-
PDF
- Split View
-
Views
-
Cite
Cite
Gloria Mazzali, Francesco Fantin, Elena Zoico, Anna Sepe, Clara Bambace, Silvia Faccioli, Martina Pedrotti, Francesca Corzato, Vanni Rizzatti, Giuseppe Faggian, Rocco Micciolo, Saverio Cinti, Francesco Santini, Mauro Zamboni, Heart Fat Infiltration In Subjects With and Without Coronary Artery Disease, The Journal of Clinical Endocrinology & Metabolism, Volume 100, Issue 9, 1 September 2015, Pages 3364–3371, https://doi.org/10.1210/jc.2015-1787
- Share Icon Share
Fat may accumulate around the heart in epicardial adipose tissue or inside the heart as lipid droplets (LDs).
To compare myocardial steatosis between subjects with and without coronary artery disease (CAD and non-CAD) and to identify which cells contain LDs.
Body mass index, waist circumference, glucose, insulin, homeostasis model assessment index, leptin, adiponectin, and high-sensitivity C-reactive protein were evaluated in CAD and non-CAD subjects. Biopsies were collected from right atrial myocardium. Immunohistochemistry for perilipin (PLIN) 1 and 2 was used to characterize LDs and their localization in adipocytes or myocardial cells, respectively. Cardiomyocytes apoptosis and hypoxia inducible factor 1 alpha were obtained in a subgroup of subjects.
The study took place in a hospital.
Male subjects consecutively undergoing elective cardiac surgery either for coronary bypass grafting (CAD, n = 23) or for valve replacement (non-CAD, n = 18).
The study was designed to compare myocardial steatosis between subjects with and without coronary artery disease.
PLIN1 and PLIN2 resulted significantly higher in CAD than in non-CAD subjects, as did apoptosis. PLIN1 was positively associated with circulating leptin, high-sensitivity C-reactive protein, and apoptosis, and negatively with adiponectin. PLIN2 was positively associated with body mass index, waist circumference, and leptin and negatively with adiponectin. After taking into account the absence/presence of hypertension, diabetes, and CAD/non-CAD, adiponectin was negatively associated with PLIN1 (r2 = 0.532); waist circumference and adiponectin were associated with PLIN2 (r2 = 0.399).
Myocardial steatosis is greater in CAD than non-CAD subjects, depending on both metabolically active adipocytes interspersed among cardiomyocytes and higher fat deposition inside cardiomyocytes; serum adiponectin and waist circumference are independent predictors of myocardial steatosis.
There has been great interest in the past years on adiposity of the heart. Studies have shown that fat may accumulate around the heart in the epicardial adipose tissue or inside the heart (1, 2). In the presence of diabetes, obesity, and metabolic syndrome (1, 3–5), there is an increased amount of epicardial adipose tissue as well as stored triglycerides in the myocardium.
Accumulation of fat inside the heart, or so-called myocardial steatosis, has been observed in experimentally induced myocardial infarction in mice (6) as well as after hypoxic treatment in HL-1 cardiomyocytes, showing that hypoxia determines a shift from oxidation of fatty acids toward glycolysis (6). Mature adipose tissue within myocardial infarction has been also described (7, 8). It has been proposed that accumulation of fat inside the heart may lead to cellular dysfunction, cell death, and, eventually, organ dysfunction, in a process termed lipotoxicity. In the pathogenesis of this process, hypoxia phenomena as well as myocardial apoptosis have been suggested as relevant steps (9–11).
In humans, myocardial steatosis has been quantified noninvasively by using magnetic resonance spectroscopy, which allows for the evaluation of triglyceride content in the myocardium (10), or invasively in myocardial biopsy by evaluating lipid droplets (LDs) in histological sections using different types of staining (7, 8). LDs are intracellular organelles that store neutral lipids within most cells, such as, for example, adipocytes and cardiac and muscle cells (12–15); however, both of these noninvasive and invasive methods do not permit the characterization of LDs.
Recently, it has been shown that there are many proteins on the surface of LDs, and that these proteins vary with cell type (15). Thus, evaluation of the perilipin (PLIN) family, the most important proteins associated with LDs, of which five are known, may lead to the identification of which cells store LDs (12–15). PLIN1 has been shown to be mainly expressed in LDs of adipocytes, whereas PLIN2 is expressed in LDs of several other cells, such as muscle and cardiac cells (14, 15).
To the best of our knowledge, no studies have characterized intramyocardial fat in humans by using PLIN evaluation. The aims of this study were to compare the amount of myocardial steatosis between subjects with and without coronary artery disease (CAD) as well as to characterize myocardial steatosis and its predictors. PLIN1, expressed specifically in adipocytes (14), was used to verify the presence of adipocytes inside the myocardium; PLIN2 was used to test triglyceride deposition into cardiomyocytes.
Materials and Methods
A total of 41 male subjects undergoing elective cardiac surgery either for coronary bypass grafting (CAD, n = 23) or for valve replacement (non-CAD, n = 18) were included in the study. Clinical records were collected prospectively. In the CAD group, 14 patients had type 2 diabetes, whereas in the non-CAD group three patients had type 2 diabetes. Patients with liver disease, kidney, or cancer diseases were excluded from the protocol study, as were subjects with a weight loss greater than 3% in the previous 6 months. No subjects were regularly involved in physical exercise. As has recently been shown, sex dimorphism in adipose tissue function and characteristics exists (16), so to avoid a confounding factor, women were not enrolled in this study.
Written informed consent was obtained from each participant and the study was approved by the Ethics Committee of the University of Verona.
Anthropometric variables
Weight and height were determined in all subjects, and body mass index (BMI) was computed as weight in kilograms divided by height in square meters. Waist circumference was also obtained with a measuring tape at the narrowest circumference of the abdomen.
Blood collection
Venous blood samples for all metabolic assessments were obtained after overnight fasting. Plasma glucose was measured with a glucose analyzer (Beckman Instruments Inc). The intra-assay coefficient of variance was 1.5%. Plasma insulin was evaluated using a direct chemiluminescent-based 2-site sandwich immunoassay (ADVIA Centaur Insulin assay; Siemens). The sensitivity of the assay was 0.5 mU/l. Insulin resistance was estimated with the homeostasis model assessment (HOMA) method (17).
Serum leptin and adiponectin were measured using specific enzyme-linked immunoassay kits (from DBC-Diagnostic Biochem Canada and B-Bridge, respectively). The sensitivity of the assays was 0.5 ng/ml for leptin and 0.02 ng/ml for adiponectin. High-sensitivity C-reactive protein (hs-CRP) was measured with the immunoturbidimetric method. The detection threshold was 0.5 mg/L, the reference interval was 3 mg/L, and the analytic variability was 5%.
Atrial myocardium collection
In the operating room, myocardial specimens were obtained after median sternotomy and heparin administration occurred before initiating cardiopulmonary bypass, approximately 1 hour within induction of anesthesia. Right atrial myocardial fragments were collected during appendix incannulation. Right atrial myocardium was then stored for immunohistochemistry.
Immunohistochemistry
Freshly isolated atrial tissue was fixed by immersion in 4% paraformaldehyde in 0.1 M phosphate buffer, pH 7.4 overnight at 4°C, and then dehydrated, cleared, and paraffin embedded. Three-micrometer-thick serial sections were obtained and stained by hematoxylin and eosin to assess morphology before immunohistochemical processing. Sections from three different levels (every 200 μm) were than obtained to get representative samples of the whole biopsy for immunohistochemistry.
Primary antibodies were rabbit anti-PLIN1 (1:200; Cell Signaling) and rabbit anti-PLIN2 (1:200; Lifespan Biosciences). Secondary antibodies were as follows: SignalStain Boost immunohistochemical analysis detection reagent HRP Rabbit (ready to use; Cell Signaling). A negative control was conducted by using a secondary antibody alone. Diaminobenzidine hydrochloride substrate kit for peroxidase was used for immunohistochemical analysis (Vector Laboratories).
Sections were dewaxed and subjected to antigen heat retrieval. Endogenous peroxidase activity and nonspecific binding were blocked by incubation with 3% hydrogen peroxide and nonimmune serum, respectively. Slides were then incubated sequentially with primary rabbit antibodies for 16 hours at 4°C, followed by incubation with the secondary antibody for 30 minutes at 20°C. Diaminobenzidine hydrochloride was used as the chromogen to visualize peroxidase activity. Sections were counterstained with hematoxylin and overlaid with coverslips.
Image capturing and analyses
Slides were observed in an Olympus BX51 photomicroscope equipped with a KY-F58 CCD camera (JVC). The images were analyzed and stored using the software Image-ProPlus on a personal computer. The quantification of PLIN1 and PLIN2 was achieved by calculation of the optical density. OD assay was performed on 10 randomly chosen areas (20 μm2 draw each), positive for PLIN1 or PLIN2, by observing images at ×200 magnification, using ImageJ software, version 1.47e.
Diameter and OD assays were performed on LDs positive for PLIN2 antibody.
Measurements were performed on a total of 47 randomly chosen fields at ×400 magnification using ImageJ software, version 1.47e (18).
The specificity of the PLIN2 antibody for the cardiomyocyte LDs was tested on serial sections of the same samples used to detect PLIN1 (Supplemental Figure 1 for PLIN2-positive cardiomyocyte LDs, with PLIN2-negative adipocytes in brown).
Apoptosis evaluation
The detection of apoptotic cardiomyocytes was performed in a subgroup (n = 27) by the terminal deoxynucleotidyltransferase-mediated dUTP nick end labeling method, using the CardioTACS in SITU Apoptosis Detection Kit, Trevigen. To apply this method, myocardial tissue from the paraffin embedded atrium was cut into thin slices (5 μm).
Finally, the atrium was observed in an Olympus BX51 photomicroscope equipped with a KY-F58 CCD camera (JVC) (cardiomyocyte apoptotic nuclei appear dark blue; nuclei of nonapoptotic nuclei appear pink); dark blue nuclei were counted using ImageJ software, version 1.49t, on five images captured at ×200 magnification.
The degree of apoptosis was expressed as the number of dark blue nuclei in square millimeters (19).
Hypoxia
Immunohistochemical analysis was performed in a subgroup (n = 27) using rabbit primary antibody anti-hypoxia inducible factor (HIF)1 alpha (Thermo Scientific, 1:500 dilution). The quantification of nuclei HIF1 alpha positive was performed in blind, observing images with an optical microscope Olympus BX51 at ×600 magnification. Data were expressed as media of nuclei HIF1 alpha positive/mm2 (20).
Statistical analysis
All results are reported as mean ± sd. Logarithmic transformation was performed for not normally distributed variables (PLIN1, leptin, adiponectin, hs-CRP, insulin, and HOMA index). Comparisons between CAD and non-CAD were performed using Student's 2-tailed unpaired test. A χ2 test was used to compare percentages. To compare metabolic variables and fat infiltration between subjects with and without CAD after adjustment for hypertension and type 2 diabetes, covariance analysis was used; Pearson simple and partial correlation and backward multiple regression analysis were used to test association between variables. As possible confounding factors among the independent variables, including absence/presence of hypertension, absence/presence of diabetes and CAD/non-CAD were considered; these variables were always included in the regression models, regardless of the significance of the corresponding terms. A significance level of 5% was always adopted. All analyses were performed using SPSS (software version 17.0, SPSS) and R (R Core Team, 2013) (21).
Results
Characteristics of the subjects are shown in Table 1. BMI and serum leptin were significantly higher in subjects with CAD than in those without, whereas serum adiponectin was significantly lower.
. | Total M ± sd(n = 41) . | CAD M ± sd(n = 23) . | Non-CAD M ± sd(n = 18) . | P . |
---|---|---|---|---|
Age (years) | 65.34 ± 8.95 | 65.65 ± 8.66 | 64.94 ± 9.52 | .805 |
Height (cm) | 171.25 ± 6.79 | 170.72 ± 4.91 | 171.88 ± 8.65 | .597 |
Weight (kg) | 80.71 ± 16.59 | 85.11 ± 19.69 | 75.33 ± 9.84 | .063 |
Waist (cm) | 99.92 ± 12.68 | 102.26 ± 13.67 | 96.33 ± 10.40 | .068 |
BMI (kg/m2) | 27.41 ± 5.15 | 28.93 ± 5.93 | 25.56 ± 3.30 | .034 |
Leptin (ng/ml) | 5.98 ± 6.75 | 8.12 ± 8.38 | 3.36 ± 2.14 | .011a |
Adiponectin (μg/mL) | 16.08 ± 20.70 | 9.08 ± 11.51 | 24.25 ± 25.90 | .002a |
hsCRP (mg/dL) | 1.80 ± 1.67 | 2.32 ± 1.88 | 1.27 ± 1.28 | .08a |
Glucose (mg/dL) | 107.12 ± 22.77 | 112.80 ± 24.17 | 98.38 ± 17.91 | .075 |
Insulin (mU/l) | 7.94 ± 8.36 | 9.195 ± 7.99 | 6.55 ± 8.77 | .210a |
HOMA index | 2.37 ± 2.87 | 2.68 ± 2.57 | 2.01 ± 3.23 | .178a |
SBP (mm Hg) | 137.21 ± 18.96 | 139.21 ± 17.81 | 134.67 ± 20.66 | .496 |
DBP (mm Hg) | 80.44 ± 10.03 | 80.79 ± 7.12 | 80.00 ± 13.10 | .824 |
. | Total M ± sd(n = 41) . | CAD M ± sd(n = 23) . | Non-CAD M ± sd(n = 18) . | P . |
---|---|---|---|---|
Age (years) | 65.34 ± 8.95 | 65.65 ± 8.66 | 64.94 ± 9.52 | .805 |
Height (cm) | 171.25 ± 6.79 | 170.72 ± 4.91 | 171.88 ± 8.65 | .597 |
Weight (kg) | 80.71 ± 16.59 | 85.11 ± 19.69 | 75.33 ± 9.84 | .063 |
Waist (cm) | 99.92 ± 12.68 | 102.26 ± 13.67 | 96.33 ± 10.40 | .068 |
BMI (kg/m2) | 27.41 ± 5.15 | 28.93 ± 5.93 | 25.56 ± 3.30 | .034 |
Leptin (ng/ml) | 5.98 ± 6.75 | 8.12 ± 8.38 | 3.36 ± 2.14 | .011a |
Adiponectin (μg/mL) | 16.08 ± 20.70 | 9.08 ± 11.51 | 24.25 ± 25.90 | .002a |
hsCRP (mg/dL) | 1.80 ± 1.67 | 2.32 ± 1.88 | 1.27 ± 1.28 | .08a |
Glucose (mg/dL) | 107.12 ± 22.77 | 112.80 ± 24.17 | 98.38 ± 17.91 | .075 |
Insulin (mU/l) | 7.94 ± 8.36 | 9.195 ± 7.99 | 6.55 ± 8.77 | .210a |
HOMA index | 2.37 ± 2.87 | 2.68 ± 2.57 | 2.01 ± 3.23 | .178a |
SBP (mm Hg) | 137.21 ± 18.96 | 139.21 ± 17.81 | 134.67 ± 20.66 | .496 |
DBP (mm Hg) | 80.44 ± 10.03 | 80.79 ± 7.12 | 80.00 ± 13.10 | .824 |
Abbreviations: DBP, diastolic blood pressure; SBP, systolic blood pressure.
P value of the Student's t test after logarithmic transformation.
. | Total M ± sd(n = 41) . | CAD M ± sd(n = 23) . | Non-CAD M ± sd(n = 18) . | P . |
---|---|---|---|---|
Age (years) | 65.34 ± 8.95 | 65.65 ± 8.66 | 64.94 ± 9.52 | .805 |
Height (cm) | 171.25 ± 6.79 | 170.72 ± 4.91 | 171.88 ± 8.65 | .597 |
Weight (kg) | 80.71 ± 16.59 | 85.11 ± 19.69 | 75.33 ± 9.84 | .063 |
Waist (cm) | 99.92 ± 12.68 | 102.26 ± 13.67 | 96.33 ± 10.40 | .068 |
BMI (kg/m2) | 27.41 ± 5.15 | 28.93 ± 5.93 | 25.56 ± 3.30 | .034 |
Leptin (ng/ml) | 5.98 ± 6.75 | 8.12 ± 8.38 | 3.36 ± 2.14 | .011a |
Adiponectin (μg/mL) | 16.08 ± 20.70 | 9.08 ± 11.51 | 24.25 ± 25.90 | .002a |
hsCRP (mg/dL) | 1.80 ± 1.67 | 2.32 ± 1.88 | 1.27 ± 1.28 | .08a |
Glucose (mg/dL) | 107.12 ± 22.77 | 112.80 ± 24.17 | 98.38 ± 17.91 | .075 |
Insulin (mU/l) | 7.94 ± 8.36 | 9.195 ± 7.99 | 6.55 ± 8.77 | .210a |
HOMA index | 2.37 ± 2.87 | 2.68 ± 2.57 | 2.01 ± 3.23 | .178a |
SBP (mm Hg) | 137.21 ± 18.96 | 139.21 ± 17.81 | 134.67 ± 20.66 | .496 |
DBP (mm Hg) | 80.44 ± 10.03 | 80.79 ± 7.12 | 80.00 ± 13.10 | .824 |
. | Total M ± sd(n = 41) . | CAD M ± sd(n = 23) . | Non-CAD M ± sd(n = 18) . | P . |
---|---|---|---|---|
Age (years) | 65.34 ± 8.95 | 65.65 ± 8.66 | 64.94 ± 9.52 | .805 |
Height (cm) | 171.25 ± 6.79 | 170.72 ± 4.91 | 171.88 ± 8.65 | .597 |
Weight (kg) | 80.71 ± 16.59 | 85.11 ± 19.69 | 75.33 ± 9.84 | .063 |
Waist (cm) | 99.92 ± 12.68 | 102.26 ± 13.67 | 96.33 ± 10.40 | .068 |
BMI (kg/m2) | 27.41 ± 5.15 | 28.93 ± 5.93 | 25.56 ± 3.30 | .034 |
Leptin (ng/ml) | 5.98 ± 6.75 | 8.12 ± 8.38 | 3.36 ± 2.14 | .011a |
Adiponectin (μg/mL) | 16.08 ± 20.70 | 9.08 ± 11.51 | 24.25 ± 25.90 | .002a |
hsCRP (mg/dL) | 1.80 ± 1.67 | 2.32 ± 1.88 | 1.27 ± 1.28 | .08a |
Glucose (mg/dL) | 107.12 ± 22.77 | 112.80 ± 24.17 | 98.38 ± 17.91 | .075 |
Insulin (mU/l) | 7.94 ± 8.36 | 9.195 ± 7.99 | 6.55 ± 8.77 | .210a |
HOMA index | 2.37 ± 2.87 | 2.68 ± 2.57 | 2.01 ± 3.23 | .178a |
SBP (mm Hg) | 137.21 ± 18.96 | 139.21 ± 17.81 | 134.67 ± 20.66 | .496 |
DBP (mm Hg) | 80.44 ± 10.03 | 80.79 ± 7.12 | 80.00 ± 13.10 | .824 |
Abbreviations: DBP, diastolic blood pressure; SBP, systolic blood pressure.
P value of the Student's t test after logarithmic transformation.
A total of 96% of patients with CAD and 94% of patients without CAD were hypertensive (χ2, NS); 61% of patients with CAD and 17% of patients without CAD were diabetic (χ2, P = .005).
To characterize LDs, immunohistochemistry was performed on all subjects using PLIN1 and PLIN2, respectively. PLIN1 was not detected in nine subjects with CAD (39.1%) and seven without (38.9%). PLIN2 was detected in all subjects (with and without CAD).
Age, BMI, waist circumference, glucose, and insulin levels of the subjects in which PLIN1 was not detected were not different from those of the subjects in which it was. No difference was also observed between the two groups in the presence of CAD or hypertension (data not shown in table).
Figure 1 shows images and histograms of PLIN1 (A) and PLIN2 (B) in the right atrial myocardium in CAD and non-CAD subjects. Both PLIN1 and PLIN2 were significantly higher in CAD than in non-CAD subjects (0.35 ± 0.04 OD versus 0.28 ± 0.05 OD; P < .01; 0.27 ± 0.05 OD versus 0.22 ± 0.05 OD; P < .01, respectively).
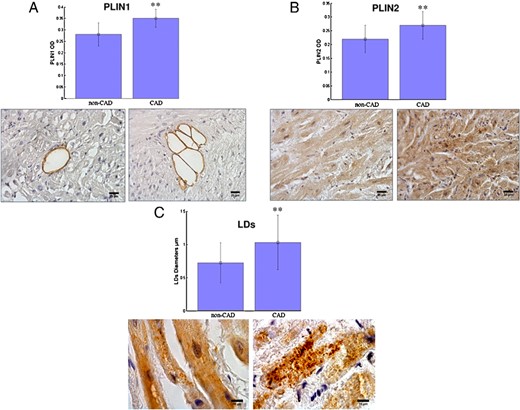
Representative images of immunohistochemical staining and histograms showing a greater expression of PLIN1 (A) and PLIN2 (B) in the right atrium of patients with CAD compared to patients without CAD (non-CAD) (**, P <.01; ×200 magnification; scale bar, 25 μm).
C, Images and histogram representing the differences of LD diameters for PLIN2 are shown (**, P <.01; ×400 magnification; scale bar, 10 μm).
An image and histogram representing LDs' diameters are also shown in Figure 1C: atrial mean LD diameters resulted significantly higher in CAD than in non-CAD subjects (1.03 ± 0.41 versus 0.72 ± 0.30, P < .01). The mean LDs' OD was higher in CAD subjects than in non-CAD subjects (0.31 ± 0.04 versus 0.27 ± 0.04, P < .01).
Even after adjusting for type 2 diabetes or BMI, differences in PLIN1 and PLIN2 in the right atrial myocardium between CAD and non-CAD were still significant (data not shown in table).
A greater number of apoptotic cardiomyocytes (A) and HIF1 alpha positive cardiomyocytes (B) were observed in CAD than in non-CAD subjects (417.1 ± 99.1 versus 209.7 ± 136.6, P < .001 and 3.30 ± 2.38 versus 1.92 ± 1.73, P < .08, NS, respectively) (Figure 2). After adjusting for type 2 diabetes and BMI, the number of apoptotic cardiomyocytes was still significantly greater in CAD than in non-CAD subjects, whereas the number of HIF1 alpha positive cardiomyocytes were not (data not shown in table).
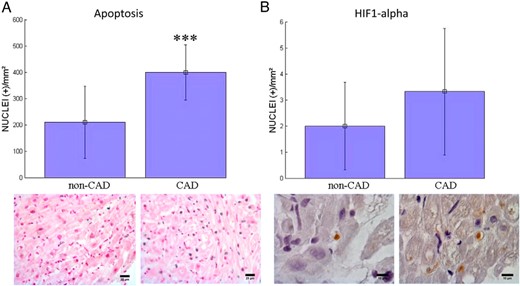
Apoptotic cardiomyocytes (A) and HIF1 alpha (B) positive cardiomyocytes at immunohistochemistry analyses in the right atrium of patients without CAD (non-CAD) and with CAD (***, P <.001).
(A, ×200 magnification; B, ×600 magnification images).
Table 2 shows correlations between PLIN1, PLIN2, and anthropometric, metabolic variables, and degree of apoptosis and HIF1 alpha. PLIN1 was positively associated with serum leptin (P < .05), hs-CRP (P < .01), and degree of apoptosis (P < .05) and negatively with adiponectin (P < .01). PLIN2 was positively associated with BMI (P < .01), waist circumference (P < .01), and leptin (P < .05) and negatively with adiponectin (P < .01). When partial correlations were calculated to adjust for hypertension, diabetes, and CAD versus non-CAD, adiponectin and degree of apoptosis remained significantly associated with PLIN1, whereas only waist circumference and adiponectin remained significantly associated with PLIN2.
Simple and Partial Correlations (Adjusted for Hypertension, Diabetes, and CAD/non-CAD) Between PLIN1 and PLIN2 and Main Variables of the Study
. | PLIN1 . | PLIN2 . | ||
---|---|---|---|---|
r . | Adjusted for Hypertension, Diabetes, and CAD/Non-CAD . | r . | Adjusted for Hypertension, Diabetes, and CAD/Non-CAD . | |
Age | 0.093 | −0.027 | −0.170 | −0.090 |
BMI | 0.360 | 0.084 | 0.400b | 0.172 |
Waist | 0.376 | 0.135 | 0.433b | 0.323a |
Leptin | 0.468a | 0.254 | 0.336a | 0.118 |
Adiponectin | −0.648b | −0.485a | −0.480b | −0.330a |
hsCRP | 0.551b | 0.446a | 0.186 | −0.083 |
HOMA index | 0.097 | −0.288 | 0.130 | −0.071 |
Apoptosis | 0.604a,c | 0.564a | 0.060d | 0.040 |
HIF1 alpha | 0.117c | −0.062 | 0.237d | −0.005 |
. | PLIN1 . | PLIN2 . | ||
---|---|---|---|---|
r . | Adjusted for Hypertension, Diabetes, and CAD/Non-CAD . | r . | Adjusted for Hypertension, Diabetes, and CAD/Non-CAD . | |
Age | 0.093 | −0.027 | −0.170 | −0.090 |
BMI | 0.360 | 0.084 | 0.400b | 0.172 |
Waist | 0.376 | 0.135 | 0.433b | 0.323a |
Leptin | 0.468a | 0.254 | 0.336a | 0.118 |
Adiponectin | −0.648b | −0.485a | −0.480b | −0.330a |
hsCRP | 0.551b | 0.446a | 0.186 | −0.083 |
HOMA index | 0.097 | −0.288 | 0.130 | −0.071 |
Apoptosis | 0.604a,c | 0.564a | 0.060d | 0.040 |
HIF1 alpha | 0.117c | −0.062 | 0.237d | −0.005 |
PLIN1, leptin, adiponectin, hs-CRP, and HOMA index are expressed in logarithm.
P < .05.
P < .01.
n = 19 subjects.
n = 27 subjects.
Simple and Partial Correlations (Adjusted for Hypertension, Diabetes, and CAD/non-CAD) Between PLIN1 and PLIN2 and Main Variables of the Study
. | PLIN1 . | PLIN2 . | ||
---|---|---|---|---|
r . | Adjusted for Hypertension, Diabetes, and CAD/Non-CAD . | r . | Adjusted for Hypertension, Diabetes, and CAD/Non-CAD . | |
Age | 0.093 | −0.027 | −0.170 | −0.090 |
BMI | 0.360 | 0.084 | 0.400b | 0.172 |
Waist | 0.376 | 0.135 | 0.433b | 0.323a |
Leptin | 0.468a | 0.254 | 0.336a | 0.118 |
Adiponectin | −0.648b | −0.485a | −0.480b | −0.330a |
hsCRP | 0.551b | 0.446a | 0.186 | −0.083 |
HOMA index | 0.097 | −0.288 | 0.130 | −0.071 |
Apoptosis | 0.604a,c | 0.564a | 0.060d | 0.040 |
HIF1 alpha | 0.117c | −0.062 | 0.237d | −0.005 |
. | PLIN1 . | PLIN2 . | ||
---|---|---|---|---|
r . | Adjusted for Hypertension, Diabetes, and CAD/Non-CAD . | r . | Adjusted for Hypertension, Diabetes, and CAD/Non-CAD . | |
Age | 0.093 | −0.027 | −0.170 | −0.090 |
BMI | 0.360 | 0.084 | 0.400b | 0.172 |
Waist | 0.376 | 0.135 | 0.433b | 0.323a |
Leptin | 0.468a | 0.254 | 0.336a | 0.118 |
Adiponectin | −0.648b | −0.485a | −0.480b | −0.330a |
hsCRP | 0.551b | 0.446a | 0.186 | −0.083 |
HOMA index | 0.097 | −0.288 | 0.130 | −0.071 |
Apoptosis | 0.604a,c | 0.564a | 0.060d | 0.040 |
HIF1 alpha | 0.117c | −0.062 | 0.237d | −0.005 |
PLIN1, leptin, adiponectin, hs-CRP, and HOMA index are expressed in logarithm.
P < .05.
P < .01.
n = 19 subjects.
n = 27 subjects.
A multiple backward regression analysis was performed using PLIN1 as a dependent variable and serum adiponectin, serum leptin, and hs-CRP as independent variables for all subjects (Table 3). In addition, among the independent variables, absence/presence of hypertension, diabetes, and CAD/non-CAD were also considered and were always included in the regression models, regardless of the significance of the corresponding terms. The initial regression model had multiple r2 = 0.627. In the first step, leptin was removed from the model because the corresponding partial regression coefficient was the least significant (P = .981); in the second step, hs-CRP was removed from the model because the corresponding partial regression coefficient was the least significant (P = .213). The final model also included adiponectin (Table 3), together with the absence/presence of hypertension, diabetes, and CAD/non-CAD.
. | PLIN1 . | PLIN2 . | ||
---|---|---|---|---|
βa . | P . | βa . | P . | |
Hypertension | −0.024 | .916 | 0.195 | .206 |
Diabetes | 0.115 | .610 | 0.021 | .907 |
CAD/non-CAD | −0.368 | .092 | −0.180 | .330 |
Adiponectin | −0.485 | .022 | −0.343 | .048 |
Waist circumference | .347 | .049 | ||
rb | 0.532 | 0.399 |
. | PLIN1 . | PLIN2 . | ||
---|---|---|---|---|
βa . | P . | βa . | P . | |
Hypertension | −0.024 | .916 | 0.195 | .206 |
Diabetes | 0.115 | .610 | 0.021 | .907 |
CAD/non-CAD | −0.368 | .092 | −0.180 | .330 |
Adiponectin | −0.485 | .022 | −0.343 | .048 |
Waist circumference | .347 | .049 | ||
rb | 0.532 | 0.399 |
When PLIN1 was the dependent variable, the initial model included serum adiponectin, serum leptin, hs-CRP, hypertension, diabetes, and CAD/non-CAD as independent variables. When PLIN2 was the dependent variable, the initial model included BMI, waist circumference, adiponectin, serum leptin, hs-CRP, hypertension, diabetes, and CAD/non-CAD as independent variables.
Standardized regression coefficients.
. | PLIN1 . | PLIN2 . | ||
---|---|---|---|---|
βa . | P . | βa . | P . | |
Hypertension | −0.024 | .916 | 0.195 | .206 |
Diabetes | 0.115 | .610 | 0.021 | .907 |
CAD/non-CAD | −0.368 | .092 | −0.180 | .330 |
Adiponectin | −0.485 | .022 | −0.343 | .048 |
Waist circumference | .347 | .049 | ||
rb | 0.532 | 0.399 |
. | PLIN1 . | PLIN2 . | ||
---|---|---|---|---|
βa . | P . | βa . | P . | |
Hypertension | −0.024 | .916 | 0.195 | .206 |
Diabetes | 0.115 | .610 | 0.021 | .907 |
CAD/non-CAD | −0.368 | .092 | −0.180 | .330 |
Adiponectin | −0.485 | .022 | −0.343 | .048 |
Waist circumference | .347 | .049 | ||
rb | 0.532 | 0.399 |
When PLIN1 was the dependent variable, the initial model included serum adiponectin, serum leptin, hs-CRP, hypertension, diabetes, and CAD/non-CAD as independent variables. When PLIN2 was the dependent variable, the initial model included BMI, waist circumference, adiponectin, serum leptin, hs-CRP, hypertension, diabetes, and CAD/non-CAD as independent variables.
Standardized regression coefficients.
A multiple backward regression analysis was performed using PLIN2 as a dependent variable and BMI, waist circumference, serum adiponectin, and serum leptin as independent variables for all subjects. Also in this case, among the independent variables, absence/presence of hypertension, diabetes, and CAD/non-CAD were considered and were always included in the regression models, regardless of the significance of the corresponding terms. The initial regression model had multiple r2 = 0.427. In the following two steps, BMI (P = .708) and leptin (P = .271) were removed from the model. The final model also included—together with absence/presence of hypertension, diabetes, and CAD/non-CAD—waist circumference and serum adiponectin (Table 3).
Table 3 shows the standardized partial regression coefficients of the variables included in the final model when considering PLIN1 or PLIN2 as dependent variables, together with the corresponding multiple r2 (0.523 and 0.399, respectively). After having taken into account the absence/presence of hypertension, diabetes, and CAD/non-CAD, adiponectin was inversely associated with both PLIN1 and PLIN2, whereas waist circumference was positively associated only with PLIN2.
Discussion
Our study shows that myocardial steatosis was significantly greater in subjects with CAD than in those without, and that this depends on both a higher presence of metabolically active adipocytes interspersed among cardiomyocytes and a higher fat deposition inside cardiomyocytes.
Myocardial steatosis has been generally considered a consequence of an impaired ability of the heart to oxidize fatty acid surplus with an increased fatty acid storage as LDs. Excessive triglyceride storage may determine accumulation of detrimental lipid intermediates such as long-chain acyl CoA, ceramide and diacylglycerol, which are correlated with contractile dysfunction in several genetically altered and mutant murine models, and are termed lipotoxic cardiomyopathy (22).
Myocardial steatosis has been observed in subjects with obesity, diabetes, and metabolic syndrome and may contribute to the development of the cardiac dysfunctions that lead to dilated cardiomyopathy (1, 2, 5).
However, our findings showing higher myocardial steatosis as well as a higher degree of apoptosis in subjects with CAD than in those without seem to be in line with previous observations (7, 8), suggesting that myocardial hypoxia itself may be responsible for myocardial steatosis. Further the positive correlation between PLIN1 and the degree of apoptosis observed in our study seems to confirm the hypothesis that excess fatty acid diversion to nonadipose tissues, including the heart, could determine damage-inducing apoptosis of cardiomyocytes. The imbalance between oxidative and nonoxidative metabolism of fatty acids in the myocardium has been suggested as being capable of regulating cardiomyocyte damage (23).
We also observed a higher, even though not significant, degree of HIF1 alpha in subjects with CAD than in those without. This finding seems to be at least partially in line with previous observations of a possible association between hypoxia and myocardial steatosis (6–8).
Years ago, Baroldi et al (7) observed a fatty metaplasia in subjects with myocardial infarction. In their examination, of a wide study sample of patients who had received heart transplantation for ischemic heart diseases, Su et al (8) observed mature adipose tissue in almost two thirds of the histological sections. In these studies, however (7, 8), myocardial steatosis was evaluated by using techniques that do not permit LD characterization. In our study, we evaluated two specific markers of LDs such as PLIN1, selectively expressed in LDs of the adipocytes and considered a marker of vital and mature adipocytes, and PLIN2, ubiquitously expressed in LD of other cells, such as cardiomyocytes but not present in adipocytes (12–15). As a result, we were able to complement and expand previous reports (7–8) by observing that the greater degree of myocardial steatosis found in subjects with CAD compared to those without depends on both greater accumulation of fat inside cardiomyocytes and larger amount of adipocytes interspersed inside the myocardium.
PLIN1 was not detected in nine subjects with CAD or in seven without, whereas PLIN2 was detected in all subjects. These findings may suggest that fat infiltration inside cardiomyocytes is quite frequent in the heart, whereas adipocytes infiltration in the myocardium is not.
In multivariate analyses, we observed that abdominal fat, as evaluated by waist circumference, together with serum adiponectin, were independent predictors of PLIN2: these findings are in line with Graner et al (24), who recently observed a significant association between visceral fat, as assessed by computed tomography, and myocardial triglycerides as assessed by magnetic resonance. The negative association observed in our study sample between adiponectin and PLIN1 and PLIN2 seems to be in line with previous observations that adiponectin increases insulin sensitivity (25) by triggering the preferential use of fatty acids as a fuel in muscles (26) and that insulin-sensitizer treatment prevents myocardial fat accumulation in animals (27).
Taken together, these results suggest that myocardial steatosis may be regulated by different factors and may be prevented by low visceral fat amounts and by improving insulin resistance. In fact, it is possible to hypothesize that the higher the visceral fat, the higher the amount of circulating fatty acids; similarly, the lower the adiponectin serum levels, the higher the deposition of fat inside the heart, both in terms of fat deposition inside cardiomyocytes and of the presence of adipocytes interspersed inside the myocardium.
The presence of fat cells interspersed in the myocardium might be an important and, until now, relatively overlooked factor responsible for myocardial toxicity. In fact, other than providing locally free fatty acids from the hydrolysis of triglycerides, the presence of adipocytes may release adipokines, which are capable of playing a major role in promoting insulin resistance and may contribute to the onset of oxidation damage and hypoxia (25). Intramyocardial adipocytes could be considered an ectopic visceral fat depot that might even lead to contractile cardiac dysfunction. According to this hypothesis, recent in vitro studies confirmed that adipocyte-related factors are directly involved in the pathogenesis of myocardial dysfunction (28). Similarly, Look et al (29) revealed that an adipocyte-conditioned medium containing all factors released from mature fat cells directly and acutely decreases cardiac force generation and coronary flow. Furthermore, a cardiodepressant peptide has been recently shown to be produced by adipocytes (30).
Mature adipocyte inside the myocardium may derive from the epicardium or perivascular regions, but it may also originate from transdifferentiation of regenerative cells existing in the myocardium (31–33) that, under specific signaling, may differentiate into fat cells or other mesenchymal cells under the influence of different microenvironmental factors.
Interestingly, our findings seem to suggest that even systemic factors, such as serum adiponectin, may play a role in myocardial adipocyte migration and/or differentiation.
Some limitations of our study should be recognized. First, the study design does not permit for evaluation of molecular mechanism underlying myocardial steatosis, both in terms of intramyocellular fat and adipocyte infiltration. Second, the cross-sectional design only shows association, not causality. Third, because only men were included in the study sample, the results cannot be generalized and transferred to women. Furthermore, although PLIN2 has been used to evaluate LD accumulation in muscle and cardiac cells, it should be acknowledged that recently PLIN5 has been suggested as a better specific surrogate marker of LDs in the heart (34).
Moreover, because of the design of the study, we were able to collect biopsies of the right atrium but no information is available for the rest of the heart, in particular on the left ventriculum; thus, our results need to be confirmed. In any case, it must be taken into account that ischemia has been shown to affect both the atrium and ventriculum (35).
Finally, unfortunately, we were able to observe only a marginally significant association between CAD status and PLIN1. It may be important to confirm this result with a larger sample.
In conclusion, our study shows greater myocardial steatosis in subjects with CAD than in those without, determined by both a higher presence of metabolically active adipocytes interspersed among cardiomyocytes and a higher LD deposition inside cardiomyocytes as well as increased apoptosis and hypoxia.
Intramyocardial adipocytes could represent a subcompartment of visceral adipose tissue with a potential metabolic impact on heart function. Further studies focusing on origin, function, and contribution of these adipocytes to heart disease are needed. Finally, our findings showing that waist circumference and serum adiponectin are independent predictors of myocardial steatosis may represent further evidence of the need to treat abdominal obesity to improve cardiac lipotoxicity.
Acknowledgments
We thank A.R. for contributing to the data acquisition and M.J.N. for the language revision.
Author contributions: M.Z., G.M., F.F., A.S., E.Z., and F.S. conceived the overall study; M.Z., G.M., F.F., E.Z., and A.S. drafted the manuscript; A.S., C.B., S.F., M.P., and G.F. performed the subject screening and data collection; A.S., R.V., and F.C. performed the light microscopy and image analysis; and R.M. performed statistical analyses.
Disclosure Summary: The authors have nothing to disclose.
Abbreviations
- BMI
body mass index
- CAD
coronary artery disease
- HIF1
hypoxia inducible factor 1 alpha
- HOMA
homeostasis model assessment
- hs-CRP
high-sensitivity C-reactive protein
- LD
liquid droplet
- PLIN
perilipin.