-
PDF
- Split View
-
Views
-
Cite
Cite
Qiu Du, Bin Hu, Yajuan Feng, Zongming Wang, Xin Wang, Dimin Zhu, Yonghong Zhu, Xiaobing Jiang, Haijun Wang, circOMA1-Mediated miR-145-5p Suppresses Tumor Growth of Nonfunctioning Pituitary Adenomas by Targeting TPT1, The Journal of Clinical Endocrinology & Metabolism, Volume 104, Issue 6, June 2019, Pages 2419–2434, https://doi.org/10.1210/jc.2018-01851
- Share Icon Share
Abstract
Nonfunctioning pituitary adenomas (NFPAs) are the major cause of hypopituitarism and infertility. However, the pathogenesis of NFPAs remains largely unknown. Previous studies have demonstrated the crucial role of miRNAs in the progression of pituitary adenomas. Increasing evidence has indicated that circular RNAs (circRNAs) might mediate miRNA transcriptional activity, providing new insights to study the pathogenesis of NFPAs.
To explore the regulation and activity of the circRNA–miRNA–mRNA axis in the tumorigenesis of NFPAs.
The function of miR-145-5p in NFPAs was investigated invitro and invivo. The mechanical details were explored and potential targets of miR-145-5p were identified. Finally, miR-145-5p–associated circRNAs were functionally recognized and confirmed.
miR-145-5p was markedly decreased in NFPA samples and correlated negatively with NFPA invasiveness. Overexpression of miR-145-5p suppressed NFPA cell proliferation and invasiveness and promoted apoptosis. Further results confirmed that translationally controlled tumor protein (TPT1) is a target of miR-145-5p and mediated the effect of miR-145-5p. TPT1 and its downstream factors Mcl-1 and Bcl-xL were downregulated, and Bax was upregulated by miR-145-5p. Moreover, circOMA1 (hsa_circRNA_0002316) was demonstrated to sponge miR-145-5p, whose suppression on NFPA cells was abrogated by circOMA1 overexpression. circOMA1 silencing exhibited a similar inhibitory effect with miR-145-5p overexpression by downregulating TPT1. We found that circOMA1 could further upregulate Mcl-1 and Bcl-xL and downregulate Bax.
circOMA1 promotes NFPA progression by acting as the sponge of tumor suppressor miR-145-5p to regulate the TPT1 signaling pathway, revealing a therapeutic target in preventing the tumorigenesis of NFPAs.
Pituitary adenomas are among the most common intracranial neoplasms. Approximately 30% of these cases will be clinical nonfunctioning pituitary adenomas (NFPAs), which are characterized by a lack of hormonal secretion (1, 2). Generally, patients with NFPAs will present with symptoms of mass effect, including headache, visual field defect, loss of libido, and hydrocephalus (3, 4). Moreover, NFPAs are the most frequent cause of hypopituitarism, which has been proved to be associated with an overall excess mortality compared with the general population (5). Currently, surgical resection is the major treatment of NFPAs. However, owing to the lack of hormone-related symptoms, a large proportion of NFPAs will have invasive growth at diagnosis, which is a neurosurgical challenge, and are also prone to relapse postoperatively, despite advancements in endoscopic techniques (6). Systemic medical treatment could be a potentially effective alternative for the management of invasive NFPAs. Also, the early detection of pituitary tumors could further help in preventing the development of invasive NFPAs. However, these strategies have been hampered by the limited understanding of the mechanisms underlying pituitary tumorigenesis. Therefore, exploring the pathogenesis of pituitary adenomas is clinically important and will help contribute to identifying novel therapeutic targets for pituitary adenomas.
Noncoding RNAs, including miRNAs and long noncoding RNAs, have long been known to be involved in the development of NFPAs (7). Using microarray analysis, we previously identified differentially expressed miRNAs between pituitary adenomas and normal pituitary samples (8). Among them, miR-145-5p was found to be of particular interest, because it was shown to suppress tumorigenesis in several malignant tumors (9–12). Furthermore, miR-145-5p is downregulated in GH-secreting adenomas and ACTH-secreting pituitary adenomas (8) and has been associated with the invasion of primary pituitary tumor cells (13). Therefore, the function and mechanism of miR-145-5p in NFPAs deserve further exploration.
Circular RNAs (circRNAs) represent a new member of the noncoding RNA family that has been the subject of intense interest during the past few years. Unlike linear RNAs, circRNAs show a covalently closed loop structure that lack 5′-3′ polarity or a polyadenylated tail (14). Moreover, circRNAs are more stable than linear RNAs because of their resistance to RNase R (14). In addition, circRNAs show high abundance and stability and exhibit multiple potential functions, usually acting as competing endogenous RNAs for miRNAs. Many circRNAs are involved in the pathogenesis of various diseases by acting as the sponges of miRNAs (15, 16). The mechanistic connections between circRNAs and miRNAs represent new types of mechanism and potential therapeutic targets for tumors. Several studies have shown that circRNAs are expressed in cell- and tissue-specific methods (17). Thus, elucidating the function of circRNAs in a specific organ and pathogenesis would be valuable.
A recent study found differentially expressed circRNAs among invasive and noninvasive NFPAs (18), which implicated the potential regulation of circRNAs on miRNAs in NFPA development. Thus, we explored whether circRNAs are involved in the regulation of miRNA-145-5p activity in NFPAs. In the present study, we found that miR-145-5p suppressed cell proliferation and invasion of NFPAs in vitro and in vivo by targeting translationally controlled tumor protein (TPT1). Furthermore, we demonstrated that circOMA1 (hsa_circRNA_0002316) acted as the sponge of miR-145-5p and subsequently upregulated the expression of TPT1. The regulation of circRNAs on miRNAs represents a mechanism in the pathogenesis of NFPAs and might serve as a potential therapeutic target for the treatment of NFPAs.
Materials and Methods
Human tissue samples
A total of 50 human NFPAs specimens were obtained from the Department of Neurosurgery and Pituitary Tumor Center of the First Affiliated Hospital, Sun Yat-sen University, from July 2015 to January 2017. The clinicopathological information of the patients has been provided in an online repository (19). Each tumor was evaluated and diagnosed histopathologically by the pathologists. Invasive pituitary adenoma (Knosp grade 3 and 4) was defined as the presence of cavernous sinus, suprasellar, or infrasellar invasion. Samples from 15 human normal pituitary (NP) tissues were obtained from autopsy material (donated from individuals who had died in a traffic accident) in the Forensic Authentication Center, Sun Yat-sen University, where each sample was confirmed to be free of any previous pathologically detectable conditions by an experienced pathologist. Consent, including a tissue sharing clause, was obtained from a family member of the deceased individual before the autopsy. To rule out RNA degradation, the NP RNA quantification and quality were confirmed by NanoDrop ND-2000 (Thermo Fisher Scientific, Waltham, MA) before use, and RNA integrity was tested by denaturing agarose gel electrophoresis (19).
The NFPA samples were collected with the consent of the patients, and the medical ethics committee of the First Affiliated Hospital, Sun Yat-sen University (Guangzhou, China) approved the experiments for research purposes.
Cell culture
A pituitary tumor derived folliculostellate (PDFS) cell line was provided by the Neuroendocrine Unit, Massachusetts General Hospital, Harvard Medical School (20). Primary tumor cells from NFPA tissues were isolated and cultured, as described previously (21). We obtained short-term culture invasive NFPA-derived cells (primary cells), which were cultured for ~10 passages. All the experiments were performed at the early stage of these cells, when they were in active status. Both PDFS and primary cells were cultured in DMEM/high glucose supplemented with 10% fetal bovine serum, 100 U/mL penicillin, and 100 μg/mL streptomycin. Human embryonic kidney (HEK) 293T was purchased from the Shanghai Institutes for Biological Sciences Cell Resource Center. HEK-293T cells were maintained in DMEM containing 10% fetal bovine serum, 1× nonessential amino acid (Gibco, Grand Island, NY), 1 mM sodium pyruvate (Gibco), and 1× GlutaMAX (Gibco). All cell lines were incubated in a humidified atmosphere of 5% carbon dioxide in air at 37°C.
Reverse transcription and quantitative real-time PCR
Total RNA was extracted from tissue samples or cells using the TRIzol reagent (Life Technologies, Carlsbad, CA) according to the manufacturer’s protocol. RNA samples were quantified using NanoDrop ND-2000 (Thermo Fisher Scientific) with 260/280 ratios of 1.8 to 2.0. For miRNA expression analysis, 1 μg total RNA was reverse transcribed using the Mir-X™ miRNA First Strand Synthesis Kit (Takara, Dalian, China), and quantitative reverse transcription (qRT)-PCR was performed using SYBR Premix Ex TaqII (Takara), according to the manufacturer’s instructions, on the Bio-Rad CFX96 system (Hercules, CA). U6 small nuclear RNA was used as the endogenous control. The expression levels of miRNAs were determined using the 2−ΔΔCt method.
To evaluate mRNA and circRNA expression, RNA was reverse transcribed using the Prime Script RT Master Mix Kit (Takara), and qRT-PCR was performed using SYBR Premix Ex TaqII on the Real-Time PCR System (Bio-Rad). The results were normalized to β-actin mRNA, as the internal control, using the 2−ΔΔCt method. The primers were ordered from Sangon (Shanghai, China). The primer sequences are provided in an online repository (19).
Construction of circOMA1 and TPT1 overexpression plasmids
The circOMA1 spliced form sequence was amplified by PCR (Toyobo, Osaka, Japan) using circOMA1 primers. The PCR-amplified fragment was inserted into BamH I and EcoR I sites in pcircRNA 2.2-hsa (Bersinbio, Guangzhou, China) to generate the plasmid (p)-circOMA1 vector. The DNA coding sequence of TPT1 was also amplified (Toyobo) and subcloned into BamH I and EcoR I sites in the pCDNA3.1(+) vector (Invitrogen, Carlsbad, CA) to construct pcDNA3.1-TPT1. Primer sequences are provided in an online repository (19).
Cell transfection
circOMA1 small interfering RNA (siRNA; 5′-ACCUCAGGUAGUCAAGUGA TT-3′), miR-145-5p mimic (5′-GTCCAGTTTTCCCAGGAATCCCT-3′), inhibitor (5′-ACGGAUUCCUGGGAAAA CUGGAC-3′), agomiR-145-5p, TPT1 siRNA (5′-AAGGTACCGAAAGCACAGT TT-3′) and the corresponding negative controls were designed and synthesized by RiboBio (Guangzhou, China). To confirm the relationship between miR-145 and TPT1, TPT1 knockdown and overexpression experiments were performed. For TPT1 silencing, PDFS cells were cotransfected with miR-145-5p mimic or inhibitor and TPT1 siRNA; cells cotransfected miR-145-5p mimic/inhibitor and siRNA-NC were used as the control. For TPT1 overexpression, PDFS cells were cotransfected with miR-145-5p mimic and pcDNA3.1-TPT1, miR-145-5p mimic, and pcDNA3.1-B were cotransfected as the control. All the transfection experiments were performed using Lipofectamine 3000 (Invitrogen), following the manufacturer’s instructions. Overexpression and silencing efficiency were analyzed using qRT-PCR. The proliferation and viability of cells were also evaluated.
Cell proliferation and colony formation assay
Cell proliferation analysis was performed with the Cell Counting Kit-8 (CCK-8; Dojindo Laboratories, Kumamoto, Japan), according to the manufacturer’s instructions. A total of 4 × 103 cells were cultured in each well of 96-well plates for 24, 48, 72, and 96 hours. Next, 10 μL of CCK-8 was added to each well, and the cells were incubated at 37°C for 2 hours. Absorbance was measured at 450 nm using a Sunrise microplate reader (Tecan, Männedorf, Switzerland).
For colony formation assays, the cells were seeded into each well of six-well plates (Corning Life Sciences, Corning, NY) and incubated for 10 to 14 days. Next, the colonies were fixed with 4% paraformaldehyde (Sigma-Aldrich, St. Louis, MO) for 10 minutes and then stained with 0.1% crystal violet (Sigma-Aldrich) for 20 minutes. The colonies were photographed and counted.
Cell apoptosis assay by flow cytometry
Cell apoptosis was quantified using the Annexin V-FITC/PI Apoptosis Detection Kit (KeyGEN, Nanjing, China). After rinsing with PBS and centrifuging twice, the cells were resuspended in binding buffer and stained with Annexin V-FITC/PI according to the manufacturer’s instructions. The cells were then analyzed using a FACS flow cytometer (Bechman Coulter, Inc. Brea, CA) to acquire the apoptotic fractions.
Cell migration and invasion assay
Transwell chamber 24-well plate (Corning Life Sciences) and Matrigel Invasion Chambers (Corning Life Sciences) with 8-μm pore size membranes were used to measure the migration and invasion ability of the cells, respectively. PDFS cells were transfected for 24 hours, and then the cells were seeded with serum-free medium (2 × 104 cells in 200 μL) into the upper chamber. The bottom wells were filled with complete medium. After incubation at 37°C for 48 hours, the cells remaining on the upper surface of the membranes were carefully removed with a cotton swab, and the cells that had moved through the membrane were fixed with 4% paraformaldehyde and stained with 0.1% crystal violet. The cells were counted by taking photomicrographs in five randomly chosen fields.
Reporter vector constructs and luciferase reporter assay
The full-length of TPT1 3′ untranslated region (UTR) fragment and circOMA1 spliced sequence were amplified by PCR (Toyobo) and cloned into a psiCheck2 vector (OBiO, Shanghai, China) to construct luciferase reporter vector (psiCheck2-TPT1 3′UTR-WT and psiCheck2 -circOMA1-WT, respectively). The sequences of putative miR-145-5p binding sites were replaced (psiCheck2-TPT1 3′UTR-MU: for TPT1 3′UTR, the mutation sequences were 5′-GACCCGTAACGG-3′ and 5′-CGTAACGC-3′; psiCheck2-circOMA1-MU: for circOMA1, the mutation sequences were 5′-CGUAACG-3′ and 5′-CGCGTAACGT-3′) to mutate the putative binding seed regions of TPT1 3′UTR and circOMA1 spliced sequences.
HEK-293T cells were seeded in 96-well plates and cotransfected with luciferase reporter plasmid (50 ng/well) and miR-145-5p mimics or miR-NC mimics (50 nmol/L) when they reached 50% to 70% confluence using Lipofectamine 3000 (Invitrogen). The luciferase activity was measured at 24 hours after transfection using the Dual-Luciferase Reporter Assay System (Promega, Madison, WI). Firefly luciferase activity was normalized against Renilla luciferase activity for each transfected well.
Fluorescence in situ hybridization
For identification of circOMA1 and miR-145-5p localization in NFPA cells, a circOMA1 probe (5′-TCATTTTTTCACTTGACTACCTGAGGTATAAGTCTATCCA-3′, red-labeled; Bersinbio, Guangzhou, China) and miR-145-5p probe (5′-TCTTTGGTTATCTAGCTGTATGA-3′, yellow-labeled; Bersinbio) were used. In brief, the slides were treated with proteinase K (CWBio, Beijing, China) after fixed with 4% paraformaldehyde and 0.1% diethyl pyrocarbonic). Next, the slides were washed and incubated with prehybridization solution. The hybridization mix was prepared with the circOMA1 probe and miR-145-5p probe in the hybridization solution, added to the slide, and hybridized overnight at 42°C. After washing with washing buffer, blocking was performed. Next, the antidigoxin rhodamine conjugate was added to the sections at 37°C for 1 hour in the humidified chamber. Subsequently, the sections were counterstained with 4′,6-diamidino-2-phenylindole (Beyotime, Shanghai, China) for nuclear staining. Fluorescence images were captured using an inverted fluorescence microscope (Nikon, Tokyo, Japan).
Xenograft tumor analysis
All animal studies were performed in the Laboratory Animal Center of Sun Yat-sen University and were performed in accordance with the Guide for Medical Experimental Animal Care and Use of Laboratory Animals.
BALB/c nude mice (female, aged 4 to 5 weeks) were purchased from the Animal Center of Sun Yat-sen University. A total of 1 × 107 transfected PDFS cells resuspended in 100 μL serum-free DMEM was injected subcutaneously into the right hind limb of the nude mice. Each group (PDFS cells with overexpression of agomiR-NC, agomiR-145-5p, p-circ_B, or p-circ_OMA1) contained five nude mice. The tumor volume (V) was evaluated by measuring the tumor length (L) and width (W) using a vernier caliper and calculated using the formula: V = 0.5 × L × W2. After 27 days, the mice were killed and the tumor tissues harvested.
Western blot
Total protein was extracted using radioimmunoprecipitation assay buffer (Beyotime) with 1× phenylmethylsulfonyl fluoride (CWBio), and the protein concentration was measured using a BCA assay kit (CWBio). The proteins were separated using sodium dodecyl sulfate-polyacrylamide gel electrophoresis and then transferred onto a polyvinylidene fluoride membrane (Millipore Corp., Billerica, MA) at 300 mA for 2 hours. After the membrane was blocked with 5% fat-free milk (Sigma-Aldrich) in Tris-buffered saline plus Tween 20 for 1 hour at room temperature, the membranes were incubated at 4°C overnight with the primary antibodies: Mcl-1 (1:1000), Bcl-xL (1:1000), Bax (1:1000), TPT1 (1:1000), β-actin (1:2000; all from Abcam, Cambridge, MA) and glyceraldehyde 3-phosphate dehydrogenase (1:2000; Invitrogen). After washing with Tris-buffered saline plus Tween 20 for three times, the membranes were incubated with goat anti-rabbit or anti-mouse horseradish peroxidase-conjugated secondary antibody (CWBio) for 1 hour at room temperature. Signal detection was performed using the ultrasensitive ECL plus Detection Reagent (Millipore Corp.) and a ChemiDoc Touch Imaging System (Bio-Rad).
Immunohistochemistry
Immunohistochemistry was performed, as described previously (13, 22). The tissues were incubated with anti-TPT1 primary antibodies (1:100; Abcam) at 4°C overnight. Tissues stained with hematoxylin and diaminobenzidine were used for the blank control. The expression of TPT1 was evaluated using the H-score system. In brief, the staining of TPT1 was assigned a mean score by multiplying the intensity (score, 0 to 3) and the proportion of positive cells (score, 0 to 4). Scores of 0 to 7 were considered to indicate low expression and scores of 8 to 12, high expression.
Statistical analysis
All the experiments were performed at least in technical triplicate and in three independent biological repeats. Numerical data are presented as the mean ± SD. The Student t test was used to determine the statistically significant differences between the two groups. Associations between circOMA1 and miR-145-5p, miR-145-5p, and TPT1 were determined using Pearson analysis. Statistical analysis was performed using SPSS, version 16.0, statistical software (IBM Corp., Armonk, NY). Values were considered statistically significant at P < 0.05.
Results
MiR-145-5p expression is decreased in NFPA specimens and correlated with tumor invasiveness
We found that the level of miR-145-5p was markedly downregulated in PDFS and NFPA primary cells compared with NP tissues (P < 0.001; Fig. 1A). The expression of miR-145-5p was then assessed in 50 NFPA samples, including 28 invasive NFPAs and 22 noninvasive NFPAs, and 15 NP tissues. As shown in Fig. 1B, miR-145-5p was significantly decreased in NFPA tissues compared with NP tissues (P < 0.001). Also, low expression of miR-145-5p was demonstrated in almost all the NFPA samples (P < 0.001; Fig. 1C). Furthermore, much greater downregulated expression of miR-145-5p was noted in the invasive tumor samples compared with the noninvasive tumor samples (P < 0.001; Fig. 1B), which suggested that downregulation of miR-145-5p might be associated with the progression and invasiveness of NFPA.
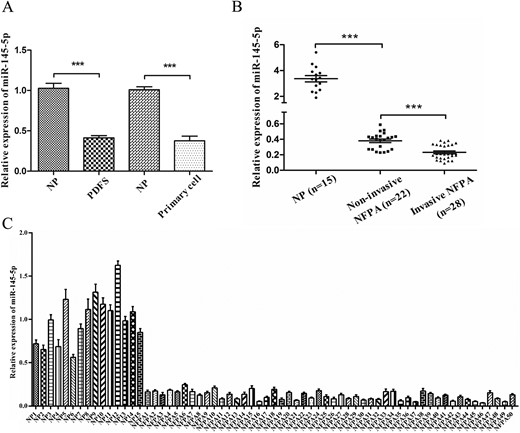
Expression of miR-145-5p in human NFPA specimens, PDFS cells, and NFPA primary cells. (A) miR-145-5p expression in PDFS and primary cells, both of which were substantially reduced. (B) Expression of miR-145-5p was significantly downregulated in human NFPA specimens. (C) The relative expression of miR-145-5p in each NFPA (n = 50) and NP tissues (n = 15) was analyzed using qRT-PCR. Values from NP were designated as 1, against which the values from pituitary tumors were normalized. ***P < 0.001.
MiR-145-5p suppresses proliferation and invasiveness of NFPAs cells in vitro
To investigate the function of miR-145-5p in the pathogenesis of NFPAs, we transfected miR-145-5p mimic and inhibitor into PDFS and NFPA primary cells. First, the level of miR-145-5p in PDFS and NFPA primary cells was overexpressed or attenuated and was confirmed with qRT-PCR (P < 0.001; Fig. 2A). PDFS cell colony formation ability was significantly reduced by overexpression of miR-145-5p and increased by silencing of miR-145-5p (P < 0.01; Fig. 2B). CCK-8 assays also showed that the proliferation of PDFS and primary cells was suppressed by overexpression of miR-145-5p and increased by silencing of miR-145-5p (Fig. 2C; P < 0.05 for all).
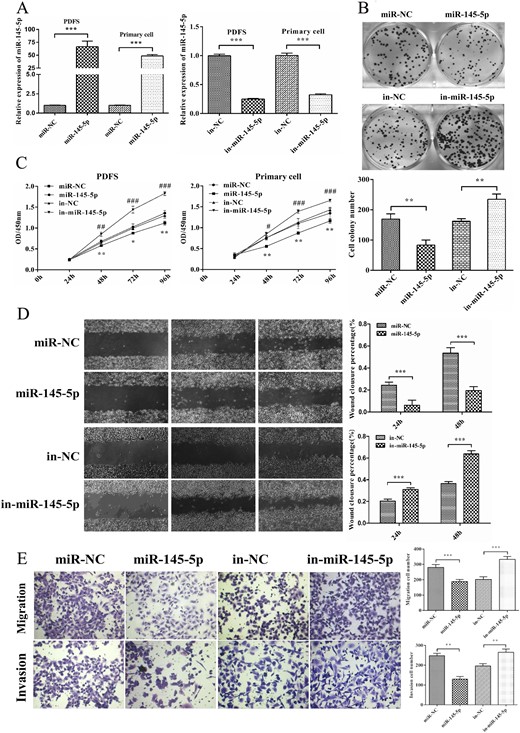
miR-145-5p inhibited cell proliferation, migration, and invasion in vitro. (A) The expression of miR-145-5p was confirmed by qRT-PCR after transfection with miR-145-5p mimics and inhibitor. (B) Colony formation assay of PDFS cells transfected with miR-145-5p mimics and inhibitor. (C) Effects of miR-145-5p on the proliferation of PDFS and primary cells were evaluated using CCK-8 assay. (D) A scratch-healing assay was used to measure the effect of miR-145-5p on the mobility of PDFS cells. (E) Effects of miR-145-5p on PDFS cell migration and invasion ability using a Transwell assay. *P < 0.05, **P < 0.01, ***P < 0.001.
To determine the effects of miR-145-5p on cell migration and invasion activities, a scratch healing assay and transwell assay were conducted with PDFS cells. Overexpression of miR-145-5p inhibited the migration and invasion ability of PDFS cells and silencing miR-145-5p significantly enhanced the migration and invasion activities (P < 0.01 for all; Fig. 2D and 2E). Taken together, these data have made it clear that miR-145-5p can suppress NFPA cell proliferation and invasion.
MiR-145-5p induces apoptosis of NFPAs cells by targeting TPT1
To determine the mechanism by which miR-145-5p suppresses NFPA cell growth, we examined the effect of miR-145-5p on cell apoptosis in PDFS and primary cells. We found that the fraction of apoptotic cells was significantly increased statistically in cells treated with the miR-145-5p mimic but decreased in cells treated with the miR-145-5p inhibitor (P < 0.01; Fig 3A). Together, these data indicate that miR-145-5p might suppress NFPA cell growth by inducing apoptosis.
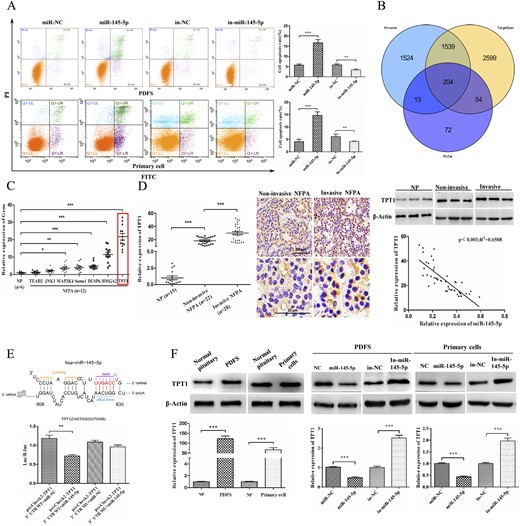
miR-145-5p induced apoptosis of NFPA cells by directly targeting TPT1. (A) Effects of miR-145-5p on cell apoptosis by flow cytometry. (B) Wayne chart of the targets of miR-145-5p predicted by three databases (TargetScan, PicTar, and MiRanda). (C) Relative expression of seven potential targets of miR-145-5p in NFPA samples (n = 12). (D) qRT-PCR evaluated the expression of TPT1 in more NFPA samples (total n = 50), indicating a greater level in the invasive NFPA tissues; immunohistochemistry and Western blot assay also confirmed the results. Correlation analysis confirmed a negative association between the expression of miR-145-5p and TPT1. Scale bars represent 50 μm and 20 μm, respectively. (E) Predicted binding sites of miR-145-5p within the 3′UTR of TPT1. miR-145-5p was cotransfected with the WT or mutant TPT1 3′-UTR vector to evaluate the effect of miR-145-5p on the luciferase activities by luciferase reporter assay. (F) Level of TPT1 in both PDFS and primary cells was evaluated with qRT-PCR and Western blot. The expression of TPT1 in miR-145-5p expressing or depleting cells was evaluated. *P < 0.05, **P < 0.01, ***P < 0.001.
To investigate the potential targets of miR-145-5p, we applied a combination of in silico prediction algorithms (TargetScan, PicTar, and miRanda). Next, the overlapping targets predicted by the three databases were identified, and the top 50 were chosen based on their binding sites and stability for further analysis (Fig. 3B). Finally, by examining previous studies, we identified 7 of these 50 genes that had been reported to be associated with cell apoptosis: TEAD2 (TEA domain transcription factor 2), JNK1 (mitogen-activated protein kinase 8), MAP2K4 (mitogen-activated protein kinase kinase 4), Sumo1 (small ubiquitin-like modifier 1), DUSP6 (dual-specificity phosphatase 6), HMGA2 (high mobility group AT-hook 2), and TPT1 (translationally controlled tumor protein) (23–26). Next, we examined the levels of each of these genes using qPCR and selected TPT1 for further analysis because TPT1 mRNA had the greatest level in NFPA specimens (n = 12; P < 0.001; Fig. 3C). Next, we examined the level of TPT1 in more NFPA samples (total of 50). Immunohistochemistry and Western blot showed that TPT1 expression was markedly greater in invasive tissues than in noninvasive tissues (P < 0.001; Fig. 3D). Moreover, the correlation analysis confirmed a negative association between the expression of miR-145-5p and TPT1 in NFPAs (P < 0.001; Fig. 3D).
With these results, we examined whether TPT1 was a direct target of miR-145-5p. Thus, a dual-luciferase reporter assay was performed. The reduction of luciferase activity was observed on cotransfection of miR-145-5p mimic and reporter plasmids psi-Check2-TPT1 wild type (WT; P < 0.01; Fig. 3E). However, mutations in the tentative miR-145-5p-binding seed region in TPT1 3′UTR abrogated the suppressive effect. These findings verified that TPT1 is a target of miR-145-5p. We further analyzed whether the expression of miR-145-5p had any effect on TPT1 levels using miR-145-5p mimics and inhibitors. We found that miR-145-5p overexpression led to a statistically significant decrease of TPT1 in both PDFS and primary cells, and silencing miR-145-5p led to greatly increased expression of TPT1 (P < 0.001; Fig. 3F).
To determine whether the effects of miR-145-5p in suppressing NFPA cell activities were mediated by repressing TPT1, we cotransfected NFPA cells with TPT1-expressing vector lacking the 3′UTR (pcDNA3.1-TPT1) and miR-145-5p mimic. First, the level of TPT1 was significantly upregulated, as expected (P < 0.01 for both; Fig. 4A). We then examined cell proliferation with CCK-8 and colony formation assays. Overexpression of WT TPT1 in NFPA cells transfected with the miR-145-5p mimic significantly rescued the inhibition of cell proliferation mediated by miR-145-5p alone [cells cotransfected with miR-145-5p mimic and pcDNA3.1-B (pcDNA3.1 blank vector); P < 0.05 for all; Fig. 4B]. Consistent with these results, the invasiveness and migration of PDFS cells were enhanced by restoring the expression of TPT1 (Fig. 4C); however, the proportion of apoptosis cells was decreased (P < 0.01; Fig. 4D). In contrast, the antiproliferative function of miR-145-5p was significantly promoted when TPT1 was silenced (P < 0.05 for all; Fig. 4E–4F).
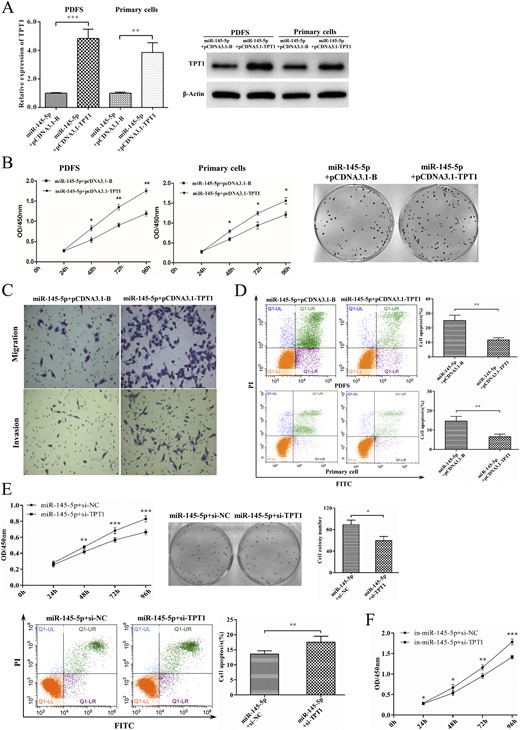
Repressing TPT1 blocked the tumor-suppressing effects of miR-145-5p. (A) Expression of TPT1 in cells cotransfected with miR-145-5p/pcDNA3.1-B and miR-145-5p/pcDNA3.1-TPT1. (B) Effect of TPT1 on miR-145-5p induced cell proliferation and colony formation. (C) Effect of TPT1 on miR-145-5p induced cell migration and invasion in vitro in Transwell chambers/Corning Matrigel Invasion Chambers. (D) Effect of TPT1 on miR-145-5p induced PDFS cell apoptosis by flow cytometry. (E) PDFS cells were cotransfected with miR-145-5p mimic and TPT1 siRNA; cells transfected with miR-145-5p mimic and siRNA-NC were set as the control. The effect of miR-145-5p was significantly enhanced when TPT1 was silenced. (F) Consistently, PDFS cells transfected with miR-145-5p inhibitor and TPT1 siRNA were significantly inhibited compared with those with miR-145-5p inhibitor and siRNA-NC. *P < 0.05, **P < 0.01, ***P < 0.001.
To verify the effect of miR-145-5p on tumorigenesis in vivo, the xenotransplanted tumor model in nude mice was established by subcutaneously injecting PDFS cells transfected with agomiR-145-5p or agomiR-NC (as a control group). The results showed that the tumorigenesis rate and size of the cells overexpressing miR-145-5p were significantly inhibited compared with those in the control group (Fig. 5A). We also examined the expression of miR-145-5p and TPT1 in miR-145-5p overexpressing xenograft tumor tissues. As expected, the expression of miR-145-5p was markedly increased and the TPT1 level was significantly downregulated (P < 0.05 for both; Fig. 5B). Together, these results have clearly indicated that miR-145-5p suppresses tumor proliferation and invasiveness in part by targeting TPT1.
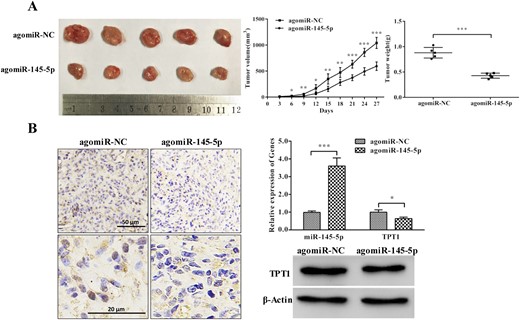
miR-145-5p inhibited PDFS cell tumorigenesis in vivo. (A) miR-145-5p inhibited PDFS cell proliferation and tumorigenesis in vivo. (B) Expression of miR-145-5p and TPT1 in miR-145-5p–expressing xenograft tumor tissues; immunohistochemistry assay confirmed significantly weaker staining of TPT1 in miR-145-5p–expressing xenograft tumor tissues. *P < 0.05, **P < 0.01, ***P < 0.001.
MiR-145-5p modulates the TPT1-Mcl-1/Bcl-xL/Bax signaling pathway
TPT1 has been demonstrated to regulate apoptosis by upregulating Mcl-1, Bcl-xL, and downregulating Bax (27–29). Similarly, we confirmed an increased expression of Mcl-1 and Bcl-xL and decreased Bax in NFPAs comparing with the NP samples (19). We, thus, assessed the effect of miR-145-5p on this signaling pathway. The levels of Mcl-1, Bcl-xL, and Bax were evaluated using qPCR and Western blot, respectively, in PDFS cells and primary cells transfected with miR-145-5p mimic or inhibitor. The levels of Mcl-1 and Bcl-xL were significantly decreased in cells transfected with miR-145-5p mimic and Bax was markedly increased compared with the levels in the controls (Fig. 6A). In contrast, significantly increased expression of Mcl-1 and Bcl-xL and decreased Bax expression were observed in cells transfected with the miR-145-5p inhibitor compared with the expression in the controls (Fig. 6B).
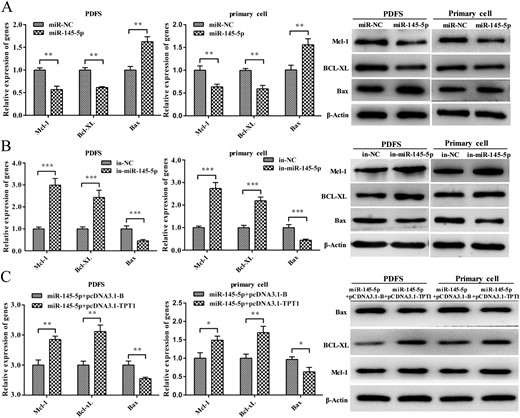
miR-145-5p regulates the TPT1 downstream signaling pathway. (A) Expression of Mcl-1, Bcl-xL, and Bax in miR-145-5p–expressing cells by qRT-PCR and Western blot. (B) Expression of Mcl-1, Bcl-xL, and Bax in miR-145-5p–silencing cells by qRT-PCR and Western blot. (C) Expression of Mcl-1, Bcl-xL, and Bax in cells cotransfected with miR-145-5p/pcDNA3.1-B and miR-145-5p/pcDNA3.1-TPT1. *P < 0.05, **P < 0.01, ***P < 0.001.
Similarly, we also transfected NFPA cells with the expression construct encoding the entire TPT1 coding sequence lacking the 3′ UTR. In cells cotransfected with this TPT1 expression vector, we found that the effect of miR-145-5p on Mcl-1, Bcl-xL, and Bax was reversed (Fig. 6C). Collectively, these data indicated miR-145-5p functions as a tumor suppressor by modulating the TPT1-Mcl-1/Bcl-xL/Bax signaling pathway.
CircOMA1 acts as a miR-145-5p sponge in NFPA cells
CircRNAs have been demonstrated to be involved in tumor progression by acting as sponges of miRNAs in various malignant tumors. Therefore, we hypothesized that circRNAs might be involved in the dysregulation of miR-145-5p in NFPAs. Using a combination of in silico prediction algorithms, an overlapping set of circRNAs predicted to bind miR-145-5p were identified, and five were selected for further analysis based on their binding sites and stability: hsa_circRNA_0002316 (circOMA1), hsa_circ_0000835, hsa_circ_0000825, hsa_circ_0007508, and hsa_circ_0000994 (19).
We next examined the expression of these circRNAs in NFPA samples. Four of them, except for hsa_circ_0007508, were significantly increased in NFPAs compared with NPs (P < 0.05; Fig. 7A). Next, we evaluated the effect of the four circRNAs on the proliferation of PDFS cells using the synthetic siRNAs, which covered the back-splicing region of circRNAs for silencing. CCK-8 assays showed that silencing of circOMA1 exhibited the greatest inhibitory effect on the proliferation of PDFS cells (19). Therefore, we identified circOMA1 as the candidate target for subsequent experiments. The expression of circOMA1 was also much greater in the invasive tissues than in the noninvasive ones, which confirmed its positive correlation with tumor invasiveness (P < 0.05; Fig. 7A). Thus, these results indicated that circOMA1 might be involved in the dysregulation of miR-145-5p.
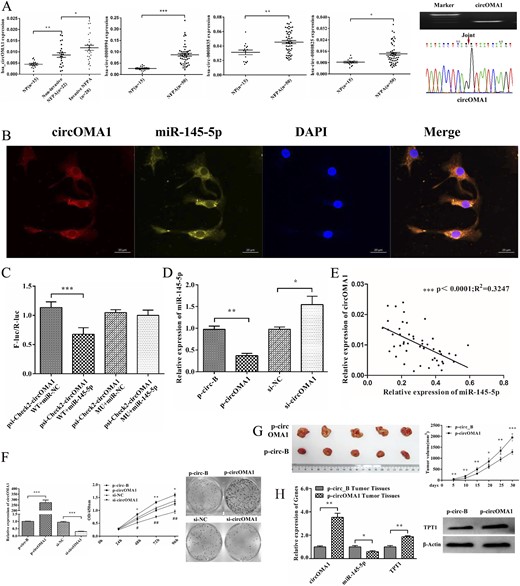
circOMA1 acts as miR-145-5p sponge in NFPA cells. (A) Expression of circOMA1, hsa_circ_0000835, hsa_circ_0000825, and hsa_circ_0000994 were validated in 50 NFPA tissues and 15 NP tissues using qRT-PCR and normalized to that of glyceraldehyde 3-phosphate dehydrogenase. circOMA1 expression was also much greater in invasive tissues than in noninvasive ones. qRT-PCR product of circOMA1 was displayed in 1% agarose gel electrophoresis and was consistent with the sequence in circBase using Sanger sequencing. The red arrow indicates the back splicing site of circOMA1. (B) miR-145-5p colocalized with circOMA1 in cytoplasm was detected by fluorescence in situ hybridization. Scale bars represent 20 μm. (C) Relative luciferase activity of psiCHECK2-circOMA1-WT or psiCHECK2-circOMA1-MU cotransfected with miR-145-5p or miR-NC. Renilla luciferase activity was normalized to the Firefly luciferase activity. (D) Effect of circOMA1 on the expression of miR-145-5p. (E) Correlation analysis between circOMA1 and miR-145-5p. (F) Effect of circOMA1 on cell proliferation and colony formation ability of PDFS cells. (G) circOMA1 promotes cell proliferation and tumorigenesis in vivo. (H) Expression of circOMA1, miR-145-5p, and TPT1 in circOMA1-expressing xenograft tumor tissue. *P < 0.05, **P < 0.01, ***P < 0.001.
Using fluorescence in situ hybridization analysis, we found that circOMA1 was colocalized with miR-145-5p in the cytoplasm in PDFS cells (Fig. 7B), indicating that circOMA1 might bind and interact with miR-145-5p in cytoplasm. Luciferase assays revealed a substantial decrease of luciferase activity on the cotransfection of the luciferase reporter vector psi-Check2-circOMA1 WT and miR-145-5p mimic compared with cotransfected with psi-Check2-circOMA1 WT and miR-NC mimic in HEK-293T cells. However, mutations in the miR-145-5p binding sites in circOMA1 counteracted the effect (P < 0.001; Fig. 7C), which confirmed that miR-145-5p can bind with circOMA1 in the complementary seed regions. Overexpression of circOMA1 resulted in reduced levels of miR-145-5p in PDFS cells, and knockdown circOMA1 led to upregulation of miR-145-5p (P < 0.05 for both; Fig. 7D). Furthermore, the circOMA1 expression level correlated negatively with the expression of miR-145-5p in NFPA samples (R2 = 0.3274; P < 0.001; Fig. 7E). Together, these results suggest that circOMA1 could reduce the activity and level of miR-145-5p in NFPA cells.
circOMA1 promotes proliferation and invasion of NFPA cells in vitro and vivo
To investigate the function of circOMA1 in NFPAs, we constructed a circOMA1 plasmid (p-circOMA1) for overexpression of circOMA1 and siRNA for silencing of circOMA1 and then assessed the effect on circOMA1. First, the level of circOMA1 was confirmed to be overexpressed or downregulated after transfection (P < 0.001 for both; Fig. 7F). Next, we investigated the effect of circOMA1 on the proliferation of NFPA cells. The results showed that circOMA1 overexpression significantly promoted the proliferation and colony formation of PDFS cells; however, the results were reversed with circOMA1 silencing (P < 0.05; Fig. 7F), possibly by inducing apoptosis (19). In addition, the invasion and migration capabilities of PDFS cells were enhanced by overexpression of circOMA1 (19). To verify the effect of circOMA1 on tumorigenesis and proliferation in vivo, we established the xenotransplanted tumor model in nude mice by subcutaneously injecting p-circOMA1 or p-circ-B (blank vector as control) transfected PDFS cells. The tumor formation rate and tumor size in the mice with p-circOMA1–transfected cells were significantly greater than the control group (Fig. 7G). We further examined the expressions of circOMA1, miR-145-5p, and TPT1 in xenograft tumor tissues from circOMA1-transfected cells. The results showed that circOMA1 was remarkably increased, miR-145-5p was decreased, and TPT1 was also significantly upregulated in these tumor tissues compared with the expression in the controls (P < 0.05 for both; Fig. 7H). Taken together, these data indicate that circOMA1 could promote NFPA cell proliferation and invasion.
circOMA1 promotes NFPA progression by sponging the activity of miR-145-5p and upregulating TPT1
To examine whether miR-145-5p silencing was required for circOMA1 promotion of cell proliferation and invasion, miR-145-5p was introduced into circOMA1-expressing PDFS cells (Fig. 8A). The effects of circOMA1 on the proliferation, colony formation, and invasion activities of PDFS cells were partly weakened with the introduction of miR-145-5p (P < 0.05 for both; Fig. 8A–8C). Reintroduction of miR-145-5p also significantly increased the percentage of apoptotic cells with circOMA1 expression (P < 0.001; Fig. 8D). Together, these results suggest that circOMA1 promotes NFPA progression by possibly reducing the level and activity of miR-145-5p.
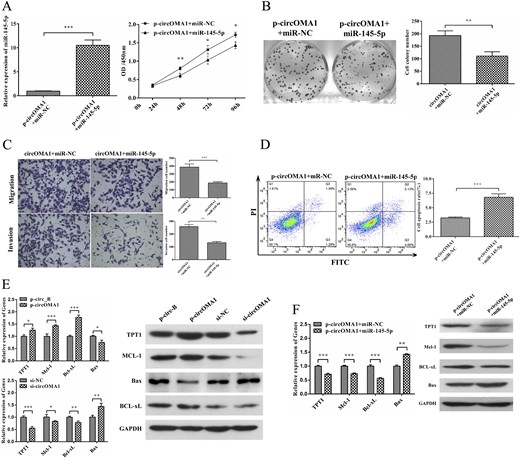
circOMA1 promotes NFPA progression by sponge activity of miR-145-5p and upregulating TPT1. (A) Expression of miR-145-5p in cells transfected with p-circOMA1 plus miR-NC and p-circOMA1 plus miR-145-5p. The effect of circOMA1 on PDFS cell proliferation was partly weakened by the introduction of miR-145-5p. (B) miR-145-5p suppressed circOMA1-inducing colony formation. (C) circOMA1-induced cell migration and invasion was weakened by miR-145-5p restoration. (D) Reintroduction of miR-145-5p mimic significantly increased the percentage of apoptosis. (E) Expression of TPT1, Mcl-1, Bcl-xL, and Bax in circ_OMA1-expressing or -silencing cells. (F) Expression of TPT1, Mcl-1, Bcl-xL, and Bax in circOMA1-expressed cells with reintroduction of miR-145-5p. *P < 0.05, **P < 0.01, ***P < 0.001.
We next examined whether circOMA1 exerts its oncogenic effects through the sponge activity of miR-145-5p. Because we found that TPT1 is a target of miR-145-5p, we first examined the effect of circOMA1 on TPT1 expression. Overexpression of circOMA1 increased the mRNA and protein levels of TPT1 in PDFS cells, and knockdown of circOMA1 had the reverse effects (Fig. 8E). We also examined the effect of circOMA1 on the gene expression of TPT1 downstream factors Mcl-1, Bcl-xL, and Bax. The levels of Mcl-1 and Bcl-xL increased significantly and that of Bax markedly decreased in circOMA1-overexpressing PDFS cells, and the reverse effects were observed when silencing circOMA1 (Fig. 8E). In addition, we detected the expression of TPT1, Mcl-1, Bcl-xL, and Bax in PDFS cells cotransfected with pcircOMA1 and miR-145-5p mimics. The results showed that the levels of TPT1, Mcl-1, and Bcl-xL had decreased markedly and the expression of Bax had increased markedly in these cells compared with cotransfected with pcircOMA1 and miR-NC mimics (Fig. 8F). Together, these data suggest that overexpression of miR-145-5p reversed the effect of circOMA1 on these genes. Together, these data have demonstrated that circOMA1 promotes NFPA progression by acting as the competing endogenous RNA of miR-145-5p and thus enhanced the oncogenic effect of the TPT1/Mcl-1/Bcl-xL/Bax axis.
Discussion
NFPAs represent the most clinically common pituitary adenomas, which manifest frequent invasion of the cavernous or parasellar structures at diagnosis. Surgical removal of invasive tumors remains a neurosurgical challenge, despite advances in endoscopic techniques (6). Exploring the mechanisms underlying the tumorigenesis of NFPAs will help to identify novel therapeutic targets. circRNA is a new member of noncoding RNAs that has been the subject of intense investigation. An increasing number of studies have shown that circRNAs are involved in the pathogenesis of many diseases by acting as sponges of miRNAs (14, 30, 31). However, as far as we know, the function of circRNAs in the pathogenesis of pituitary adenomas has not yet been explored. Based on our previous microarray analysis (8) and studies showing that miR-145-5p suppresses the tumorigenesis of several malignant tumors (9–12), we hypothesized that miR-145-5p might function as a tumor suppressor in NFPAs. We have demonstrated that miR-145-5p inhibits the proliferation and invasion of NFPAs in vitro and in vivo by targeting TPT1. We further confirmed that circOMA1 could enhance the oncogenic effects of TPT1 by sponging miR-145-5p antitumor activity. To the best of our knowledge, the present study is the first to reveal the role of a circRNA–miRNA–mRNA axis in pituitary adenomas, and this axis might represent a therapeutic target for NFPAs.
Previous studies have demonstrated that miR-145-5p could suppress cell proliferation and migration in various malignant tumors, including breast cancer, esophageal carcinoma, melanoma, glioma, and colorectal carcinoma (10, 12, 32–34). miR-145-5p could either induce cell cycle arrest (12) or increase the apoptosis of tumors cells (32, 33). Consistent with these results, we found substantial downregulation of miR-145-5p in NFPAs and an association with the invasiveness of NFPAs. Furthermore, overexpression of miR-145-5p reduced the growth of NFPA cells in vitro and in vivo by increasing cell apoptosis. Several targets of miR-145-5p have been identified, and these targets varied among the different types of tumors (e.g., Sp1, NRAS, BNIP3, PAK4) (10, 12, 32–34). Therefore, the targets and underlying mechanisms of miR-145-5p in regulating NFPAs might also be distinct from those for other cancers.
By applying a combination of in silico prediction algorithms, TPT1 was predicted as a target of miR-145-5p, and our luciferase reporter analysis verified this prediction. The expression of TPT1 was upregulated and correlated negatively with miR-145-5p expression in NFPA samples. Furthermore, miR-145-5p overexpression significantly decreased the level of TPT1 in NFPA cells. We assessed apoptosis-related proteins, including the antiapoptotic proteins Mcl-1 and Bcl-xL and the proapoptotic protein Bax, which have been reported to interact with TPT1 (27–29). The expression of Mcl-1 and Bcl-xL was significantly decreased in cells transfected with miR-145-5p that of Bax was markedly increased. These data indicate that miR-145-5p suppresses the proliferation of NFPA cells by targeting TPT1. However, because overexpression of TPT1 only partly blocked the effect of miR-145-5p, other pathways could also be involved in the effect of miR-145-5p on NFPA cells.
circRNAs can function as sponges of miRNAs to promote the expression of miRNA targets. For example, circMTO1 was demonstrated to suppress hepatocellular carcinoma cell growth by acting as the sponge of oncogenic miR-9 to promote p21 expression (30). Circulating IRAK3 promotes the metastasis of breast cancer cells by sponging miR-3607 and subsequently upregulating forkhead box C1 (35). Moreover, the results from a circRNA array indicated dysregulated expression of circRNAs between invasive and noninvasive NFPA samples (18). We, thus, explored whether circRNAs were involved in the dysregulation of miR-145-5p in NFPAs. Using bioinformatics analysis, we identified five potential circRNAs competitively binding miR-145-5p. Among them, circOMA1 exhibited a negative correlation with miR-145-5p and promoted NFPA xenograft tumor growth. Furthermore, circOMA1 and miR-145-5p colocalized in the cytoplasm of NFPA cells. circOMA1 was shown to suppress the activity of miR-145-5p and thus upregulate the expression of TPT1. Our preliminary data also indicated that circOMA1 might degrade miR-145-5p, because the level of miR-45-5p was significantly decreased in NFPA cells transfected with p-circOMA1. According to previous data, circRNAs have been shown to be able to downregulate the expression of miRNAs (36, 37). Because the main purpose of the present study was to investigate the function of miR-145-5p, the potential mechanisms underlying circOMA1 downregulation of miR-145-5p was not explored and requires further studies in the future. However, other mechanisms might also be involved in regulating the expression of oncogenic or tumor suppressive genes. For example, circRNA can bind to RNA-associated proteins to form RNA–protein complexes that regulate gene transcription (38). Moreover, circRNAs regulate the transcription of their parent genes in a cis manner (39). Sequence analysis showed that circOMA1 contains an open reading frame and two internal ribosome entry site elements, indicating that circOMA1 might translate a functional protein or peptide in NFPA. Further studies are warranted to reveal the functions and mechanisms of circOMA1 on NFPA tumorigenesis.
No real human cell lines of pituitary adenomas are available, seriously limiting the progression of pituitary adenoma investigations. In the present study, PDFS cells and a NFPA-derived cell line were used for the in vitro and in vivo studies. The PDFS cell line is folliculostellate in origin (20). Normal folliculostellate cells account for ≤10% of the cell population of the anterior pituitary and are known to be a part of pituitary tumors. Evidence has shown that some pituitary tumors could originate from folliculostellate cells (40). Therefore, PDFS has been used to explore the pathogenesis of pituitary adenomas in several previous studies (21, 41). In addition, we used a NFPA-derived cell line as complementary evidence for PDFS cell line. Although it is just a short-term cultured pituitary adenoma-derived cell, it remains a satisfactory study model for in vitro study, because no real human pituitary adenoma cell lines are available. Similarly, many previous studies have used such cells to explore the pathogenesis of pituitary adenomas (42).
Conclusions
Our study has shown that miR-145-5p is significantly decreased in NFPAs and correlates with the invasiveness of tumors in vivo and NFPA cells in vitro. Functionally, miR-145-5p could inhibit cell proliferation and invasiveness by targeting TPT1, suggesting its tumor suppressive role in NFPA development. Moreover, circOMA1 sponges the activity of miR-145-5p and subsequently upregulates oncogenic TPT1 expression. The regulation of the circOMA1–miR-145-5p–TPT1 axis represents a mechanism in the pathogenesis of NFPAs and might serve as a potential therapeutic target for the treatment of NFPAs.
Abbreviations:
- CCK-8
Cell Counting Kit-8
- circRNA
circular RNA
- HEK
human embryonic kidney
- NFPA
nonfunctioning pituitary adenoma
- NP
normal pituitary
- p-
plasmid-
- PDFS
pituitary tumor derived folliculostellate cell
- siRNA
small interfering RNA
- TPT1
translationally controlled tumor protein
- UTR
untranslated region
- WT
wild type
Acknowledgments
We thank Dr. Xun Zhang (Neuroendocrine Unit, Massachusetts General Hospital, Harvard Medical School, Boston, MA) for the gift of the PDFS cell line.
Financial Support: This research was supported by the National Nature and Science Grant of China (grant 81773943 to Y.Z.), National Natural Science Foundation of China for Young Scholars (grant 81702479 to X.J.), Science and Technology Planning Project of Guangdong Province (grants 2016A050502024 to H.W. and 2017A050501011 to Y.Z.), Guangzhou Science and Technology Project (grant 201704020085 to H.W.), and Doctoral Program of Natural Science Foundation of Guangdong Province (grant 2017A030310201 to X.J.).
Disclosure Summary: The authors have nothing to disclose.
References
Du Q, Hu B, Feng Y, Wang Z, Wang X, Zhu D, Zhu Y, Jiang X, Wang H. Data from: circOMA1-mediated miR-145-5p suppresses tumor growth of nonfunctioning pituitary adenomas by targeting TPT1. Figshare 2018. Deposited 24 November 2018. https://figshare.com/s/627feff25e62085a7879.
Author notes
Q.D. and B.H. contributed equally to this work.