-
PDF
- Split View
-
Views
-
Cite
Cite
Katrine M Lauritsen, Esben Søndergaard, Thien V Luong, Niels Møller, Lars C Gormsen, Acute Hyperketonemia Does Not Affect Glucose or Palmitate Uptake in Abdominal Organs or Skeletal Muscle, The Journal of Clinical Endocrinology & Metabolism, Volume 105, Issue 6, June 2020, Pages 1785–1790, https://doi.org/10.1210/clinem/dgaa122
- Share Icon Share
Abstract
It has recently been hypothesized that ketone bodies may have independent cardioprotective effects due to increased myocardial efficiency and that this may explain the improved survival of individuals with type 2 diabetes treated with mildly ketogenic sodium–glucose cotransporter-2 inhibitors.
To determine whether ketone bodies are selectively utilized in tissues critical for preservation of conscience and circulation. We investigated the effect of acute hyperketonemia on substrate metabolism in less prioritized tissues such as abdominal organs, adipose tissue, and skeletal muscle.
Acute, randomized, single-blinded, crossover design.
Ambulatory care.
Eight healthy participants completed the study. Two additional participants withdrew because of claustrophobia during the scans.
Infusions of saline and ketone bodies during a hyperinsulinemic-euglycemic clamp.
Organ-specific glucose and palmitate uptake was determined by dynamic positron emission tomography/computed tomography (PET/CT) scans with 18F-fluorodeoxyglucose (18F-FDG) and 11C-palmitate. Blood flow to abdominal organs was measured with O-15-labeled water (15O-H2O) perfusion PET. The study was performed as a post hoc analysis.
We found that ketone body infusion did not affect glucose uptake, palmitate uptake, or blood flow to abdominal organs and skeletal muscles.
Acute hyperketonemia does not affect glucose or palmitate uptake in skeletal muscle or abdominal tissues, supporting the notion that ketone bodies are selectively used by critical organs such as the heart and brain.
Ketone bodies are substrates for oxidation when carbohydrates are depleted or cannot be utilized (eg, during starvation or diabetic ketoacidosis). Most extrahepatic tissues have the enzymatic capacity to oxidize ketone bodies (1), but it is not fully understood to what extent this occurs during hyperketonemia. In our recent studies, we investigated the effect of an exogenous ketone body infusion on cardiac and brain metabolism and found that elevated levels of circulating ketone bodies reduce cardiac and brain glucose uptake (2, 3) indicating a shift from glucose toward ketone body oxidation. Whether ketone bodies also replace glucose as the preferred oxidative fuel in other tissues remains largely unknown. Sodium–glucose cotransporter-2 (SGLT-2) inhibition is an effective treatment for type 2 diabetes (4, 5) and is known to increase ketogenesis. SGLT-inhibitors also have remarkable cardioprotective effects (4, 5), but the mechanisms behind this remains unknown. Since ketone bodies are an energy-efficient substrate for oxidation with a lower oxygen consumption per generated adenosine triphosphate, increases in ketogenesis during treatment may be the underlying cause of the cardioprotective effects (the “thrifty substrate” hypothesis) (6, 7). Therefore, we aimed to determine the effect of an exogenous ketone body infusion on organ-specific substrate metabolism and perfusion in abdominal organs, adipose tissue, and skeletal muscle in healthy adults. We hypothesized that an acute increase in ketone bodies would not affect glucose or fatty acid uptake in abdominal organs, adipose tissue, or skeletal muscle. This would confirm our hypothesis that ketone bodies are selectively channeled toward the heart and the brain.
Materials and Methods
Study protocol
The study was performed as a single-blinded, randomized, crossover study in 8 healthy participants (see Table 1 for baseline characteristics). The study protocol is illustrated in Figure 1A and described in detail in a previous publication (2). In brief, participants underwent 2 study days after an overnight fast with ketone body infusion (0.18 g/kg/hour Na‐3‐β‐hydroxy‐butyrate) and saline (0.9% saline) in a random order. On both study days, a hyperinsulinemic-euglycemic (H-E) clamp was initiated at the beginning of the study day, with continuous infusion of insulin (0.3 mIE/kg/min [Humulin, Eli-Lilly, US]) and continuous adjustment of plasma glucose (200 g/L [Fresenius Kabi]) to ensure euglycemia (5 mmol/L).
Baseline Anthrometry and Biochemistry. Sex Is Expressed as Number of Participants and Continuous Variables as Mean (Standard Deviation)
Anthropometry . | |
---|---|
Sex (M/F) | 5/3 |
Age (years) | 59 (7) |
BMI (kg/m2) | 26.8 (3.9) |
Systolic BP (mm Hg) | 156 (21) |
Diastolic BP (mm Hg) | 98 (9) |
HbA1C (mmol/mmol) | 39 (3) |
eGFR (mL/min) | 76 (10) |
CRP (mg/liter) | 1.9 (1.3) |
Hb (mmol/liter) | 8.8 (0.7) |
Total cholesterol (mmol/liter) | 6.3 (0.9) |
HDL-cholesterol (mmol/liter) | 1.8 (0.6) |
LDL-cholesterol (mmol/liter) | 3.8 (1.1) |
Triglycerides (mmol/liter) | 1.5 (0.8) |
Anthropometry . | |
---|---|
Sex (M/F) | 5/3 |
Age (years) | 59 (7) |
BMI (kg/m2) | 26.8 (3.9) |
Systolic BP (mm Hg) | 156 (21) |
Diastolic BP (mm Hg) | 98 (9) |
HbA1C (mmol/mmol) | 39 (3) |
eGFR (mL/min) | 76 (10) |
CRP (mg/liter) | 1.9 (1.3) |
Hb (mmol/liter) | 8.8 (0.7) |
Total cholesterol (mmol/liter) | 6.3 (0.9) |
HDL-cholesterol (mmol/liter) | 1.8 (0.6) |
LDL-cholesterol (mmol/liter) | 3.8 (1.1) |
Triglycerides (mmol/liter) | 1.5 (0.8) |
Abbreviations: BMI, body mass index; BP, blood pressure; CRP, C-reactive protein; eGFR, estimated glomerular filtration rate; F, female; M, male; Hb, hemoglobin; HbA1c, hemoglobin A1c; HDL, high-density lipoprotein; LDL, low-density lipoprotein.
Baseline Anthrometry and Biochemistry. Sex Is Expressed as Number of Participants and Continuous Variables as Mean (Standard Deviation)
Anthropometry . | |
---|---|
Sex (M/F) | 5/3 |
Age (years) | 59 (7) |
BMI (kg/m2) | 26.8 (3.9) |
Systolic BP (mm Hg) | 156 (21) |
Diastolic BP (mm Hg) | 98 (9) |
HbA1C (mmol/mmol) | 39 (3) |
eGFR (mL/min) | 76 (10) |
CRP (mg/liter) | 1.9 (1.3) |
Hb (mmol/liter) | 8.8 (0.7) |
Total cholesterol (mmol/liter) | 6.3 (0.9) |
HDL-cholesterol (mmol/liter) | 1.8 (0.6) |
LDL-cholesterol (mmol/liter) | 3.8 (1.1) |
Triglycerides (mmol/liter) | 1.5 (0.8) |
Anthropometry . | |
---|---|
Sex (M/F) | 5/3 |
Age (years) | 59 (7) |
BMI (kg/m2) | 26.8 (3.9) |
Systolic BP (mm Hg) | 156 (21) |
Diastolic BP (mm Hg) | 98 (9) |
HbA1C (mmol/mmol) | 39 (3) |
eGFR (mL/min) | 76 (10) |
CRP (mg/liter) | 1.9 (1.3) |
Hb (mmol/liter) | 8.8 (0.7) |
Total cholesterol (mmol/liter) | 6.3 (0.9) |
HDL-cholesterol (mmol/liter) | 1.8 (0.6) |
LDL-cholesterol (mmol/liter) | 3.8 (1.1) |
Triglycerides (mmol/liter) | 1.5 (0.8) |
Abbreviations: BMI, body mass index; BP, blood pressure; CRP, C-reactive protein; eGFR, estimated glomerular filtration rate; F, female; M, male; Hb, hemoglobin; HbA1c, hemoglobin A1c; HDL, high-density lipoprotein; LDL, low-density lipoprotein.
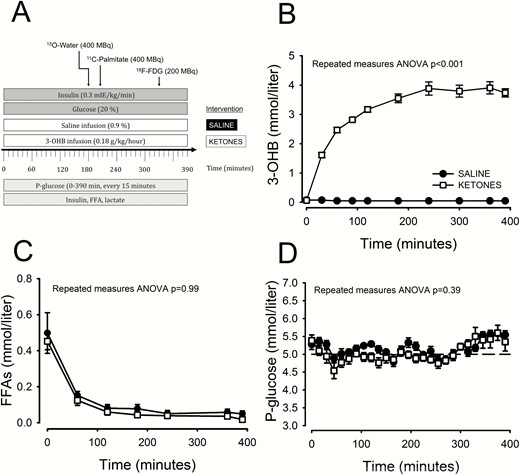
Study flowchart (A), 3-OHB (B), FFA (C), and glucose (D) concentration. ANOVA, analysis of variance; 3-OHB, β-hydroxybutyrate; 18F-FDG, 18F-fluorodeoxyglucose; FFA, free fatty acid; min, minutes.
All participants provided written informed consent prior to the study. The study protocol was approved by an independent ethics committee and was conducted according to the Declaration of Helsinki for medical research.
Blood samples
All blood samples excluding glucose were stored at −80 °C and analyzed in the same assay after all participants had completed both study days. Free fatty acid (FFA) was quantified using the in vitro enzymatic colorimetric method assay NEFA-HR (Wako Chemicals GmbH, Germany), glucose and lactate concentrations were measured using a YSI 2300 model STAT plus (YSI Incorporated, Netherlands), and insulin was measured by enzyme-linked immunosorbent assay. Serum concentrations of 3-β-hydroxybutyrate (3-OHB) were measured using hydrophilic interaction liquid chromatography–tandem mass spectrometry (8).
PET scan protocol
All PET procedures, image acquisition, and reconstruction parameters have been described in detail in our previous publication (2). In brief, PET/CT scans were conducted on a Siemens Biograph 64 PET/CT system (Siemens, Erlangen, Germany). Participants underwent 2 successive PET/CT scans with radiotracers injected into an antecubital vein. The 15O-H2O perfusion scan was initiated at a time of 180 minutes, the 11C-palmitate scan (50 minutes) at a time of 210 minutes, and the 18F-FDG scan (50 minutes) at a time of 330 minutes. Arterial samples were drawn during the 11C-palmitate scan in a radial artery. To obtain tissue time-activity curves, volumes of interest (VOIs) were drawn in the humeral flexor muscle (n = 8), erector spinae muscle (n = 8), subcutaneous fat (n = 8), visceral fat (n = 8), spleen (n = 8), kidney (n = 7, kidneys outside field of view in 1 participant), liver (n = 8), pancreas (n = 8), and jejunum (n = 8). For 11C-palmitate, the input function was measured in arterial samples, whereas the input function for 18F-FDG was obtained from a VOI drawn in the aorta. The best fit for calculating uptake was in each tissue evaluated using linear graphical analysis for 18F-FDG and 11C-palmitate. Hence, 18F-FDG tissue uptake (µmol/g/minute) was estimated with Patlak plots in the humeral flexor muscle and erector spinae muscle, whereas the 18F-FDG uptake in the subcutaneous fat, visceral fat, spleen, kidney, and liver was estimated as a volume of distribution (Vd) using a Logan plot. 11C-palmitate uptake was calculated with Patlak plots in all tissues. Absolute uptake rates were calculated as k-values multiplied by substrate concentrations for the Patlak plots. 18F-FDG uptake in muscle analyzed by Patlak plots was further adjusted using the lumped constant. The 15O-H2O perfusion PET data were analyzed using a simple 1-tissue compartmental model with an image-derived input function derived from a VOI drawn in the abdominal aorta (9).
Statistics
Normal distribution was ensured by inspection of quantile-quantile (QQ) plots. Results are presented as mean ± standard deviation. Comparison between 2 two study days was made with a paired t test with a statistical significance level set at P < 0.05.
Data availability
The datasets generated during and/or analyzed during the current study are available from the corresponding author on reasonable request.
Results
Hormones and metabolites
Ketone body infusion led to a rapid increase in 3-OHB, reaching a mean [SD] plateau after 300 minutes at 3.8 [0.5] mmol/L. Throughout the saline infusion day, ketone body levels were below detection limit (Fig. 1B). FFA levels were suppressed to a similar extent on the ketone and saline study days (Fig. 1C). Plasma glucose levels were stable and similar on the ketone study day compared with the saline study day (Fig. 1D). Insulin level increases were similar during the H-E clamp on the ketone study day compared with the saline study day (178 ± 63 vs 176 ± 45 pmol/L, P = 0.89).
15O-H2O perfusion in kidney, pancreas, jejunum, and spleen
Perfusion in the kidney (198 ± 41 vs 174 ± 44 mL/100 g tissue/minute; P = 0.32), pancreas (161 ± 50 vs 155 ± 34 mL/100 g tissue/minute; P = 0.64), jejunum (51 ± 19 vs 52 ± 29 mL/100 g tissue/minute; P = 0.94), and spleen (235 ± 52 vs 192 ± 76 mL/100 g tissue/minute; P = 0.19) was similar during ketone body and saline infusion, respectively (Fig. 2C).
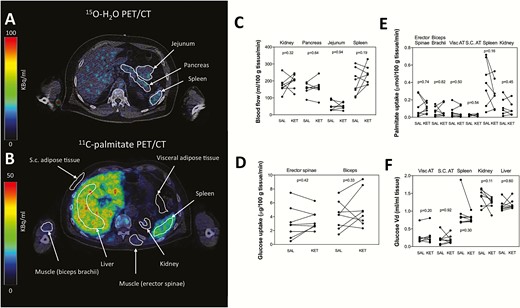
Substrate uptake and perfusion. Volumes of interest (VOIs) in the kidney, pancreas, jejunum, and spleen for detection of perfusion measured with 15O-H2O PET/CT (A). VOIs in the humeral flexor muscle, erector spinae muscle, subcutaneous fat, visceral fat, spleen, kidney, and liver for estimation of palmitate and FDG uptake (B). Perfusion, 18F-FDG uptake and palmitate uptake was unchanged during ketone body infusion in all tissues (C, D, E, F). Liver data not shown in figure E due to a several fold higher palmitate uptake compared with other tissues. 18F-FDG, 18F-fluorodeoxyglucose; KET, ketone; min, minutes; PET/CT, positron emission tomography/computed tomography; SAL, saline; S.C., subcutaneous; Vd, volume of distribution; visc, visceral.
18F-FDG uptake in skeletal muscle, kidney, spleen, liver, subcutaneous and visceral fat
Skeletal muscle glucose uptake was unaffected by the ketone body infusion compared with saline infusion both in erector spinae muscle (3.5 ± 1.4 vs 3.1 ± 2.3 µmol/100 g/minute; P = 0.42) and humeral flexor muscle (5.1 ± 2.6 vs 4.2 ± 2.0 µmol/100 g tissue/minute; P = 0.33), respectively (Fig. 2D). Likewise, glucose Vd was not affected by the ketone body infusion compared with saline infusion in visceral fat (0.3 ± 0.2 vs 0.3 ± 0.2 mL plasma/mL tissue; P = 0.21), subcutaneous fat (0.2 ± 0.1 vs 0.2 ± 0.2 mL plasma/mL tissue; P = 0.92), spleen (0.8 ± 0.1 vs 0.9 ± 0.4 mL plasma/mL tissue; P = 0.30), kidney (1.2 ± 0.2 vs 1.4 ± 0.2 mL plasma/mL tissue; P = 0.11) and liver (1.2 ± 0.1 vs 1.2 ± 0.1 mL plasma/mL tissue; P = 0.60) respectively (Fig. 2E).
11C-palmitate uptake in skeletal muscle, kidney, spleen, liver, visceral and subcutaneous fat
Absolute palmitate uptake rates were similar during ketone body infusion compared with saline infusion in the erector spinae muscle (0.11 ± 0.06 vs 0.12 ± 0.12 µmol/100 g/minute; P = 0.74), humeral flexor muscle (0.07 ± 0.07 vs 0.07 ± 0.06 µmol/100 g/minute; P = 0.82), visceral fat (0.06 ± 0.06 vs 0.06 ± 0.07 µmol/100 g/minute; P = 0.50), subcutaneous fat (0.02 ± 0.01 vs 0.03 ± 0.01 µmol/100 g/minute, P = 0.54), spleen (0.27 ± 0.17 vs 0.40 ± 0.26 µmol/100 g/minute; P = 0.16), kidneys (0.10 ± 0.08 vs 0.12 ± 0.10 µmol/100 g/minute; P = 0.45), and liver (0.67 ± 0.53 vs 0.97 ± 0.83 µmol/100 g/minute; P = 0.16), respectively (Fig. 2E).
Discussion
In the present study, we determined the effect of acute hyperketonemia on organ-specific glucose and fatty acid uptake in abdominal organs, adipose tissue, and skeletal muscle as well as blood flow in the kidney, pancreas, jejunum, and spleen. As hypothesized, we found that hyperketonemia does not affect glucose or palmitate uptake in the skeletal muscle, kidney, spleen, liver, or subcutaneous or visceral fat. This contrasts the strikingly suppressive effect of ketones on myocardial and cerebral glucose uptake and supports the notion that ketone bodies are selectively channeled toward tissues critical for cognition and preservation of the circulation.
Physiological ketonemia is present under energy-restricted conditions and during ketogenic dieting, where ketone bodies serve as an adjunct substrate for oxidation (1). This is most marked in the brain, where FFAs cannot cross the blood–brain barrier, leaving ketone bodies as the only quantitatively important alternative to glucose (10). However, since oxidation of ketone bodies requires less oxygen than FFAs to generate a similar amount of energy, ketone bodies may also function as an oxygen-sparing substrate for the heart. This has been suggested as an explanation for the cardioprotective effect of SGLT-2 inhibitors, which on average doubles the levels of circulating ketone bodies (7, 11, 12). In this study, the level of ketone bodies was markedly higher compared with what is typically observed during SGLT-2 inhibition or modified very low-carbohydrate diets, in fact comparable to levels observed during prolonged fasting (>48 hours) or strict ketogenic dieting. The metabolic milieu observed in the present study was, therefore, not directly comparable to conditions observed in patients treated with SGLT2 inhibitors. However, we find it unlikely that there should be an effect of ketone bodies at levels between 300 and 600 micromol/liter when none is observed at 3 to 4 mmol/liter. We still believe that our negative results in the high physiological range can be extrapolated into the range observed during SGLT2 inhibition.
In addition to the cardioprotective effect, SGLT-2 inhibition has been associated with delayed progression of kidney disease in type 2 diabetes (5, 13). Again, it has been hypothesized that this is due to a shift toward energy-efficient ketone body oxidation (11). Limited data exist on the effect of hyperketonemia on kidney substrate metabolism, but an older study of 3-OHB infusions in dogs showed renal uptake of ketone bodies in preference for lactate, which may indicate that ketone bodies are a substrate for oxidation in the kidneys (14). In the present study, we did not observe any effect of hyperketonemia on kidney glucose or palmitate uptake, implying that ketone bodies may not be an important oxidative substrate in the kidneys. However, although not statistically significant, we did observe a decrease (P = 0.11) in renal Vd of glucose during ketone body infusion. Since the study included only a limited number of participants, we cannot rule out a type 2 error.
Whether ketone bodies are a quantitatively important source of fuel for skeletal muscle is debated, and experimental data point in different directions. Oral ingestion of ketone bodies has thus recently been demonstrated to improve exercise performance in athletes (15), and infusion of ketone bodies has been shown to reduce glucose uptake in skeletal muscle (16). However, several other studies including this have failed to detect any effects of hyperketonemia on skeletal muscle glucose uptake (17, 18). Nevertheless, it is still possible that ketone bodies may become an important source of energy in skeletal muscle during exercise when energy demands are high and oxygen demand may outstrip supply.
Hyperketonemia affects blood flow in several organs with an increase of 75%, 30%, and 15% in myocardial (2), cerebral (3), and forearm blood flow (17), respectively. In addition, hyperketonemia increases cardiac output by 40% (19). The observed increase in blood flow may arise from a direct vasodilatory effect or may be secondary to the increase in cardiac output. To determine how hyperketonemia also affects blood flow in abdominal organs, we measured blood flow in the kidney, pancreas, jejunum, or spleen and observed no effect of hyperketonemia. This indicates that besides increasing cardiac output, ketone bodies shift blood flow toward critical organs without impairing the blood flow to abdominal organs.
Several limitations to the study need to be acknowledged, most of which are related to the experimental setup involving an H-E clamp. Ketone bodies are potent inhibitors of the hormone-sensitive lipase, and thus lipolysis (20) and experimentally induced hyperketonemia without H-E clamping results not only in reduced fatty acid levels but also in decreased blood glucose levels (21). The H-E clamp was chosen to ensure comparable levels of these metabolites and hormones on both study days, However, since fatty acid levels were suppressed by insulin to near the detection level, our ability to detect alterations in absolute palmitate uptake was severely hampered. Second, it can be argued that concomitantly increased insulin and ketone body levels do not reflect any physiological conditions. Third, tissue ketone body uptake was not directly measured using a suitable PET tracer such as 11C-hydroxybutyrate but was inferred from tissue uptake of the other major sources of fuel, glucose, and lipids. Finally, this is a post hoc analysis, and our sample size was determined based on cardiac glucose uptake.
In conclusion, acute hyperketonemia does not affect glucose or palmitate uptake in abdominal organs or skeletal muscle during resting conditions. Ketone bodies, therefore, appear to be selectively channeled toward the heart and the brain.
Abbreviations
- 3-OHB
3-b-hydroxybutyrate
- 15O-H2O
O-15-labeled water
- 18F-FDG
18F-fluorodeoxyglucose
- H-E
hyperinsulinemic-euglycemic
- FFA
free fatty acid
- PET/CT
positron emission tomography/computed tomography
- SGLT
sodium–glucose cotransporter-2
- Vd
volume of distribution
- VOIs
volumes of interest
Acknowledgments
Financial Support: The study was supported by grants from the Danish Council for Strategic Research, the Danish Council for Independent Research | Medical Sciences, Aase Danielsen Fund, Ferd og Ellen Hindsgavls Fund, the Danish Medical Association, the Novo Nordisk Foundation, the Danish Diabetes Association, the Danish Diabetes Academy supported by the Novo Nordisk Foundation, the Health Research Fund of Central Denmark Region, and the A.P. Møller Foundation.
Author Contributions: N.M. and L.C.G. conceptualized the study and wrote the protocol. K.M.L., E.S., T.V.L., and L.C.G. researched the data. K.M.L., E.S., T.V.L., N.M, and L.C.G contributed to the discussion. K.M.L. wrote the manuscript, and E.S., T.V.L., N.M., and L.C.G. reviewed and edited the manuscript. N.M. and L.C.G. are the guarantors of this work and, as such, had full access to all the data in the study and take responsibility for the integrity of the data and the accuracy of the data analysis.
Clinical Trial Information: ClinicalTrials.gov Identifier: NCT02814474 (registered June 27, 2016).
Additional Information
Disclosure Summary: The authors have nothing to disclose.
Data Availability: The datasets generated during and/or analyzed during the current study are not publicly available but are available from the corresponding author on reasonable request.
References