-
PDF
- Split View
-
Views
-
Cite
Cite
Arash Derakhshan, Tim I M Korevaar, Peter N Taylor, Deborah Levie, Monica Guxens, Vincent W V Jaddoe, Scott M Nelson, Henning Tiemeier, Robin P Peeters, The Association of Maternal Thyroid Autoimmunity During Pregnancy With Child IQ, The Journal of Clinical Endocrinology & Metabolism, Volume 103, Issue 10, October 2018, Pages 3729–3736, https://doi.org/10.1210/jc.2018-00743
- Share Icon Share
Abstract
Thyroperoxidase antibody (TPOAb) positivity is a major risk factor for gestational thyroid dysfunction. During the first 18 to 20 weeks of pregnancy, high concentrations of human chorionic gonadotropin (hCG) stimulate the thyroid to ensure adequate thyroid hormone availability for the developing fetus. However, TPOAb-positive women have an impaired thyroidal response to hCG stimulation.
To study the association of maternal TPOAb positivity during pregnancy with child IQ.
This study was embedded in two prospective birth cohorts: Generation R (Rotterdam, the Netherlands) and Avon Longitudinal Study of Parents and Children (ALSPAC; United Kingdom). Mother-child pairs with available data on early pregnancy TPOAb (≤18 weeks of gestation) and offspring IQ were included (n = 3637 for Generation R and n = 2396 for ALSPAC).
Child IQ at 5 to 10 years of age.
In Generation R, TPOAb positivity was associated with a 2.0 ± 0.9-point lower mean child IQ (P = 0.03). Sensitivity analyses showed negative effect estimates already from TPOAb concentrations considerably lower than currently used manufacturer cutoffs. In ALSPAC, neither TPOAb positivity nor TPOAb concentrations below manufacturer cutoffs were associated with child IQ (TPOAb positivity: 0.7 ± 1.0; P = 0.45). Adjustment for maternal TSH or free T4 concentrations or urinary iodine/creatinine ratio did not change the results.
TPOAb positivity during pregnancy was associated with lower child IQ in Generation R but not in ALSPAC. Further studies are needed to elucidate whether differences between the study populations, such as maternal iodine status, could be the underlying cause for these differences.
Thyroperoxidase antibody (TPOAb) positivity occurs in about 2% to 17% of all pregnant women worldwide, and its prevalence differs according to maternal iodine intake and ethnicity (1). TPOAb positivity reflects thyroid autoimmunity, which typically results in higher serum TSH concentrations, lower serum free T4 (FT4) concentrations, and ultimately hypothyroidism (2, 3). Human chorionic gonadotropin (hCG) is a pregnancy-specific hormone that exerts thyrotropic activity via its affinity for the TSH receptor (4). During pregnancy, high hCG concentrations are associated with a ≤50% increase in FT4 concentrations (5). This increase in thyroid hormone availability ensures sufficient thyroxine availability for placental thyroxine transfer to the developing fetus (6). Although we recently showed that TPOAb positivity severely impairs the thyroidal response to hCG stimulation, it remains unknown whether this could affect early fetal development (7).
The fetal thyroid gland is not functionally mature until the 18th to 20th week of pregnancy; therefore, fetal thyroid hormone availability largely depends on the placental transfer of maternal thyroid hormones during early development (6, 8). In humans, neurogenesis starts from approximately the fifth week of pregnancy and thyroid hormone receptors are detected in the fetal brain from the eighth week of pregnancy (8). Various critical processes of fetal brain development that reach peak activity before the 18th to 20th week of pregnancy are regulated by thyroid hormone (9). Interestingly the specific period during which early fetal brain development depends on maternal thyroid hormones overlaps with peaking of hCG concentrations, facilitating a concomitant increase in maternal thyroid hormone concentrations (roughly 6 to 15 weeks of pregnancy) (5, 10).
The current guidelines of the American Thyroid Association (ATA) state that for TPOAb-positive women, levothyroxine treatment can be considered when TSH concentrations are >2.5 mU/L (1). This recommendation is predominantly based on studies showing that TPOAb positivity is associated with a higher risk for miscarriage and premature delivery (1, 11–14). Mild maternal thyroid dysfunction, particularly hypothyroxinemia, has been associated with suboptimal child neurodevelopmental outcomes, such as attention-deficit/hyperactivity disorder symptoms, lower IQ, autism, and schizophrenia (15–18); however, studies on the association of maternal TPOAb positivity with child neurodevelopment remain sparse. Although some studies have shown that maternal TPOAb positivity is associated with lower child IQ or other adverse neurodevelopmental outcomes (19–22), most of these studies were retrospective, had a small sample size, were unable to adjust for potential confounders, and/or did not specifically investigate the combination of TPOAb positivity and an elevated TSH concentration.
Considering that an attenuated thyroidal response to hCG stimulation in TPOAb-positive women likely leads to a relative form of thyroid hormone shortage during early pregnancy, during which fetal brain development depends on maternal thyroid hormone, we hypothesized that TPOAb positivity is associated with lower child IQ. To address this, we examined the association of maternal TPOAb positivity during pregnancy with child IQ in two large, prospective, population-based cohorts.
Methods
This study was embedded in two prospective birth cohorts: Generation R (Rotterdam, Netherlands) and the Avon Longitudinal Study of Parents and Children (ALSPAC, United Kingdom).
Study design and participants
In Generation R, 7069 women with a delivery date between April 2002 and January 2006 were enrolled during early pregnancy (≤18 weeks) in hospitals and midwife practices in Rotterdam (23). Blood samples were drawn in 6398 of these women and 5793 had enough material for measurement of TPOAbs. When the children reached 5 years of age, all enrolled mothers and children were invited to visit the research center at the Erasmus MC Sophia Children’s Hospital in Rotterdam, where 3753 (64%) children underwent IQ assessments. The general study design, all research aims, and the specific measurements in the Generation R Study have been approved by the Medical Ethical Committee of the Erasmus Medical Center, Rotterdam. Written informed consent was obtained from all participants and/or the children’s parents or guardians.
In ALSPAC, eligible women were those living in the former Avon area in Southwest England, United Kingdom, with an expected delivery date between April 1991 and December 1992. In total, blood samples were available in 7501 pregnant women, of whom 4947 were enrolled during early pregnancy (≤18 weeks) (24); 4916 women had TPOAb measurements. Subsequently, all participants were invited to attend a research clinic, where trained psychologists measured the IQ of 2552 children. The study website contains details of all the data that are available through a fully searchable database (http://www.bris.ac.uk/alspac/researchers/data-access/data-dictionary/). Ethical approval for the study was obtained from the ALSPAC Ethics and Law Committee and the Local Research Ethics Committees.
The exclusion criteria for both cohorts were twin pregnancies, women with preexisting thyroid disease or fertility treatment, and outliers of child IQ (defined as ±2.5 × median absolute deviation).
Laboratory measurements
In Generation R, maternal blood samples were stored at −80°C. TPOAbs were measured by using the Phadia 250 immunoassay (Phadia AB, Uppsala, Sweden) and were considered positive when the serum concentrations were >60 IU/mL. FT4 and TSH were measured by using chemiluminescence assays (Vitros ECI Immunodiagnostic System; Ortho Clinical Diagnostics, Rochester, NY). The intra-assay and interassay coefficients of variation were <4.1% for TSH at a range of 3.97 to 22.7 mU/L and <5.4% for FT4 at a range of 14.3 to 25.0 pmol/L. Details of the urinary iodine and creatinine measurement are reported elsewhere (25).
In ALSPAC, TPOAb, FT4, and TSH were measured in stored serum samples by using an Abbott Architect i2000 (Chicago, IL). Interassay and intra-assay coefficients of variation were <5% for all analytes. TPOAbs were considered positive when the serum concentrations were ≥6 IU/mL. Details on urinary iodine and creatinine measurements on a very limited subset are reported elsewhere (26).
Outcomes
In Generation R, nonverbal child IQ was evaluated by using two subtests of a Dutch nonverbal intelligence test, the Snijders-Oomen Niet-Verbale Intelligentietest, when the children were 5 to 8 years of age. The test generally evaluates a range of intelligence functions without relying on language skills and is therefore suitable for assessing the cognitive abilities of the children of ethnic minorities and children with verbal communication problems (27). The two subtests were mosaics (evaluating spatial visualization abilities) and categories (evaluating abstract reasoning abilities), and the correlation between subtests with complete test were r = 0.86. Raw test scores were converted into nonverbal IQ scores using normal values tailored to exact age. Research staff who did the IQ tests were unaware of any other mother-child measurements and outcomes.
In ALSPAC, child IQ was measured in a research clinic by using a well-validated age-adjusted shortened form of the Wechsler Intelligence Scale for Children, which provides a well-standardized assessment of performance and verbal intelligence when children were 7 to 10 years of age (26, 28). These assessments were administered by trained psychologists. To compare analyses to those conducted for Generation R, the performance component of child IQ was used as the primary outcome; supplemental analyses were also performed for the verbal component.
Statistical analysis
We used multivariable linear regression analyses to investigate the association of maternal TPOAb positivity with child IQ. We have recently shown that thyroid function and the response to hCG stimulation is already lower from concentrations below currently used TPOAb positivity cutoffs as provided by assay manufacturers (29). Therefore, we also performed sensitivity analyses to evaluate the effects of cutoffs below the currently used manufacturer-based cutoffs. TPOAbs were categorized at 20, 30, 40, 50, and 60 IU/mL in Generation R (corresponding to population-based percentiles: 90.6, 92.2, 93, 93.6, and 94.1, respectively). Considering that the manufacturer’s cutoff of TPOAb in the ALSPAC population (6 IU/mL) already stood at the 87.2nd percentile, to enable comparison between cohorts, population-based cutoffs equivalent to the cutoffs in Generation R were defined in ALSPAC (14.2, 29.6, 41.4, 54.8, and 63.1 IU/mL, respectively). The effect estimates for these cutoffs were compared with TPOAb <10 IU/mL (population-based percentile of 83) in Generation R and the corresponding percentile (<4.16 IU/mL) in ALSPAC. The severely skewed distribution of (log-transformed) TPOAb concentrations did not allow for reliable analyses with use of TPOAb concentrations as a continuous exposure.
On the basis of the current ATA guidelines (1), we additionally investigated the group of TPOAb-positive women with a TSH concentration > 2.5 mU/L [n = 118 (3.4%) and n = 52 (2.46%) in Generation R and ALSPAC, respectively]. Because maternal iodine status is a well-known determinant of both thyroid autoimmunity and child IQ (26, 30), in a subset of mothers with available data on early-pregnancy iodine (n = 1330 in Generation R and n = 1065 in ALSPAC), we investigated the possible effects of maternal iodine status on the association of TPOAbs with child IQ by: (1) studying the association of TPOAbs with maternal urinary iodine/creatinine ratio (UICr) using a linear regression model; (2) additionally adjusting all analyses for maternal UICr; and (3) stratifying analyses in both cohorts according to a UICr <150 µg/g and >150 µg/g. Furthermore, we also investigated whether the association of maternal TPOAbs with child IQ would be (partially) mediated via changes in maternal thyroid function by additionally adjusting all models for maternal FT4 and TSH.
All analyses were adjusted for maternal age, body mass index and gestational age at the time of blood sampling, parity, smoking status, education level, ethnicity, child sex, and birth weight. We used multiple imputation by chained equations to deal with missing data of covariates (31). The maximum percentages of missing data were 10.3% in Generation R and 3.7% for ALSPAC. The number of imputations was based on the percentage of missing data by using at least one imputation per percentage of incomplete cases. All statistical analyses were performed by using SPSS software, version 21.0 for Windows (IBM, Armonk, NY), or R statistical software, version 3.3.2 (packages mice and rms; R Foundation, Vienna, Austria).
Results
After exclusions, the final study population included 6033 mother-child pairs (Generation R: n = 3564; ALSPAC: n = 2362) (Fig. 1). Mother-child characteristics of the study population are shown in Table 1. In Generation R, the prevalence of TPOAb positivity was 5.9%, mean gestational age at blood sampling was 13.4 (SD, 1.9) weeks, and the study population was mainly of Dutch ethnicity (57.3%). In ALSPAC, the prevalence of TPOAb positivity was 12.8%, mean gestational age at blood sampling was 10.9 (SD, 3.1) weeks, and the study population was mainly of white ethnicity (98.5%). In both cohorts, maternal TPOAb positivity and thyroid function did not differ between mother-child pairs with or without IQ data available (Supplemental Tables 1 and 2).
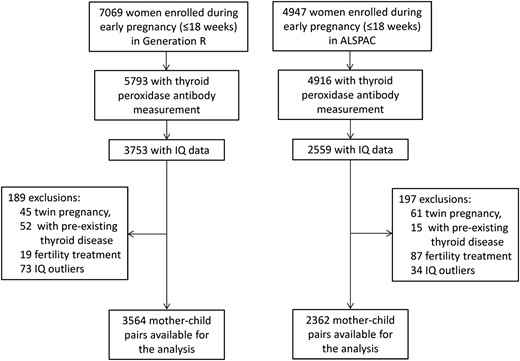
Characteristics of Mother-Child Pairs From the Generation R Study and ALSPAC
Characteristic . | Generation R (n = 3564) . | ALSPAC (n = 2362) . |
---|---|---|
Maternal characteristics | ||
TPOAb, IU/mL | 3.2 (0.0–7.1) | 1.9 (1.3–3.1) |
TPOAb positivity, n (%) | 214 (5.9) | 286 (12.8) |
FT4, pmol/L | 15.2 (3.6) | 16.5 (2.5) |
TSH, mU/L | 1.37 (0.85–2.05) | 0.99 (0.64–1.44) |
Gestational age, wk | 13.4 (1.8) | 10.9 (3.1) |
Age, y | 30.3 (4.8) | 28.2 (4.5) |
BMI, kg/m2 | 24.4 (4.2) | 22.8 (3.5) |
Education level, % | ||
None or primary only | 8.4 | 13.1 |
Secondary phase 1 (3–4 y) | 12.5 | 9.0 |
Secondary phase 2 (4–5 y) | 31.6 | 35.6 |
Higher phase 1 (6–8 y) | 22.3 | 26.6 |
Higher phase 2 (>8 y) | 25.2 | 15.7 |
Parity, % | ||
0 | 59.0 | 47.2 |
1 | 29.1 | 34.2 |
≥2 | 11.7 | 18.6 |
Smoking status, % | ||
Nonsmokers | 73.4 | 81.0 |
Previous smokers | 9.65 | 4.8 |
Current smokers | 16.9 | 14.2 |
Ethnic origin (Generation R) % | ||
Dutch | 57.3 | — |
Moroccan | 5.14 | — |
Turkish | 7.34 | — |
Surinamese | 7.80 | — |
Cape Verdian | 4.09 | — |
Asian | 4.26 | — |
Other European, North American, or Australian | 8.71 | — |
Other ethnicities | 5.30 | — |
Ethnic origin (ALSPAC), % | ||
Caucasian | — | 98.5 |
Other ethnicities | — | 1.5 |
Child characteristics | ||
IQ | 101.41 (15.1) | 99.5 (16.9) |
Birthweight, g | 3434 (548) | 3460 (523) |
Female, % | 50.8 | 49.8 |
Characteristic . | Generation R (n = 3564) . | ALSPAC (n = 2362) . |
---|---|---|
Maternal characteristics | ||
TPOAb, IU/mL | 3.2 (0.0–7.1) | 1.9 (1.3–3.1) |
TPOAb positivity, n (%) | 214 (5.9) | 286 (12.8) |
FT4, pmol/L | 15.2 (3.6) | 16.5 (2.5) |
TSH, mU/L | 1.37 (0.85–2.05) | 0.99 (0.64–1.44) |
Gestational age, wk | 13.4 (1.8) | 10.9 (3.1) |
Age, y | 30.3 (4.8) | 28.2 (4.5) |
BMI, kg/m2 | 24.4 (4.2) | 22.8 (3.5) |
Education level, % | ||
None or primary only | 8.4 | 13.1 |
Secondary phase 1 (3–4 y) | 12.5 | 9.0 |
Secondary phase 2 (4–5 y) | 31.6 | 35.6 |
Higher phase 1 (6–8 y) | 22.3 | 26.6 |
Higher phase 2 (>8 y) | 25.2 | 15.7 |
Parity, % | ||
0 | 59.0 | 47.2 |
1 | 29.1 | 34.2 |
≥2 | 11.7 | 18.6 |
Smoking status, % | ||
Nonsmokers | 73.4 | 81.0 |
Previous smokers | 9.65 | 4.8 |
Current smokers | 16.9 | 14.2 |
Ethnic origin (Generation R) % | ||
Dutch | 57.3 | — |
Moroccan | 5.14 | — |
Turkish | 7.34 | — |
Surinamese | 7.80 | — |
Cape Verdian | 4.09 | — |
Asian | 4.26 | — |
Other European, North American, or Australian | 8.71 | — |
Other ethnicities | 5.30 | — |
Ethnic origin (ALSPAC), % | ||
Caucasian | — | 98.5 |
Other ethnicities | — | 1.5 |
Child characteristics | ||
IQ | 101.41 (15.1) | 99.5 (16.9) |
Birthweight, g | 3434 (548) | 3460 (523) |
Female, % | 50.8 | 49.8 |
Data are median (interquartile range), mean (SD), or percentage.
Characteristics of Mother-Child Pairs From the Generation R Study and ALSPAC
Characteristic . | Generation R (n = 3564) . | ALSPAC (n = 2362) . |
---|---|---|
Maternal characteristics | ||
TPOAb, IU/mL | 3.2 (0.0–7.1) | 1.9 (1.3–3.1) |
TPOAb positivity, n (%) | 214 (5.9) | 286 (12.8) |
FT4, pmol/L | 15.2 (3.6) | 16.5 (2.5) |
TSH, mU/L | 1.37 (0.85–2.05) | 0.99 (0.64–1.44) |
Gestational age, wk | 13.4 (1.8) | 10.9 (3.1) |
Age, y | 30.3 (4.8) | 28.2 (4.5) |
BMI, kg/m2 | 24.4 (4.2) | 22.8 (3.5) |
Education level, % | ||
None or primary only | 8.4 | 13.1 |
Secondary phase 1 (3–4 y) | 12.5 | 9.0 |
Secondary phase 2 (4–5 y) | 31.6 | 35.6 |
Higher phase 1 (6–8 y) | 22.3 | 26.6 |
Higher phase 2 (>8 y) | 25.2 | 15.7 |
Parity, % | ||
0 | 59.0 | 47.2 |
1 | 29.1 | 34.2 |
≥2 | 11.7 | 18.6 |
Smoking status, % | ||
Nonsmokers | 73.4 | 81.0 |
Previous smokers | 9.65 | 4.8 |
Current smokers | 16.9 | 14.2 |
Ethnic origin (Generation R) % | ||
Dutch | 57.3 | — |
Moroccan | 5.14 | — |
Turkish | 7.34 | — |
Surinamese | 7.80 | — |
Cape Verdian | 4.09 | — |
Asian | 4.26 | — |
Other European, North American, or Australian | 8.71 | — |
Other ethnicities | 5.30 | — |
Ethnic origin (ALSPAC), % | ||
Caucasian | — | 98.5 |
Other ethnicities | — | 1.5 |
Child characteristics | ||
IQ | 101.41 (15.1) | 99.5 (16.9) |
Birthweight, g | 3434 (548) | 3460 (523) |
Female, % | 50.8 | 49.8 |
Characteristic . | Generation R (n = 3564) . | ALSPAC (n = 2362) . |
---|---|---|
Maternal characteristics | ||
TPOAb, IU/mL | 3.2 (0.0–7.1) | 1.9 (1.3–3.1) |
TPOAb positivity, n (%) | 214 (5.9) | 286 (12.8) |
FT4, pmol/L | 15.2 (3.6) | 16.5 (2.5) |
TSH, mU/L | 1.37 (0.85–2.05) | 0.99 (0.64–1.44) |
Gestational age, wk | 13.4 (1.8) | 10.9 (3.1) |
Age, y | 30.3 (4.8) | 28.2 (4.5) |
BMI, kg/m2 | 24.4 (4.2) | 22.8 (3.5) |
Education level, % | ||
None or primary only | 8.4 | 13.1 |
Secondary phase 1 (3–4 y) | 12.5 | 9.0 |
Secondary phase 2 (4–5 y) | 31.6 | 35.6 |
Higher phase 1 (6–8 y) | 22.3 | 26.6 |
Higher phase 2 (>8 y) | 25.2 | 15.7 |
Parity, % | ||
0 | 59.0 | 47.2 |
1 | 29.1 | 34.2 |
≥2 | 11.7 | 18.6 |
Smoking status, % | ||
Nonsmokers | 73.4 | 81.0 |
Previous smokers | 9.65 | 4.8 |
Current smokers | 16.9 | 14.2 |
Ethnic origin (Generation R) % | ||
Dutch | 57.3 | — |
Moroccan | 5.14 | — |
Turkish | 7.34 | — |
Surinamese | 7.80 | — |
Cape Verdian | 4.09 | — |
Asian | 4.26 | — |
Other European, North American, or Australian | 8.71 | — |
Other ethnicities | 5.30 | — |
Ethnic origin (ALSPAC), % | ||
Caucasian | — | 98.5 |
Other ethnicities | — | 1.5 |
Child characteristics | ||
IQ | 101.41 (15.1) | 99.5 (16.9) |
Birthweight, g | 3434 (548) | 3460 (523) |
Female, % | 50.8 | 49.8 |
Data are median (interquartile range), mean (SD), or percentage.
In Generation R, maternal TPOAb positivity was associated with lower mean child IQ (−2.0 ± 0.9 points; P = 0.03) (Table 2). Subsequent sensitivity analyses showed that mean child IQ was already lower at TPOAb cutoffs below the currently used manufacturer-based cutoff for TPOAb positivity (Table 2). In ALSPAC, with use of cutoffs equivalent to Generation R, neither TPOAb positivity nor TPOAb cutoffs below the manufacturer-based cutoff were associated with child IQ (TPOAb positivity: 0.7 ± 0.9 points; P = 0.45) (Table 2). The combination of TPOAb positivity with a TSH >2.5 mU/L was not associated with child IQ in Generation R (P for interaction = 0.52), whereas this combination was associated with a higher mean child IQ in ALSPAC (P for interaction = 0.09) (Supplemental Table 3). All results remained essentially unchanged after adjustment for maternal FT4 concentrations (Table 2), UICr (Supplemental Table 4), maternal TSH concentrations (data not shown), or hCG concentrations (Generation R only; data not shown). In Generation R, the association of TPOAbs with child IQ did not differ according to maternal ethnicity.
Differences in Mean Child IQ Scores According to TPOAbs in Generation R and ALSPAC
TPOAb . | β ± SEM . | P Value . | β ± SEM (+FT4) a . | P Value . |
---|---|---|---|---|
Generation R | ||||
Positivityb | −2.10 ± 0.92 | 0.02 | −2.00 ± 0.92 | 0.03 |
vs <10c | ||||
>20 | −1.30 ± 0.75 | 0.08 | −1.14 ± 0.75 | 0.13 |
>30 | −1.72 ± 0.81 | 0.03 | −1.55 ± 0.82 | 0.05 |
>40 | −1.80 ± 0.85 | 0.03 | −1.69 ± 0.85 | 0.04 |
>50 | −2.17 ± 0.89 | 0.01 | −2.06 ± 0.89 | 0.02 |
ALSPAC | ||||
Positivityb | 0.74 ± 0.97 | 0.43 | 0.72 ± 0.97 | 0.45 |
vs <4.16c | ||||
>14.2 | 1.73 ± 1.12 | 0.12 | 1.68 ± 1.12 | 0.13 |
>29.6 | 1.79 ± 1.21 | 0.13 | 1.73 ± 1.21 | 0.15 |
>41.4 | 1.80 ± 1.27 | 0.15 | 1.74 ± 1.28 | 0.17 |
>54.8 | 2.29 ± 1.32 | 0.08 | 2.22 ± 1.33 | 0.09 |
>63.1 | 2.11 ± 1.38 | 0.12 | 2.03 ± 1.38 | 0.14 |
TPOAb . | β ± SEM . | P Value . | β ± SEM (+FT4) a . | P Value . |
---|---|---|---|---|
Generation R | ||||
Positivityb | −2.10 ± 0.92 | 0.02 | −2.00 ± 0.92 | 0.03 |
vs <10c | ||||
>20 | −1.30 ± 0.75 | 0.08 | −1.14 ± 0.75 | 0.13 |
>30 | −1.72 ± 0.81 | 0.03 | −1.55 ± 0.82 | 0.05 |
>40 | −1.80 ± 0.85 | 0.03 | −1.69 ± 0.85 | 0.04 |
>50 | −2.17 ± 0.89 | 0.01 | −2.06 ± 0.89 | 0.02 |
ALSPAC | ||||
Positivityb | 0.74 ± 0.97 | 0.43 | 0.72 ± 0.97 | 0.45 |
vs <4.16c | ||||
>14.2 | 1.73 ± 1.12 | 0.12 | 1.68 ± 1.12 | 0.13 |
>29.6 | 1.79 ± 1.21 | 0.13 | 1.73 ± 1.21 | 0.15 |
>41.4 | 1.80 ± 1.27 | 0.15 | 1.74 ± 1.28 | 0.17 |
>54.8 | 2.29 ± 1.32 | 0.08 | 2.22 ± 1.33 | 0.09 |
>63.1 | 2.11 ± 1.38 | 0.12 | 2.03 ± 1.38 | 0.14 |
TPOAb values are expressed in IU/mL. β ± SEM values were calculated by using a linear regression model, adjusted for gestational age at the time of sampling, maternal age, maternal body mass index, education, ethnicity, smoking status, parity, birth weight, and child sex.
Additionally adjusted with FT4.
Defined as TPOAb > 60 IU/mL or TPOAb > 6 IU/mL in Generation R and ALSPAC, respectively.
Those with TPOAbs values between 10 IU/mL and 4.16 IU/mL and each cutoff were excluded from the analysis.
Differences in Mean Child IQ Scores According to TPOAbs in Generation R and ALSPAC
TPOAb . | β ± SEM . | P Value . | β ± SEM (+FT4) a . | P Value . |
---|---|---|---|---|
Generation R | ||||
Positivityb | −2.10 ± 0.92 | 0.02 | −2.00 ± 0.92 | 0.03 |
vs <10c | ||||
>20 | −1.30 ± 0.75 | 0.08 | −1.14 ± 0.75 | 0.13 |
>30 | −1.72 ± 0.81 | 0.03 | −1.55 ± 0.82 | 0.05 |
>40 | −1.80 ± 0.85 | 0.03 | −1.69 ± 0.85 | 0.04 |
>50 | −2.17 ± 0.89 | 0.01 | −2.06 ± 0.89 | 0.02 |
ALSPAC | ||||
Positivityb | 0.74 ± 0.97 | 0.43 | 0.72 ± 0.97 | 0.45 |
vs <4.16c | ||||
>14.2 | 1.73 ± 1.12 | 0.12 | 1.68 ± 1.12 | 0.13 |
>29.6 | 1.79 ± 1.21 | 0.13 | 1.73 ± 1.21 | 0.15 |
>41.4 | 1.80 ± 1.27 | 0.15 | 1.74 ± 1.28 | 0.17 |
>54.8 | 2.29 ± 1.32 | 0.08 | 2.22 ± 1.33 | 0.09 |
>63.1 | 2.11 ± 1.38 | 0.12 | 2.03 ± 1.38 | 0.14 |
TPOAb . | β ± SEM . | P Value . | β ± SEM (+FT4) a . | P Value . |
---|---|---|---|---|
Generation R | ||||
Positivityb | −2.10 ± 0.92 | 0.02 | −2.00 ± 0.92 | 0.03 |
vs <10c | ||||
>20 | −1.30 ± 0.75 | 0.08 | −1.14 ± 0.75 | 0.13 |
>30 | −1.72 ± 0.81 | 0.03 | −1.55 ± 0.82 | 0.05 |
>40 | −1.80 ± 0.85 | 0.03 | −1.69 ± 0.85 | 0.04 |
>50 | −2.17 ± 0.89 | 0.01 | −2.06 ± 0.89 | 0.02 |
ALSPAC | ||||
Positivityb | 0.74 ± 0.97 | 0.43 | 0.72 ± 0.97 | 0.45 |
vs <4.16c | ||||
>14.2 | 1.73 ± 1.12 | 0.12 | 1.68 ± 1.12 | 0.13 |
>29.6 | 1.79 ± 1.21 | 0.13 | 1.73 ± 1.21 | 0.15 |
>41.4 | 1.80 ± 1.27 | 0.15 | 1.74 ± 1.28 | 0.17 |
>54.8 | 2.29 ± 1.32 | 0.08 | 2.22 ± 1.33 | 0.09 |
>63.1 | 2.11 ± 1.38 | 0.12 | 2.03 ± 1.38 | 0.14 |
TPOAb values are expressed in IU/mL. β ± SEM values were calculated by using a linear regression model, adjusted for gestational age at the time of sampling, maternal age, maternal body mass index, education, ethnicity, smoking status, parity, birth weight, and child sex.
Additionally adjusted with FT4.
Defined as TPOAb > 60 IU/mL or TPOAb > 6 IU/mL in Generation R and ALSPAC, respectively.
Those with TPOAbs values between 10 IU/mL and 4.16 IU/mL and each cutoff were excluded from the analysis.
The median maternal UICr differed considerably between Generation R and ALSPAC [median (interquartile range), 295 (199 to 425) µg/g vs 117 (80 to 190) µg/g; P < 0.001]. In ALSPAC, but not in Generation R, higher TPOAb concentrations or TPOAb positivity was associated with higher maternal UICr, although these analyses did not reach statistical significance in the smaller subgroups (Supplemental Table 5). Sensitivity analyses suggested that the association of maternal TPOAb positivity with child IQ may differ according to maternal iodine status, although we lacked adequate statistical power for this analysis (Supplemental Table 6). There was no association of UICr with thyroid function in the two cohorts (data not shown).
Discussion
In this study, we investigated the association of TPOAb positivity during early pregnancy with child IQ in two large prospective population-based cohorts. We show that TPOAb positivity, as defined by currently used manufacturer-based cutoffs, was associated with lower mean child IQ in the Netherlands (Generation R) but not in the United Kingdom (ALSPAC). Furthermore, the association of TPOAbs with lower child IQ in the Netherlands was already present from TPOAb cutoffs below the currently used manufacturer-based cutoffs. Additional adjustment for maternal FT4 or TSH or UICr did not change the results.
There is an overlap in the period of fetal brain development’s peak activity, fetal dependency on placental transfer of maternal thyroid hormones, and hCG-mediated increase in maternal FT4 concentrations (4, 8, 9). However, TPOAb-positive women have an impaired response to the thyroidal stimulation by hCG, and low maternal thyroid hormone availability is associated with lower child IQ in some but not all studies (32–35). In the current study, TPOAb positivity was associated with lower child IQ in Generation R, which might have been due to a lack of hCG-mediated increase in FT4 concentrations during early pregnancy (29). Alternatively, TPOAb positivity could be associated with lower child IQ because TPOAb positivity reflects a higher general susceptibility to autoimmunity. Maternal autoimmunity or a familial history of autoimmune disorders has been associated with a higher risk of child autism (36, 37). A third potential explanation would be a direct effect of TPOAbs on the brain. TPOAbs can cross the placenta and have been detected in the cerebrospinal fluid of patients with Hashimoto encephalitis (38, 39). In addition, because newborn and childhood TSH or FT4 do not differ between TPOAb-positive and TPOAb-negative mothers (40) and newborn thyroid function is not associated with neurocognitive outcomes (41, 42), a pathway via changes in child thyroid function is highly unlikely.
However, although TPOAb positivity was associated with a lower child IQ in Generation R, there was no association in ALSPAC, with point estimates even suggesting a positive association of TPOAb positivity with child IQ. There might be several reasons for the discrepancy between the two cohorts.
First, iodine status of pregnant women greatly differs between the Netherlands (where it is more than sufficient) and United Kingdom (where it is mildly deficient), as was also reflected by the UICr analyses in the current study. Both low and high iodine intake are risk factors for low maternal thyroid hormone availability, and both are associated with an increased risk for thyroid autoimmunity (30, 43).
Previous studies have shown that in the iodine-sufficient population of Generation R, a single low maternal UICr measurement is not associated with child IQ, whereas a low UICr measurement in the iodine-deficient ALSPAC cohort is associated with lower child IQ (26, 44). Stratified analysis suggested that in Generation R, the association of TPOAb positivity with lower child IQ was driven predominantly by women with a UICr ≥150 µg/g. This would indicate that in Generation R, low iodine status is not the underlying mechanism. In ALSPAC, there was a suggestive association of higher TPOAb concentrations or TPOAb positivity with a higher maternal UICr, although the small size of the subset with available data did not provide adequate statistical power. Similarly, a higher iodine availability could underlie the higher mean IQ in the small subset of TPOAb-positive women with a TSH >2.5 mU/L. However, neither in Generation R nor in ALSPAC could we adequately asses iodine status in this small subset because of a lack of data availability (data not shown). In addition, our study had power only to detect a roughly 3.6-point IQ difference in the subset of TPOAb-positive women with a TSH >2.5 mU/L in Generation R.
Taken together, these factors might suggest that the difference between Generation R and ALSPAC could be due to the differences in iodine status between the two cohorts. Unfortunately, data on UICr were available only in a small subset for both studies, precluding adequate analyses to investigate the role of UICr as an underlying cause for the differences between the two cohorts. In line with our hypothesis, TPOAb positivity has been associated with impaired child cognition, autism, and behavioral problems in other studies from iodine sufficient populations (21, 22, 45), whereas a Scottish study in which 40% of women were iodine deficient did not find an association with neurodevelopmental outcomes (20).
The second possible reason for a discrepancy between the two cohorts concerns the timing of sample collection. Whereas serum samples in Generation R were collected between 2002 and 2005, and TPOAbs were measured in 2006, ALSPAC samples were collected between 1991 and 1992 and measured in 2016. A study from Finland showed that in stored serum samples, there is a strong positive association of storage time with TPOAb concentrations, with storage time explaining 19.7% of the total variation in TPOAb concentrations (46). This indicates that TPOAb concentrations in ALSPAC are much more likely to be subject to measurement error than those in Generation R. Although it is unknown whether the extent of the increase in TPOAb concentration by storage time is differential on factors that may affect IQ, the difference in storage time may hamper the comparisons between Generation R and ALSPAC in the current study.
The current ATA guidelines recommend that treatment can be considered in TPOAb-positive women if the TSH concentration is >2.5 mU/L (1). In the current study, the association of TPOAb positivity with lower child IQ in Generation R did not differ according to a TSH <2.5 mU/L or >2.5 mU/L. Given that the guideline recommendations are predominantly based on studies focusing on adverse obstetric outcomes, the lack of effects in the current study cannot be considered as an argument against current recommendations. Nonetheless, further studies are required to investigate from which TSH threshold the risk for adverse outcomes in TPOAb-positive women starts to increase.
In the current study, we also showed that TPOAb cutoffs below the currently used manufacturer-based cutoffs for TPOAb positivity were associated with a lower child IQ. This is in line with a previous study from our group, showing that TPOAb concentrations already below currently used manufacturer cutoffs are associated with a higher TSH and a higher risk for premature delivery (29). Taken together, this suggests that the clinically relevant cutoff for TPOAb positivity may differ from the currently used manufacturer-based cutoffs and that future studies should focus on identifying the optimal threshold for TPOAb positivity for different pregnancy and offspring outcomes.
This study assessed the association of early-pregnancy TPOAb positivity with child IQ in two large prospective, population-based cohorts. We were able to study this association in two study populations with a different population iodine status with detailed data that allowed us to adjust the models for important confounders and run additional sensitivity analyses.
A potential limitation of this study is that data on maternal iodine excretion were not available for all mothers, which left us with inadequate statistical power for sensitivity analysis investigating the potential role of iodine intake. Further studies are therefore needed to investigate the role of maternal thyroid autoimmunity in relation to iodine status with regard to child cognitive development. In addition, the number of TPOAb-positive women with a TSH >2.5 mU/L was small, hampering an adequately powered analysis for this subgroup. Finally, the comparisons of the two cohorts was limited mainly because of differences in tests used to assess IQ. In ALSPAC, a verbal IQ test was performed that differs from nonverbal IQ as tested in Generation R, the latter of which can overcome potential interference by language development delays. Nonetheless, in a recent study comparing three cohorts using three different IQ tests, similar results were obtained for the effects of low thyroid function (47).
In conclusion, we demonstrate that TPOAb positivity during early pregnancy is associated with lower child IQ in a Dutch, iodine-sufficient population but not in a mildly iodine-deficient population from the United Kingdom. In addition, TPOAb cutoffs below the current manufacturer-based cutoffs were associated with lower mean child IQ in the Netherlands. Further studies are needed to investigate the association of TPOAbs with child neurodevelopment outcomes in different populations and to evaluate whether factors that affect thyroid autoimmunity, such as iodine status, might possibly modify this association.
Abbreviations:
- ALSPAC
Avon Longitudinal Study of Parents and Children
- ATA
American Thyroid Association
- FT4
free T4
- hCG
human chorionic gonadotropin
- TPOAb
thyroperoxidase antibody
- UICr
urinary iodine/creatinine ratio
Acknowledgments
The Generation R study is conducted by the Erasmus Medical Center (Rotterdam) in close collaboration with the School of Law and Faculty of Social Sciences of the Erasmus University Rotterdam, the Municipal Health Service Rotterdam area, Rotterdam, the Rotterdam Homecare Foundation, Rotterdam, and the Stichting Trombosedienst and Artsenlaboratorium Rijnmond, Rotterdam. We gratefully acknowledge the contribution of children and parents, general practitioners, hospitals, midwives, and pharmacies in Rotterdam.
We thank all the families who took part in ALSPAC; the midwives for help in recruiting them; and the whole ALSPAC team, which includes interviewers, computer and laboratory technicians, clerical workers, research scientists, volunteers, managers, receptionists, and nurses. Thyroid function tests in ALSPAC were performed using grants from the Bupa Research Foundation, British Thyroid Association, and the Above and Beyond Foundation.
Financial Support: This work was supported by a clinical fellowship from ZonMw, project number 90 700 412 (to R.P.P.). The general design of the Generation R Study is made possible by financial support from the Erasmus Medical Center, Rotterdam, the Erasmus University Rotterdam, The Netherlands Organization for Health Research and Development, The Netherlands Organisation for Scientific Research, the Ministry of Health, Welfare, and Sport, and the Ministry of Youth and Families. The UK Medical Research Council and Wellcome (grant 102215/2/13/2) and the University of Bristol provide core support for ALSPAC. Data collection is funded from a wide range of sources, which are detailed on the ALSPAC Web site: http://www.bristol.ac.uk/alspac/about/.
Disclosure: The authors have nothing to disclose.