effects. Diabetes education must be personalized based on each patient’s experiences, and a brief visit with a diabetes educator and a pharmacist is warranted after transplant before discharge home.
Inpatient Hyperglycemia Management
In the posttransplant hospitalized setting, insulin remains the standard of care for hyperglycemia along with avoidance of all noninsulin antidiabetic agents in accordance with the American Diabetes Association (ADA) standards of care (74). The anti-inflammatory and antioxidant effects of insulin have contributed to the recommendation of its use for correction of postsurgical stress-induced hyperglycemia (61). Optimization of glycemic control is difficult with noninsulin agents and is limited by side effects. Renal transplant patients are more sensitive to glucose variability and may suffer from hypoglycemia, which is also a well-recognized risk factor for complications and could be associated with renal failure in the posttransplant patient (75). Glycemic variability (both hyperglycemia and hypoglycemia) has also been shown to be a risk factor for falls in hospitalized patients (76).
Immediately after transplantation, some institutes might manage their patients in the intensive care unit and treat hyperglycemia aggressively with intravenous insulin, whereas other institutions might manage patients on the regular nursing floor with subcutaneous insulin. A randomized controlled study of kidney transplant recipients designed to assess the effect of tighter glycemic control during the posttransplant hospitalization period (BG 70-110 mg/dL vs < 180 mg/dL) showed that the intensive group experienced not only more hypoglycemia but also more rejection episodes, although the latter was not statistically significant (77). The ADA recommends a target glucose range of 80 to 180 mg/dL in the perioperative period (78). For critically ill patients, the American Association of Clinical Endocrinology (AACE) and the ADA recommend starting an intravenous insulin infusion and frequent glucose monitoring for patients with a BG greater than or equal to 180 mg/dL, targeting a BG of 140 to 180 mg/dL, with lower targets of 110 to 140 mg/dL in selected patients (79). For noncritically ill patients, the Endocrine Society and AACE/ADA guidelines recommended a target premeal BG of less than 140 mg/dL and an RG of less than 180 mg/dL, with a subcutaneous basal-bolus regimen preferred (80). Control of hyperglycemia assists in wound healing, decreases the risk of infection, and reduces osmotic diuresis, which is essential as posttransplant diuresis is a risk factor for volume depletion and hemodynamic instability. Given the hyperglycemia exacerbation caused by glucocorticoids, insulin is used during the inpatient period after transplant, and most individuals are discharged on insulin as well.
Glucose Monitoring and Glycemic Goals
In the early posttransplant period and a few weeks after discharge, frequent point-of-care glucose testing is important to determine glycemic status since HbA1c measures about 3 months of glycemic control and will not be useful in this setting. Postchallenge 2-hour serum glucose measured early after renal transplantation has been found to be superior to FG to detect hyperglycemia even after adjustments for confounding risk factors (59).
Current ADA targets are recommended, using a patient-centered approach, with a goal premeal blood glucose of 80 to 130 mg/dL and a postprandial BG of less than 180 mg/dL (81). In transplant recipients with advanced microvascular and macrovascular complications and/or high hypoglycemic risk, a less stringent premeal goal of 110 to 140 mg/dL is acceptable to avoid hypoglycemia.
Insulin Regimen at Discharge
The current recommendation is insulin therapy on discharge for patients with EPTH, with the introduction of oral hypoglycemic agents as tolerated outpatient on an individualized basis (1). The insulin treatment regimen required at the time of discharge is highly variable and does not readily lend itself to a fixed insulin per kilogram dose in part because of the variability in kidney function, glucocorticoid, and other immunosuppressant doses used, severity of obesity, and other factors that drive insulin resistance in this group. Drug-drug interactions, glucocorticoid dosing changes, and oral intake can influence further regimen choice and dosage.
Discharge algorithms in transplant patients have not been well studied; however, it is reasonable to consider basal-bolus insulin therapy if the total daily dose required at discharge is greater than or equal to 0.25 units/kg (on stable glucocorticoids) (82). Basal insulin with a correctional insulin scale or an oral agent can be considered for those on less than 0.25 units/kg/day insulin, minimal nutritional intake, and stable glucocorticoid dosing. Studies suggest that regimens that include basal insulin significantly reduce the risk for PTDM at 1 year, presumably via insulin-mediated protection of β cells (13). Twelve months after transplantation, all patients in the treatment group (with basal insulin) were insulin independent, whereas 28% controls (on short-acting insulin) required antidiabetic agents. The groups did not differ with regard to insulin sensitivity, but the treatment group showed better β-cell function throughout the 1-year follow-up, as measured by the insulinogenic index.
Noninsulin Agents
Though insulin is the usual management post renal transplant, consideration has been given to noninsulin agents for managing EPTH. Traditional oral agents, such as metformin and sulfonylureas, require dose adjustment for renal function (which varies during the initial posttransplant period) and have significant side effects including gastrointestinal upset and hypoglycemia, respectively. Most studies of noninsulin agents initiate these agents years after transplant and in patients who develop PTDM (Table 4).
Noninsulin agents that could be used for management of early post–renal transplant hyperglycemia
Noninsulin agent . | Considerations for use in EPTH . | Reference studies . | Comments . |
---|---|---|---|
Metformin | o Avoid with tenuous renal function | Not favored in EPTH | |
o Gastrointestinal upset | |||
o Can rarely cause lactic acidosis in impaired renal function | |||
Sulfonylurea | o Require dose adjustment with renal function, which varies in the early posttransplant period | Not favored in EPTH | |
o High risk of hypoglycemia | |||
o Weight gain | |||
GLP-1 RA | o Potential risk of acute kidney injury with higher dose | (83–85) | Need further studies for use in EPTH |
o Cardiovascular benefit | |||
o Weight loss | |||
DPP-4 inhibitors | o Better HOMA-IR and HOMA-B compared to insulin | (86–91) | Favorable studies, need randomized controlled trials to compare glycemic control for EPTH |
o Linagliptin does not require renal dose adjustment | |||
SGLT2 inhibitors | o Avoidance with tenuous kidney function | (92) | Need further studies for use in EPTH |
o Potential for causing dehydration | |||
o Genitourinary infections | |||
o Weight loss | |||
o Blood pressure control | |||
o Increased risk of volume depletion and ketoacidosis perioperatively | |||
o Canagliflozin showed glycemic improvement at 6 months post transplant without significant adverse effects | |||
Thiazolidinediones | o Potential for fluid retention | Not favored | |
o Slow onset of action in improving hyperglycemia | |||
o Weight gain | |||
o Increased risk for heart failure |
Noninsulin agent . | Considerations for use in EPTH . | Reference studies . | Comments . |
---|---|---|---|
Metformin | o Avoid with tenuous renal function | Not favored in EPTH | |
o Gastrointestinal upset | |||
o Can rarely cause lactic acidosis in impaired renal function | |||
Sulfonylurea | o Require dose adjustment with renal function, which varies in the early posttransplant period | Not favored in EPTH | |
o High risk of hypoglycemia | |||
o Weight gain | |||
GLP-1 RA | o Potential risk of acute kidney injury with higher dose | (83–85) | Need further studies for use in EPTH |
o Cardiovascular benefit | |||
o Weight loss | |||
DPP-4 inhibitors | o Better HOMA-IR and HOMA-B compared to insulin | (86–91) | Favorable studies, need randomized controlled trials to compare glycemic control for EPTH |
o Linagliptin does not require renal dose adjustment | |||
SGLT2 inhibitors | o Avoidance with tenuous kidney function | (92) | Need further studies for use in EPTH |
o Potential for causing dehydration | |||
o Genitourinary infections | |||
o Weight loss | |||
o Blood pressure control | |||
o Increased risk of volume depletion and ketoacidosis perioperatively | |||
o Canagliflozin showed glycemic improvement at 6 months post transplant without significant adverse effects | |||
Thiazolidinediones | o Potential for fluid retention | Not favored | |
o Slow onset of action in improving hyperglycemia | |||
o Weight gain | |||
o Increased risk for heart failure |
Abbreviations: DPP-4, dipeptidyl peptidase-4; EPTH, early post–renal transplant hyperglycemia; GLP-1 RA, glucagon-like peptide-1 receptor agonist; HOMA-B, homeostatic model assessment of β-cell function; HOMA-IR, homeostatic model assessment of insulin resistance; SGLT2, sodium-glucose cotransporter-2.
Noninsulin agents that could be used for management of early post–renal transplant hyperglycemia
Noninsulin agent . | Considerations for use in EPTH . | Reference studies . | Comments . |
---|---|---|---|
Metformin | o Avoid with tenuous renal function | Not favored in EPTH | |
o Gastrointestinal upset | |||
o Can rarely cause lactic acidosis in impaired renal function | |||
Sulfonylurea | o Require dose adjustment with renal function, which varies in the early posttransplant period | Not favored in EPTH | |
o High risk of hypoglycemia | |||
o Weight gain | |||
GLP-1 RA | o Potential risk of acute kidney injury with higher dose | (83–85) | Need further studies for use in EPTH |
o Cardiovascular benefit | |||
o Weight loss | |||
DPP-4 inhibitors | o Better HOMA-IR and HOMA-B compared to insulin | (86–91) | Favorable studies, need randomized controlled trials to compare glycemic control for EPTH |
o Linagliptin does not require renal dose adjustment | |||
SGLT2 inhibitors | o Avoidance with tenuous kidney function | (92) | Need further studies for use in EPTH |
o Potential for causing dehydration | |||
o Genitourinary infections | |||
o Weight loss | |||
o Blood pressure control | |||
o Increased risk of volume depletion and ketoacidosis perioperatively | |||
o Canagliflozin showed glycemic improvement at 6 months post transplant without significant adverse effects | |||
Thiazolidinediones | o Potential for fluid retention | Not favored | |
o Slow onset of action in improving hyperglycemia | |||
o Weight gain | |||
o Increased risk for heart failure |
Noninsulin agent . | Considerations for use in EPTH . | Reference studies . | Comments . |
---|---|---|---|
Metformin | o Avoid with tenuous renal function | Not favored in EPTH | |
o Gastrointestinal upset | |||
o Can rarely cause lactic acidosis in impaired renal function | |||
Sulfonylurea | o Require dose adjustment with renal function, which varies in the early posttransplant period | Not favored in EPTH | |
o High risk of hypoglycemia | |||
o Weight gain | |||
GLP-1 RA | o Potential risk of acute kidney injury with higher dose | (83–85) | Need further studies for use in EPTH |
o Cardiovascular benefit | |||
o Weight loss | |||
DPP-4 inhibitors | o Better HOMA-IR and HOMA-B compared to insulin | (86–91) | Favorable studies, need randomized controlled trials to compare glycemic control for EPTH |
o Linagliptin does not require renal dose adjustment | |||
SGLT2 inhibitors | o Avoidance with tenuous kidney function | (92) | Need further studies for use in EPTH |
o Potential for causing dehydration | |||
o Genitourinary infections | |||
o Weight loss | |||
o Blood pressure control | |||
o Increased risk of volume depletion and ketoacidosis perioperatively | |||
o Canagliflozin showed glycemic improvement at 6 months post transplant without significant adverse effects | |||
Thiazolidinediones | o Potential for fluid retention | Not favored | |
o Slow onset of action in improving hyperglycemia | |||
o Weight gain | |||
o Increased risk for heart failure |
Abbreviations: DPP-4, dipeptidyl peptidase-4; EPTH, early post–renal transplant hyperglycemia; GLP-1 RA, glucagon-like peptide-1 receptor agonist; HOMA-B, homeostatic model assessment of β-cell function; HOMA-IR, homeostatic model assessment of insulin resistance; SGLT2, sodium-glucose cotransporter-2.
Glucagon-like peptide-1 receptor agonists (GLP-1 RAs) may be a relatively safe and effective treatment for kidney transplant recipients. The benefit of GLP-1 RAs extends beyond glycemic control to weight loss and improved cardiovascular risk. Multiple recent trials have shown that therapy with GLP-1 RAs 3 to 5 years after transplant has no adverse effect on the kidney allograft outcomes, has a similar side effect profile to the general population, and has no significant effect on tacrolimus dosing (83-85). Importantly, these agents facilitated significant insulin dose reduction allowing for fewer hypoglycemic events.
In preclinical models, dipeptidyl peptidase-4 (DPP-4) inhibitors have demonstrated efficacy in preserving β-cell function under stress (86). Thus, the use of DPP-4 inhibitors could offset the possible calcineurin inhibitor–induced toxic effect on β cells and improve insulin resistance. Linagliptin is a DPP-4 inhibitor that does not have the side effects associated with insulin or metformin use and does not require dose adjustment with reduced renal function as is commonly seen in the early posttransplant setting (87). A small comparative study of 28 patients showed that the use of linagliptin plus insulin provided better glycemic control with a lower insulin dose and less severe hypoglycemia in comparison to insulin alone in patients with hyperglycemia immediately after renal transplantation (POD 0-POD 5) (88). Another study compared intensive surveillance and early commencement (as early as 2 days after transplant) of oral therapy with linagliptin to conventional treatment in a historical cohort of patients with posttransplant immunosuppression. Patients who were treated with linagliptin had better homeostatic model assessment of insulin resistance and homeostatic model assessment of β-cell function scores along with better blood glucose at every time point after a glucose load (89). Vildagliptin has been shown to improve β-cell function in PTDM (90), and sitagliptin has been shown to improve HbA1c in kidney transplant recipients (91).
The use of sodium-glucose cotransporter 2 (SGLT2) inhibitors in post–renal transplant patients is limited by the risk of renal graft dysfunction and urinary tract infections (UTIs). Canagliflozin was studied in patients who underwent renal transplant with prior history of diabetes or if they developed PTDM, and the results were favorable in causing reductions in body weight, blood pressure, and HbA1c and requirement of other hypoglycemic agents without any hypoglycemic episodes or significant adverse effects including UTIs and diabetic ketoacidosis (93). However, the initiation of canagliflozin in these patients was, on average, 0.2 to 13.2 years after transplantation and not exclusively during the early posttransplant period.
While insulin is an appropriate agent to control hyperglycemia in the early posttransplant period, the timing of initiation of agents other than insulin requires further investigation for safety and efficacy. Our case 1 patient had stable renal function 8 weeks after transplant and, since he had diabetes before the transplant, was aware of hypoglycemic symptoms to be alerted by. For this reason, he was transitioned to glimepiride, a sulfonylurea. Our patient in case 2 was transitioned to an oral agent (alogliptin, a DPP-4 inhibitor) about 6 weeks after transplant.
Lifestyle Modifications
Lifestyle modification including dietitian referral, exercise program, and weight loss benefits kidney transplant recipients with impaired glucose tolerance and should be implemented on discharge. Intensive lifestyle modifications and a diet rich in omega-3 and omega-9 fatty acids showed promising benefits in kidney transplant recipients (92). Exercise aimed at building muscle mass and reduction of fat, as opposed to weight loss, has been postulated to be beneficial in the renal transplant population (94).
Table 5 summarizes our recommendations for management of EPTH.
Recommendations for inpatient and discharge management of hyperglycemia after renal transplant
Inpatient | Diabetes education even before transplant with reinforcement of information afterward |
Medication reconciliation and ensuring insurance coverage is critical for successful discharge | |
Blood glucose target is 140-180 mg/dL while inpatient | |
o Lower target 70-110 mg/dL increases hypoglycemia without improving outcome | |
Therapy of choice is insulin | |
o Noninsulin therapy side effect profile can be significant | |
o Risk of unpredictable hypoglycemia with certain oral agents | |
o Basal/bolus recommended if total daily dose ≥ 0.25 units/kg | |
o Basal with correctional scale or oral for those < 0.25 units/kg/d or minimal nutrition | |
Early outpatient | BG target 80-130 mg/dL premeal and < 180 mg/dL postprandial |
o In patients with DM complications or high-risk hypoglycemia, can target premeal 110-140 mg/dL | |
For insulin therapy | |
o Quick follow-up with endocrinology post discharge for titration | |
o If glucocorticoids are being weaned, no specific titration of insulin proven to be more efficacious and is performed at discretion of physician | |
o If bolus requirements are low can consider noninsulin therapy to replace | |
For noninsulin therapy | |
o GLP-1 RA is safe and effective with benefits of weight loss and reduced cardiovascular risk | |
o DPP-4 inhibitor linagliptin, vildagliptin, and sitagliptin have all been studied and are safe and effective in reducing hyperglycemia | |
o SGLT2 inhibitors have been avoided out of concern for UTI and graft dysfunction | |
For lifestyle recommendations | |
o Weight loss should not be emphasized but rather improvement in muscle mass and reduction in fat mass |
Inpatient | Diabetes education even before transplant with reinforcement of information afterward |
Medication reconciliation and ensuring insurance coverage is critical for successful discharge | |
Blood glucose target is 140-180 mg/dL while inpatient | |
o Lower target 70-110 mg/dL increases hypoglycemia without improving outcome | |
Therapy of choice is insulin | |
o Noninsulin therapy side effect profile can be significant | |
o Risk of unpredictable hypoglycemia with certain oral agents | |
o Basal/bolus recommended if total daily dose ≥ 0.25 units/kg | |
o Basal with correctional scale or oral for those < 0.25 units/kg/d or minimal nutrition | |
Early outpatient | BG target 80-130 mg/dL premeal and < 180 mg/dL postprandial |
o In patients with DM complications or high-risk hypoglycemia, can target premeal 110-140 mg/dL | |
For insulin therapy | |
o Quick follow-up with endocrinology post discharge for titration | |
o If glucocorticoids are being weaned, no specific titration of insulin proven to be more efficacious and is performed at discretion of physician | |
o If bolus requirements are low can consider noninsulin therapy to replace | |
For noninsulin therapy | |
o GLP-1 RA is safe and effective with benefits of weight loss and reduced cardiovascular risk | |
o DPP-4 inhibitor linagliptin, vildagliptin, and sitagliptin have all been studied and are safe and effective in reducing hyperglycemia | |
o SGLT2 inhibitors have been avoided out of concern for UTI and graft dysfunction | |
For lifestyle recommendations | |
o Weight loss should not be emphasized but rather improvement in muscle mass and reduction in fat mass |
Abbreviations: DM, diabetes mellitus; DPP-4, dipeptidyl peptidase-4; GLP-1 RA, glucagon-like peptide-1 receptor agonist; SGLT2, sodium-glucose cotransporter-2; UTI, urinary tract infection.
Recommendations for inpatient and discharge management of hyperglycemia after renal transplant
Inpatient | Diabetes education even before transplant with reinforcement of information afterward |
Medication reconciliation and ensuring insurance coverage is critical for successful discharge | |
Blood glucose target is 140-180 mg/dL while inpatient | |
o Lower target 70-110 mg/dL increases hypoglycemia without improving outcome | |
Therapy of choice is insulin | |
o Noninsulin therapy side effect profile can be significant | |
o Risk of unpredictable hypoglycemia with certain oral agents | |
o Basal/bolus recommended if total daily dose ≥ 0.25 units/kg | |
o Basal with correctional scale or oral for those < 0.25 units/kg/d or minimal nutrition | |
Early outpatient | BG target 80-130 mg/dL premeal and < 180 mg/dL postprandial |
o In patients with DM complications or high-risk hypoglycemia, can target premeal 110-140 mg/dL | |
For insulin therapy | |
o Quick follow-up with endocrinology post discharge for titration | |
o If glucocorticoids are being weaned, no specific titration of insulin proven to be more efficacious and is performed at discretion of physician | |
o If bolus requirements are low can consider noninsulin therapy to replace | |
For noninsulin therapy | |
o GLP-1 RA is safe and effective with benefits of weight loss and reduced cardiovascular risk | |
o DPP-4 inhibitor linagliptin, vildagliptin, and sitagliptin have all been studied and are safe and effective in reducing hyperglycemia | |
o SGLT2 inhibitors have been avoided out of concern for UTI and graft dysfunction | |
For lifestyle recommendations | |
o Weight loss should not be emphasized but rather improvement in muscle mass and reduction in fat mass |
Inpatient | Diabetes education even before transplant with reinforcement of information afterward |
Medication reconciliation and ensuring insurance coverage is critical for successful discharge | |
Blood glucose target is 140-180 mg/dL while inpatient | |
o Lower target 70-110 mg/dL increases hypoglycemia without improving outcome | |
Therapy of choice is insulin | |
o Noninsulin therapy side effect profile can be significant | |
o Risk of unpredictable hypoglycemia with certain oral agents | |
o Basal/bolus recommended if total daily dose ≥ 0.25 units/kg | |
o Basal with correctional scale or oral for those < 0.25 units/kg/d or minimal nutrition | |
Early outpatient | BG target 80-130 mg/dL premeal and < 180 mg/dL postprandial |
o In patients with DM complications or high-risk hypoglycemia, can target premeal 110-140 mg/dL | |
For insulin therapy | |
o Quick follow-up with endocrinology post discharge for titration | |
o If glucocorticoids are being weaned, no specific titration of insulin proven to be more efficacious and is performed at discretion of physician | |
o If bolus requirements are low can consider noninsulin therapy to replace | |
For noninsulin therapy | |
o GLP-1 RA is safe and effective with benefits of weight loss and reduced cardiovascular risk | |
o DPP-4 inhibitor linagliptin, vildagliptin, and sitagliptin have all been studied and are safe and effective in reducing hyperglycemia | |
o SGLT2 inhibitors have been avoided out of concern for UTI and graft dysfunction | |
For lifestyle recommendations | |
o Weight loss should not be emphasized but rather improvement in muscle mass and reduction in fat mass |
Abbreviations: DM, diabetes mellitus; DPP-4, dipeptidyl peptidase-4; GLP-1 RA, glucagon-like peptide-1 receptor agonist; SGLT2, sodium-glucose cotransporter-2; UTI, urinary tract infection.
Conclusion
Patients with preexisting diabetes experience better glycemic control while in the ESRD state, with a resurgence of hyperglycemia after kidney transplantation. Renal insulin clearance decreases significantly during ESRD while renal transplant increases insulin clearance, paving the path for the resurgence of hyperglycemia. EPTH occurring within 45 days of renal transplant puts recipients at increased risk for rehospitalization due to infections such as CMV, acute graft rejection within 20 days of transplant, and increases the rate of return to dialysis, cardiovascular events, and PTDM. Patients with and without known diabetes before transplant can develop EPTH. It is important to identify risk factors for EPTH including elevated FG levels before transplant, preexisting diabetes, glucocorticoid use, surgical stress, postoperative pain, preexisting chronic infections such as chronic hepatitis C or CMV, and posttransplant infections. Screening patients without a known diagnosis of diabetes before renal transplant, using tools such as an oral glucose tolerance test, will help recognize patients with impaired FG and impaired glucose tolerance. Early recognition and management strategies include providing education to patients before discharge, frequent self-monitoring of BG after discharge, and close follow-up with an endocrinology provider and pharmacist. Current ADA targets are recommended, using a patient-centered approach, with a goal premeal BG of 80 to 130 mg/dL and postprandial blood glucose of less than 180 mg/dL. Insulin is the current standard for management; however, noninsulin agents like DPP-4 inhibitors (particularly linagliptin) have shown some promise in the early posttransplant setting. Other agents such as GLP-1 RAs and SGLT-2 inhibitors might also be effective, but studies are lacking. There is a need for further studies looking at safety profiles and the efficacy of injectable and oral noninsulin agents in the early post–renal transplant setting to decrease the risk of hypoglycemia associated with insulin and reduce long-term complications stemming from EPTH.
Abbreviations
- AACE
American Association of Clinical Endocrinology
- ADA
American Diabetes Association
- CMV
cytomegalovirus
- CNI
calcineurin inhibitor
- DPP-4
dipeptidyl peptidase-4
- EPTH
early post–renal transplant hyperglycemia
- ESRD
end-stage renal disease
- FG
fasting glucose
- GLP-1 RA
glucagon-like peptide-1 receptor agonist
- HbA1c
glycated hemoglobin A1c
- mTOR
mechanistic target of rapamycin
- NODAT
new-onset diabetes after transplant
- NPH
neutral protamine Hagedorn
- POD
postoperative day
- PTDM
posttransplant diabetes mellitus
- RG
random glucose
- SGLT2
sodium-glucose cotransporter-2
- UTI
urinary tract infection
Additional Information
Disclosures: A.I. has no relevant conflicts of interest to declare. M.C.L. is a consultant for the Sanofi group of companies. K.Z. has no relevant conflicts of interest to declare. S.K. serves as a consultant on the advisory board for Fractyl Inc and GI Dynamics.
Data Availability
Data sharing is not applicable to this article because no data sets were generated or analyzed during the present study.