-
PDF
- Split View
-
Views
-
Cite
Cite
Liliana C. Patiño, Kelly L. Walton, Thomas D. Mueller, Katharine E. Johnson, William Stocker, Dulama Richani, David Agapiou, Robert B. Gilchrist, Paul Laissue, Craig A. Harrison, BMP15 Mutations Associated With Primary Ovarian Insufficiency Reduce Expression, Activity, or Synergy With GDF9, The Journal of Clinical Endocrinology & Metabolism, Volume 102, Issue 3, 1 March 2017, Pages 1009–1019, https://doi.org/10.1210/jc.2016-3503
- Share Icon Share
Abstract
Bone morphogenetic protein (BMP)15 is an oocyte-specific growth factor, which, together with growth differentiation factor (GDF) 9, regulates folliculogenesis and ovulation rate. Multiple mutations in BMP15 have been identified in women with primary ovarian insufficiency (POI), supporting a pathogenic role; however, the underlying biological mechanism of many of these mutants remains unresolved.
To determine how mutations associated with ovarian dysfunction alter the biological activity of human BMP15.
The effects of 10 mutations in BMP15 on protein production, activation of granulosa cells, and synergy with GDF9 were assessed.
Sequencing of 35 patients with POI identified both an unrecognized BMP15 variant (c.986G>A, R329H) and a variant (c.581T>C, F194S) previously associated with the condition. Assessing expression and activity of these and 8 other BMP15 mutants identified: (1) multiple variants, including L148P, F194S, and Y235C, with reduced mature protein production; (2) three variants (R138H, A180T, and R329H) with ∼fourfold lower activity than wild-type BMP15; and (3) 3 variants (R68W, F194S, and N196K) with a significantly reduced ability to synergize with GDF9.
Mutations in BMP15 associated with POI reduce mature protein production, activity, or synergy with GDF9. The latter effect is perhaps most interesting given that interactions with GDF9 most likely underlie the physiology of BMP15 in the human ovary.
Primary ovarian insufficiency (POI) is defined as the acquisition of hypergonadotropic hypogonadism in women before the age of 40. These women present with amenorrhea and have elevated serum follicle-stimulating hormone (FSH) levels (>40 IU/L) on 2 separate occasions, measured at least 1 month apart, and parallel low estradiol. The POI incidence is ∼1:100 for women under 40 years of age and 1:1000 for women before age 30 (1, 2).
Many studies have examined the potential involvement of bone morphogenetic protein (BMP)15 in POI (3–9). BMP15 is an oocyte-specific member of the transforming growth factor (TGF)-β superfamily with major functions on the growth and differentiation of granulosa cells (GCs), which in turn supply the oocyte with the support necessary for future healthy embryo/fetal development (10–14). BMP15 is synthesized as a precursor molecule consisting of an N-terminal prodomain and a C-terminal mature domain. During synthesis, the prodomain directs folding and dimerization of the mature growth factor (15). Furin-like proteases cleave BMP15, which is secreted from the oocyte noncovalently associated with its prodomain. Extracellularly, prodomains most likely localize mature BMP15 in the vicinity of their target GCs. Unlike most TGF-β proteins, BMP15 lacks the cysteine residue that forms an intermolecular disulfide bond (16) and, therefore, functions as a noncovalent dimer. Following prodomain displacement, human BMP (hBMP)15 binds to complexes of type I (ALK6) and type II (BMPRII) receptors on the surface of GCs. Receptor binding leads to the activation of Smad1/5 transcription factors and the expression of genes, such as those (Ptx3, Has2, and Ptgs2) involved in cumulus cell expansion (17).
In mono-ovular species, BMP15 is coexpressed with the closely-related growth differentiation factor (GDF) 9 throughout most of oogenesis, and, hence, these factors should always be considered in combination. Indeed, there is ample evidence for synergistic interactions between BMP15 and GDF9 at genetic, biochemical, and functional levels (18). In 2004, McNatty and colleagues (19) proposed that a GDF9:BMP15 heterodimer may underlie the marked synergism between these 2 proteins. Recently, we (18) and 1 other group (17) have produced this heterodimer, which we named cumulin, and demonstrated its potent activity relative to GDF9 and BMP15 homodimers. The propensity of BMP15 to interact with GDF9 to form cumulin is likely to play an important role in the regulation of female fertility in mammals.
Support for the participation of BMP15 in ovarian pathologies came with the identification of 2 point mutations in sheep (V299D and Q291X) that caused increased ovulation rate and litter size in heterozygotes, but sterility, via a block in folliculogenesis at the primary stage, in homozygotes (20). Four other loss-of-function mutations have since been identified in sheep exhibiting this fecundity/POI phenotype (21–23). The exact mechanism underlying the effects of these BMP15 mutations in sheep remains to be determined, but is most likely due to a greater level of precocious follicular development (24), with more immature follicles with GCs acquiring luteinizing hormone receptivity and capable of ovulating at a smaller diameter (25, 26).
Subsequent screening for BMP15 mutations in women with POI has identified numerous heterozygous missense mutations not found in control women (3–9). The majority of the hBMP15 mutations are located in the prodomain (Table 1), which is distinct from the sheep in which the inactivating mutations are predominantly found in the mature domain. In this study, we use molecular modeling to map the location of 10 mutations on pro-BMP15. We describe the effects of these mutations on synthesis, secretion, biological activity, and synergy with GDF9. For these studies, we used luciferase reporter assays in the COV434 GC line and gene expression analysis in mouse primary mural GCs. Our findings provide evidence that BMP15 mutations found in women with POI can affect many aspects of BMP15 physiology, which, in turn, may disrupt ovarian function and female fertility.
Nucleotide Variation . | Amino Acid Variation . | Protein Domain . | No. of Cases . | No. of Controls . | Phenotype . | Reference . | Effect of Mutation on Expression/Activity/ Synergy with GDF9a . |
---|---|---|---|---|---|---|---|
c.202C>T | R68W | Pro | 3/466 | 0/397 | Secondary amenorrhea | (4,8) | Minor reductions in secretion and activity, poor synergy with GDF9 |
c.413G>A | R138H | Pro | 1/300 | 0/216 | Primary amenorrhea | (8) | Significant reductions in secretion and activity |
c.443T>C | L148P | Pro | 5/719 | 5/665 | Primary and Secondary amenorrhea | (4,7–9) | Major reduction in secretion |
c.538G>A | A180T | Pro | 19/891 | 1/810 | Primary and Secondary amenorrhea | (4,5,7,8) | Minor reductions in secretion, activity, and synergy with GDF9 |
c.581T>C | F194S | Pro | 2/85 | 1/214 | Secondary amenorrhea | (9), this study | Significant reduction in secretion, poor synergy with GDF9 |
c.588T>A | N196K | Pro | 1/202 | 0/197 | Secondary amenorrhea | (5) | Very poor synergy with GDF9 |
c.617G>A | R206H | Pro | 1/202 | 0/197 | Secondary amenorrhea | (5) | Minor reductions in activity and synergy with GDF9 |
c.631C>T | Q211X | Pro | 1/202 | 0/197 | Primary amenorrhea | (5) | Mature protein not produced |
c.704A>G | Y235C | Pro | 2 (familial case) | 0/120 | Primary amenorrhea | (3) | Significant reductions in secretion |
c.986G>A | R329H | Mature | 1/35 | NA | Secondary amenorrhea | This study | Significant reduction in activity and minor reduction in synergy with GDF9 |
Nucleotide Variation . | Amino Acid Variation . | Protein Domain . | No. of Cases . | No. of Controls . | Phenotype . | Reference . | Effect of Mutation on Expression/Activity/ Synergy with GDF9a . |
---|---|---|---|---|---|---|---|
c.202C>T | R68W | Pro | 3/466 | 0/397 | Secondary amenorrhea | (4,8) | Minor reductions in secretion and activity, poor synergy with GDF9 |
c.413G>A | R138H | Pro | 1/300 | 0/216 | Primary amenorrhea | (8) | Significant reductions in secretion and activity |
c.443T>C | L148P | Pro | 5/719 | 5/665 | Primary and Secondary amenorrhea | (4,7–9) | Major reduction in secretion |
c.538G>A | A180T | Pro | 19/891 | 1/810 | Primary and Secondary amenorrhea | (4,5,7,8) | Minor reductions in secretion, activity, and synergy with GDF9 |
c.581T>C | F194S | Pro | 2/85 | 1/214 | Secondary amenorrhea | (9), this study | Significant reduction in secretion, poor synergy with GDF9 |
c.588T>A | N196K | Pro | 1/202 | 0/197 | Secondary amenorrhea | (5) | Very poor synergy with GDF9 |
c.617G>A | R206H | Pro | 1/202 | 0/197 | Secondary amenorrhea | (5) | Minor reductions in activity and synergy with GDF9 |
c.631C>T | Q211X | Pro | 1/202 | 0/197 | Primary amenorrhea | (5) | Mature protein not produced |
c.704A>G | Y235C | Pro | 2 (familial case) | 0/120 | Primary amenorrhea | (3) | Significant reductions in secretion |
c.986G>A | R329H | Mature | 1/35 | NA | Secondary amenorrhea | This study | Significant reduction in activity and minor reduction in synergy with GDF9 |
Outcomes of this study.
Nucleotide Variation . | Amino Acid Variation . | Protein Domain . | No. of Cases . | No. of Controls . | Phenotype . | Reference . | Effect of Mutation on Expression/Activity/ Synergy with GDF9a . |
---|---|---|---|---|---|---|---|
c.202C>T | R68W | Pro | 3/466 | 0/397 | Secondary amenorrhea | (4,8) | Minor reductions in secretion and activity, poor synergy with GDF9 |
c.413G>A | R138H | Pro | 1/300 | 0/216 | Primary amenorrhea | (8) | Significant reductions in secretion and activity |
c.443T>C | L148P | Pro | 5/719 | 5/665 | Primary and Secondary amenorrhea | (4,7–9) | Major reduction in secretion |
c.538G>A | A180T | Pro | 19/891 | 1/810 | Primary and Secondary amenorrhea | (4,5,7,8) | Minor reductions in secretion, activity, and synergy with GDF9 |
c.581T>C | F194S | Pro | 2/85 | 1/214 | Secondary amenorrhea | (9), this study | Significant reduction in secretion, poor synergy with GDF9 |
c.588T>A | N196K | Pro | 1/202 | 0/197 | Secondary amenorrhea | (5) | Very poor synergy with GDF9 |
c.617G>A | R206H | Pro | 1/202 | 0/197 | Secondary amenorrhea | (5) | Minor reductions in activity and synergy with GDF9 |
c.631C>T | Q211X | Pro | 1/202 | 0/197 | Primary amenorrhea | (5) | Mature protein not produced |
c.704A>G | Y235C | Pro | 2 (familial case) | 0/120 | Primary amenorrhea | (3) | Significant reductions in secretion |
c.986G>A | R329H | Mature | 1/35 | NA | Secondary amenorrhea | This study | Significant reduction in activity and minor reduction in synergy with GDF9 |
Nucleotide Variation . | Amino Acid Variation . | Protein Domain . | No. of Cases . | No. of Controls . | Phenotype . | Reference . | Effect of Mutation on Expression/Activity/ Synergy with GDF9a . |
---|---|---|---|---|---|---|---|
c.202C>T | R68W | Pro | 3/466 | 0/397 | Secondary amenorrhea | (4,8) | Minor reductions in secretion and activity, poor synergy with GDF9 |
c.413G>A | R138H | Pro | 1/300 | 0/216 | Primary amenorrhea | (8) | Significant reductions in secretion and activity |
c.443T>C | L148P | Pro | 5/719 | 5/665 | Primary and Secondary amenorrhea | (4,7–9) | Major reduction in secretion |
c.538G>A | A180T | Pro | 19/891 | 1/810 | Primary and Secondary amenorrhea | (4,5,7,8) | Minor reductions in secretion, activity, and synergy with GDF9 |
c.581T>C | F194S | Pro | 2/85 | 1/214 | Secondary amenorrhea | (9), this study | Significant reduction in secretion, poor synergy with GDF9 |
c.588T>A | N196K | Pro | 1/202 | 0/197 | Secondary amenorrhea | (5) | Very poor synergy with GDF9 |
c.617G>A | R206H | Pro | 1/202 | 0/197 | Secondary amenorrhea | (5) | Minor reductions in activity and synergy with GDF9 |
c.631C>T | Q211X | Pro | 1/202 | 0/197 | Primary amenorrhea | (5) | Mature protein not produced |
c.704A>G | Y235C | Pro | 2 (familial case) | 0/120 | Primary amenorrhea | (3) | Significant reductions in secretion |
c.986G>A | R329H | Mature | 1/35 | NA | Secondary amenorrhea | This study | Significant reduction in activity and minor reduction in synergy with GDF9 |
Outcomes of this study.
Materials and Methods
Patients
Thirty-five patients presenting with at least 6 months of amenorrhea before their 40th birthday, a normal karyotype and serum FSH of >40 IU/L, and low estradiol <20 pg/ml (on at least 2 occasions) attended the Genetics and Genomics Research Center at the Universidad del Rosario (Bogotá, Colombia). All underwent BMP15 mutation screening using Sanger sequencing, as previously described (7). All clinical steps were approved by Universidad del Rosario’s Ethic Committee and were conducted in line with the Declaration of Helsinki. Patients provided informed consent.
Production of BMP15 mutants
The construction of the hBMP15-FLAG/pcDNA3.1 expression vector has been previously described (27). Mutations were introduced into full-length hBMP15-FLAG using the GeneArt Site-Directed Mutagenesis System (Life Technologies, Carlsbad, CA), following the manufacturer’s instructions. For each construct, the mutated region was confirmed by DNA sequencing. Wild-type and mutant hBMP15 proteins were produced by transient transfection in HEK-293T cells using Lipofectamine 2000 (Invitrogen, Carlsbad, CA). Briefly, HEK-293T cells were plated at 4 × 105 cells/well in a 12-well plate. Wild-type or mutant hBMP15 DNA (2.5 µg) was combined with Lipofectamine 2000 for 20 minutes, according to the manufacturer’s instructions. DNA-Lipofectamine complexes were added directly to cells and incubated in OPTI-MEM medium for 48 hours at 37°C in 5% CO2. Conditioned media and cell lysates were collected, and comparable protein levels were confirmed using the BCA Protein Assay Kit (Thermo Fisher Scientific, Waltham, MA). Conditioned media was concentrated 10-fold using Nanosep microconcentrators (10 kDa; Pall Life Sciences, Port Washington, NY). BMP15 expression in media and lysates was assessed by Western blotting of reduced samples on 10% sodium dodecyl sulfate–polyacrylamide gel electrophoresis gels (Bio-Rad, Hercules, CA) transferred onto ECL Hybond membranes (GE Health Care, Buckinghamshire, UK). Blots were probed with BMP15 mAb28 (Oxford Brookes University, Oxford, UK; 1:5000), or anti–glyceraldehyde-3-phosphate dehydrogenase (Santa Cruz Biotechnology, Dallas, TX; 1:10,000) as an internal control, followed by horseradish peroxidase–conjugated anti-mouse IgG (1:10,000) with detection using Lumi-light chemiluminescence reagents.
Purification of recombinant BMP15 variants
Large-scale production of wild-type and mutant hBMP15 proteins was achieved following transient transfection in HEK-293T cells using polyethylenimine (PEI)-MAX (Polysciences, Warrington, PA). Briefly, HEK-293T cells were plated at 8 × 105 cells/well in 6-well plates. Wild-type or mutant hBMP15 DNA (5 µg) was combined with PEI for 10 minutes. DNA-PEI complexes were added directly to cells and incubated in OPTI-MEM medium for 4 hours at 37°C in 5% CO2 before replacing with fresh OPTI-MEM medium. After 24 hours, the medium was replaced with production media [Dulbecco’s modified Eagle medium (DMEM):F12 medium containing l-glutamine, 0.02% bovine serum albumin (BSA), 0.01% heparin (Sigma-Aldrich, St. Louis, MO)] for 48 hours. Conditioned media from the BMP15 variant-transfected cells was centrifuged, concentrated (Centricon Plus-70; Millipore, Billerica, MA), and then resuspended in Tris-buffered saline, prior to immunoaffinity purification using anti-FLAG M2 affinity agarose gel (Invitrogen). FLAG-tagged BMP15 variants were eluted from the agarose using 3× FLAG peptide (Sigma-Aldrich) diluted in Tris-buffered saline/0.05% BSA. Western blotting was used to assess the amount of purified protein.
GC bioassays
Initially, hBMP15 variants were compared for their ability to stimulate a luciferase response in a human granulosa tumor cell line (COV434). In brief, cells were plated at 1 × 105 cells/well in 48-well plates in complete medium (DMEM supplemented with 10% fetal calf serum) at 37°C in 5% CO2. After 24-hour incubation, cells were transfected (250 ng/well) with a BMP response element–luciferase reporter construct (27) using Lipofectamine 3000 (Invitrogen), according to the manufacturer's instructions, for 24 hours. The cells were treated with increasing doses of FLAG-purified BMP15 variants for 16 hours. The medium was then aspirated; the cells were solubilized in solubilization buffer [25 mm glycylglycine (pH 7.8), 15 mm MgSO4, 4 mm EGTA, 1% Triton X-100, and 1 mm dithiothreitol]; and luciferase reporter activity was measured, as previously described (27). Subsequently, the BMP15 variants were tested for their ability to synergize with GDF9 (18). COV434 cells were plated as above and transfected with the GDF9-responsive A3-luciferase reporter construct (50 ng/well). After 24 hours, cells were treated with GDF9 (1.2 to 33 ng/ml) ± equal concentrations of BMP15 variants (1.2 to 33 ng/ml), and luciferase activity was determined, as described earlier.
Reverse transcription polymerase chain reaction
Mural GC were collected from 21- to 26-day-old C57BL/6 female mice 46 hours after intraperitoneal administration of gonadotropin priming. Mice were maintained in accordance with the Australian Code for the Care and Use of Animals for Scientific Purposes and with the approval of the University of New South Wales Animal Ethics Committee. Mural GC (1 × 105 live cells per 24-plate well) were cultured for 18 hours in bicarbonate-buffered DMEM (Gibco Life Technologies, Grand Island, NY) supplemented with 10% fetal bovine serum (Sigma-Aldrich). Cells were then treated for 24 hours with fresh media containing 3 mg/ml BSA (Sigma-Aldrich), recombinant human FSH (50 mIU/ml, Puregon; Organon, Oss, The Netherlands), plus the following treatments, as indicated: recombinant GDF9 (30 ng/mL); BMP15 variants (30 ng/mL); or recombinant GDF9 plus BMP15 variants (30 ng/mL each). RNA extraction, reverse transcription, and quantitative polymerase chain reaction were performed, as previously described (28), using their reported primers; however, polymerase chain reactions were performed on a Roche LightCycler 480, and results are expressed as raw gene expression levels relative to the geometric mean of 2 housekeeping genes (Mrpl19 and Ppia) using the 2−(∆∆CT) method. Data are means ± standard error of the mean of 4 replicate experiments.
Molecular modeling
Homology modeling of the hBMP15 proprotein complex was performed manually employing the software package Quanta2008 (MSI Accelrys, San Diego, CA) and using the structure of pro-BMP9 as template (29). The sequence alignment of TGF-β members as published by Mi et al. (29) was used, and residues differing between the template hBMP9 and the model target hBMP15 were exchanged using the tools ProteinDesign and X-BUILT of the software package Quanta2008. Insertions and deletions as present in the sequence alignment were performed manually; the backbone torsion angles of affected residues were maintained in the allowed regions of the Ramachandran plot. This initial three-dimensional (3D) model was subsequently refined to exhibit good backbone and side chain geometries. Van der Waals overlap from manual residue exchanges was removed (or minimized), first employing rotamer searches for the side chains of affected residues using the X-BUILT tool of Quanta2008; thereafter, energy minimization was performed using only geometrical energy terms and employing the CHARMM27 force field (MSI Accelrys). To maintain the template’s backbone geometry as far as possible, energy minimization was applied with a stepwise protocol, first with all backbone atoms fully restrained and only allowing side chain atoms to move (harmonic positional restraints with a force constant of 25 kcal mol−1 Å−2 were used) until atom movements were minimal. Thereafter, harmonic positional restraints were applied for all amino acid exchanges involving glycine and proline residues, and a short energy minimization was performed with all other backbone atoms kept fixed. Finally, the backbone torsion angles of residues occupying energetically disfavored regions in the Ramachandran plot were manually modeled to acquired torsion angles in allowed regions. The final model consists of residues Ser66 to Glu258 (hBMP15 prodomain) and Asn288 to Arg392 (hBMP15 mature region) and exhibits good side chain and backbone geometry. Residues 19 to 65 (N terminus, including the proposed helix α1 as observed in the proprotein complex of TGF-β1) and 259 to 287 (linker region between the prodomain and the mature region, including the furin protease cleavage site) could not be modeled due to lack of template structure. Figures were produced using the software Pymol (Schroedinger, San Diego, CA).
Statistical analysis
For messenger RNA expression data, differences between means were assessed by 1-way analysis of variance, followed by Tukey’s multiple comparison test. COV434 bioassay data are the mean ± standard deviation of triplicate determinations from representative experiments, relative to an adjusted value of 1.0 for the mean of the control wells. The experiments were repeated at least 3 times, with each treatment applied to 3 replicate wells in each experiment. The data were analyzed using the Prism program (version 5.0; GraphPad Software, San Diego, CA). P < 0.05 was considered statistically significant.
Results
Direct sequencing
The analysis of the BMP15 coding region from 35 patients revealed 2 mutations. The first corresponded to a previously reported heterozygous mutation c.581T>C leading to a F194S change in the prodomain (9). The second was a heterozygous mutation c.986G>A leading to a R329H change in the mature peptide (Table 1).
Molecular modeling of hBMP15 variants
The 9 previously identified BMP15 mutations that were characterized in this study are located in the prodomain, whereas the mutation (R329H) is situated in the mature domain [Fig. 1(A)]. Recently, the crystal structures of the proprotein complexes of TGF-β1, BMP9, and activin A were determined (15, 29, 30). Amino acid sequence homology suggests that the architecture of the BMP15 proprotein complex is similar to that of pro-BMP9, rather than resembling the structures of pro–TGF-β1 and proactivin A, and thus our model of the BMP15 proprotein complex is based on the structure of pro-BMP9 (Protein Data Bank ID 4YCG) [Fig. 1(B) and 1(C)] (29). All 10 BMP15 mutations were located in structurally important regions of the molecule [Fig. 1(D) and 1(E)]. The mutation R68W is situated at the start of helix α2 of the prodomain, a region that possibly contacts the mature domain of BMP15 during synthesis, and, thus, this exchange could lead to misfolded BMP15 proprotein. The other mutations [ i.e., R138H (β3), L148P (β4 loop), A180T (β5β6 loop), F194S (helix α4), N196K (α4β7 loop), R206H (β7), and Y235C (β10)], are located in the β-sheets, α-helices, or connecting loops of the prodomain arm, a highly structured region critical for controlling the synthesis, secretion, and activity of the mature ligand (15, 29, 30). Within the mature domain, the mutation R329H may cause displacement of the prodomain and hence affect activation, as it is in close proximity to helix α5 of the prodomain. Alternatively, due to its location in the so-called prehelix loop, which was shown to be essential for type I receptor interaction and recognition (31), the mutation could also impair type I receptor binding and hence decrease BMP15 activity (27). Variant Q211X harbors a nonsense mutation within the structured core of the prodomain that would result in an incomplete prodomain, and, hence, this mutation would impede protein synthesis of this mutant.
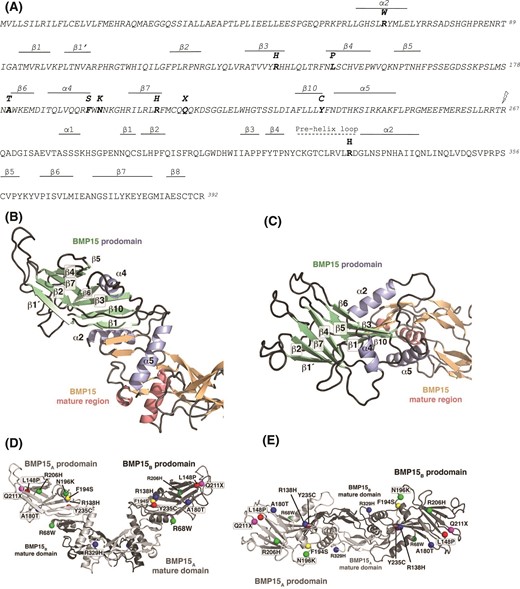
Molecular modeling of pro-BMP15 (O95972). (A) Sequence of hBMP15 with the prodomain, indicated by italics. Secondary structure elements (β-sheets and α-helices) are depicted above the sequence. Mutations associated with POI are indicated (bold), as is the cleavage site between the pro- and mature domains (lightning bolt). (B–E) 3D homology model of hBMP15 proprotein complex based on the crystal structure of the proprotein complex of hBMP9 (Protein Data Bank ID 4YCG). (B) Cartoon representation of the BMP15 proprotein homology model to highlight the secondary structure elements of the prodomain (colored in green and light blue); the mature region is indicated with β-strands and α-helices colored in orange and light red, respectively. (C) As in (B), but rotated clockwise around the x-axis by 90°. The denotation of the secondary structure elements follows the definition as defined in Mi et al. (29); β-strands and helices missing in the BMP15 proprotein complex were either not present in the BMP9 structure template (e.g., helix α1) or are absent in BMP9 (and hence BMP15) due to structural differences between the prodomain architectures of BMP9 and TGF-β1, the latter of which was used to originally define the folding motif. (D) Cartoon representation of the 3D model of the BMP15 proprotein complex with the 10 mutations presented in this study highlighted. To indicate the location of the amino acid exchange, the Cα atom of the respective amino acid is shown as a sphere. The different mutations are colored as follows: mutations affecting protein secretion are marked in red, mutations leading to a decreased BMP15 signaling are shown in blue, and mutations resulting in a reduction of synergism with GDF9 are colored in green. The position of the nonsense mutation Q211X resulting in a premature stop in the prodomain is highlighted in magenta. As the BMP15 variant harboring the mutation F194S not only exhibits a significantly lowered protein yield, but also shows a decreased synergism with GDF9, it is marked in yellow. (E) As in (D), but rotated clockwise around the x-axis by 90°.
Effect of mutations on the synthesis and secretion of BMP15
To evaluate the impact of the 10 mutations in hBMP15 on protein synthesis and secretion, the mutations were introduced into hBMP15 cDNA by in vitro site-directed mutagenesis. HEK-293T cells were then transiently transfected with expression vectors for wild-type hBMP15 or the variant forms. The conditioned media and cell lysates were collected, assayed for total protein content, and analyzed by Western blot (Fig. 2). Using BMP15 mAb28, BMP15 variants (except Q211X) were detected in monomeric precursor (55-kDa) and mature (17-kDa) forms. Mutations L148P, F194S, and Y235C resulted in major reductions in BMP15 expression (>60%), whereas variant R138H was also expressed at a significantly (P < 0.05) reduced level compared with wild-type BMP15 (Fig. 2). An analysis of cell lysates indicated that the Q211X mutation abrogated BMP15 precursor production, whereas the Y235C mutation significantly reduced precursor formation [Fig. 2(A)]. For the other mutants, variation in BMP15 secretion was not due to a defect in synthesis because intracellular BMP15 precursor levels were similar [Fig. 2(A)]. Glyceraldehyde-3-phosphate dehydrogenase was included as a loading control in these experiments.
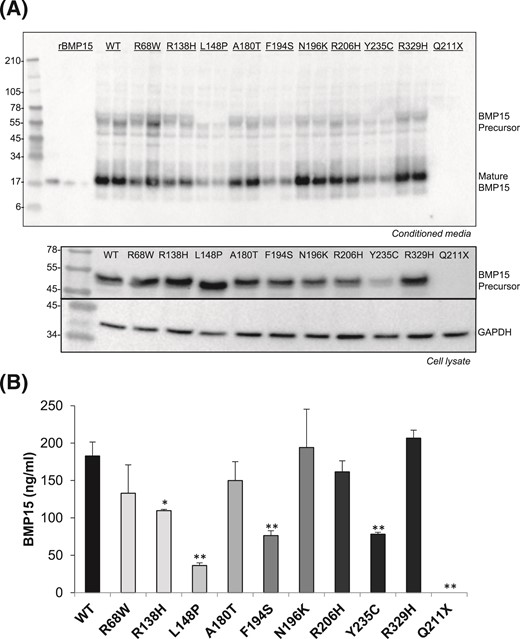
Effects of POI BMP15 mutations on growth factor synthesis and secretion. (A) Mutations were substituted into hBMP15 using in vitro site-directed mutagenesis. To determine the effects of the mutations on BMP15 production, conditioned media and cell lysates from HEK-293T cells transfected with either wild-type (WT) or mutant constructs were analyzed by Western blot. Samples were detected under reducing conditions using BMP15 mAb28 specific for the mature domain. The 55-kDa BMP15 precursor and 18-kDa mature peptide are shown. For cell lysates, blots were probed with antibodies against BMP15 or glyceraldehyde-3-phosphate dehydrogenase (loading control). Recombinant BMP15 (45, 15, and 5 ng in lanes 1, 2, and 3, respectively) was used to quantify mature peptide. (B) Densitometric quantification of wild-type and mutant BMP15 mature peptide using the Bio-Rad ChemiDoc MP system. Transfections and Western blots were performed in 4 separate experiments, with representative images shown. The images were analyzed using Image Laboratory software (Bio-Rad). The stars indicate significant differences at P < 0.05 (*) or at P < 0.01 (**) compared with wild-type BMP15.
Pro- and mature domain mutations affect the bioactivity of BMP15
We have previously shown mature BMP15 is secreted from cells noncovalently associated with its prodomain (27). Therefore, we performed large-scale production of BMP15 variants in HEK-293T cells and used FLAG-affinity chromatography to isolate pro-BMP15 complexes (Supplemental Fig. 1). The final concentrations of purified pro-BMP15 variants ranged from 497 to 1493 ng/ml. To examine Smad1/5 signaling by the different BMP15 proprotein complexes, we conducted transcriptional reporter assays using the human GC line, COV434, as previously reported (18). Wild-type pro-BMP15 dose dependently activated Smad1/5 signaling in COV434 cells, producing high levels of BMP response element–Luc reporter activity at doses >1 ng/ml (Fig. 3). Interestingly, 2 of the poorly secreted variants, L148P and Y235C, consistently induced higher maximal Smad1/5 responses than wild-type pro-BMP15 [Fig. 3(A)], suggesting that these mutations weakened promature interactions and enabled BMP15 to more readily engage its signaling receptors. Variants R68W, F194S, N196K, and R206H displayed similar activity to wild-type BMP15 [Fig. 3(B)], whereas variants R138H, A180T, and R329H were each ∼fourfold less potent [Fig. 3(C)].
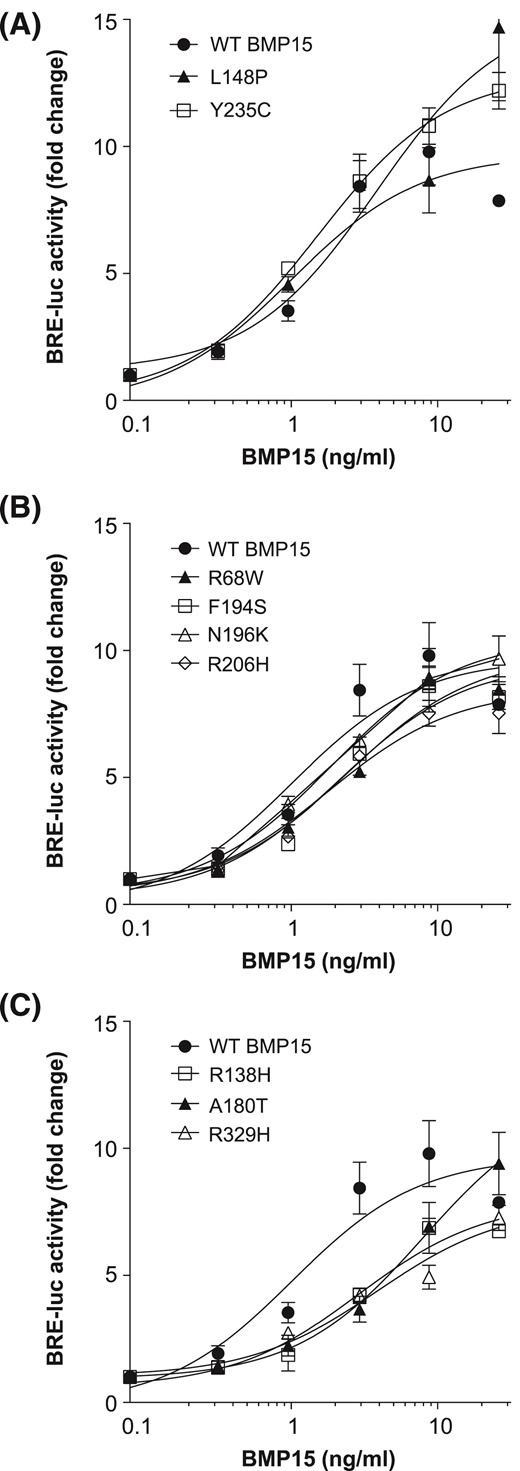
Bioactivity of pro-BMP15 variants. COV434 human GCs, transfected with a Smad1/5/8-responsive luciferase reporter, were stimulated with increasing concentrations (0.3 to 12 ng/ml) of FLAG affinity-purified hBMP15 variants. Luciferase activity was measured and based on activity at 10 ng/ml; BMP15 variants were separated into (A) high, (B) medium, and (C) low responders. Data are the mean ± standard deviation of triplicate determinations from representative experiments, relative to an adjusted value of 1.0 for the mean of the control wells. The experiments were repeated 4 times. WT, wild-type.
Prodomain mutations affect BMP15 synergy with GDF9
Recently, we showed that BMP15 and GDF9 form a heterodimer, termed cumulin, which potently activates the alternate Smad2/3 pathway (18, 32). In this study, we examined whether BMP15 variants had altered ability to synergize with recombinant human GDF9 (mature region homodimer) in a Smad2/3-dependent luciferase assay. At the low doses tested, GDF9 weakly stimulated A3-luciferase activity, but this response was enhanced >20-fold in the presence of wild-type BMP15 [Fig. 4(A)]. BMP15 alone did not activate the Smad2/3 pathway (data not shown). Two variants, R138H and L148P, synergized normally with GFD9 [Fig. 4(A)], whereas 4 other variants, A180T, R206H, Y235C, and R329H, displayed a ∼twofold reduction in their synergistic response [Fig. 4(B)]. Interestingly, 3 variants, R68W, F194S, and N196K, exhibited significantly reduced synergy with GDF9 [Fig. 4(C)], indicating that these mutations probably affect cumulin formation.
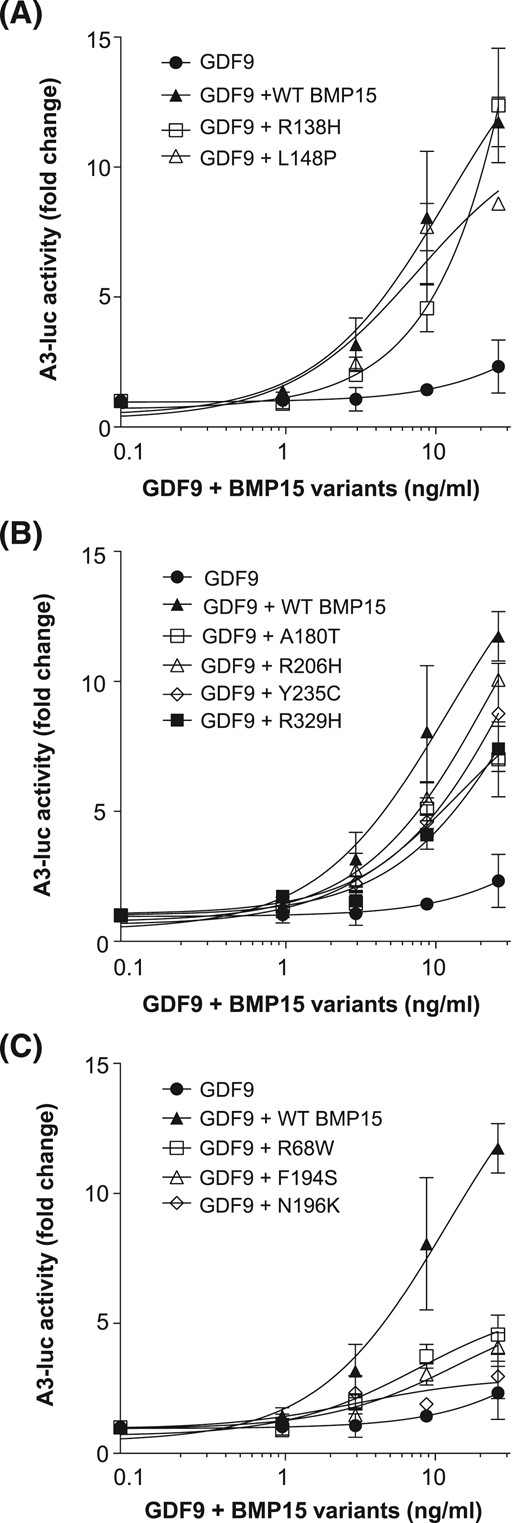
Mutations in the BMP15 prodomain affect synergy with GDF9. COV434 human GCs, transfected with a Smad2/3-responsive luciferase reporter, were stimulated with equal concentrations (1 to 12 ng/ml) of recombinant human GDF9 and FLAG affinity-purified hBMP15 variants. Luciferase activity was measured, and BMP15 variants were separated into those that demonstrated (A) high, (B) moderate, or (C) minimal synergy with GDF9. Data are the mean ± standard deviation of triplicate determinations from representative experiments, relative to an adjusted value of 1.0 for the mean of the control wells. The experiments were repeated 3 times. WT, wild-type.
One of the physiological roles of oocyte-secreted BMP15 and GDF9 is to direct GC differentiation toward the cumulus cell phenotype (10, 33), which can be characterized by expression of genes required for cumulus cell mucification (Ptx3, Tnfaip6, and Ptgs2) (17). Using this system, we confirmed the limited capacity of variants R68W, A180T, F194S, and N196K to synergize with GDF9 (Fig. 5).
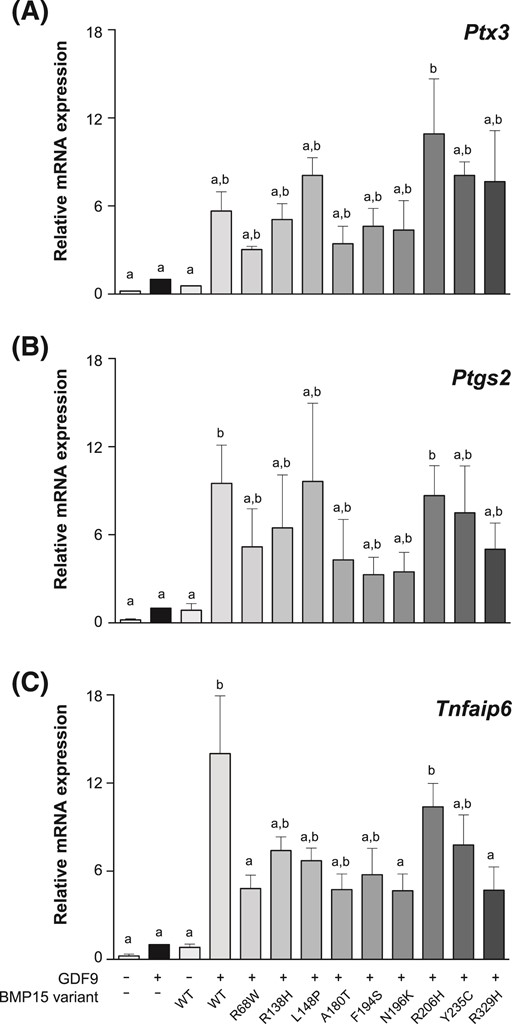
Synergistic activity of GDF9 and BMP15 variants on the expression of genes associated with cumulus cell differentiation. Mouse primary mural GCs were treated for 24 hours with fresh media containing recombinant human FSH plus recombinant GDF9, BMP15 variants, or recombinant GDF9 plus BMP15 variants. (A) Ptx3, (B) Ptgs2, and (C) Tnfaip6 messenger RNA expression were evaluated. All of the proteins were added at 30 ng/ml. Data are the mean ± standard deviation of triplicate determinations from representative experiments. The experiments were repeated 4 times. The bars (means ± standard error of the mean) with no common lowercase letters indicate significant differences at P < 0.05 within the same graph. WT, wild-type.
Discussion
BMP15 is an oocyte-specific growth factor with central roles in mammalian reproduction, regulating species-specific fecundity, ovarian follicular somatic cell differentiation, and oocyte quality (34). Previously, >10 mutations in BMP15 have been associated with POI (3–9), and we identified an additional variant in the BMP15 gene (c.986G>A, R329H), following direct sequencing in 35 women with this condition. Although several studies have provided some molecular and functional characterization of the detected variants (4, 5, 7–9), they have done so without reference to the structure of pro-BMP15, or to BMP15’s synergistic interactions with GDF9. In this study, we modeled the pro-BMP15 structure, based on the recently published crystal structure of pro-BMP9 (29). In this model, mapping localized the various BMP15 mutations (R68W, R138H, L148P, A180T, F194S, N196K, R206H, Q211X, and Y235C) to structurally important regions of the prodomain, or the type I–binding interface of the mature domain (R329H). In vitro analysis of these variants identified mutations that: (1) suppressed mature BMP15 secretion; (2) reduced BMP15 activity; or (3) inhibited BMP15 synergy with GDF9.
The ability of BMP15 to synergize with GDF9 to regulate GC growth and differentiation is one of the emerging themes in reproductive biology (17, 18). Recently, we showed that this synergism is mediated via increased phosphorylation of Smad2/3 (18), indicating that BMP15 activates latent human GDF9. Mechanistically, coaddition or coexpression of BMP15 and GDF9 results in the formation of the heterodimer, cumulin, which elicits an enhanced signaling response due to altered type I receptor affinity and/or specificity (18). Extracellular, posttranslational cumulin formation is possible because, unusual among TGF-β proteins, BMP15 and GDF9 lack the cysteine residues that covalently dimerize the individual subunits (16). In this study, we identified three BMP15 prodomain variants (R68W, F194S, and N196K) that displayed significantly (five- to 15-fold) reduced synergy with GDF9, despite their signaling via the BMP-Smad1/5 pathway being unaffected. A similar lack of synergy is observed when a covalently stabilized version of BMP15 is combined with wild-type GDF9 (18). One explanation for these findings might be that the homodimers of these 3 BMP15 variants do not readily dissociate and, thereby, limit cumulin formation in the presence of wild-type GDF9 by maintaining BMP15 in a homodimeric state [see also (35)]. These variant BMP15 homodimers could still activate the Smad1/5 pathway, but could not potentiate GDF9 activation of the Smad2/3 pathway. The two neighboring mutations F194S and N196K are located at the C-terminal end of helix α4. Phe194 is in direct contact with the hydrophobic core of 4-stranded β-sheets, and its replacement by a hydrophilic small serine residue possibly destabilizes the hydrophobic core of the arm domain. Asn196 is in the loop between helix α4 and β-strand 7 and potentially interacts with further residues in this loop as well as of the neighboring loop between β-strands 2 and 3, thereby affecting the location of these two loops. The mutation R68W is located at the N terminus of helix α2, which directly contacts the convex side of the finger region of the mature growth factor domain and blocks the type II receptor binding site. The exchange of the charged arginine residue by a large hydrophobic tryptophan most likely affects folding of the α-helix and thus alters homo- as well as heteromeric interactions between the prodomain and the mature growth factor region. Thus, the location and type of amino acid exchange in these 3 variants potentially lead to local structural rearrangements in the prodomain, which alter the interactions between the prodomain and the mature region and attenuate heterodimer formation. The potential pathogenic mechanism of these mutations in POI patients has important implications for ovarian physiology. To date, cumulin formation has remained an in vitro phenomenon because we lack the tools to definitively identify this noncovalent heterodimer in vivo. However, the identification of POI-associated BMP15 variants with disrupted synergy with GDF9, but normal expression and activity (e.g., N196K), provides strong support for a role of cumulin in vivo.
As previously described (4, 5, 7, 8), a decrease in BMP15 production also appears sufficient to disrupt ovarian function. Western analysis of the 10 BMP15 variants indicated that most were expressed at levels significantly lower than the wild-type protein. Other than variant Q211X, which introduces a premature stop codon within the prodomain, variants L148P, F194S, and Y235C were the most disruptive for BMP15 secretion. With reference to our pro-BMP15 model, Leu148 is located at the N terminus of β-strand 4 with direct contact to Cys150, which forms an intrachain disulfide bond with Cys209. Mutation of Leu148 to Pro would not only disrupt the conformation of the β4-sheet, but also potentially impairs the formation of the stabilizing disulfide bond. In the absence of the intrachain disulfide bond, the reduced stability of the prodomain might result in enhanced degradation of partially unfolded BMP15 protein during endoplasmic reticulum quality control, and it most likely also lowers correct folding of mature BMP15. A similar mechanism most likely underlies the reduction in secretion of variant Y235C. Tyr235 is in β-strand 10, a region highly conserved across TGF-β proteins. The strand β10 of the prodomain is in close proximity to helix α2, the latter of which makes important contacts with and blocks type II receptor binding to the mature domain. The conserved hydrophobic residues on β-strand 10, which mediate the interaction with respective hydrophobic residues on helix α2, are located in part at the solvent-accessible protein surface. Unpaired solvent-accessible cysteine residues, as would be introduced by the mutation Y235C, are known as degradation signals in endoplasmic reticulum quality control during protein synthesis and might thus significantly lower protein secretion from this compartment (36, 37).
Based on our in vitro analysis, women heterozygous for these and other disruptive mutations would be expected to have between 15% and 40% reduction in their BMP15 levels. Interestingly, a link between reduced BMP15 levels and enhanced ovulation rate is well established in sheep. Ewes heterozygous for inactivating mutations in BMP15 (e.g., V299D, C321Y, and S367I) have increased fecundity (20). Although the exact mechanism underlying this enhanced prolificacy has not been resolved, it is postulated that reduced BMP15 signaling impairs GC proliferation, but enhances luteinizing hormone sensitivity of follicles in the ovaries of these heterozygous ewes (25, 26), leading to numerous, small follicles being selected and ovulated (24). If a reduction in BMP15 levels causes a similar increase in ovulation rate in women, then ovarian reserve may be more rapidly exhausted.
A final set of variants (R138H, A180T, and R329H) had ∼fourfold lower activity in a BMP-responsive luciferase assay and displayed either relatively normal (R138H) or slightly reduced (A180T and R329H) synergy with GDF9. The reduced activity of the variant R329H was of particular interest. Arg329 is located at the type I receptor-binding interface of hBMP15 and is equivalent with Arg438 in human GDF5, which was shown to determine type I receptor specificity (31, 38). Previously, we have shown that Arg329 and the adjacent Asp330 are responsible for the high activity of hBMP15, relative to murine (Pro329/Tyr330) and ovine (His329/Tyr330) BMP15 (27). Therefore, it was not surprising that mutation of Arg329 to the histidine found in sheep would significantly reduce BMP15 activity. The reduced activity of the R138H variant was also supported by a previous study (8). In contrast, the A180T variant has been described as a polymorphism devoid of functional significance (8). The reason we uncovered subtleties in activity for this variant that were not apparent in earlier studies is most likely due to the fact that we produced and purified each BMP15 variant and tested them at matching concentrations in a range of assays.
In conclusion, this study provides molecular and functional characterization of 10 hBMP15 mutations identified in women with POI. The findings support the concept that any decrease in BMP15 secretion, activity, or synergy with GDF9 is capable of disrupting ovarian function and female fertility.
Abbreviations:
- 3D
3-dimensional
- BMP
bone morphogenetic protein
- BSA
bovine serum albumin
- DMEM
Dulbecco’s modified Eagle medium
- FSH
follicle-stimulating hormone
- GC
granulosa cell
- GDF
growth differentiation factor
- hBMP
human BMP
- PEI
polyethylenimine
- POI
primary ovarian insufficiency
- TGF
transforming growth factor
Acknowledgments
This work was supported by National Health and Medical Research Council Grants 1078879 and 1062762; Universidad del Rosario Grant CS/ABN062/GENIUROS 016; and Colciencias Project/Grant 122265741115. A scholarship from Colombian Administrative Department of Science, Technology, and Innovation (Colciencias Grant 617) supports L.C.P. A Victorian Cancer Agency Early Career Seed Grant supports K.L.W., and Australian postgraduate awards support K.E.J. and W.S.
Disclosure Summary: The authors have nothing to disclose.
References
Author notes
Address all correspondence and requests for reprints to: Craig A. Harrison, Department of Physiology, Monash University, Clayton VIC 3800 Australia. E-mail: [email protected].