-
PDF
- Split View
-
Views
-
Cite
Cite
Sushmita D. Lahiri, Patricia A. Bradford, Wright W. Nichols, Richard A. Alm, Structural and sequence analysis of class A β-lactamases with respect to avibactam inhibition: impact of Ω-loop variations, Journal of Antimicrobial Chemotherapy, Volume 71, Issue 10, October 2016, Pages 2848–2855, https://doi.org/10.1093/jac/dkw248
- Share Icon Share
Abstract
There exists a significant diversity among class A β-lactamases and the proliferation of these enzymes is a significant medical concern due to the ability of some members to efficiently hydrolyse both extended-spectrum cephalosporins and carbapenems. Avibactam is a novel non-β-lactam β-lactamase inhibitor that, in combination with ceftazidime, has recently obtained regulatory approval in the USA. Although avibactam is known to efficiently inhibit key class A enzymes, the diversity of this enzyme family warranted a more complete investigation to understand the breadth of the potential spectrum of inhibition.
Using the known residues critical for avibactam binding, a thorough structural and sequence-based conservation analysis was performed across >650 class A enzymes. Several variations that had the potential to impact avibactam inhibition were observed and representative enzymes were cloned and expressed isogenically to evaluate the impact of these variations.
The majority of the key residues involved in avibactam binding were well conserved across the different sub-families of class A β-lactamases, although some differences were observed. The differences in the Ω-loop of PER enzymes were found to impact the ability of avibactam to effectively protect β-lactams against hydrolysis. However, substitutions in a key hydrogen-bonding residue (N170) in some of the GES variants were found to not have a significant impact on avibactam inhibition.
Overall, the computational and experimental analyses suggest that the vast majority of class A β-lactamases should be well inhibited by avibactam, although a very small number of outliers exist.
Introduction
A critical need exists for new antibacterial agents for the treatment of infections caused by resistant Gram-negative bacteria.1,2 In particular, the clinical value of β-lactam drugs to treat these infections is being compromised by the prolific expansion of ESBL and carbapenemase enzymes3 and the dissemination of β-lactamase-producing clones such as those that occur within the population of clinical isolates of Klebsiella pneumoniae.4 Of the four Ambler groups of β-lactamases, the class A structural fold has evolved the most diverse substrate specificity and functional diversity using the same catalytic motifs.5 Analyses of the various class A structures have shown that this functional diversity often results from minor changes in the amino acid sequence, which can be located at a significant distance from the catalytic site. Additionally, these changes prevent many of the class A enzymes from being efficiently inhibited by clavulanic acid, tazobactam and sulbactam.6
Avibactam, a novel non-β-lactam β-lactamase inhibitor, potentially addresses part of this medical need.7 This inhibitor, based on a diazabicyclooctane (DBO) core scaffold, has been shown to have a broad β-lactamase inhibition profile which includes class A, class C and some of the class D enzymes.8–10 The combination of avibactam and ceftazidime, in advanced clinical development,11 has been approved by the US FDA for use in adults with certain infections who have limited or no alternative treatment options.12
It is impractical to study the inhibitory activity of avibactam at the level of enzyme kinetics or to obtain structural information against all, or even most, existing β-lactamases, and the approach has been to make such detailed studies on clinically important example enzymes of the different structural classes.8–10,13–17 The extent to which this inhibition of β-lactamases translates to restoration of ceftazidime antibacterial activity has then been evaluated against greater numbers of β-lactamases at the microbiological level by measuring the MIC or MBC values of ceftazidime/avibactam against molecularly characterized clinical isolates,18–23 as well as in surveillance of general clinical isolates.24–28
The broader understanding of the inhibition of multiple β-lactamase variants by avibactam at the molecular level has also been informed by structure-based analyses based on crystal structures of avibactam in complex with representative enzymes from class A (CTX-M-15), class C (AmpC) and class D (OXA-24 and OXA-48).14–16 The rigid binding mode of avibactam is conserved in all three classes and any variability in inhibition is either due to changes in the structural loops close to the binding pocket or due to changes in residues that interact with avibactam directly.16,29,30 Previously, we analysed the level of conservation of the avibactam binding pockets in a large number of class C and class D enzymes.15,16 In the present work, we apply structural predictive analyses to establish a broad predictive understanding of the inhibition by avibactam of class A β-lactamases of widely diverse structures, including the variants of TEM, SHV, CTX-M, KPC, GES and PER β-lactamases, and also extended these predictions to other class A variants. Furthermore, for several enzyme classes where there was a potential risk for decreased avibactam inhibition, representative isoforms were cloned and characterized in more detail to enable a complete understanding of the spectrum of class A β-lactamase inhibition by avibactam.
Materials and methods
Antimicrobial susceptibility testing
The MIC for each isolate was determined using the broth microdilution method following the CLSI guidelines.31Escherichia coli ATCC 25922 and K. pneumoniae ATCC 700603 were used as the quality control isolates. Aztreonam was obtained from MP Biomedicals (Santa Ana, CA, USA). Meropenem, amoxicillin, cefuroxime and clavulanic acid were all obtained from US Pharmacopeia (Rockville, MD, USA). Ceftaroline (the active metabolite of ceftaroline fosamil) and ceftazidime were provided by Cerexa (Oakland, CA, USA) and GlaxoSmithKline, respectively. Avibactam was provided by AstraZeneca.
Cloning of representative GES and PER enzymes
Surveillance isolates were provided by International Health Management Associates (Schaumburg, IL, USA). Isolates were sequenced using the Illumina MiSeq platform and whole genome data were analysed using CLC Bio Workbench software v. 5.5.1 (CLC Bio, Cambridge, MA, USA). Specific GES and PER isozymes were PCR amplified from representative isolates using High-Fidelity PCR Mix (Roche, Nutley, NJ, USA) along with 50 ng genomic DNA as template and specific oligonucleotides designed from the genomic sequences that also incorporated BamHI and SacI restriction endonuclease sites at the 5′ and 3′ ends, respectively. The genes for CTX-M-15 and Pseudomonas aeruginosa AmpC (PDC-1) were also amplified as control enzymes. The PCR products were purified using a QiaQuick PCR purification kit (Qiagen, Valencia, CA, USA), digested with restriction enzymes (ThermoFisher, Waltham, MA, USA) and ligated into the pSU19 vector.32 The ligation products were transformed into E. coli DH5α competent cells with chloramphenicol (20 mg/L) selection. Resultant transformants were sequence verified to ensure the integrity of the cloned β-lactamase gene.
Sequence and structural analyses
The class A β-lactamase sequences were compiled from GenBank using the accession numbers provided in the curated Lahey database (www.lahey.org/Studies), a database that has recently been transferred to NCBI for ongoing curation (http://www.ncbi.nlm.nih.gov/pathogens/submit_beta_lactamase/). Multiple sequence alignments were generated using the Clustal X program. The bioinformatics tool ConSurf (www.consurf.tau.ac.il) was used to map the multiple sequence alignment to the avibactam crystal structure bound to a representative class A enzyme, CTX-M-1513 (PDB:4HBU), to visualize the three-dimensional sequence diversity. Pymol (www.pymol.org) was used to display the diversity, overlay the different β-lactamase structures, and also to perform a virtual mutation to depict the residue substitutions identified in the resistant variants.
Results and discussion
Structural conservation of the avibactam binding pocket in class A β-lactamases
In a previous study, the crystal structure of avibactam, solved in complex with the representative class A β-lactamase CTX-M-15, provided insight into the residues involved in the binding of avibactam to the active site, particularly in the covalently modified form.14 There were 12 residues that either made interactions with avibactam or were close enough that certain substitutions might impact binding efficiency (Figure 1). Four of these, S70, K73, S130 and E166, are catalytic residues that are involved in the acylation and deacylation of avibactam. Five other residues, N104, N132, N170, K234 and T235, make strong hydrogen bonds to either the sulphonate or carboxamide groups. Of the remaining three, Y105 makes a hydrophobic interaction with the six-membered ring of avibactam, whereas T216 makes no direct interaction with the inhibitor. Although the S237 residue makes a hydrogen bond with the sulphonate group of avibactam, this interaction is not strong as multiple conformations of S237 were observed in the higher resolution structure of avibactam complexed with CTX-M-15.14 Because both T216 and S237 are in close proximity to avibactam, it is possible that certain larger substitutions could impair the ability of avibactam to bind. Indeed, a substitution in the equivalent location of a class C enzyme that resulted in reduced avibactam activity has been observed in laboratory-selected resistant mutants.15
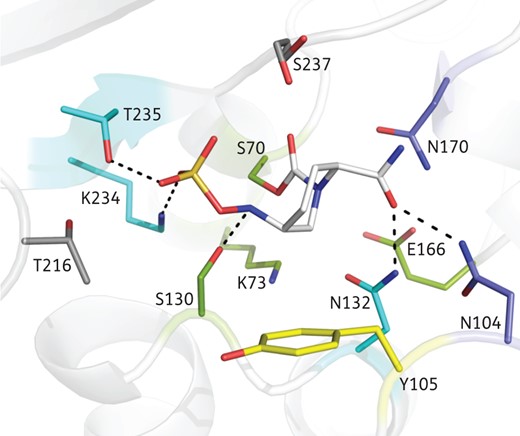
Avibactam binding pocket in class A β-lactamase. Avibactam (depicted as white sticks) covalently bound to a representative class A structure (CTX-M-15; 4HBU13) shows that 12 residues (using standard class A numbering) in the binding pocket are within 4 Å, such that changes in these might impact inhibitor affinity. The four catalytic residues (S70, K73, S130 and E166) are shown in green and the three that make strong hydrogen bonds (N132, K234 and T235) are shown in cyan. The two additional polar residues (N104 and N170) are shown in blue and the one hydrophobic residue (Y105) is shown in yellow. There are two residues (T216 and S237) shown in grey that, although in close proximity, have no direct interaction with the inhibitor.
To understand the structural diversity of the avibactam binding pocket, all class A β-lactamase structures available in the public domain were analysed by overlaying them onto the CTX-M-15::avibactam structure and assessing the variability in the binding region. The very rigid binding mode of avibactam observed in CTX-M-15, as well as representative class C and class D enzymes,14–16 enables direct overlays of the other class A β-lactamase structures. The largest groups, with more than a hundred variants each, are the TEM, the SHV and the CTX-M β-lactamases (Table 1) with large variations in substrate specificity that range from penicillinase and narrow-spectrum cephalosporinase activity to the ESBL phenotype. A comparison of the representative structures from these functional subgroups with the CTX-M-15::avibactam structure demonstrated that the avibactam-binding region remained well conserved across most groups (Figure 2a) and enabled predictions as to the ability of avibactam to bind to the other class A enzyme subgroups. This similarity included both the secondary structures and the loop regions around the binding pocket, as well as the conservation of the key interacting residues in the binding pocket (Figure 1). Any structural changes that result in the diversity of the β-lactam spectrum in these groups should not impact the efficiency of avibactam binding.
Conservation of key residues required for binding of avibactam among class A β-lactamases
Group . | Number . | Ser70 . | Lys73 . | Asn104c . | Tyr105c . | Ser130 . | Asn132 . | Glu166 . | Asn170 . | Thr216 . | Lys234 . | Thr235 . | Ser237c . |
---|---|---|---|---|---|---|---|---|---|---|---|---|---|
CTX-M | 131 | 131 | 131 | 131 | 131 | 131 | 130e | 131 | 131 | 130h | 129i | 131 | 130 |
SHV | 160 | 159b | 160 | 0 | 160 | 159d | 160 | 159f | 160 | 0h | 156i | 159j | 0 |
TEM | 188 | 188 | 188 | 0 | 186 | 184d | 188 | 187f | 187g | 0h | 188 | 0j | 0 |
GES | 25 | 25 | 25 | 0 | 0 | 24d | 25 | 25 | 2g | 25 | 25 | 25 | 0 |
PER | 7 | 7 | 7 | 0 | 0 | 6d | 7 | 7 | 0g | 7 | 7 | 7 | 0 |
VEB | 8 | 8 | 8 | 0 | 0 | 8 | 8 | 8 | 0g | 7h | 8 | 8 | 0 |
OXY | 23 | 23 | 23 | 0 | 0 | 23 | 23 | 23 | 23 | 23 | 23 | 23 | 0 |
OKP | 34 | 34 | 34 | 0 | 34 | 34 | 34 | 34 | 34 | 0h | 34 | 34 | 0 |
KPC | 17 | 17 | 17 | 0 | 0 | 17 | 17 | 17 | 17 | 17 | 17 | 17 | 0 |
LEN | 23 | 23 | 23 | 0 | 23 | 23 | 23 | 23 | 23 | 0h | 23 | 23 | 0 |
BEL | 3 | 3 | 3 | 0 | 3 | 3 | 3 | 3 | 3 | 3 | 3 | 3 | 3 |
CARB | 12 | 12 | 12 | 0 | 12 | 12 | 12 | 12 | 12 | 0h | 0i | 3j | 0 |
IMI | 4 | 4 | 4 | 0 | 1 | 4 | 4 | 4 | 4 | 4 | 4 | 4 | 4 |
KLUC | 4 | 4 | 4 | 4 | 4 | 4 | 4 | 4 | 4 | 4 | 4 | 4 | 4 |
SME | 4 | 4 | 4 | 0 | 1 | 4 | 4 | 4 | 4 | 4 | 4 | 4 | 4 |
Othersa | 18 | 18 | 18 | 15 | 15 | 18 | 18 | 18 | 16g | 18 | 18 | 18 | 15 |
Total | 661 | 660 | 661 | 150 | 572 | 654 | 660 | 659 | 618 | 242 | 645 | 463 | 160 |
Group . | Number . | Ser70 . | Lys73 . | Asn104c . | Tyr105c . | Ser130 . | Asn132 . | Glu166 . | Asn170 . | Thr216 . | Lys234 . | Thr235 . | Ser237c . |
---|---|---|---|---|---|---|---|---|---|---|---|---|---|
CTX-M | 131 | 131 | 131 | 131 | 131 | 131 | 130e | 131 | 131 | 130h | 129i | 131 | 130 |
SHV | 160 | 159b | 160 | 0 | 160 | 159d | 160 | 159f | 160 | 0h | 156i | 159j | 0 |
TEM | 188 | 188 | 188 | 0 | 186 | 184d | 188 | 187f | 187g | 0h | 188 | 0j | 0 |
GES | 25 | 25 | 25 | 0 | 0 | 24d | 25 | 25 | 2g | 25 | 25 | 25 | 0 |
PER | 7 | 7 | 7 | 0 | 0 | 6d | 7 | 7 | 0g | 7 | 7 | 7 | 0 |
VEB | 8 | 8 | 8 | 0 | 0 | 8 | 8 | 8 | 0g | 7h | 8 | 8 | 0 |
OXY | 23 | 23 | 23 | 0 | 0 | 23 | 23 | 23 | 23 | 23 | 23 | 23 | 0 |
OKP | 34 | 34 | 34 | 0 | 34 | 34 | 34 | 34 | 34 | 0h | 34 | 34 | 0 |
KPC | 17 | 17 | 17 | 0 | 0 | 17 | 17 | 17 | 17 | 17 | 17 | 17 | 0 |
LEN | 23 | 23 | 23 | 0 | 23 | 23 | 23 | 23 | 23 | 0h | 23 | 23 | 0 |
BEL | 3 | 3 | 3 | 0 | 3 | 3 | 3 | 3 | 3 | 3 | 3 | 3 | 3 |
CARB | 12 | 12 | 12 | 0 | 12 | 12 | 12 | 12 | 12 | 0h | 0i | 3j | 0 |
IMI | 4 | 4 | 4 | 0 | 1 | 4 | 4 | 4 | 4 | 4 | 4 | 4 | 4 |
KLUC | 4 | 4 | 4 | 4 | 4 | 4 | 4 | 4 | 4 | 4 | 4 | 4 | 4 |
SME | 4 | 4 | 4 | 0 | 1 | 4 | 4 | 4 | 4 | 4 | 4 | 4 | 4 |
Othersa | 18 | 18 | 18 | 15 | 15 | 18 | 18 | 18 | 16g | 18 | 18 | 18 | 15 |
Total | 661 | 660 | 661 | 150 | 572 | 654 | 660 | 659 | 618 | 242 | 645 | 463 | 160 |
aThe 18 others include 9 FONA variants, 3 RAHN variants, BES-1, IBC-1, IBC-2, SMO-1, SFO-1 and LEMI.
bSHV-179 has Asn70.
cThe variation in this residue is detailed in Table 2.
dSHV-10 has a Gly130; TEM-211 has Thr130 and three variants (TEM-59, TEM-76 and TEM-89) have Gly130; GES-10 has Cys130; and PER-4 has Thr130.
eCTX-M-81 has His132.
fSHV-122 and TEM-193 have Gly166.
gTEM-193 has Thr170; all PER and VEB variants have His170; 10 GES variants (4, 5, 6, 14, 15, 16, 18, 20, 21 and 24) have Ser170, whereas 13 GES variants (1, 3, 7, 7variant, 8, 9, 10, 11, 12, 17, 19, 22 and 23) have Gly170; and both IBC variants have Gly170.
hCTX-M-45 has Tyr216; VEB-8 has Ala216; and all the SHV, TEM, OKP, LEN and CARB variants have Val216.
iCTX-M-106, CTX-M-107, SHV-56, 72, 73 and 84, and all CARB variants have Arg234.
jSHV-107 has Ala235; all TEM variants and nine CARB variants (1, 2, 3, 4, 6, 7, 9, 11 and 12) have Ser235.
Conservation of key residues required for binding of avibactam among class A β-lactamases
Group . | Number . | Ser70 . | Lys73 . | Asn104c . | Tyr105c . | Ser130 . | Asn132 . | Glu166 . | Asn170 . | Thr216 . | Lys234 . | Thr235 . | Ser237c . |
---|---|---|---|---|---|---|---|---|---|---|---|---|---|
CTX-M | 131 | 131 | 131 | 131 | 131 | 131 | 130e | 131 | 131 | 130h | 129i | 131 | 130 |
SHV | 160 | 159b | 160 | 0 | 160 | 159d | 160 | 159f | 160 | 0h | 156i | 159j | 0 |
TEM | 188 | 188 | 188 | 0 | 186 | 184d | 188 | 187f | 187g | 0h | 188 | 0j | 0 |
GES | 25 | 25 | 25 | 0 | 0 | 24d | 25 | 25 | 2g | 25 | 25 | 25 | 0 |
PER | 7 | 7 | 7 | 0 | 0 | 6d | 7 | 7 | 0g | 7 | 7 | 7 | 0 |
VEB | 8 | 8 | 8 | 0 | 0 | 8 | 8 | 8 | 0g | 7h | 8 | 8 | 0 |
OXY | 23 | 23 | 23 | 0 | 0 | 23 | 23 | 23 | 23 | 23 | 23 | 23 | 0 |
OKP | 34 | 34 | 34 | 0 | 34 | 34 | 34 | 34 | 34 | 0h | 34 | 34 | 0 |
KPC | 17 | 17 | 17 | 0 | 0 | 17 | 17 | 17 | 17 | 17 | 17 | 17 | 0 |
LEN | 23 | 23 | 23 | 0 | 23 | 23 | 23 | 23 | 23 | 0h | 23 | 23 | 0 |
BEL | 3 | 3 | 3 | 0 | 3 | 3 | 3 | 3 | 3 | 3 | 3 | 3 | 3 |
CARB | 12 | 12 | 12 | 0 | 12 | 12 | 12 | 12 | 12 | 0h | 0i | 3j | 0 |
IMI | 4 | 4 | 4 | 0 | 1 | 4 | 4 | 4 | 4 | 4 | 4 | 4 | 4 |
KLUC | 4 | 4 | 4 | 4 | 4 | 4 | 4 | 4 | 4 | 4 | 4 | 4 | 4 |
SME | 4 | 4 | 4 | 0 | 1 | 4 | 4 | 4 | 4 | 4 | 4 | 4 | 4 |
Othersa | 18 | 18 | 18 | 15 | 15 | 18 | 18 | 18 | 16g | 18 | 18 | 18 | 15 |
Total | 661 | 660 | 661 | 150 | 572 | 654 | 660 | 659 | 618 | 242 | 645 | 463 | 160 |
Group . | Number . | Ser70 . | Lys73 . | Asn104c . | Tyr105c . | Ser130 . | Asn132 . | Glu166 . | Asn170 . | Thr216 . | Lys234 . | Thr235 . | Ser237c . |
---|---|---|---|---|---|---|---|---|---|---|---|---|---|
CTX-M | 131 | 131 | 131 | 131 | 131 | 131 | 130e | 131 | 131 | 130h | 129i | 131 | 130 |
SHV | 160 | 159b | 160 | 0 | 160 | 159d | 160 | 159f | 160 | 0h | 156i | 159j | 0 |
TEM | 188 | 188 | 188 | 0 | 186 | 184d | 188 | 187f | 187g | 0h | 188 | 0j | 0 |
GES | 25 | 25 | 25 | 0 | 0 | 24d | 25 | 25 | 2g | 25 | 25 | 25 | 0 |
PER | 7 | 7 | 7 | 0 | 0 | 6d | 7 | 7 | 0g | 7 | 7 | 7 | 0 |
VEB | 8 | 8 | 8 | 0 | 0 | 8 | 8 | 8 | 0g | 7h | 8 | 8 | 0 |
OXY | 23 | 23 | 23 | 0 | 0 | 23 | 23 | 23 | 23 | 23 | 23 | 23 | 0 |
OKP | 34 | 34 | 34 | 0 | 34 | 34 | 34 | 34 | 34 | 0h | 34 | 34 | 0 |
KPC | 17 | 17 | 17 | 0 | 0 | 17 | 17 | 17 | 17 | 17 | 17 | 17 | 0 |
LEN | 23 | 23 | 23 | 0 | 23 | 23 | 23 | 23 | 23 | 0h | 23 | 23 | 0 |
BEL | 3 | 3 | 3 | 0 | 3 | 3 | 3 | 3 | 3 | 3 | 3 | 3 | 3 |
CARB | 12 | 12 | 12 | 0 | 12 | 12 | 12 | 12 | 12 | 0h | 0i | 3j | 0 |
IMI | 4 | 4 | 4 | 0 | 1 | 4 | 4 | 4 | 4 | 4 | 4 | 4 | 4 |
KLUC | 4 | 4 | 4 | 4 | 4 | 4 | 4 | 4 | 4 | 4 | 4 | 4 | 4 |
SME | 4 | 4 | 4 | 0 | 1 | 4 | 4 | 4 | 4 | 4 | 4 | 4 | 4 |
Othersa | 18 | 18 | 18 | 15 | 15 | 18 | 18 | 18 | 16g | 18 | 18 | 18 | 15 |
Total | 661 | 660 | 661 | 150 | 572 | 654 | 660 | 659 | 618 | 242 | 645 | 463 | 160 |
aThe 18 others include 9 FONA variants, 3 RAHN variants, BES-1, IBC-1, IBC-2, SMO-1, SFO-1 and LEMI.
bSHV-179 has Asn70.
cThe variation in this residue is detailed in Table 2.
dSHV-10 has a Gly130; TEM-211 has Thr130 and three variants (TEM-59, TEM-76 and TEM-89) have Gly130; GES-10 has Cys130; and PER-4 has Thr130.
eCTX-M-81 has His132.
fSHV-122 and TEM-193 have Gly166.
gTEM-193 has Thr170; all PER and VEB variants have His170; 10 GES variants (4, 5, 6, 14, 15, 16, 18, 20, 21 and 24) have Ser170, whereas 13 GES variants (1, 3, 7, 7variant, 8, 9, 10, 11, 12, 17, 19, 22 and 23) have Gly170; and both IBC variants have Gly170.
hCTX-M-45 has Tyr216; VEB-8 has Ala216; and all the SHV, TEM, OKP, LEN and CARB variants have Val216.
iCTX-M-106, CTX-M-107, SHV-56, 72, 73 and 84, and all CARB variants have Arg234.
jSHV-107 has Ala235; all TEM variants and nine CARB variants (1, 2, 3, 4, 6, 7, 9, 11 and 12) have Ser235.
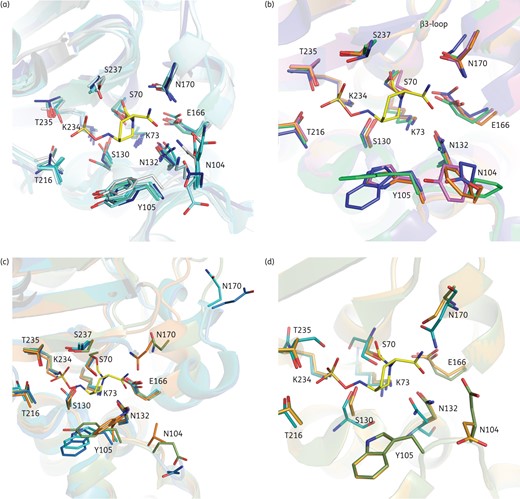
Avibactam binding pocket comparison. CTX-M-15 covalently bound to avibactam (shown as yellow sticks) is overlaid with various enzyme structures. Residue labels represent standard class A numbering. (a) ESBL and non-ESBL class A β-lactamases, where the five TEM enzymes are in various shades of cyan, SHV-1 is in blue and CTX-M-15 bound to avibactam is in grey. (b) Overlay of class A carbapenemases: KPC-2 (green), SME-1 (pink), NMC-A (orange) and GES-5 (blue). (c) Overlays of PER-1 (blue), PER-2 (cyan), SME-1 (magenta), BEL-1 (green) and OXY-1 (orange). (d) Overlay of representative GES enzymes containing Gly (GES-1, green), Ser (GES-5, orange) or Asn (GES-2) at position 170. For clarity, the backbone of the CTX-M-15 protein has been removed from panels (b)–(d).
Among the other smaller groups of class A enzymes, representative structures of most carbapenemases (KPC, SME, NMC-A and some of the GES enzymes) are available. However, a structure for the IMI group of enzymes was not available. Although largely conserved, the only noticeable difference in the avibactam binding pocket amongst these enzymes was the loop closest to the avibactam carboxamide binding pocket, situated just after the KTG motif on strand β3 (marked as β3-loop in Figure 2b). In the case of the KPC, SME and NMC-A enzymes, this loop was slightly larger than that in GES carbapenemases which is more similar to CTX-M-15. However, restoration of ceftazidime MICs in the presence of avibactam for strains carrying KPC enzymes,17,20,23,33 as well as supportive biochemical data,8 suggest that there is minimal impact of this slightly larger loop on the activity of avibactam. Overall, therefore, this structural variation in the class A carbapenemases did not impact the inhibitory activity of avibactam.
The other smaller class A subgroups are the PER, OXY, VEB, LEN, OKP, BEL, CARB and KLUC groups, as well as several other singletons (Table 1). As noted recently, the diversity in these groups is poorly represented in existing X-ray crystallographic data.5 Of these, structures are available only of PER-1, PER-2, OXY-1 and BEL-1. OXY-1 is most similar to CTX-M-15 and, although the Ω-loop region in BEL-1 is slightly larger, the residues that interact with avibactam are highly conserved. The structures of both PER-1 and PER-2 were the most different from the others. The Ω-loop region in these enzymes is not only much larger but has a different fold compared with the conserved features of most class A enzymes.5,34 This Ω-loop change significantly increases the size of avibactam's carboxamide binding pocket, and also removes a polar interaction from the carboxamide group of avibactam (Figure 2c). Similar alterations in the loop regions have been observed in several extended-spectrum class C (ESAC) β-lactamases that result in both increased ceftazidime hydrolysis and decreased avibactam inhibition.13,29,30 Therefore, the in silico prediction would be that the class A PER enzymes would be less efficiently inhibited by avibactam along with the robust ceftazidimase activity that has been observed.35
There are several subgroups with no structural information, including the VEB, OKP, LEN, CARB, IMI and KLUC enzymes, and hence these could not be analysed for structural variability in the avibactam binding pocket. However, given the overall structural conservation of the avibactam binding pocket in the majority of class A members it is highly likely that these groups of class A enzymes will be inhibited by avibactam. Indeed, recent data demonstrate that avibactam can inhibit members of the VEB group.36
Sequence conservation of the avibactam binding pocket amongst class A β-lactamases
During the examination of the avibactam binding pocket in these enzymes, the sequence conservation of key avibactam binding residues across a large number of class A enzymes was also assessed and is depicted in Table 1. The residues that were conserved across all 661 class A β-lactamase sequences to a level of ≥99% were the four catalytically important residues (S70, K73, S130 and E166), as well as N132. Those residues with 85%–95% conservation were Y105, N170 and K234, whereas N104, T216, T235 and S237 were conserved to a level of <50% compared with CTX-M-15.
Among the variations observed in the catalytic residues, SHV-179 with an S70N substitution was expected to result in a non-functional protein. The S130 catalytic residue, which is important for avibactam kinetics, was less conserved.14 Biochemical data have shown that a mutation in this residue significantly impairs the ability of avibactam to acylate and deacylate SHV and KPC enzymes.17,37 The most common variation observed was S130G found in some members of the TEM group (TEM-59, TEM-76, TEM-89—the IRT phenotype38,39) and in one SHV (SHV-10) variant which also contains a premature stop codon resulting in a truncated and inactive protein.40 Whereas an S130G change impacts inhibition by avibactam,37 it is important to note that many of the enzymes that carry this change are also compromised in their ability to hydrolyse cephalosporins, including ceftazidime. Thus, an S130G variant will not reduce the susceptibility to a ceftazidime/avibactam combination. The other variants of S130 are those with an S130C (GES-10) or an S130T (TEM-211 and PER-4) substitution. The S130C change is a conservative substitution of a nucleophilic catalyst and should have little impact on the kinetics of avibactam. However, the S130T variants are more likely to be recalcitrant to avibactam inhibition.
There are only two variants that carry an E166G catalytic residue change (TEM-193 and SHV-122). However, there is evidence that this change may also result in minimal ceftazidime hydrolysis,41 much like the S130G change, and as a result should have no impact on the ceftazidime/avibactam combination.
The remaining residue conserved to a level of >99% was N132, involved in a polar hydrogen bond to the avibactam carboxamide group. All changes to this residue were conservative (e.g. an N130H seen in CTX-M-81). This high level of conservation is not surprising given the importance of N132 for β-lactam catalysis.42 The residues that were 85%–95% conserved were the hydrophobic residue, Y105, and those that contribute polar interactions, N170 and K234. Of these, most variants of Y105 were to a functionally conservative hydrophobic residue (either Y105W in all GES, PER, VEB, OXY and KPC enzymes, a Y105F variation in TEM-213, or a Y105H change observed in three IMI and three SME enzymes). The only exception was a Y105N change in TEM-212. The N170 residue contributes an important polar interaction to the carboxamide group of avibactam. A conservative substitution to N170T (TEM-193), N170S (GES-10) or N170H (all PER and VEB enzymes) should not cause any change in avibactam inhibition. However, the N170G change observed in 13 GES variants could reduce the avibactam binding by eliminating a hydrogen bond to the avibactam carboxamide group (Figure 2d). All variations observed at K234 were to a functionally conservative arginine and are predicted to have no impact on avibactam activity.
The less conserved (<50%) residues were N104, T216, T235 and S237. Most of the variations in these residues were functionally conservative and provide an alternative polar or hydrogen bonding residue for the interaction with avibactam (Table 1 and Table 2). The only non-conservative change observed at position 104 was an N104Y and N104F found in the SME and IMI variants, respectively. However, the carboxamide group of avibactam forms a hydrogen bond with both N104 and N132, of which N132 is highly conserved. Thus the impact of variability in N104 may not be as significant. Additionally, the crystal structure of SME-143 shows that the tyrosine substitution at this position is pointed away from the binding pocket, suggesting that a larger residue would not sterically clash with the ligand upon avibactam binding. The changes in N104 have been shown to cause differences in β-lactam hydrolysis,44,45 especially oxyimino-cephalosporins, and while variation in substrate hydrolysis might still result in differential restoration in combination with specific β-lactam partners, it does not appear to be directly affecting avibactam binding. Further experimental studies would need to be done to confirm this hypothesis.
Amino acid composition at most variable residues required for binding of avibactam among class A β-lactamases
Group . | Number . | Number of enzymes with residues . | ||
---|---|---|---|---|
Position 104 . | Position 105 . | Position 237 . | ||
CTX-M | 131 | Asn (131) | Tyr (131) | Ser (130), Asn (1) |
SHV | 160 | Asp (159), Gly (1) | Tyr (160) | Ala (160) |
TEM | 188 | Glu (137), Lys (51) | Tyr (186), Asn (1), Phe (1) | Ala (178), Thr (9), Gly (1) |
GES | 25 | Glu (18), Lys (7) | Trp (25) | Thr (24), Ala (1) |
PER | 7 | Thr (7) | Trp (7) | Thr (7) |
VEB | 8 | Pro (8) | Trp (8) | Thr (8) |
OXY | 23 | Val (23) | Trp (23) | Ala (17), Gly (5), Thr (1) |
OKP | 34 | Asp (34) | Tyr (34) | Ala (34) |
KPC | 17 | Pro (12), Arg (4), Leu (1) | Trp (17) | Thr (17) |
LEN | 23 | Asp (23) | Tyr (23) | Ala (23) |
BEL | 3 | Glu (3) | Tyr (3) | Ser (3) |
CARB | 12 | Thr (12) | Tyr (12) | Ala (12) |
IMI | 4 | Phe (4) | His (3), Tyr (1) | Ser (4) |
KLUC | 4 | Asn (4) | Tyr (4) | Ser (4) |
SME | 4 | Tyr (4) | His (3), Tyr (1) | Ser (4) |
Othersa | 18 | Asn (15), Ala (1), Lys (1), Glu (1) | Tyr (15), Trp (3) | Ser (15), Thr (3) |
Group . | Number . | Number of enzymes with residues . | ||
---|---|---|---|---|
Position 104 . | Position 105 . | Position 237 . | ||
CTX-M | 131 | Asn (131) | Tyr (131) | Ser (130), Asn (1) |
SHV | 160 | Asp (159), Gly (1) | Tyr (160) | Ala (160) |
TEM | 188 | Glu (137), Lys (51) | Tyr (186), Asn (1), Phe (1) | Ala (178), Thr (9), Gly (1) |
GES | 25 | Glu (18), Lys (7) | Trp (25) | Thr (24), Ala (1) |
PER | 7 | Thr (7) | Trp (7) | Thr (7) |
VEB | 8 | Pro (8) | Trp (8) | Thr (8) |
OXY | 23 | Val (23) | Trp (23) | Ala (17), Gly (5), Thr (1) |
OKP | 34 | Asp (34) | Tyr (34) | Ala (34) |
KPC | 17 | Pro (12), Arg (4), Leu (1) | Trp (17) | Thr (17) |
LEN | 23 | Asp (23) | Tyr (23) | Ala (23) |
BEL | 3 | Glu (3) | Tyr (3) | Ser (3) |
CARB | 12 | Thr (12) | Tyr (12) | Ala (12) |
IMI | 4 | Phe (4) | His (3), Tyr (1) | Ser (4) |
KLUC | 4 | Asn (4) | Tyr (4) | Ser (4) |
SME | 4 | Tyr (4) | His (3), Tyr (1) | Ser (4) |
Othersa | 18 | Asn (15), Ala (1), Lys (1), Glu (1) | Tyr (15), Trp (3) | Ser (15), Thr (3) |
aThe 18 others include 9 FONA variants, 3 RAHN variants, BES-1, IBC-1, IBC-2, SMO-1, SFO-1 and LEMI.
Amino acid composition at most variable residues required for binding of avibactam among class A β-lactamases
Group . | Number . | Number of enzymes with residues . | ||
---|---|---|---|---|
Position 104 . | Position 105 . | Position 237 . | ||
CTX-M | 131 | Asn (131) | Tyr (131) | Ser (130), Asn (1) |
SHV | 160 | Asp (159), Gly (1) | Tyr (160) | Ala (160) |
TEM | 188 | Glu (137), Lys (51) | Tyr (186), Asn (1), Phe (1) | Ala (178), Thr (9), Gly (1) |
GES | 25 | Glu (18), Lys (7) | Trp (25) | Thr (24), Ala (1) |
PER | 7 | Thr (7) | Trp (7) | Thr (7) |
VEB | 8 | Pro (8) | Trp (8) | Thr (8) |
OXY | 23 | Val (23) | Trp (23) | Ala (17), Gly (5), Thr (1) |
OKP | 34 | Asp (34) | Tyr (34) | Ala (34) |
KPC | 17 | Pro (12), Arg (4), Leu (1) | Trp (17) | Thr (17) |
LEN | 23 | Asp (23) | Tyr (23) | Ala (23) |
BEL | 3 | Glu (3) | Tyr (3) | Ser (3) |
CARB | 12 | Thr (12) | Tyr (12) | Ala (12) |
IMI | 4 | Phe (4) | His (3), Tyr (1) | Ser (4) |
KLUC | 4 | Asn (4) | Tyr (4) | Ser (4) |
SME | 4 | Tyr (4) | His (3), Tyr (1) | Ser (4) |
Othersa | 18 | Asn (15), Ala (1), Lys (1), Glu (1) | Tyr (15), Trp (3) | Ser (15), Thr (3) |
Group . | Number . | Number of enzymes with residues . | ||
---|---|---|---|---|
Position 104 . | Position 105 . | Position 237 . | ||
CTX-M | 131 | Asn (131) | Tyr (131) | Ser (130), Asn (1) |
SHV | 160 | Asp (159), Gly (1) | Tyr (160) | Ala (160) |
TEM | 188 | Glu (137), Lys (51) | Tyr (186), Asn (1), Phe (1) | Ala (178), Thr (9), Gly (1) |
GES | 25 | Glu (18), Lys (7) | Trp (25) | Thr (24), Ala (1) |
PER | 7 | Thr (7) | Trp (7) | Thr (7) |
VEB | 8 | Pro (8) | Trp (8) | Thr (8) |
OXY | 23 | Val (23) | Trp (23) | Ala (17), Gly (5), Thr (1) |
OKP | 34 | Asp (34) | Tyr (34) | Ala (34) |
KPC | 17 | Pro (12), Arg (4), Leu (1) | Trp (17) | Thr (17) |
LEN | 23 | Asp (23) | Tyr (23) | Ala (23) |
BEL | 3 | Glu (3) | Tyr (3) | Ser (3) |
CARB | 12 | Thr (12) | Tyr (12) | Ala (12) |
IMI | 4 | Phe (4) | His (3), Tyr (1) | Ser (4) |
KLUC | 4 | Asn (4) | Tyr (4) | Ser (4) |
SME | 4 | Tyr (4) | His (3), Tyr (1) | Ser (4) |
Othersa | 18 | Asn (15), Ala (1), Lys (1), Glu (1) | Tyr (15), Trp (3) | Ser (15), Thr (3) |
aThe 18 others include 9 FONA variants, 3 RAHN variants, BES-1, IBC-1, IBC-2, SMO-1, SFO-1 and LEMI.
Avibactam inhibitory activity in selected GES and PER backgrounds
The structural analyses suggest that the group of class A enzymes with the most significant structural variation in the avibactam binding pocket was the PER group, where the Ω-loop is in a different conformation. In addition, the sequence conservation analyses highlighted several of the GES enzymes that contained an N170G variation. To experimentally evaluate whether these different variations resulted in weaker avibactam activity, representative enzymes were selected that would address both the Ω-loop variation in PER enzymes and the N170G substitution in some GES variants.
The seven PER enzymes identified to date are divided into two sub-groups with ∼85% identity: the PER-1-like, which includes PER-3, PER-4, PER-5 and PER-7, and the PER-2-like, which includes PER-6.46 Both groups contain the unique conformation of the Ω-loop.34,46 The genes encoding PER-2 and PER-3 were cloned as representative enzymes from these subgroups. In addition, PER-4 was also cloned as it also contained the S130T change that carries the additional risk of being recalcitrant to avibactam inhibition due to variation in this catalytic residue.
The N170G variation observed in 13 of the GES enzymes would eliminate the conserved hydrogen bond to the carboxamide group of avibactam. There are also 10 GES variants that contain a conservative substitution of N170S in which case this polar interaction would be maintained. To understand the impact of these changes in this position, isogenic E. coli strains that contained representative enzymes from each of these two subgroups were generated. Specifically, three enzymes that contained an N170G change (GES-1, GES-7 and GES-12) and two variants that contained an N170S change (GES-5 and GES-20) were cloned and the activity profiles are depicted in Table 3.
Enzyme . | MIC (mg/L) . | |||||||||||||||||
---|---|---|---|---|---|---|---|---|---|---|---|---|---|---|---|---|---|---|
Amoxicillina . | Ceftazidimea . | CFX . | CPT . | ATM . | MEM . | |||||||||||||
alone . | AVI (0.5) . | AVI (1) . | AVI (2) . | AVI (4) . | CLA (1) . | CLA (2) . | CLA (4) . | CLA (8) . | alone . | AVI (0.5) . | AVI (1) . | AVI (2) . | AVI (4) . | |||||
Noneb | 8 | 2 | 2 | 2 | 1 | 8 | 8 | 8 | 4 | 0.25 | 0.25 | 0.25 | 0.25 | 0.25 | 8 | 0.125 | 0.125 | 0.03 |
PDC-1c | 64 | 8 | 4 | 2 | 1 | 64 | 64 | 64 | 32 | 1 | 0.25 | 0.25 | 0.25 | 0.25 | 16 | 0.25 | 0.25 | 0.015 |
CTX-M-15 | >1024 | 8 | 4 | 4 | 2 | 16 | 16 | 8 | 8 | 4 | 0.25 | 0.25 | 0.25 | 0.25 | >512 | >512 | 32 | 0.03 |
GES-1 | 128 | 16 | 8 | 4 | 2 | 32 | 16 | 16 | 4 | 4 | 0.5 | 0.5 | 0.25 | 0.25 | 16 | 1 | 0.25 | 0.015 |
GES-7 | 128 | 16 | 8 | 4 | 2 | 64 | 32 | 16 | 4 | 32 | 2 | 1 | 0.5 | 0.5 | 32 | 2 | 2 | 0.03 |
GES-5 | 256 | 16 | 8 | 4 | 2 | 128 | 64 | 32 | 16 | 1 | 0.5 | 0.5 | 0.25 | 0.25 | 8 | 0.5 | 0.25 | 0.06 |
GES-12 | 1024 | 8 | 4 | 4 | 2 | 16 | 8 | 4 | 2 | 64 | 64 | 0.125 | 0.06 | 0.06 | 16 | 2 | 4 | 0.03 |
GES-20 | 256 | 8 | 4 | 4 | 2 | 128 | 64 | 32 | 16 | 0.5 | 0.25 | 0.25 | 0.25 | 0.25 | 4 | 0.5 | 0.125 | 0.06 |
PER-2 | >1024 | 256 | 128 | 128 | 32 | 16 | 16 | 8 | 8 | 512 | 32 | 16 | 4 | 2 | 512 | 16 | 512 | 0.03 |
PER-3 | 512 | 64 | 16 | 8 | 4 | 4 | 4 | 4 | 2 | 128 | 4 | 2 | 1 | 0.5 | 256 | 4 | 64 | 0.015 |
PER-4 | 512 | 512 | 512 | 256 | 256 | 8 | 8 | 4 | 4 | 256 | 256 | 256 | 256 | 256 | 512 | 32 | 64 | 0.03 |
Enzyme . | MIC (mg/L) . | |||||||||||||||||
---|---|---|---|---|---|---|---|---|---|---|---|---|---|---|---|---|---|---|
Amoxicillina . | Ceftazidimea . | CFX . | CPT . | ATM . | MEM . | |||||||||||||
alone . | AVI (0.5) . | AVI (1) . | AVI (2) . | AVI (4) . | CLA (1) . | CLA (2) . | CLA (4) . | CLA (8) . | alone . | AVI (0.5) . | AVI (1) . | AVI (2) . | AVI (4) . | |||||
Noneb | 8 | 2 | 2 | 2 | 1 | 8 | 8 | 8 | 4 | 0.25 | 0.25 | 0.25 | 0.25 | 0.25 | 8 | 0.125 | 0.125 | 0.03 |
PDC-1c | 64 | 8 | 4 | 2 | 1 | 64 | 64 | 64 | 32 | 1 | 0.25 | 0.25 | 0.25 | 0.25 | 16 | 0.25 | 0.25 | 0.015 |
CTX-M-15 | >1024 | 8 | 4 | 4 | 2 | 16 | 16 | 8 | 8 | 4 | 0.25 | 0.25 | 0.25 | 0.25 | >512 | >512 | 32 | 0.03 |
GES-1 | 128 | 16 | 8 | 4 | 2 | 32 | 16 | 16 | 4 | 4 | 0.5 | 0.5 | 0.25 | 0.25 | 16 | 1 | 0.25 | 0.015 |
GES-7 | 128 | 16 | 8 | 4 | 2 | 64 | 32 | 16 | 4 | 32 | 2 | 1 | 0.5 | 0.5 | 32 | 2 | 2 | 0.03 |
GES-5 | 256 | 16 | 8 | 4 | 2 | 128 | 64 | 32 | 16 | 1 | 0.5 | 0.5 | 0.25 | 0.25 | 8 | 0.5 | 0.25 | 0.06 |
GES-12 | 1024 | 8 | 4 | 4 | 2 | 16 | 8 | 4 | 2 | 64 | 64 | 0.125 | 0.06 | 0.06 | 16 | 2 | 4 | 0.03 |
GES-20 | 256 | 8 | 4 | 4 | 2 | 128 | 64 | 32 | 16 | 0.5 | 0.25 | 0.25 | 0.25 | 0.25 | 4 | 0.5 | 0.125 | 0.06 |
PER-2 | >1024 | 256 | 128 | 128 | 32 | 16 | 16 | 8 | 8 | 512 | 32 | 16 | 4 | 2 | 512 | 16 | 512 | 0.03 |
PER-3 | 512 | 64 | 16 | 8 | 4 | 4 | 4 | 4 | 2 | 128 | 4 | 2 | 1 | 0.5 | 256 | 4 | 64 | 0.015 |
PER-4 | 512 | 512 | 512 | 256 | 256 | 8 | 8 | 4 | 4 | 256 | 256 | 256 | 256 | 256 | 512 | 32 | 64 | 0.03 |
AVI, avibactam; CLA, clavulanic acid; CFX, cefuroxime; CPT, ceftaroline; ATM, aztreonam; MEM, meropenem.
aThese drugs were tested alone or in combination with various concentrations, indicated in parentheses in mg/L, of avibactam or clavulanic acid.
bThe pSU19 vector alone was present in this strain.
cThe chromosomal class C β-lactamase was cloned from P. aeruginosa as a control enzyme.
Enzyme . | MIC (mg/L) . | |||||||||||||||||
---|---|---|---|---|---|---|---|---|---|---|---|---|---|---|---|---|---|---|
Amoxicillina . | Ceftazidimea . | CFX . | CPT . | ATM . | MEM . | |||||||||||||
alone . | AVI (0.5) . | AVI (1) . | AVI (2) . | AVI (4) . | CLA (1) . | CLA (2) . | CLA (4) . | CLA (8) . | alone . | AVI (0.5) . | AVI (1) . | AVI (2) . | AVI (4) . | |||||
Noneb | 8 | 2 | 2 | 2 | 1 | 8 | 8 | 8 | 4 | 0.25 | 0.25 | 0.25 | 0.25 | 0.25 | 8 | 0.125 | 0.125 | 0.03 |
PDC-1c | 64 | 8 | 4 | 2 | 1 | 64 | 64 | 64 | 32 | 1 | 0.25 | 0.25 | 0.25 | 0.25 | 16 | 0.25 | 0.25 | 0.015 |
CTX-M-15 | >1024 | 8 | 4 | 4 | 2 | 16 | 16 | 8 | 8 | 4 | 0.25 | 0.25 | 0.25 | 0.25 | >512 | >512 | 32 | 0.03 |
GES-1 | 128 | 16 | 8 | 4 | 2 | 32 | 16 | 16 | 4 | 4 | 0.5 | 0.5 | 0.25 | 0.25 | 16 | 1 | 0.25 | 0.015 |
GES-7 | 128 | 16 | 8 | 4 | 2 | 64 | 32 | 16 | 4 | 32 | 2 | 1 | 0.5 | 0.5 | 32 | 2 | 2 | 0.03 |
GES-5 | 256 | 16 | 8 | 4 | 2 | 128 | 64 | 32 | 16 | 1 | 0.5 | 0.5 | 0.25 | 0.25 | 8 | 0.5 | 0.25 | 0.06 |
GES-12 | 1024 | 8 | 4 | 4 | 2 | 16 | 8 | 4 | 2 | 64 | 64 | 0.125 | 0.06 | 0.06 | 16 | 2 | 4 | 0.03 |
GES-20 | 256 | 8 | 4 | 4 | 2 | 128 | 64 | 32 | 16 | 0.5 | 0.25 | 0.25 | 0.25 | 0.25 | 4 | 0.5 | 0.125 | 0.06 |
PER-2 | >1024 | 256 | 128 | 128 | 32 | 16 | 16 | 8 | 8 | 512 | 32 | 16 | 4 | 2 | 512 | 16 | 512 | 0.03 |
PER-3 | 512 | 64 | 16 | 8 | 4 | 4 | 4 | 4 | 2 | 128 | 4 | 2 | 1 | 0.5 | 256 | 4 | 64 | 0.015 |
PER-4 | 512 | 512 | 512 | 256 | 256 | 8 | 8 | 4 | 4 | 256 | 256 | 256 | 256 | 256 | 512 | 32 | 64 | 0.03 |
Enzyme . | MIC (mg/L) . | |||||||||||||||||
---|---|---|---|---|---|---|---|---|---|---|---|---|---|---|---|---|---|---|
Amoxicillina . | Ceftazidimea . | CFX . | CPT . | ATM . | MEM . | |||||||||||||
alone . | AVI (0.5) . | AVI (1) . | AVI (2) . | AVI (4) . | CLA (1) . | CLA (2) . | CLA (4) . | CLA (8) . | alone . | AVI (0.5) . | AVI (1) . | AVI (2) . | AVI (4) . | |||||
Noneb | 8 | 2 | 2 | 2 | 1 | 8 | 8 | 8 | 4 | 0.25 | 0.25 | 0.25 | 0.25 | 0.25 | 8 | 0.125 | 0.125 | 0.03 |
PDC-1c | 64 | 8 | 4 | 2 | 1 | 64 | 64 | 64 | 32 | 1 | 0.25 | 0.25 | 0.25 | 0.25 | 16 | 0.25 | 0.25 | 0.015 |
CTX-M-15 | >1024 | 8 | 4 | 4 | 2 | 16 | 16 | 8 | 8 | 4 | 0.25 | 0.25 | 0.25 | 0.25 | >512 | >512 | 32 | 0.03 |
GES-1 | 128 | 16 | 8 | 4 | 2 | 32 | 16 | 16 | 4 | 4 | 0.5 | 0.5 | 0.25 | 0.25 | 16 | 1 | 0.25 | 0.015 |
GES-7 | 128 | 16 | 8 | 4 | 2 | 64 | 32 | 16 | 4 | 32 | 2 | 1 | 0.5 | 0.5 | 32 | 2 | 2 | 0.03 |
GES-5 | 256 | 16 | 8 | 4 | 2 | 128 | 64 | 32 | 16 | 1 | 0.5 | 0.5 | 0.25 | 0.25 | 8 | 0.5 | 0.25 | 0.06 |
GES-12 | 1024 | 8 | 4 | 4 | 2 | 16 | 8 | 4 | 2 | 64 | 64 | 0.125 | 0.06 | 0.06 | 16 | 2 | 4 | 0.03 |
GES-20 | 256 | 8 | 4 | 4 | 2 | 128 | 64 | 32 | 16 | 0.5 | 0.25 | 0.25 | 0.25 | 0.25 | 4 | 0.5 | 0.125 | 0.06 |
PER-2 | >1024 | 256 | 128 | 128 | 32 | 16 | 16 | 8 | 8 | 512 | 32 | 16 | 4 | 2 | 512 | 16 | 512 | 0.03 |
PER-3 | 512 | 64 | 16 | 8 | 4 | 4 | 4 | 4 | 2 | 128 | 4 | 2 | 1 | 0.5 | 256 | 4 | 64 | 0.015 |
PER-4 | 512 | 512 | 512 | 256 | 256 | 8 | 8 | 4 | 4 | 256 | 256 | 256 | 256 | 256 | 512 | 32 | 64 | 0.03 |
AVI, avibactam; CLA, clavulanic acid; CFX, cefuroxime; CPT, ceftaroline; ATM, aztreonam; MEM, meropenem.
aThese drugs were tested alone or in combination with various concentrations, indicated in parentheses in mg/L, of avibactam or clavulanic acid.
bThe pSU19 vector alone was present in this strain.
cThe chromosomal class C β-lactamase was cloned from P. aeruginosa as a control enzyme.
Susceptibility testing of the isogenic strains carrying these enzymes showed elevated amoxicillin MIC values from 8 to ≥128-fold compared with the control, demonstrating that all enzymes were expressed. The GES-12 and PER-2 enzymes generated the highest MIC (≥1024 mg/L), comparable to the CTX-M-15 control, whereas strains expressing the remaining GES and PER enzymes tested yielded MIC values between 128 and 512 mg/L, which was between those of strains expressing CTX-M-15 and P. aeruginosa AmpC AZPC-1. As expected of the ESBL phenotype, all three PER enzymes were also able to hydrolyse the cephalosporins including ceftazidime and ceftaroline, as well as the monobactam, aztreonam; however, they were ineffective in hydrolysing meropenem. The GES enzymes that contained the N170S substitution (GES-5 and GES-20) showed weaker cephalosporinase activity when compared with their N170G counterparts (GES-1, GES-7 and GES-12) (Table 3). None of the cloned GES enzymes displayed activity against meropenem, as the N170 residue is important for this activity (Table 3).
Importantly, all of the five GES variants tested, including those containing the N170G change, were effectively inhibited by avibactam when tested in combination with either amoxicillin or ceftazidime, demonstrating that the removal of the hydrogen bond to the carboxamide moiety of avibactam was not deleterious. Although not a clinical combination, amoxicillin was used to enable better comparison of avibactam inhibition as not all enzymes could efficiently hydrolyse ceftazidime. Increasing concentrations of avibactam from 0.5 to 8 mg/L showed a concentration-dependent restoration of the activity of both amoxicillin and ceftazidime in the strains that showed ceftazidime hydrolysis. Whereas the restoration in the presence of clavulanic acid was significantly lower than that observed with avibactam in all GES variants, the enzymes that contained a N170G substitution restored the amoxicillin activity in the presence of avibactam slightly better compared with the strains with enzymes containing N170S variants of GES, in line with previous studies.47 The overall data suggested that avibactam effectively inhibited all of the GES variants and that the N170G substitution did not significantly impact its activity.
In the case of the PER enzymes, both the PER-2 and PER-3 enzymes were equally inhibited by avibactam based on the similar improvement (128-fold) of the MIC of the parent β-lactam partner (ceftazidime). However, the absolute MICs values of amoxicillin or ceftazidime combined with avibactam were lower for the strain expressing PER-3 compared with that of the strain expressing PER-2, probably due to the weaker substrate hydrolysis profile of PER-3 compared with PER-2 (Table 3). Interestingly, PER-4, which contained the S130T change, was completely recalcitrant to the restoration of β-lactam MICs by avibactam. This suggested that, whereas a change in Ω-loop configuration alone did not eliminate the ability of avibactam to restore the activity in these enzymes, the change of a catalytic residue to S130T, perhaps in conjunction with the altered Ω-loop configuration, significantly affected avibactam inhibition.
Conclusions
Avibactam represents a new generation of β-lactamase inhibitors, and is distinguished by an expanded spectrum of inhibition that includes class C and some class D enzymes, as well as class A β-lactamases. The computational and experimental analyses presented here suggest that the vast majority of class A β-lactamases will be well inhibited by avibactam, although a very small number of outliers exist. A key attribute of avibactam is that successful binding utilizes the same residues as those that are required for efficient β-lactam hydrolysis. As such, these residues are highly conserved, resulting, in turn, in a highly conserved avibactam binding pocket across the majority of β-lactamase enzymes. Indeed, this fact underpins the broad spectrum of avibactam inhibition as many of the subtle changes found across the class A enzymes which could impact the inhibitory ability of avibactam are also prone to diminish the hydrolysis of cephalosporins such as ceftazidime. This balance between function and inhibition could delay the emergence of novel β-lactamase variants that confer resistance to ceftazidime/avibactam, which would positively benefit the clinical use of ceftazidime/avibactam.
Funding
This study was funded by AstraZeneca.
Transparency declarations
All authors were employees of AstraZeneca at the time of this study and held stock or stock options in the company.
References
Author notes
Present address: Macrolide Pharmaceuticals, 480 Arsenal Street, Suite 130, Watertown, MA 02472, USA.