-
PDF
- Split View
-
Views
-
Cite
Cite
Takashi Amoh, Keiji Murakami, Reiko Kariyama, Kenji Hori, Darija Viducic, Katsuhiko Hirota, Jun Igarashi, Hiroaki Suga, Matthew R. Parsek, Hiromi Kumon, Yoichiro Miyake, Effects of an autoinducer analogue on antibiotic tolerance in Pseudomonas aeruginosa, Journal of Antimicrobial Chemotherapy, Volume 72, Issue 8, August 2017, Pages 2230–2240, https://doi.org/10.1093/jac/dkx132
- Share Icon Share
Abstract
Objectives: Antibiotic tolerance causes chronic, refractory and persistent infections. In order to advance the development of a new type of drug for the treatment of infectious diseases, we herein investigated the effects of a newly synthesized analogue of the Pseudomonas aeruginosa quorum-sensing autoinducer named AIA-1 (autoinducer analogue) on antibiotic tolerance in P. aeruginosa.
Methods: A P. aeruginosa luminescent strain derived from PAO1 was injected into neutropenic ICR mice and bioluminescence images were acquired for a period of time after treatments with antibiotics and AIA-1. In vitro susceptibility testing and killing assays for the planktonic and biofilm cells of PAO1 were performed using antibiotics and AIA-1. The expression of quorum-sensing-related genes was examined using real-time PCR.
Results:In vivo and in vitro assays showed that AIA-1 alone did not exert any bactericidal effects and also did not affect the MICs of antibiotics. However, the combined use of AIA-1 and antibiotics exerted markedly stronger therapeutic effects against experimental infection than antibiotics alone. The presence of AIA-1 also enhanced the killing effects of antibiotics in planktonic and biofilm cells. Although AIA-1 did not inhibit the expression of lasB and rhlA genes, which are directly regulated by quorum sensing, it clearly suppressed expression of the rpoS gene.
Conclusions: The new compound, AIA-1, did not alter the antibiotic susceptibility of P. aeruginosa by itself; however, its addition enhanced the antibacterial activity of antibiotics. AIA-1 did not inhibit quorum sensing, but reduced the antibiotic tolerance of P. aeruginosa by suppressing rpoS gene expression.
Introduction
Pseudomonas aeruginosa is a Gram-negative bacterium that possesses strong adaptability and can thrive in diverse environments. The pathogenic potential of P. aeruginosa, which places this bacterium as one of the major causes of infections in immunocompromised hosts, has been attributed to the production of different virulence factors, such as elastase, pyocyanin and rhamnolipids. The production of these virulence factors is controlled by a mechanism known as a quorum sensing (QS) system,1 which is a bacterial cell-to-cell communication process that depends on bacterial population density and involves small diffusible signalling molecules called autoinducers.2,3,P. aeruginosa produces two main acyl-homoserine lactone autoinducers, 3-oxo-C12-HSL (OdDHL) and C4-HSL (BHL), which are synthesized by members of the Lux-I family of proteins, LasI and RhlI, respectively.2,3 Since its disruption reduces the pathogenicity of P. aeruginosa, the QS system remains a crucial target for drug design.4 The QS system is also involved in biofilm formation,5 and bacteria that grow as a biofilm often exhibit increased tolerance to antimicrobials.6
Antibiotic tolerance is defined as the ability of bacteria to survive, but not grow, in the presence of antibiotics.7 This mechanism is not caused by a mutation; it reflects phenotypic variations in cells. Tolerant bacteria survive in the presence of high concentrations of antibiotics, and surviving cells start to re-grow once antibiotics are removed or inactivated.7 Furthermore, antibiotic tolerance is one of the main reasons for the development of chronic infections. To date, several factors have been linked to antibiotic tolerance, such as stress responses8 and nutrient starvation,9 and it has been reported that stationary-phase bacteria exhibit higher tolerance to antibiotics than their counterparts in logarithmic growth.10 Previous studies have indicated that the alternative sigma factors RpoN and RpoS contribute to antibiotic tolerance.11–13 In addition, Kayama et al.14 demonstrated that the rpoS gene and QS system are involved in ofloxacin tolerance.
Since QS controls the production of virulence factors and secondary metabolites, and biofilm formation, which are responsible for the pathogenicity of this bacterium, QS is a target for inhibition by antimicrobial agents. Therefore, it is considered important to identify compounds with the potential to function as QS-specific drugs.
Smith et al.15,16 synthesized a library of P. aeruginosa autoinducer analogues (AIAs) with variations targeted to the acyl-homoserine lactone, and demonstrated that certain analogues produced significant reductions in the production of virulence factors and biofilm formation.
The study had two aims: (i) to investigate the effects of one of the AIAs, AIA-1, which is an analogue of OdDHL, on antibiotic tolerance in P. aeruginosa; and (ii) to elucidate the relationship between AIA-1 and factors related to antibiotic tolerance.
Materials and methods
Strains and plasmids
The bacterial strains and plasmids used in this study are listed in Table 1. The strains were incubated at 37°C in lysogeny broth (LB) or on LB agar plates. Antibiotics were used at concentrations of 20 mg/L tetracycline, 20 mg/L gentamicin and 100 mg/L ampicillin for Escherichiacoli, and 200 mg/L tetracycline, 500 mg/L carbenicillin and 200 mg/L gentamicin for P. aeruginosa; when necessary, supplementation with 10% sucrose for conjugation experiments and 0.2% arabinose was used in overexpression studies.
Strain . | Relevant characteristics . | Reference . |
---|---|---|
P. aeruginosa | ||
PAO1 | WT | |
PAO1 plac-lux | one copy of the lac promoter linked to the luxCDABE gene in the att site of the P. aeruginosa chromosome | this study |
PAO1ΔlasI | PAO1 in-frame deletion of lasI | this study |
PAO1ΔlasIΔrhlI | PAO1 in-frame deletion of lasI and rhlI | this study |
PAO1ΔrpoS | PAO1 in-frame deletion of rpoS | this study |
PAO1/pJN105 | PAO1, derivative harbouring pJN105 | this study |
PAO1/pJN105-rpoS | PAO1, derivative harbouring pJN105-rpoS | this study |
E. coli | ||
S17-1 | thi endA recA hsdRchromosome::RP4-2Tc::Mu-Km::Tn7 | 20 |
DH5α | F−, Φ80dlacZΔM15, Δ(lacZYA-argF)U169, deoR, recA1, endA1, hsdR17(, ), phoA, supE44, λ−, thi-1, gyrA96, relA1 | TaKaRa |
BL21 | F−, ompT, hsdSB(), gal, dcm | TaKaRa |
Plasmids | ||
mini-CTX2 | plasmid for integration of genes into the att site of the P. aeruginosa chromosome, TcR | 17 |
pXen13 | cloning vector carrying the original Photorhabdus luminescens luxCDABE operon | Xenogen |
pUCP18 | P. aeruginosa–E. coli shuttle vector | 18 |
pJN105 | araC-PBAD cassette cloned into pBBR1MCS-5; GmR | 21 |
miniCTX-plac-lux | one copy of lac promoter linked to the luxCDABE gene inserted into miniCTX-2 | this study |
pFLP2 | FLP recombinase-expressing plasmid | 19 |
pEX18Gm | broad-host-range replacement vector; sacB, GmR | 19 |
pEX18Gm-lasI | lasI deletion suicide vector | this study |
pEX18Gm-rhlI | rhlI deletion suicide vector | this study |
pEX18Gm-rpoS | rpoS deletion suicide vector | this study |
pJN105-rpoS | rpoS cloned into pJN105; GmR | this study |
pGEX-6P-2 | GST fusion expressing vector; ApR | GE Healthcare |
pGEX-6P-2-rpoS | rpoS cloned into pGEX-6P-2; ApR | this study |
Strain . | Relevant characteristics . | Reference . |
---|---|---|
P. aeruginosa | ||
PAO1 | WT | |
PAO1 plac-lux | one copy of the lac promoter linked to the luxCDABE gene in the att site of the P. aeruginosa chromosome | this study |
PAO1ΔlasI | PAO1 in-frame deletion of lasI | this study |
PAO1ΔlasIΔrhlI | PAO1 in-frame deletion of lasI and rhlI | this study |
PAO1ΔrpoS | PAO1 in-frame deletion of rpoS | this study |
PAO1/pJN105 | PAO1, derivative harbouring pJN105 | this study |
PAO1/pJN105-rpoS | PAO1, derivative harbouring pJN105-rpoS | this study |
E. coli | ||
S17-1 | thi endA recA hsdRchromosome::RP4-2Tc::Mu-Km::Tn7 | 20 |
DH5α | F−, Φ80dlacZΔM15, Δ(lacZYA-argF)U169, deoR, recA1, endA1, hsdR17(, ), phoA, supE44, λ−, thi-1, gyrA96, relA1 | TaKaRa |
BL21 | F−, ompT, hsdSB(), gal, dcm | TaKaRa |
Plasmids | ||
mini-CTX2 | plasmid for integration of genes into the att site of the P. aeruginosa chromosome, TcR | 17 |
pXen13 | cloning vector carrying the original Photorhabdus luminescens luxCDABE operon | Xenogen |
pUCP18 | P. aeruginosa–E. coli shuttle vector | 18 |
pJN105 | araC-PBAD cassette cloned into pBBR1MCS-5; GmR | 21 |
miniCTX-plac-lux | one copy of lac promoter linked to the luxCDABE gene inserted into miniCTX-2 | this study |
pFLP2 | FLP recombinase-expressing plasmid | 19 |
pEX18Gm | broad-host-range replacement vector; sacB, GmR | 19 |
pEX18Gm-lasI | lasI deletion suicide vector | this study |
pEX18Gm-rhlI | rhlI deletion suicide vector | this study |
pEX18Gm-rpoS | rpoS deletion suicide vector | this study |
pJN105-rpoS | rpoS cloned into pJN105; GmR | this study |
pGEX-6P-2 | GST fusion expressing vector; ApR | GE Healthcare |
pGEX-6P-2-rpoS | rpoS cloned into pGEX-6P-2; ApR | this study |
Strain . | Relevant characteristics . | Reference . |
---|---|---|
P. aeruginosa | ||
PAO1 | WT | |
PAO1 plac-lux | one copy of the lac promoter linked to the luxCDABE gene in the att site of the P. aeruginosa chromosome | this study |
PAO1ΔlasI | PAO1 in-frame deletion of lasI | this study |
PAO1ΔlasIΔrhlI | PAO1 in-frame deletion of lasI and rhlI | this study |
PAO1ΔrpoS | PAO1 in-frame deletion of rpoS | this study |
PAO1/pJN105 | PAO1, derivative harbouring pJN105 | this study |
PAO1/pJN105-rpoS | PAO1, derivative harbouring pJN105-rpoS | this study |
E. coli | ||
S17-1 | thi endA recA hsdRchromosome::RP4-2Tc::Mu-Km::Tn7 | 20 |
DH5α | F−, Φ80dlacZΔM15, Δ(lacZYA-argF)U169, deoR, recA1, endA1, hsdR17(, ), phoA, supE44, λ−, thi-1, gyrA96, relA1 | TaKaRa |
BL21 | F−, ompT, hsdSB(), gal, dcm | TaKaRa |
Plasmids | ||
mini-CTX2 | plasmid for integration of genes into the att site of the P. aeruginosa chromosome, TcR | 17 |
pXen13 | cloning vector carrying the original Photorhabdus luminescens luxCDABE operon | Xenogen |
pUCP18 | P. aeruginosa–E. coli shuttle vector | 18 |
pJN105 | araC-PBAD cassette cloned into pBBR1MCS-5; GmR | 21 |
miniCTX-plac-lux | one copy of lac promoter linked to the luxCDABE gene inserted into miniCTX-2 | this study |
pFLP2 | FLP recombinase-expressing plasmid | 19 |
pEX18Gm | broad-host-range replacement vector; sacB, GmR | 19 |
pEX18Gm-lasI | lasI deletion suicide vector | this study |
pEX18Gm-rhlI | rhlI deletion suicide vector | this study |
pEX18Gm-rpoS | rpoS deletion suicide vector | this study |
pJN105-rpoS | rpoS cloned into pJN105; GmR | this study |
pGEX-6P-2 | GST fusion expressing vector; ApR | GE Healthcare |
pGEX-6P-2-rpoS | rpoS cloned into pGEX-6P-2; ApR | this study |
Strain . | Relevant characteristics . | Reference . |
---|---|---|
P. aeruginosa | ||
PAO1 | WT | |
PAO1 plac-lux | one copy of the lac promoter linked to the luxCDABE gene in the att site of the P. aeruginosa chromosome | this study |
PAO1ΔlasI | PAO1 in-frame deletion of lasI | this study |
PAO1ΔlasIΔrhlI | PAO1 in-frame deletion of lasI and rhlI | this study |
PAO1ΔrpoS | PAO1 in-frame deletion of rpoS | this study |
PAO1/pJN105 | PAO1, derivative harbouring pJN105 | this study |
PAO1/pJN105-rpoS | PAO1, derivative harbouring pJN105-rpoS | this study |
E. coli | ||
S17-1 | thi endA recA hsdRchromosome::RP4-2Tc::Mu-Km::Tn7 | 20 |
DH5α | F−, Φ80dlacZΔM15, Δ(lacZYA-argF)U169, deoR, recA1, endA1, hsdR17(, ), phoA, supE44, λ−, thi-1, gyrA96, relA1 | TaKaRa |
BL21 | F−, ompT, hsdSB(), gal, dcm | TaKaRa |
Plasmids | ||
mini-CTX2 | plasmid for integration of genes into the att site of the P. aeruginosa chromosome, TcR | 17 |
pXen13 | cloning vector carrying the original Photorhabdus luminescens luxCDABE operon | Xenogen |
pUCP18 | P. aeruginosa–E. coli shuttle vector | 18 |
pJN105 | araC-PBAD cassette cloned into pBBR1MCS-5; GmR | 21 |
miniCTX-plac-lux | one copy of lac promoter linked to the luxCDABE gene inserted into miniCTX-2 | this study |
pFLP2 | FLP recombinase-expressing plasmid | 19 |
pEX18Gm | broad-host-range replacement vector; sacB, GmR | 19 |
pEX18Gm-lasI | lasI deletion suicide vector | this study |
pEX18Gm-rhlI | rhlI deletion suicide vector | this study |
pEX18Gm-rpoS | rpoS deletion suicide vector | this study |
pJN105-rpoS | rpoS cloned into pJN105; GmR | this study |
pGEX-6P-2 | GST fusion expressing vector; ApR | GE Healthcare |
pGEX-6P-2-rpoS | rpoS cloned into pGEX-6P-2; ApR | this study |
Reagents
AIA-1 is N-(piperidin-4-yl)-dodecanamide and its structure is shown in Figure 1(a). Biapenem was purchased from Meiji Seika Pharma Co., Ltd (Tokyo, Japan). Tobramycin and levofloxacin were purchased from Sigma-Aldrich (St Louis, MO, USA).
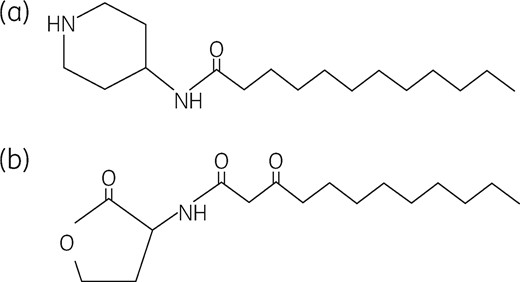
(a) Structure of AIA-1, an analogue of OdDHL. (b) Structure of OdDHL.
Plasmid construction and generation of mutant strains
In in vivo bioluminescence imaging, the miniCTX-lux plasmid was constructed by ligating SacI/BamHI-digested mini-CTX217 with a SacI/BamHI fragment digested from pXen13 (Xenogen, CA, USA), encompassing the lux operon. The lac promoter region was amplified by PCR using the pUCP18 plasmid18 as a template. The amplified lac promoter region and miniCTX-lux were digested with XhoI/BamHI and ligated, resulting in miniCTX-Plac::lux. PAO1 Plac::lux was created by the integration of miniCTX-Plac::lux at the attB site of PAO1, followed by FLP recombinase-mediated excision of the plasmid backbone.19 Colonies were screened for tetracycline and carbenicillin susceptibility and the loss of sucrose susceptibility. In order to construct the lasI and rhlI double-deletion mutant, ∼500 bp of the upstream and downstream regions of each gene was amplified from PAO1 chromosomal DNA and cloned into pEX18Gm19 using BamHI and EcoRI restriction sites to generate pEX18Gm-lasI and pEX18Gm-rhlI. pEX18Gm-lasI was mobilized from E. coli S17–120 into P. aeruginosa by mating, resulting in PAO1ΔlasI. PAO1ΔlasIΔrhlI was constructed by transferring pEX18Gm-rhlI from E. coli S17–1 to PAO1ΔlasI. Colonies were screened for gentamicin susceptibility and the loss of sucrose susceptibility. In order to generate the rpoS in-frame deletion mutant, the aforementioned procedure was used. In rpoS overexpression studies, the rpoS ORF was amplified by PCR, and was cloned as EcoRI/XbaI-digested product into EcoRI/XbaI-digested pJN105,21 resulting in pJN105-rpoS. This plasmid and pJN105 were electroporated into PAO1, resulting in PAO1/pJN105-rpoS and PAO1/pJN105, respectively.
Ethics
Mouse infection studies were approved by the Animal Care and Use Committee, Okayama University, Process number OKU-2013055. All procedures were performed according to the Policy on the Care and Use of Laboratory Animals, Okayama University.
Mouse infection model and bioluminescence imaging
PAO1 Plac::lux was inoculated into neutropenic ICR mice aged 5–7 weeks. An inoculum containing ∼106 cfu was injected into one thigh of each animal. AIA-1 (50 mg/kg body weight) was intraperitoneally administered twice in 8 h. Two hours after the inoculum injection, mice were subcutaneously administered the selected concentrations of biapenem (0.78 mg/kg body weight) or tobramycin (4 mg/kg body weight) four times over a period of 8 h. At 0, 2, 4, 6, 8 and 10 h after the inoculum injection, mice were anaesthetized using a constant flow of 2.5% isoflurane mixed with oxygen, and bioluminescence images were acquired using an IVIS® Lumina system (Caliper, Alameda, CA, USA). At 10 h, photons emitted per second by mice were quantified, and thighs from infected mice were homogenized in saline. A portion of the homogenate was serially diluted and spread on a nutrient agar plate to measure viable counts of bacteria.
Susceptibility assay
MIC was assessed by the standard microbroth dilution method.22 In susceptibility assays including both antibiotics and AIA-1, AIA-1 was used at a concentration of 32 mg/L.
Time–kill assay
Strains were grown to an OD600 of ∼0.25 in LB medium. At this point, cells were incubated in the presence of antibiotics and/or AIA-1 for various periods of time. Biapenem, tobramycin and AIA-1 were used at a concentration of 32 mg/L and levofloxacin was used at 4 mg/L. The number of viable cells was measured by culturing cells on LB agar plates. By assuming that survival at time 0 was 100%, cfu values were converted to percentages.
Virulence factor production
In elastase production, PAO1 was grown to an OD600 of 0.25. Then, cells were incubated in the presence or absence of 32 mg/L AIA-1 for 48 h. Elastase production was assessed using the elastin Congo red (ECR) assay following the method of Zhu et al.23 Rhamnolipid production was evaluated using the method of Köhler et al.24 with the following modifications: M9-based agar plates containing 32 mg/L AIA-1 were used and rhamnolipid production was measured as the diameter of the halo surrounding the colony. Pyocyanin production was measured using the method of Déziel et al.25 with the following modifications: PAO1 was grown to an OD600 of 0.25 in 2 mL of LB medium. Then, cells were incubated in the presence or absence of 32 mg/L AIA-1 for 24 h.
Biofilm assay
Biofilms were grown using the MBEC physiology and genetics assay kit (Innovotech Inc., Edmonton, Canada).26,27 In order to examine the effects of AIA-1 on biofilm formation, cultures were incubated in the presence or absence of 32 mg/L AIA-1 at 37°C for 24 h. In the killing assay for biofilm cells, biofilms were exposed to 32 mg/L AIA-1 at 37°C for 1 h. Biofilms were then exposed to biapenem 32 mg/L, tobramycin 32 mg/L or levofloxacin 2 mg/L, at 37°C for 24 h. Biofilm cells were disrupted by sonication and bacterial survival was determined by culturing cells on LB agar plates. By assuming that survival before AIA-1 exposure was 100%, cfu values were converted to percentages.
RNA isolation, reverse transcription and quantitative RT–PCR
PAO1 was grown to an OD600 of 0.25. At this point, cultures were incubated in the presence or absence of 32 mg/L AIA-1. At 2, 4 and 8 h after the addition of AIA-1, total RNA was isolated using an RNeasy Mini Kit (Qiagen, Valencia, CA, USA). A Transcriptor First Strand cDNA Synthesis Kit (Roche Diagnosis, Mannheim, Germany) was used for reverse transcription. qRT–PCR was performed using StepOnePlus™ Real-Time PCR System with Fast SYBR® Green Master Mix (Thermo Fisher Scientific, MA, USA). Expression was normalized to rpsL.
RpoS purification, antisera production and immunoblot analysis
BL21 cells expressing RpoS-GST (pGEX-6P-2-rpoS) were grown in LB at 37°C. Cells were centrifuged and resuspended in PBS. Cells were then lysed by sonication, proteins were purified by affinity chromatography and the GST tag was cleaved with PreScission Protease (GE Healthcare UK Ltd, UK). Antisera were generated in rabbits using a standard protocol (Scrum Inc., Tokyo, Japan).
PAO1 was grown to an OD600 of 0.25 in LB medium, continuing the incubation in the presence or absence of 32 mg/L AIA-1. At 4 or 8 h after the addition of AIA-1, cell pellets were resuspended in PBS and lysed by sonication. Immunoblots were performed with cell lysates with equal amounts of total protein in each lane. Protein concentrations were measured using the Bradford assay (Bio-Rad, Hercules, CA, USA) before being loaded onto a 4%–15% pre-cast SDS–PAGE gel (Bio-Rad). Proteins were transferred to PVDF membrane. RpoS proteins were detected using an α-RpoS antibody (1:2000) and α-rabbit horseradish peroxidase-conjugated antibody (1:20 000, Thermo Scientific). Protein bands were visualized on X-ray film with the use of the ECL Prime Western Blotting Detection System (GE Healthcare).
Results
AIA-1 does not affect antibiotic susceptibility
The MICs of antibiotics and AIA-1 are shown in Table 2. The MIC of AIA-1 ranged between 64 and 128 mg/L, demonstrating very weak antibacterial activity against P. aeruginosa. In the presence of 32 mg/L AIA-1, the MICs of the antibiotics did not change, indicating that AIA-1 did not affect susceptibility to antibiotics.
Strain . | MIC (mg/L) . | ||||||
---|---|---|---|---|---|---|---|
BIA . | LVX . | TOB . | AIA-1 . | BIA+AIA-1 . | LVX+AIA-1 . | TOB+AIA-1 . | |
PAO1 | 0.5 | 0.5 | 2 | 128 | 0.5 | 0.5 | 2 |
PAO1 plac-lux | 1 | 0.5 | 2 | 64 | 1 | 0.5 | 2 |
PAO1 ΔlasIΔrhlI | 0.25 | 1 | 2 | 128 | 0.25 | 1 | 2 |
PAO1 ΔrpoS | 1 | 1 | 2 | 128 | 1 | 1 | 2 |
PAO1/pJN105 | 0.5 | 0.5 | 8 | 128 | 0.5 | 0.5 | 4 |
PAO1/pJN105-rpoS | 0.5 | 0.5 | 8 | 128 | 0.5 | 0.25 | 8 |
Strain . | MIC (mg/L) . | ||||||
---|---|---|---|---|---|---|---|
BIA . | LVX . | TOB . | AIA-1 . | BIA+AIA-1 . | LVX+AIA-1 . | TOB+AIA-1 . | |
PAO1 | 0.5 | 0.5 | 2 | 128 | 0.5 | 0.5 | 2 |
PAO1 plac-lux | 1 | 0.5 | 2 | 64 | 1 | 0.5 | 2 |
PAO1 ΔlasIΔrhlI | 0.25 | 1 | 2 | 128 | 0.25 | 1 | 2 |
PAO1 ΔrpoS | 1 | 1 | 2 | 128 | 1 | 1 | 2 |
PAO1/pJN105 | 0.5 | 0.5 | 8 | 128 | 0.5 | 0.5 | 4 |
PAO1/pJN105-rpoS | 0.5 | 0.5 | 8 | 128 | 0.5 | 0.25 | 8 |
BIA, biapenem; LVX, levofloxacin; TOB, tobramycin.
Strain . | MIC (mg/L) . | ||||||
---|---|---|---|---|---|---|---|
BIA . | LVX . | TOB . | AIA-1 . | BIA+AIA-1 . | LVX+AIA-1 . | TOB+AIA-1 . | |
PAO1 | 0.5 | 0.5 | 2 | 128 | 0.5 | 0.5 | 2 |
PAO1 plac-lux | 1 | 0.5 | 2 | 64 | 1 | 0.5 | 2 |
PAO1 ΔlasIΔrhlI | 0.25 | 1 | 2 | 128 | 0.25 | 1 | 2 |
PAO1 ΔrpoS | 1 | 1 | 2 | 128 | 1 | 1 | 2 |
PAO1/pJN105 | 0.5 | 0.5 | 8 | 128 | 0.5 | 0.5 | 4 |
PAO1/pJN105-rpoS | 0.5 | 0.5 | 8 | 128 | 0.5 | 0.25 | 8 |
Strain . | MIC (mg/L) . | ||||||
---|---|---|---|---|---|---|---|
BIA . | LVX . | TOB . | AIA-1 . | BIA+AIA-1 . | LVX+AIA-1 . | TOB+AIA-1 . | |
PAO1 | 0.5 | 0.5 | 2 | 128 | 0.5 | 0.5 | 2 |
PAO1 plac-lux | 1 | 0.5 | 2 | 64 | 1 | 0.5 | 2 |
PAO1 ΔlasIΔrhlI | 0.25 | 1 | 2 | 128 | 0.25 | 1 | 2 |
PAO1 ΔrpoS | 1 | 1 | 2 | 128 | 1 | 1 | 2 |
PAO1/pJN105 | 0.5 | 0.5 | 8 | 128 | 0.5 | 0.5 | 4 |
PAO1/pJN105-rpoS | 0.5 | 0.5 | 8 | 128 | 0.5 | 0.25 | 8 |
BIA, biapenem; LVX, levofloxacin; TOB, tobramycin.
AIA-1 enhances biapenem activity in mouse infection
The bioluminescence images obtained in mice infected with P. aeruginosa plac-lux showed no apparent therapeutic effects of the treatment with AIA-1 alone. However, markedly stronger therapeutic effects were observed in mice infected with the P. aeruginosa plac-lux strain when AIA-1 was used in combination with biapenem than when biapenem alone was used (Figure 2a and b). These therapeutic effects were confirmed by counting the number of surviving cells in the thigh after 10 h. Reductions in the numbers of cells were significantly greater with the combined treatment of AIA-1 and biapenem than with biapenem alone (Figure 2c).
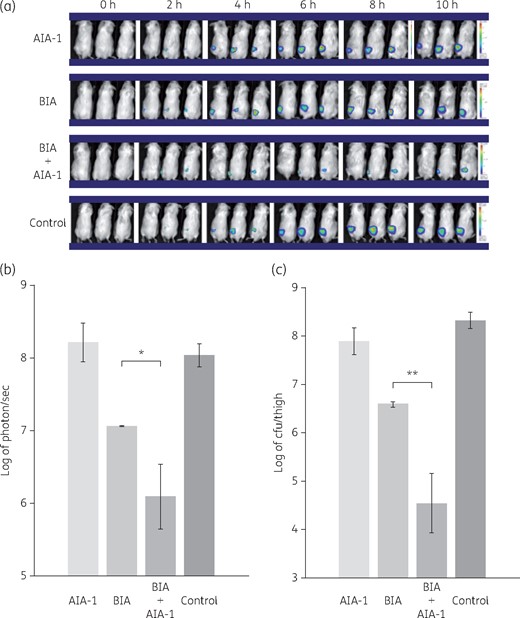
Effects of biapenem (BIA) and/or AIA-1 on in vivo PAO1 plac-lux bioluminescence signals. PAO1 plac-lux (106 cfu) was inoculated into one thigh of each animal. Therapy was performed with biapenem alone or combined with AIA-1, or AIA-1 alone. (a) Representative in vivo bioluminescence in colour-scale images of thighs of mice. (b) Bacterial counts as measured by in vivo bioluminescence (photons/s) at 10 h. (c) Bacterial counts as measured by cfu at 10 h. *P < 0.05; **P < 0.01 (Student’s t-test). Error bars show SDs for three experiments. This figure appears in colour in the online version of JAC and in black and white in the print version of JAC.
AIA-1 impacts biapenem tolerance without affecting QS
Since QS plays an important role in modulating responses to antimicrobials, we attempted to evaluate the influence of AIA-1 on QS. In order to achieve this, we initially performed time–kill assay on PAO1 in the presence of AIA-1 at a concentration of ¼ MIC (32 mg/L). No killing effects were observed in the presence of 32 mg/L AIA-1 alone, which was consistent with the results obtained in the mouse infection model. However, survival at the 24 h timepoint was 100-fold lower with the combined use of biapenem and AIA-1 than with biapenem alone (Figure 3a). This result prompted us to propose a potential role for AIA-1 in the inhibition of QS, which may explain increased susceptibility to biapenem. In order to confirm this hypothesis, we constructed a lasI, rhlI double deletion mutant (PAO1ΔlasIΔrhlI), and performed a killing assay in the presence of biapenem. The results obtained indicated that the survival rate of PAO1ΔlasIΔrhlI did not differ from that of PAO1 (Figure 3b). We then examined the effects of the combined use of biapenem and AIA-1 on the survival rate of PAO1ΔlasIΔrhlI. As suspected, the combination of AIA-1 and biapenem was more effective than biapenem alone (Figure S1, available as Supplementary data at JAC Online). Collectively, these results suggest that las and rhl QS are not directly involved in biapenem tolerance and also that the observed effects of AIA-1 on biapenem tolerance appear to be independent of QS.
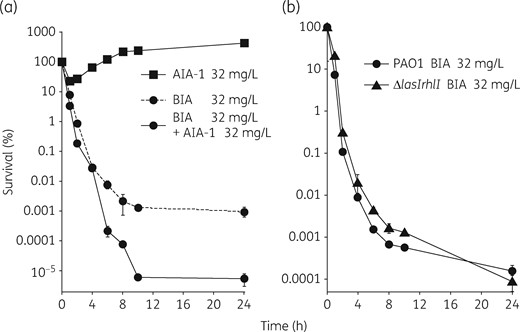
Time–kill assays for PAO1 and PAO1ΔlasIΔrhlI using biapenem (BIA). (a) Time–kill assay for PAO1 in the presence of biapenem and/or AIA-1 at 32 mg/L. (b) Time–kill assay for PAO1 and PAO1ΔlasIΔrhlI in the presence of biapenem at 32 mg/L. Assuming that survival at time 0 was 100%, cfu values were converted to percentages. Experiments were performed in triplicate. Error bars, show SDs for three experiments.
No suppression of virulence factor production by AIA-1
In order to clarify the effects of AIA-1 as an autoinducer analogue on QS, we measured the levels of elastase, pyocyanin and rhamnolipids, which are directly controlled by QS, in the presence of AIA-1 at a concentration of 32 mg/L. Our results demonstrated that AIA-1 did not suppress the production of elastase, pyocyanin or rhamnolipids (Figure 4a–c). This result prompted us to further establish the expression levels of lasB and rhlA genes, which are also regulated by LasRI and RhlRI, respectively. The results obtained corroborated those observed for virulence factor production, indicating that AIA-1 did not directly exert its effects on QS because its presence was not associated with altered levels of the lasB or rhlA gene (Figure 4d).
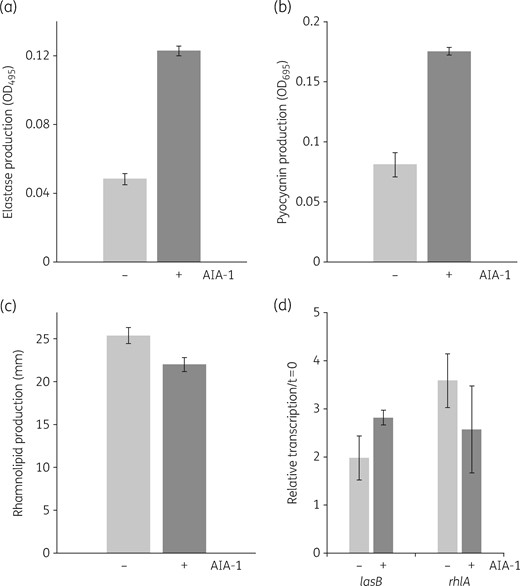
Effects of AIA-1 on the production of P. aeruginosa virulence factors. (a) Elastase production. (b) Pyocyanin production. (c) Rhamnolipid production. (d) Expression of lasB and rhlA in PAO1. A culture grown overnight was diluted 100-fold and grown to an OD600 of 0.2–0.25, and was then incubated with AIA-1 for 8 h. Transcription levels at 8 h were measured by qRT–PCR and standardized to rpsL expression. Each experiment was performed in triplicate. Error bars show SDs for three experiments.
AIA-1 affects survival against tobramycin and levofloxacin
We further investigated whether the observed effects of AIA-1 on tolerance are also related to other antimicrobials, such as aminoglycosides and fluoroquinolones, which have a mode of action that is different from that of carbapenem antibiotics. In order to achieve this, we assessed the effects of killing by tobramycin and levofloxacin in combination with AIA-1. In time–kill assays, survival rates at the 4 h timepoint were 10- or 100-fold lower with AIA-1 in combination with tobramycin or levofloxacin, respectively, than in the absence of AIA-1 (Figure 5a and b). We also performed combination therapy with tobramycin and AIA-1 in an in vivo mouse infection model. The combination of AIA-1 and tobramycin resulted in weaker bioluminescent signals and fewer cells than those with tobramycin alone (Figure 6a–c). These results suggest that AIA-1 may affect antibiotic tolerance through broad-spectrum activities .
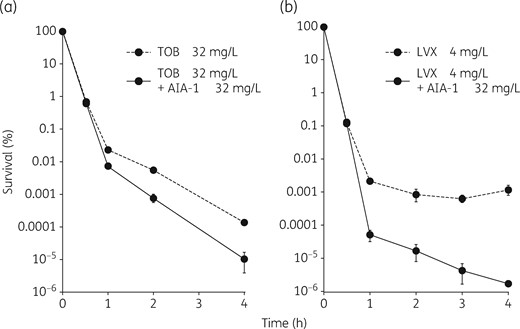
Time–kill assay for PAO1 using tobramycin (TOB) or levofloxacin (LVX). (a) Time–kill assay for PAO1 in the presence of tobramycin at 32 mg/L and/or AIA-1 at 32 mg/L. (b) Time–kill assay for PAO1 in the presence of levofloxacin at 4 mg/L and/or AIA-1 at 32 mg/L. Assuming that survival at time 0 was 100%, cfu values were converted to percentages. Experiments were performed in triplicate. Error bars show SDs for three experiments.
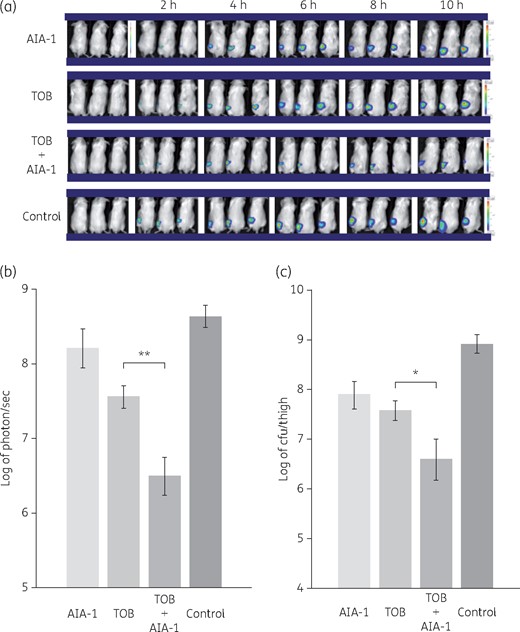
Effects of tobramycin and/or AIA-1 on in vivo PAO1 plac-lux bioluminescence signals. PAO1 plac-lux (2 × 106 cfu) was inoculated into one thigh of each animal. Therapy was performed with tobramycin (TOB) alone or combined with AIA-1, or AIA-1 alone. (a) Representative in vivo bioluminescence on colour-scale images of thighs of mice. (b) Bacterial counts as measured by in vivo bioluminescence (photons/s) at 10 h. (c) Bacterial counts as measured by cfu at 10 h. *P < 0.05; **P < 0.01 (Student’s t-test). Error bars show SDs for three experiments. This figure appears in colour in the online version of JAC and in black and white in the print version of JAC.
AIA-1 affects biofilm tolerance
There is increasing evidence to show that QS is essential for the formation of biofilm; therefore, we focused on the role that AIA-1 may play in biofilm formation. Our results demonstrated that AIA-1 at a concentration of 32 mg/L did not exert inhibitory effects on biofilm formation (Figure 7a). Conversely, the addition of AIA-1 in the killing assay in combination with biapenem at a concentration of 32 mg/L led to a survival rate for biofilm that was 40-fold lower than that observed for PAO1 in the presence of biapenem (Figure 7b). Similar results were obtained for the combination of AIA-1 and tobramycin (Figure 7c) or levofloxacin (Figure 7d), leading to ∼10- and 40-fold lower survival rates, respectively. These results suggest that AIA-1 at a concentration of 32 mg/L had no effect on biofilm formation; however, it increased the effect of antibiotics against biofilm cells.
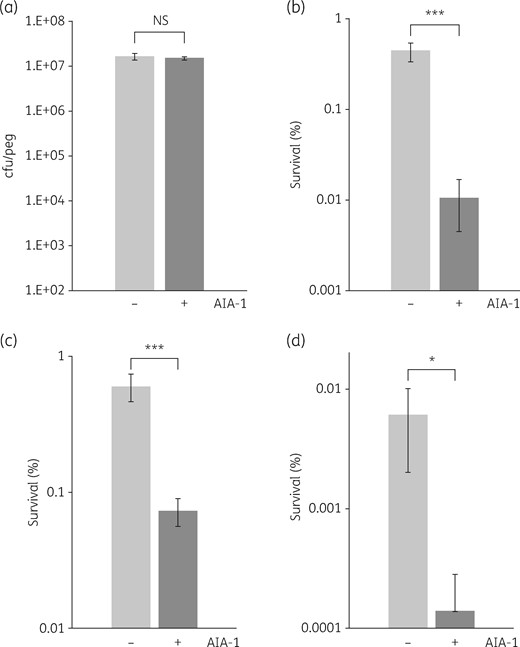
Efficacy of AIA-1 against PAO1 biofilms. (a) Effects of AIA-1 on PAO1 biofilm formation. Killing assay for biofilm cells using (b) 32 mg/L biapenem, (c) 32 mg/L tobramycin and (d) 2 mg/L levofloxacin. By assuming that survival before AIA-1 exposure was 100%, cfu values were converted to percentages. *P < 0.05; ***P < 0.001; NS, not significant (Student’s t-test). Error bars show SDs for four experiments.
AIA-1 acts on rpoS to modulate biapenem tolerance
The presence of AIA-1 in cells during exposures to various antibiotics has suggested that this analogue of the QS autoinducer is involved in modulating tolerance to antimicrobial agents. Since the regulation of QS is an important factor for defining responses to antibiotics, and in an attempt to find a potential link between AIA-1 and QS genes, we measured the expression of the lasR, rhlR, vqsR, qscR, rsmA, gacA, pqsA, rpoN and rpoS genes using qRT–PCR. Although no significant differences were observed in the expression profiles of the lasR, rhlR, vqsR, qscR, rsmA, gacA, pqsA and rpoN genes in the presence of AIA-1 (Figure S2), the rpoS gene demonstrated significant changes in its expression profile at the 8 h timepoint following the addition of AIA-1. The transcriptional levels of rpoS were up-regulated in a time-dependent manner in control cells, whereas rpoS expression was clearly inhibited in the presence of AIA-1 (Figure 8a). We performed western blot analysis to measure rpoS gene expression with or without AIA-1. In addition to the results of qRT–PCR, the western blot analysis showed that rpoS gene expression was clearly repressed by AIA-1 at 4 and 8 h (Figure 8b). In order to further understand the importance of AIA-1 in regulatory control over rpoS, we constructed an rpoS in-frame deletion mutant and performed a time–kill assay with biapenem. The survival rate of the PAO1ΔrpoS mutant at the 24 h timepoint was ∼10-fold lower than that of PAO1 (Figure 8c). We also constructed a strain containing rpoS cloned under the control of the arabinose-inducible promoter for expression studies, and performed time–kill assays in the presence of biapenem and AIA-1. The strain overexpressing rpoS had a survival rate at the 24 h timepoint that was 10-fold higher than that of PAO1 bearing an empty vector (Figure 8d). A similar tendency was observed with tobramycin; however, the rpoS gene had little effect with levofloxacin (Figure S3). These results indicated that AIA-1 exerts its effects on antibiotic tolerance partially by repressing the expression of the rpoS gene and the role of the rpoS gene depends on the specific antibiotic.
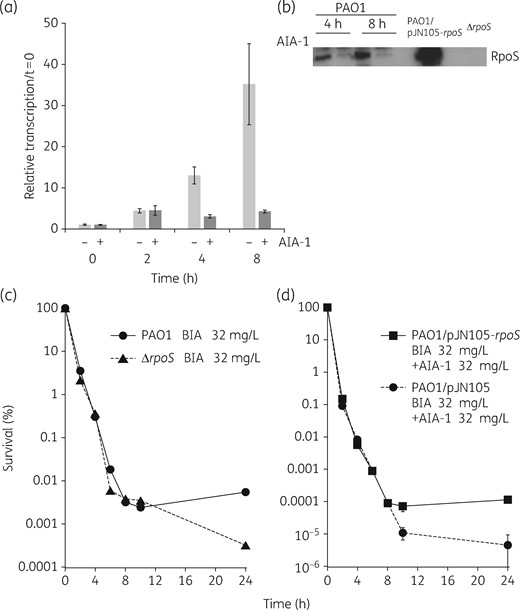
(a) Expression levels of the rpoS gene in PAO1. A culture grown overnight was diluted 100-fold, grown to an OD600 of 0.2–0.3, and then harvested at 2, 4, and 8 h following growth in the presence or absence of AIA-1 at 32 mg/L. Transcription levels were measured by qRT–PCR and standardized to rpsL expression. (b) Western blot analysis of the rpoS gene. An overnight culture was diluted 100-fold, grown to an OD600 of 0.2–0.25, and then harvested at 4 and 8 h in the presence or absence of AIA-1 at 32 mg/L. (c) Time–kill assay for PAO1 or PAO1ΔrpoS in the presence of biapenem (BIA) at 32 mg/L. (d) Time–kill assay for PAO1/pJN105 or PAO1/pJN105-rpoS in the presence of biapenem and AIA-1 at 32 mg/L supplemented with 0.2% arabinose. Assuming that survival at time 0 was 100%, cfu values were converted to percentages. Experiments were performed in triplicate. Error bars show SDs for three experiments.
Discussion
We herein present a new compound, AIA-1, an analogue of the P. aeruginosa autoinducer OdDHL, which enhanced the antibacterial effects of biapenem in an in vivo mouse infection model (Figure 2) and an in vitro time–kill assay (Figure 3). AIA-1 also enhanced the antibacterial effects of other antibiotics, such as levofloxacin (Figure 5b) and tobramycin (Figures 5a and 6). These effects were observed not only in planktonic cells, but also in biofilm cells (Figure 7). AIA-1 did not induce changes in the MICs of antibiotics (Table 2), suggesting that it exerted its effects on antibiotic tolerance without affecting MIC levels.
Antibiotic tolerance is clinically important because bacterial cells that acquire antibiotic tolerance cause infections that are often recalcitrant to antibiotic therapy. Therefore, new strategies are required in order to overcome antibiotic tolerance. A previous study reported that QS inhibitors (QSIs) such as baicalin hydrate (BH) and cinnamaldehyde (CA) increased the susceptibility of P. aeruginosa biofilms to tobramycin in in vitro and in vivo models.28 Pan et al.29 showed that synthetic brominated furanone restored the antibiotic susceptibility of PAO1 planktonic and biofilm-associated persister cells. In addition, this compound was effective against biofilm cells of the P. aeruginosa mucoid-type strain PDO300, which had higher tolerance to tobramycin than wild-type biofilms.30 Starkey et al.31 reported that multiple virulence factor regulator (MvfR) regulon QSIs restrict the ability of bacteria to form antibiotic-tolerant cells and consequently restrict P. aeruginosa persistent infections in mice. Concordant with the mechanism of action observed for the compound used in this study, it was demonstrated that these compounds did not affect the MICs of antibiotics, but rather decreased the antibiotic tolerance of P. aeruginosa. Starkey et al.31 also proposed that LasR-regulated QSIs may have less clinical applicability than MvfR inhibitors because of the frequent emergence of lasR mutants in human clinical isolates. Although AIA-1 is an analogue of the LasR autoinducer OdDHL, this compound did not inhibit the Las-Rhl QS system (Figure 4). This result suggests that the effects of AIA-1 on antibiotic tolerance are independent of QS and also that other mechanisms are involved.
Antibiotic tolerance is defined as the ability of bacteria to survive, but not grow, in the presence of antibiotics, and is not caused by a gene mutation, but by the presence of dormant phenotypic variants of cells, referred to as persisters.32 Several environmental conditions induce tolerant phenotypes such as nutrient starvation,9 entry into the stationary phase10 and biofilm formation.6 In addition, Que et al.33 reported that 2′-amino-acetophenone (2-AA) promoted antibiotic tolerance in response to QS signalling not only in P. aeruginosa, but also in the non-2-AA producer Acinetobacter baumannii, implying that 2-AA plays a common important role in antibiotic tolerance in Gram-negative bacteria.
We previously reported that the rpoS gene has a crucial role in antibiotic tolerance;13,14 therefore, one of the aims of this study was to investigate the potential effects of AIA-1 on the expression of rpoS. The results of the transcriptional analysis demonstrated that rpoS levels are negatively affected by the presence of AIA-1 (Figure 8a and b). In time–kill assays, the survival rate of the rpoS mutant at the 24 h timepoint was lower than that of PAO1 (Figure 8c). In addition, AIA-1 had no effects on the rpoS-overexpressing strain in the presence of biapenem (Figure 8d). These results indicate that rpoS is partially involved in antibiotic tolerance, and that AIA-1 acts on rpoS by suppressing its expression, thereby decreasing antibiotic tolerance.
In P. aeruginosa, RpoS is a central regulator of the general stress response and regulates ∼700 genes in the stationary phase.34 The regulation of RpoS is very complex and the synthesis of RpoS is also regulated by several factors, including ppGpp, which is an alarmone and the regulator of stringent responses.35 RpoS and QS have been shown to mutually regulate each other.36–39 In the present study, the lasR, rhlR, relA, rsmA and gacA genes were not affected by AIA-1, whereas rpoS expression was significantly affected; therefore, the relationship between AIA-1 and rpoS requires further study.
In conclusion, the P. aeruginosa QS autoinducer analogue AIA-1 markedly decreased antibiotic tolerance of planktonic and biofilm cells in P. aeruginosa not only in vitro, but also in vivo. This effect appears to be partially due to the suppression of rpoS gene expression. Future studies will focus on investigating other mechanisms of action of AIA-1 in antibiotic tolerance as well as its efficacy against antibiotic-resistant strains.
Funding
This work was supported by JSPS KAKENHI Grant Number JP 25461512.
Transparency declarations
None to declare.
Supplementary data
Figures S1 to S3 are available as Supplementary data at JAC Online.