-
PDF
- Split View
-
Views
-
Cite
Cite
Adam Heikal, Kiel Hards, Chen-Yi Cheung, Ayana Menorca, Mattie S. M. Timmer, Bridget L. Stocker, Gregory M. Cook, Activation of type II NADH dehydrogenase by quinolinequinones mediates antitubercular cell death, Journal of Antimicrobial Chemotherapy, Volume 71, Issue 10, October 2016, Pages 2840–2847, https://doi.org/10.1093/jac/dkw244
- Share Icon Share
Abstract
Quinolinequinones (QQ) have been shown to inhibit the growth of mycobacterial species, but their mode(s) of action and molecular target(s) remain unknown. To facilitate further development of QQ as antimycobacterial drugs, we investigated the molecular mechanism and target of QQ in mycobacteria.
Cell viability of Mycobacterium tuberculosis and Mycobacterium bovis bacillus Calmette–Guérin was determined in the presence of QQ8c, a representative QQ compound, and isoniazid, a frontline antitubercular drug. The effect of QQ8c on mycobacterial energetics was studied using inverted membrane vesicles. NADH oxidation and formation of reactive oxygen species (ROS) were measured in the presence and absence of KCN. Generation of ROS was measured via oxygen consumption in an oxygen electrode. The effects of QQ8c were compared with the antimycobacterial drug clofazimine in side-by-side experiments.
QQ8c challenge resulted in complete sterilization of cultures with no refractory resistant population observed. QQ8c stimulated NADH oxidation by type II NADH dehydrogenase (NDH-2) and oxygen consumption in inverted membrane vesicles. Large quantities of ROS were produced in the presence of QQ8. Even when oxygen consumption was blocked with KCN, activation of NDH-2 by QQ8c occurred suggesting QQ8c was redox cycling.
QQ8c targets NDH-2 of the mycobacterial respiratory chain leading to activation of NADH oxidation and generating bactericidal levels of ROS in a manner similar to, but more effectively than, the antimycobacterial drug clofazimine. Our results validate respiratory-generated ROS as an avenue for antimycobacterial drug development.
Introduction
Quinones play a vital role in important biological redox processes such as electron transport in oxidative phosphorylation. Quinolinequinones (QQ) display a wide spectrum of biological properties. While they are perhaps best known for their anticancer1–3 and anti-inflammatory activities,4 modification of the QQ scaffold can also lead to derivatives that exhibit minimal toxicity and show promise in the treatment of TB,5 though the antimycobacterial mode of action remains unknown. They are readily reduced and some derivatives interact, either as inhibitors or substrates, with the human quinone reductase enzyme NAP(P)H:quinone oxidoreductase 1 (NQO1).6–8 NQO1 catalyses the one and two-electron reduction of the anticancer quinone bio-reductive drug mitomycin C, leading to its cytotoxic antitumour activity.9 Similarly, NQO1-mediated redox activation of the quinone-containing compound β-lapachone leads to generation of cytotoxic levels of reactive oxygen species (ROS) in cancer cells.10 Streptonigrin, a naturally occurring antibiotic 5,8-quinolinequinone from Streptomyces flocculus,11,12 has been shown to be selectively toxic in cancer cell lines overexpressing NQO1.13
We previously prepared a library of 6,7-substituted-5,8-quinolinequinones and assessed these compounds for their ability to inhibit the growth of Mycobacterium bovis bacillus Calmette–Guérin (BCG), as well as their antitumour and anti-inflammatory activities.14 From this library, a number of promising antitubercular drug leads containing amine and halogen functionality were identified, exhibiting tuberculostatic activity in the range 3–12.5 mg/L (∼10–45 μM).14 This initial investigation gave no insight into the mode of action of the compounds nor did it determine whether they were ultimately bactericidal. The low micromolar MIC prompted us to identify the molecular target and elucidate the mode of action, not only to rule out non-specific toxicity, but also to shed light on a possible new pathway for cidality in TB complex organisms. Such studies would allow for a more tailored approach towards the synthesis of a second generation library of 6,7-substituted-5,8-quinolinequinones as potential antimycobacterial drugs.
The respiratory chain has recently been identified as a new and potentially rich source of antimycobacterial drug targets. Compounds that target the F1F0-ATP synthase (bedaquiline),15–17 cytochrome bc1 complex (Q203)18 and menaquinone biosynthesis (SQ109),19 and are capable of uncoupling the electron transport chain (bedaquiline, SQ109),20,21 have all shown promise as new antitubercular candidates. Naturally, attention has turned to the remaining elements of the mycobacterial oxidative phosphorylation machinery, in the hope of identifying and advancing further drug targets. The target of the antimycobacterial drug clofazimine was identified as the respiratory type II NADH dehydrogenase (NDH-2) where redox cycling produces bactericidal ROS.22 NDH-2 catalyses the transfer of electrons from NADH to menaquinone and is the primary entry point of electrons into the mycobacterial respiratory chain.23,24 By virtue of its essentiality for growth in mycobacteria, and absence from mammalian mitochondria, it is an excellent candidate target for antimycobacterial drugs.23,25,26 Quinone analogues have been identified by phenotypic high-throughput screening for inhibitors of Mycobacterium tuberculosis H37Rv and whilst their potential reactivity has traditionally been treated with caution in drug discovery, it has been suggested that they may possess the ability to interrupt the mycobacterial electron chain.27,28 The interactions of quinone-containing drugs with NQO1, in particular their ability to produce ROS,10 led us to investigate whether NDH-2 was the site of QQ activity in mycobacteria.
Materials and methods
Bacterial strains, media and growth conditions
Mycobacterium smegmatis mc215529 and the expression strain M. smegmatis mc2451730 were maintained on LB agar plates supplemented with 0.05% (w/v) Tween 80 (Sigma–Aldrich) (LBT) or grown in LBT broth at 37°C with agitation (200 rpm). Mycobacterium bovis BCG Pasteur was grown in Middlebrook 7H9 broth supplemented with ADS [5% (w/v) BSA, 2% (w/v) glucose and 1% (w/v) NaCl] and 0.05% (w/v) Tween 80. M. tuberculosis ΔRD1 ΔpanCD31 was grown in Middlebrook 7H9 broth supplemented with OADC [0.05% (w/v) oleic acid, 5% (w/v) BSA, 0.85% (w/v) NaCl 2% glucose and 4 μg/mL catalase (CAT)] and 50 μg/mL pantothenate and leucine.
Cloning and expression of NDH-2 from M. tuberculosis H37Rv
The expression vector pYUB1049 was used to construct expression plasmid pYUB-NdhHisC bearing C-terminally 6-histidine tagged NDH-2 from M. tuberculosis H37Rv. The ndh (Rv1854c) gene sequence from M. tuberculosis H37Rv was amplified using primers MtbNdhHisCFw (5′ AAATTTCATATGTGAGAAGGAGATATAATGAGTCCCCAGCAAGAACCCACA 3′) and MtbNdhHisCRv (5′ AAATTTGGATCCGAGGCCACCTTAGCGCTTGCCGC 3′). The resulting PCR products were restriction digested with NdeI and BamHI and cloned into pYUB1049,32 digested with NdeI and BamHI. The resulting ligation product was transformed into Escherichia coli DH10B and transformants were selected on LB containing hygromycin B (200 mg/L). M. smegmatis mc24517 was transformed with pYUB-NdhHisC and grown in LBT with the addition of kanamycin (20 mg/L) and hygromycin B (50 mg/L) at 37°C with agitation (200 rpm).
Synthesis of 7-chloro-6-(2-chloro-ethylamino)-5,8-quinolinequinone (QQ8c)
QQ8c (Figure S1, available as Supplementary data at JAC Online) was synthesized as described by Mulchin et al.14
Determination of cell viability
MIC was determined by the visual presence/absence of a cell pellet after 7 days in U-bottomed 96-well microtitre plates inoculated with M. bovis BCG Pasteur or M. tuberculosis ΔRD1 ΔpanCD (OD600 0.005) in the presence of QQ8c (0.1–138.8 mg/L) and incubated at 37°C with agitation (200 rpm). Resazurin (0.03% w/v) was then added and plates incubated for a further 7 days. Viable cells reduce resazurin (blue) to resofurin (pink) indicating the MBC.33 To determine the kinetics of killing, M. bovis BCG Pasteur was grown in T25 tissue culture flasks, with no shaking, to an OD600 of ∼0.75, then diluted 1/20 and treated with isoniazid (1 mg/L, 10× MIC M. bovis BCG Pasteur,34 20× MIC M tuberculosis H37Rv35) or QQ8c (2.7 and 13.6 mg/L). Cell number was followed over 28 days and determined by an adapted most probable number (MPN) method.36,37 Cells were prepared in 10-fold serial dilutions, in triplicate, in U-bottomed 96-well microtitre plates and incubated at 37°C for 7 days. MPN was determined by visual presence/absence of a cell pellet according to published tables37 and adjusted for dilution.
Preparation of inverted membrane vesicles (IMVs) from mycobacteria
IMVs were prepared from either M. smegmatis mc24517 or M. bovis BCG as previously described.38 Briefly, following harvesting via centrifugation, cells were homogenized six times on ice, before being passed through a French pressure cell six times at 20 000 psi. Unbroken cells were removed by high-speed centrifugation (10 000 g, 10 min, 4°C) before the membrane fraction was isolated from the clarified cell lysate by ultracentrifugation (100 000 g, 1 h, 4°C). Membranes were resuspended using a 22G hypodermic needle to form IMVs and stored at −80°C.
Measurement of NADH oxidation and QQ8c reduction in IMVs
Aerobic NADH oxidation was followed at 340 nm at 37°C as previously described,39 with the following exceptions. Reaction mixtures contained 50 mM Tris-HCl (pH 7.5), 5 mM MgCl2 and 50 μg/mL IMVs. Clofazimine was added to reaction mixtures from a concentrated stock solution dissolved in DMSO. Owing to its low aqueous solubility we report clofazimine as mg/L rather than potentially inaccurate molar concentrations. Reactions were initiated by the addition of 200 μM NADH and were performed in triplicate. Rates of NADH oxidation were expressed as μmol.NADH min−1 (mg protein)−1 where protein refers to total membrane protein. Anaerobic NADH oxidation was performed with the following additions, which remove dissolved oxygen from the assay buffer. Buffers, excluding membranes or NADH, were boiled for 5 min, sealed in a serum vial with a butyl rubber stopper and then cooled under a flow of argon. All subsequent steps were performed in a Starna Anaerobic Cuvette under a continuous flow of argon. Glucose oxidase (60 μg/mL), CAT (4 μg/mL) and glucose (40 mM) were added to 2 mL of buffer to enzymatically remove oxygen as previously described.40 Prior to each experiment, these concentrations were confirmed to remove all detectable oxygen in a Clark-type oxygen electrode in <1 min (data not shown). Membranes were subsequently added and reaction initiated. Reduction of QQ8c was followed in the above experiments simultaneously by following absorbance at 473 nm, using the extinction coefficient ε473 = 3.256 mM−1 cm−1. The extinction coefficient for QQ8c was determined from the absorbance maxima of air oxidized and sodium dithionite reduced compound (Supplementary Data).
Measurement of oxygen consumption rates
Oxygen consumption was measured using a Rank Brothers Clark-type oxygen electrode at 37°C as previously described41 with the exception that 1 mM NADH was used to stimulate respiration by IMVs. To determine the extent of oxygen consumption that results in superoxide production, 25 U superoxide dismutase (SOD) and 900 U CAT was added where indicated. The combined reactions result in a 75% recovery of superoxide as molecular oxygen, translating into an apparent inhibition of oxygen consumption (75% maximal inhibition in the case where 100% of oxygen consumption is linked to superoxide production).
Proton translocation (ΔpH) dependent fluorescence quenching
Quenching of Acridine Orange (AO) via proton translocation in IMVs was measured as previously described.20 Briefly IMVs (1 mg total membrane protein) were incubated with 5 μM AO in 10 mM HEPES (pH 7.5), 100 mM KCl, 5 mM MgCl2 for 1 min to establish a baseline, before quenching was initiated by the addition of 1 mM NADH; 13.6 mg/L QQ8c or 2.5 μM CCCP was added after 3 min, and 2 μL DMSO was used as a vehicle control.
Results
QQ8c is bactericidal against M. bovis BCG and prevents emergence of persister cells
QQ8c (Supplementary Data) was shown previously to be tuberculostatic with an MIC of 6.3–12.5 mg/L (23–46 μM).14 In this study, we determined the MIC to be 2.2 mg/L (Supplementary Data). QQ8c was bactericidal against M. tuberculosis and M. bovis BCG and the MIC and MBC (both 2.2 mg/L) were comparable for both species (Supplementary Data). The kinetics of killing were broadly similar regardless of whether the concentration of QQ8c was ∼1× MIC (2.7 mg/L) or 5× MIC (13.6 mg/L) (Figure 1), suggesting the bactericidal effect was time-dependent rather than concentration-dependent. The initial reduction in cell numbers (viability) was, however, ∼100-fold greater for 13.6 mg/L QQ8 (Figure 1). Over 28 days both concentrations of QQ8c reduced M. bovis BCG cell numbers below the limit of detection (MPN <3, e.g. no growth detected in a 10−1 dilution) indicating sterilizing bactericidal activity (Figure 1). The addition of 10× MIC34 isoniazid to M. bovis BCG (20× MIC reported for M. tuberculosis H37Rv35) caused a 10 000-fold reduction in cell numbers over the first 3 days (Figure 1). Consistent with observations made by others,35 isoniazid-resistant mutants appeared by day 7 and by day 28, cell numbers as determined by MPN, were identical to the control.35 By contrast, no resistance was observed in cells treated with either 2.7 or 13.6 mg/L QQ8c, resulting in complete sterilization with no detectable refractory persister cell populations (Figure 1).

Effect of QQ8c and isoniazid on M. bovis BCG Pasteur cell viability. M. bovis was grown in 7H9 supplemented with ADS either untreated (control) or treated with 1 mg/L (10× MIC) isoniazid (INH) or QQ8c (2.7 or 13.6 mg/L). Cell viability was expressed as log10 most probable number (MPN). Error bars represent the standard deviation from three independent technical replicates.
NADH oxidation is stimulated by QQ8c in M. bovis BCG IMVs
Owing to its quinone substructure (Supplementary Data), we investigated the effect of QQ8c on NADH oxidation and its concomitant reduction in M. bovis BCG IMVs, under both aerobic and anaerobic assay conditions. The basal (e.g. using endogenous menaquinone present in IMVs) rate of NADH oxidation by IMVs was 0.198 ± 0.006 μmol.NADH min−1 (mg protein)−1 under aerobic conditions (Figure 2a). Incubation of IMVs with increasing concentrations of QQ8c stimulated NADH oxidation with saturation kinetics observed (Figure 2a). Concomitant reduction of QQ8c by IMVs was measured under these conditions though due, presumably, to the speed of non-enzymatic re-oxidation, very little (<5%) was detected (Figure 2a). Basal NADH oxidation was greatly reduced under anaerobic conditions (Figure 2b). QQ8c also stimulated NADH oxidation under anaerobic conditions and high rates of QQ8 reduction were detected (Figure 2b). There was a visible, red to yellow colour change observed at the completion of anaerobic reactions. This correlates with the observed spectrophotometric absorbance change at 473 nm, confirming that QQ8c was indeed being reduced following oxidation of NADH in M. bovis BCG IMVs in the absence of molecular oxygen. No oxidation of NADH was observed in the absence of IMVs under either aerobic or anaerobic assay conditions.
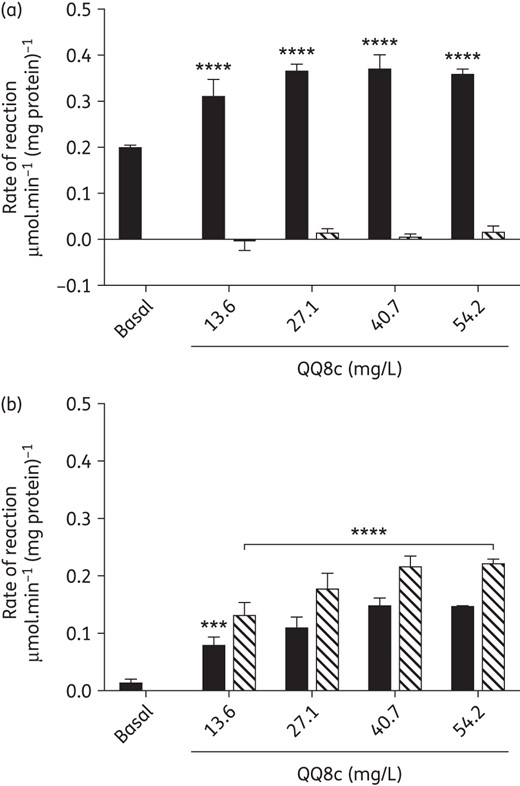
QQ8c stimulates NADH oxidation by serving as an intermediary reductant to oxygen. (a) Stimulation of NADH oxidation (black bars) by QQ8c in aerobic conditions. (b) Stimulation of NADH oxidation by QQ8c in anaerobic conditions. Reduction of QQ8c (hashed bars) was followed simultaneously with NADH oxidation. Basal refers to the NADH oxidation rate of the untreated control. All experiments were performed in inverted membrane vesicles prepared from M. bovis BCG. ***P ≤ 0.001 and ****P < 0.0001 versus basal (two-way ANOVA, Dunnett's multiple comparison test). All columns underneath the horizontal bar were ****P < 0.0001. Error bars represent the standard deviation from three independent technical replicates.
QQ8c-stimulated NADH oxidation in IMVs is insensitive to cyanide inhibition and uncoupled from respiration
The stimulation of NADH oxidation and the ability of QQ8c to accept electrons implicated the electron transport chain as the bactericidal site of action. To investigate this possibility, the potential of QQ8c to interact with respiratory complexes was assessed. In M. bovis BCG IMVs, basal NADH oxidation was severely ablated, <15% untreated activity, by addition of KCN (20 mM), indicating almost complete inhibition of the terminal oxidases, e.g. aa3-type cytochrome c oxidase (Figure 3). However, in the presence 20 mM KCN, QQ8c-stimulated IMVs NADH oxidation was >150% that of untreated IMV activity (Figure 3). Clofazimine is an antimycobacterial drug previously reported to be capable of restoring NADH oxidation to KCN-treated M. smegmatis membranes.22 The addition of 4.7 mg/L clofazimine restored NADH oxidation to KCN-treated IMVs to ∼60% that of untreated IMVs (Figure 3). The degree of restoration of NADH oxidation following KCN treatment by both QQ8c (P < 0.0001) and clofazimine (P < 0.01) was determined to be statistically significant by one-way ANOVA (Tukey's multiple comparison test). These data suggested that, as in the case of clofazimine, QQ8c restored NADH oxidation via a redox-cycling mechanism and may be capable of generating large, bactericidal amounts of ROS.
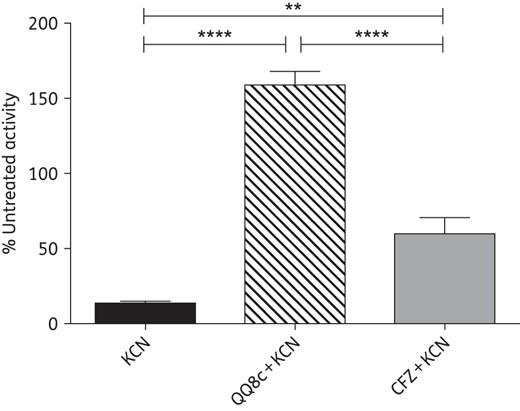
Restoration of NADH oxidation in inverted membrane vesicles (IMVs) by either QQ8c or clofazimine (CFZ) in the presence of KCN. The ability of 13.6 mg/L QQ8c (hashed column) and 4.7 mg/L CFZ (grey column) to restore NADH oxidation following treatment with 20 mM KCN was measured in M. bovis BCG IMVs. Activities are expressed as a percentage relative to untreated IMVs. KCN (black column) refers to NADH oxidation in the presence of 20 mM KCN and absence of QQ8c and CFZ. ****P < 0.0001 QQ8c + KCN versus KCN and QQ8c + KCN versus CFZ + KCN, **P < 0.01 CFZ + KCN versus KCN (one-way ANOVA, Tukey's multiple comparison test). Error bars represent the standard deviation from three independent technical replicates.
NADH-QQ8c oxidoreduction produces ROS
If the ability of QQ8c to restore NADH oxidation in KCN-inhibited IMVs was similar to clofazimine,22 i.e. linked to redox cycling and ROS production, then a combination of the ROS-scavenging enzymes SOD and CAT would reduce the apparent rate of oxygen consumption. Accordingly, we measured oxygen consumption by IMVs during basal, and QQ8c- and clofazimine-stimulated NADH oxidation (Figure 4). In the presence of 1 mM NADH as the electron donor for respiration, the basal rate of oxygen consumption was 148 ± 8 nmol oxygen.min−1 (mg protein)−1 (Figure 4). Oxygen consumption was increased significantly on addition of 13.6 mg/L QQ8c and inclusion of a mixture of SOD and CAT (SOD + CAT) abolished this activation, suggesting that a large portion of the oxygen consumption was due to the generation of ROS (Figure 4). Two-way ANOVA revealed differences between rates of oxygen consumption in the presence and absence of QQ8c and SOD + CAT were statistically significant (P < 0.01; Sidak's multiple comparison test). By contrast, 23.7 mg/L clofazimine did not elicit a significant increase in oxygen consumption and consequently the rates were both significantly lower than in the presence of QQ8c (P < 0.0001) and insensitive to SOD + CAT, suggesting that under these conditions clofazimine does not produce significant levels of ROS (Figure 4).
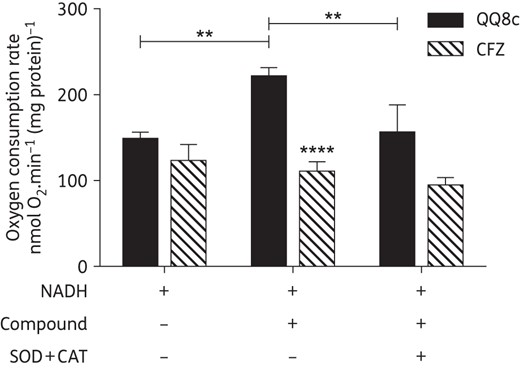
QQ8c stimulates oxygen consumption by promoting the generation of ROS. The degree of stimulation of oxygen consumption from NADH-induced respiration by QQ8c or clofazimine (CFZ) was measured in M. bovis BCG inverted membrane vesicles (IMVs). In a separate experiment, further supplementation with superoxide dismutase and catalase (SOD + CAT) was performed to assess the production of ROS. Plus and minus symbols indicate the presence and absence, respectively, of NADH (1 mM), QQ8c (13.6 mg/L), CFZ (23.7 mg/L) or SOD + CAT in each independent experiment. **P < 0.01 QQ8c versus untreated and QQ8c in the presence versus QQ8c in absence of SOD + CAT, ****P < 0.0001 QQ8c in the absence of SOD + CAT versus CFZ in the absence of SOD + CAT (two-way ANOVA, Sidak's multiple comparison test). Error bars represent the standard deviation from three independent technical replicates.
QQ8c is not an uncoupler of respiration in M. bovis BCG IMVs
To determine whether QQ8c was acting as an uncoupler of respiration, due to its ability to activate NADH-dependent oxygen consumption, we used quenching of AO fluorescence to assay the translocation of protons into the lumen of IMVs (acidification) (Supplementary Data). On addition of 1 mM NADH to IMVs we observed quenching of the fluorescent dye AO as a result of IMVs-lumen proton accumulation, i.e. generation of a transmembrane pH gradient (ΔpH) (Supplementary Data). This was easily reversed on addition of the uncoupling molecule CCCP (2.5 μM) indicating a collapse of the ΔpH (Supplementary Data). Neither the DMSO control nor 13.6 mg/L QQ8c caused reversal of the NADH-induced proton accumulation and associated AO fluorescence quenching (Supplementary Data). This indicated that stimulation of NADH oxidation and oxygen consumption was not the result of QQ8c acting as an uncoupling molecule.
Clofazimine is less effective than QQ8c in restoring oxygen consumption
The lack of detectable ROS production on the addition of clofazimine to IMVs, in spite of the restoration of NADH oxidation in the presence of KCN, appeared to contradict previous observations22 (Figure 3). In contrast to Yano et al.22 who employed an enzyme-linked ROS assay, we chose to measure oxygen consumption directly. This technique, whilst possibly less sensitive than an enzyme-linked assay, allowed for measurement of oxygen consumption in KCN-inhibited IMVs. We observed KCN-sensitive stimulation of oxygen consumption in the presence 0.5 mM NADH, which could then be restored on addition of 4.7 mg/L clofazimine (Figure 5). No further increase in respiration was noted with the addition of 23.7 mg/L clofazimine (Figure 5). By contrast, 13.6 mg/L QQ8c was able to activate respiration to a greater extent, demonstrating greater effectiveness in redox cycling. The difference in the abilities of QQ8c and clofazimine to generate ROS through redox cycling may explain why we were unable to detect increased or SOD + CAT-sensitive oxygen consumption in clofazimine-treated IMVs (Figure 4).

QQ8c restores KCN-inhibited oxygen consumption to inverted membrane vesicles (IMVs). Traces indicate oxygen consumption (nmol O2) in M. bovis BCG IMVs. NADH (0.5 mM), KCN (20 mM) and either QQ8c (13.6 mg/L) or clofazimine (CFZ, 4.7 or 23.7 mg/L) were added where indicated (arrows). The slope indicates the rate (nmol O2/s) at which oxygen was consumed on addition of each compound. The identity and concentration of the compound added is to the right of the relevant trace. Traces are representative of three independent experiments.
NDH-2 is a target of QQ8c and the site of bactericidal ROS production
The canonical type I NADH dehydrogenase (NDH-1) contributes as little as 5% of the total NADH dehydrogenase activity in the mycobacterial electron transport chain,42 leaving NDH-2 as the overwhelmingly dominant NADH:quinone oxidoreductase. To confirm NDH-2 was the site of QQ8c action, we compared the effect of QQ8c on NADH oxidation in IMVs with and without elevated NDH-2 levels. To generate IMVs with high levels of NDH2 we overexpressed NDH-2 from M. tuberculosis H37Rv in M. smegmatis mc24715 (Supplementary Data). IMVs containing native levels of NDH-2 were obtained from M. smegmatis mc2155. As expected from the saturation kinetics observed (Figure 2a), 2.7 mg/L QQ8c elicited only a minor stimulation of NADH oxidation in M. smegmatis mc2155 IMVs above the basal rate (Figure 6). By contrast, 2.7 mg/L QQ8c elicited a large (>4-fold) stimulation in the rate of NADH oxidation (Figure 6). Taken together, these data suggest that QQ8c does indeed act at NDH-2 and has the capacity to activate the enzyme as an alternative substrate.
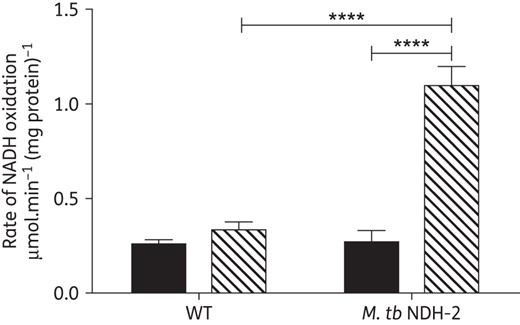
Mycobacterial type II NADH dehydrogenase is the target of QQ8c. Stimulation of NADH oxidation in inverted membrane vesicles (IMVs) prepared from M. smegmatis Mc2155 (WT) and M. smegmatis Mc24517 overexpressing M. tuberculosis H37Rv type II NADH dehydrogenase (M. tb NDH-2). NADH oxidation measured in untreated IMVs (black bars) or 2.7 mg/L QQ8c-treated IMVs (hashed bars). ****P < 0.0001 M. tb NDH-2 basal versus M. tb NDH-2 QQ8c-treated and WT QQ8c-treated versus M. tb NDH-2 QQ8c treated (two-way ANOVA, Tukey's multiple comparison test). Error bars represent the standard deviation from three independent technical replicates.
Discussion
To combat rising antimicrobial resistance, including the emergence of MDR-TB, there is an urgent need for not only new antibiotic molecules but also new antibiotic pathways.43,44 Several new, antitubercular drug candidates purport to target respiration and oxidative phosphorylation in M. tuberculosis.18,19 It has become increasingly clear, however, that the antitubercular mechanisms are not well understood and these drugs may, in fact, have multiple modes of action.21 Bedaquiline, the first new TB therapeutic in decades, binds to the c ring of the F1F0-ATP synthase with nanomolar potency15,45,46 but has recently been shown to act as an electroneutral uncoupler of respiration-driven ATP synthesis.20 The promising drug candidate, SQ109, entered Phase I clinical trials before a mode of action disruption of the mycobacterial cell wall assembly47 was put forward. Subsequently, multiple SQ109 targets have been proposed, including menaquinone biosynthesis and electron transport.21 Moreover, SQ109 has been shown to act also as an uncoupler of respiration capable of collapsing the pH gradient and Δψ.21,48 Contrary to expectations, the discovery that antitubercular antibiotics act as uncouplers of respiration is not limited to new drug candidates.48 Several FDA-approved drugs, including clofazimine, display multi-target activity, acting both on enzyme targets and as uncouplers.48
In this study, we set out to determine the mode of action of QQ against M. tuberculosis and M. bovis BCG and in doing so showed the cidal activity was most likely due to NDH-2-mediated generation of ROS. A striking observation was the ability of QQ8c to prevent the emergence of a persister cell population. Antibiotic-generated ROS have recently been shown to play a significant role in the elimination of persister populations undergoing antibiotic treatment.49 Consistent with other studies35 we observed that even in the presence of 10× MIC isoniazid, a frontline TB drug, resistance was quickly established and cell numbers returned to match that of the untreated control. By contrast, both concentrations of QQ8c tested achieved time-dependent complete sterilization with no refractory population detected.
It has long been known that QQ-type molecules are readily reduced, and the exploitation of NQO1 enzyme function to generate damaging levels of ROS from quinone-containing molecules or activate bio-reductive drugs has been explored for anticancer therapy.9,10 NDH-2 has previously been shown to be capable of producing bactericidal levels of ROS in the presence of the antimycobacterial drug clofazimine.22 NDH-2 is a non-proton translocating, membrane-bound monotopic enzyme that is the primary entry point from which electrons from central metabolism enter the electron transport chain. NDH-2 was proposed as an antitubercular drug target following the discovery that its inhibition by phenothiazines was bactericidal;50,51 however, clinically effective concentrations of phenothiazines cannot be achieved.52 Despite its undoubted appeal as a drug target, potent and specific NDH-2 inhibitors have remained elusive despite several compounds displaying inhibitory and antimycobacterial effects.51,53 Inspection of the quinone-binding tunnel, located in the lipid bilayer of the cell membrane, reveals minimal binding between amino acid side chains and quinone.24 This may, in part, explain why no potent, tight-binding inhibitors of NDH-2 have been developed in the decade since it was first proposed as a drug target.
We therefore investigated the possibility that QQ8c was, in fact, an activator of NDH-2's NADH dehydrogenase activity. QQ8c-stimulated oxidation of NADH in IMVs and under anaerobic assay conditions was itself reduced. This hinted at the possibility that redox cycling was occurring under aerobic conditions. Typically, high-throughput screening has sought to identify antimicrobials by screening large compound libraries to discover inhibitors, either of enzyme function or cell growth/division.54,55 By contrast, activation or exploitation of enzyme function is almost never considered as an alternative pathway for antibiotic action. Acyldepsipeptides, a new class of antibiotics, are an exception to this rule, and act via dysregulation of the ClpP proteolytic machinery,56 removing the safeguard that protects cytoplasmic proteins from random degradation, triggering cell death.56
Strict coupling of the electron transport chain is vital for growth and survival of mycobacteria,20 so any perturbation, or dysregulation, of oxidative phosphorylation homeostasis has the potential to be a potent antimycobacterial drug.57 In this investigation, we observed that QQ8c stimulated/restored both NADH oxidation and oxygen consumption in M. bovis BCG IMVs, even in the presence of the terminal oxidase inhibitor KCN. The increased oxidation of NADH and oxygen consumption was initially suggestive of a collapse of the transmembrane pH gradient (ΔpH), similar to that caused by the action of a protonophore, e.g. CCCP, which has been shown to lead to mycobacterial cell death.58,59 However, we demonstrated that, in contrast to both bedaquiline20,48 and clofazimine,48 QQ8c did not dissipate the ΔpH in IMVs and therefore did not act as an uncoupler. In our study, consistent with Yano et al.,22 clofazimine was able to restore both NADH oxidation and oxygen consumption to KCN-inhibited IMVs, but not the extent of QQ8c. QQ8c-stimulated oxygen consumption was shown to be sensitive to ROS-detoxifying enzymes, indicating that under aerobic conditions, large quantities of ROS were produced. In contrast with previous observations that clofazimine induced ROS22 we did not detect any ROS-detoxifying enzyme sensitive oxygen consumption in the presence of clofazimine. It is likely that the levels of ROS produced by clofazimine were below the limit of detection for our apparatus. This is consistent with our observation that clofazimine stimulation of IMV's NADH oxidation was lower as compared with QQ8c. Furthermore, when we measured the rate of oxygen consumption restored to KCN-inhibited IMVs by both QQ8c and clofazimine, QQ8c was able to not only restore oxygen consumption completely, but increased the rate far above that achieved with the same concentration of clofazimine.
Although NDH-2 is the site of clofazimine-mediated ROS production, following restoration of NADH oxidation, no stimulation of NDH-2 activity above basal was observed.22 In this investigation, NADH oxidation in IMVs overexpressing M. tuberculosis H37Rv NDH-2 was significantly higher in the presence of QQ8c, indicating a role for activation of this enzyme in the bactericidal mode of action. The structural similarity of QQ8c to menaquinone, the physiological substrate of other mycobacterial respiratory enzymes such as succinate dehydrogenase, raises the possibility that other QQ8c targets exist within the electron transport chain. With no direct evidence to the contrary, and the recently demonstrated multi-target nature of other antitubercular drugs,48 this possibility must be acknowledged. However, the ability of QQ8c to stimulate NADH oxidation in KCN-treated IMVs despite its inability to collapse the proton motive force, strongly implicates NDH-2 as the primary target. We therefore propose that QQ8c stimulates the catalytic activity NDH-2, causing uncontrolled, dysregulated, oxidation of NADH. The effect of this is likely 2-fold, producing damaging levels of ROS via NDH-2-mediated redox cycling and starving the electron transport chain of the electron flow vital for respiratory ATP synthesis. Unlike bedaquiline, QQ8c does not uncouple the electron transport chain.20 The activation of NDH-2 by QQ8c demonstrates a pathway to produce a new generation of anti-TB drugs by exploiting the obligate oxidoreductase activity of respiratory chain components to exert an antitubercular effect.
Funding
This work was funded by the Maurice Wilkins Centre for Molecular Biodiscovery and the Health Research Council of New Zealand. K. H. was supported by the Otago Medical Research Foundation and a University of Otago Doctoral Scholarship.
Transparency declarations
None to declare.
Acknowledgements
The authors thank Miss Zhi Shean Tee for technical assistance and Professor William R. Jacobs Jr for kindly providing M. tuberculosis ΔRD1 ΔpanCD.
References
Author notes
These authors contributed equally to this work.
- oxygen
- oxidation
- clofazimine
- antitubercular agents
- bacillus
- cell death
- cell survival
- isoniazid
- tissue membrane
- mycobacterium
- mycobacterium bovis
- mycobacterium tuberculosis
- nicotinamide adenine dinucleotide (nad)
- cytochrome c reductase
- oxidation-reduction
- oxygen consumption
- reactive oxygen species
- tuberculosis
- vesicle
- antimycobacterial agents
- molecular target
- respiratory chain