-
PDF
- Split View
-
Views
-
Cite
Cite
Caroline M. Pule, Samantha L. Sampson, Robin M. Warren, Philippa A. Black, Paul D. van Helden, Tommie C. Victor, Gail E. Louw, Efflux pump inhibitors: targeting mycobacterial efflux systems to enhance TB therapy, Journal of Antimicrobial Chemotherapy, Volume 71, Issue 1, January 2016, Pages 17–26, https://doi.org/10.1093/jac/dkv316
- Share Icon Share
Abstract
The emergence of drug resistance continues to plague TB control, with a global increase in the prevalence of MDR-TB. This acts as a gateway to XDR-TB and thus emphasizes the urgency for drug development and optimal treatment options. Bedaquiline is the first new anti-TB drug approved by the FDA in 40 years and has been shown to be an effective treatment option for MDR Mycobacterium tuberculosis infection. Bedaquiline has also recently been included in clinical trials for new regimens with the aim of improving and shortening treatment periods. Alarmingly, efflux-mediated bedaquiline resistance, as well as efflux-mediated cross-resistance to clofazimine, has been identified in treatment failures. This mechanism of resistance results in efflux of a variety of anti-TB drugs from the bacterial cell, thereby decreasing the intracellular drug concentration. In doing so, the bacillus is able to render the antibiotic treatment ineffective. Recent studies have explored strategies to reverse the resistance phenotype conferred by efflux pump activation. It was observed that the addition of efflux pump inhibitors partially restored drug susceptibility in vitro and in vivo. This has significant clinical implications, especially in MDR-TB management where treatment options are extremely limited. This review aims to highlight the current efflux pump inhibitors effective against M. tuberculosis, the effect of efflux pump inhibitors on mycobacterial growth and the clinical promise of treatment with efflux pump inhibitors and standard anti-TB therapy.
Introduction
Perhaps the most clinically significant role of efflux pumps in Mycobacterium tuberculosis drug resistance is the involvement of efflux activity in bedaquiline resistance.1–3 Bedaquiline is the first new anti-TB drug approved by the FDA in 40 years and has been shown to be an effective treatment option for MDR M. tuberculosis infection.2,4–7 Bedaquiline has also recently been included in clinical trials for new regimens with the aim of improving and shortening treatment periods.8 Efflux-mediated resistance to bedaquiline, as well as efflux-mediated cross-resistance to clofazimine, another anti-TB drug of interest, is therefore alarming. However, recent studies have highlighted the ability of the efflux pump inhibitor verapamil to decrease the MIC of bedaquiline, thereby diminishing the toxic effect and enhancing treatment.3 In addition, verapamil treatment reversed the observed cross-resistance to clofazimine.1,3
Efflux pump inhibitors can influence drug susceptibility phenotypes in drug-susceptible, drug-tolerant and genetically resistant strains, although to varying degrees. For selected drugs, several studies have reported that the inhibition of efflux pumps does not significantly affect phenotypes in drug-susceptible strains. For example, the intracellular accumulation of drugs such as norfloxacin, rifampicin and KRM-1648 (broad-spectrum benzoxazinorifamycin) could not be modulated by the addition of the efflux pump inhibitors reserpine, verapamil or 2,4-dinitrophenol (DNP) in drug-susceptible mycobacteria.9–13 This suggests that efflux pumps targeted by the above-mentioned efflux pump inhibitors are not active in susceptible strains or that they are expressed at a very low level under the given experimental conditions. In contrast to these results, verapamil has been reported to decrease the MIC of bedaquiline for drug-susceptible isolates.3 It is thus evident that the impact of efflux pump inhibitors in drug-susceptible strains will be influenced by the characteristics of the drug, the nature of the efflux pump inhibitor, strain genetic background and other experimental factors.
In drug-resistant strains with drug resistance-conferring mutations, it is evident that the impact of efflux pump inhibitors is not limited to a single drug,1,3,9 implying that efflux pumps are activated in drug-resistant strains (as described below). Similarly, it has been shown that upon macrophage infection, efflux-mediated drug tolerance is observed for numerous anti-TB drugs including rifampicin, isoniazid, moxifloxacin and bedaquiline.14,15 In a separate study, it was shown that upon rifampicin exposure, ofloxacin tolerance was induced.9 This ofloxacin tolerance phenotype was reversed by efflux pump inhibitors.9 Together, these results demonstrate the role of efflux pumps in host- and drug-induced tolerance. Importantly, recent studies have implicated efflux pump activity in low-level resistance to azithromycin in Mycobacterium avium and to isoniazid in M. tuberculosis.16,17 The low-level efflux-mediated isoniazid resistance observed was followed by the acquisition of an isoniazid resistance-causing mutation, resulting in the hypothesis that efflux pump activity may act as a gateway to the development of drug resistance-causing mutations in M. tuberculosis.17
Findings such as those described above highlight the important role efflux may play in cross-resistance, drug tolerance and the development of drug resistance. Efflux pumps are therefore an attractive target for anti-TB drug design, a research direction that has been investigated in recent years. To date, efflux pump inhibitors have been predominantly used to further understand the role of efflux pumps in mycobacteria.9,15 More recently, efflux pump inhibitors have been considered as potential treatment adjuncts for M. tuberculosis infection.3 It is therefore important to investigate and fully understand efflux pump systems in M. tuberculosis, as well as the use of efflux pump inhibitors in treatment.
This review aims to highlight the current efflux pump inhibitors effective against mycobacteria, especially M. tuberculosis, the effect of efflux pump inhibitors on mycobacterial growth and the clinical significance of treatment with efflux pump inhibitors in combination with standard anti-TB therapy.
Classes of bacterial efflux pumps
Bacterial efflux pumps are categorized into five distinct superfamilies with different structural morphologies, substrate specificities and energy sources.18,19 These include the ATP-binding cassette (ABC) superfamily, the major facilitator superfamily (MFS), the small multidrug resistance (SMR) superfamily, the resistance–nodulation–cell division (RND) superfamily and the multidrug and toxic compound extrusion (MATE) superfamily. All the families are chromosomally encoded and require different energy sources. Efflux pumps of the ABC family, which are generally referred to as primary transporters, utilize the energy of ATP hydrolysis to extrude drugs from the cell.20,21 In contrast, the MFS and SMR, RND and MATE superfamilies are secondary multidrug transporters and use the transmembrane proton-motive force (PMF) or sodium/hydronium ion electrochemical gradient to energize the export of drugs out of the cell.21 One example of the efflux pumps driven by the PMF in Escherichia coli is the AcrAB-TolC system, also known as the RND/PMF efflux machinery. Acriflavine resistance protein A (AcrA) drives the passage of water, in return mediating contact between AcrB and TolC.22 The latter three components have been reported to be necessary for the MDR phenotype.23 Of interest, it was previously shown that RND and ABC transporter activation can be influenced by environmental conditions, specifically pH in E. coli.24 It was demonstrated that AcrAB, driven by PMF, extruded ethidium bromide without the need for metabolic energy at low pH 5 in E. coli, while at high pH 8 the extrusion of ethidium bromide was driven by ABC transporters dependent on metabolic energy.24 Moreover, the ABC transporter efflux pump's action and metabolism resulted in the production of hydronium ions and decrease in host pH,25,26 thereby suggesting that the pH of the environment indirectly regulates mechanisms employed to drive efflux.
Efflux pump inhibitors
The emergence of MDR- and XDR-TB has increased the complexity of designing appropriate treatment regimens given the limited number of anti-TB drugs available. This has emphasized the urgency for drug development and has accelerated progress in new drug regimen development pipelines. Recently, efflux pump inhibitors have been described as putative new drug compounds, since they have the ability to partially restore susceptibility to existing anti-TB drugs by inhibiting the activity of efflux pumps.27 This restoration requires the presence of the efflux pump inhibitor in combination with the anti-TB drugs at subinhibitory concentrations. The anti-TB drug combination is important as the efflux pump inhibitors alone at their subinhibitory concentrations exhibit little or no mycobacterial growth inhibition.9,27 Different types of efflux pump inhibitors exhibit different modes of action that can be either broad or specific to one class of efflux pump.
Calcium (Ca2+) channel blockers
Ca2+ channel blockers (verapamil and phenothiazines) inhibit efflux pump activity by reduction of the transmembrane potential.28–31In vitro and in vivo studies have shown that these compounds inhibit mostly the activity of efflux pumps belonging to the MFS in mycobacteria.32–34
Verapamil
The Ca2+ channel blocker verapamil (Figure 1a) belongs to the phenylalkylamine prototype class. Clinically, verapamil is used to treat various cardiac disorders, headaches and migraines.35–38 In mammalian cells, it acts by inhibiting vesicular monoamine transporters and P-glycoprotein.38 However, in prokaryotes, verapamil inhibits ATP-dependent multidrug transporters and MDR pumps39 by interfering with the generation of the PMF.40 Verapamil has been shown to have a significant inhibitory effect on mycobacterial efflux pump activity.14,15,17,41,42 Recently, it was observed that verapamil could partially restore susceptibility to rifampicin in rifampicin-monoresistant and MDR M. tuberculosis clinical strains.10 Additionally, rifampicin-induced ofloxacin tolerance was reversed and ofloxacin resistance was decreased by >2-fold in M. tuberculosis through the addition of verapamil.43 Similarly, it was shown in vitro that verapamil could restore susceptibility to isoniazid, rifampicin, ofloxacin, streptomycin, ethidium bromide and macrolides in M. tuberculosis and M. avium strains.31,40,42,44 Interestingly, verapamil treatment resulted in a 2- to 16-fold reduction in the level of resistance to the new anti-TB drugs bedaquiline and clofazimine.3 Additionally, verapamil analogues were shown to be effective in reducing the level of resistance to rifampicin by binding to the efflux pump Rv1258c.45 Recently, it was shown that addition of verapamil to M. tuberculosis-infected macrophages resulted in a 34% reduction in cfu, thus enhancing intracellular killing by macrophages in mycobacteria.15 Adams et al.14 also showed that inhibition of mycobacterial efflux pumps with verapamil reduced macrophage-induced tolerance to rifampicin by 2-fold in the Mycobacterium marinum-infected larval zebrafish model. It has further been demonstrated that the addition of verapamil to standard TB chemotherapy in a mouse infection model accelerated the clearance of MDR M. tuberculosis41 and resulted in a significant decrease in the lung bacillary load.9 However, the clinical use of verapamil at high concentrations is not advised, due to severe adverse effects.35,37 Therefore, cytotoxicity studies of verapamil and its analogues are needed to optimize dosage and their clinical use.
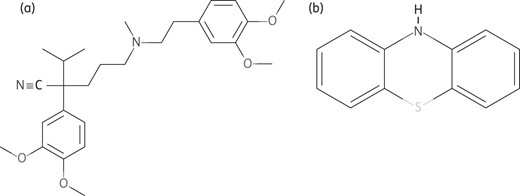
Structures of chemically synthesized Ca2+ channel blockers. (a) Verapamil. (b) Phenothiazines. Obtained from PubChem; http://www.ncbi.nlm.nih.gov/pccompound.
Phenothiazines
Phenothiazine (Figure 1b) is a tricyclic compound of which derivatives including chlorpromazine, piperidine and thioridazine are widely used clinically as antipsychotic and antihistaminic compounds.46 Phenothiazine and its derivatives are potential inhibitors of K+ transport and Ca2+ channels, with the ability to reverse the MDR phenotype in M. tuberculosis, Mycobacterium smegmatis and M. avium complex.47–49 They also inhibit the PMF-dependent MFS pumps by reducing transmembrane potential.50,51 Recent studies have shown that both thioridazine and chlorpromazine inhibit ethidium bromide efflux in M. smegmatis and M. avium complex.31,52 Moreover, both compounds have been proven to reduce clarithromycin and isoniazid resistance in M. tuberculosis complex.40 Thioridazine was shown to specifically enhance the intracellular killing of phagocytized M. tuberculosis.49,52,53 Thioridazine also exhibits in vitro, in vivo and ex vivo growth inhibition of susceptible and resistant M. tuberculosis strains.54 Due to its limited inhibitory effect in vitro,40,54 it has been suggested that modifying phenothiazine's basic structure will enhance its efficacy significantly.54 However, increasing the potency and efficacy of these compounds might result in an increase in cytotoxicity, thus limiting their use clinically.
Protonophores
Protonophores, which include CCCP, DNP and valinomycin, inhibit efflux pump activity by reducing the transmembrane potential.28,29,55In vitro and in vivo studies have shown that these compounds inhibit largely the activity of efflux pumps belonging to the MFS in mycobacteria.32–34 CCCP (Figure 2a) and DNP (Figure 2b) disperse the membrane PMF by means of modification of the transmembrane electrochemical potential; thus, these compounds tend to be toxic to the cell and are experimentally studied at sublethal concentrations.34,55 Additionally, these compounds are ionophores that act as chemical inhibitors of oxidative phosphorylation, which, in turn, serves to inhibit the activity of ATP synthase; therefore, CCCP and DNP have an effect on ABC superfamily pumps.56 In recent in vitro studies, it was observed that the addition of CCCP or DNP to ofloxacin-resistant M. tuberculosis clinical isolates with an ofloxacin resistance-conferring gyrA mutation resulted in a 2- to 8-fold decrease in the ofloxacin MIC.32 In a separate study, it was observed that a number of ofloxacin-resistant M. tuberculosis strains exhibited a >2-fold decrease in the ofloxacin MIC with the addition of CCCP.43 This suggests the importance of induced efflux pump activity in fluoroquinolone resistance.32,57 Another study showed that the addition of CCCP decreased resistance to each of rifampicin, isoniazid, streptomycin and ofloxacin in M. tuberculosis isolates.44 CCCP also reduced the level of resistance to isoniazid, ethambutol, ethionamide, erythromycin and acriflavine in M. tuberculosis overexpressing efflux pumps JefA (Rv2459) and Mmr (Rv3065), thereby supporting CCCP as an effective efflux pump inhibitor.42,57 Both CCCP and DNP showed the ability to inhibit the activity of the P55 tap-like efflux pump, where P55 is a tap-like MFS multidrug efflux pump that confers low-level resistance to a broad spectrum of compounds.58,59 In addition, CCCP inhibited the activity of MmpS5-MmpL5 efflux pumps in M. tuberculosis and Mycobacterium bovis bacillus Calmette–Guérin (BCG) WT strains as measured by [3H]enconazole levels.34,60 Together, these findings suggest that the inhibitory effect of CCCP and DNP may lead to a decrease in the level of resistance, thereby potentially improving the efficacy of the administered TB treatment. However, high concentrations of CCCP are toxic in vivo, thereby emphasizing the need for CCCP optimization studies, in order to determine optimal safe and effective concentrations in vivo.
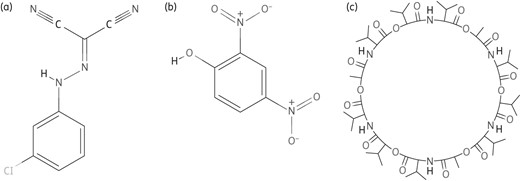
Structures of chemically synthesized PMF blockers. (a) CCCP. (b) DNP. (c) Valinomycin. Obtained from PubChem; http://www.ncbi.nlm.nih.gov/pccompound.
Valinomycin
Valinomycin (Figure 2c) is a compound that depletes the electrochemical gradient generated by K+.34,61 It is commercially known as dodecadepsipeptide, a compound extracted from a Streptomyces species.61 Valinomycin is a potassium-specific efflux pump inhibitor with high selectivity for Na+ and K+. It facilitates the movement of K+ through lipid membranes ‘down’ an electrochemical potential gradient and targets the MFS and ABC superfamily.61,62 Valinomycin has been shown to inhibit the P55 efflux pump, which relies on transmembrane proton and electrochemical gradients as sources of energy for the active export of compounds in M. tuberculosis.58 Microarray analysis of M. tuberculosis strains treated with valinomycin showed a significant decrease of p27 and p55 expression levels, therefore suggesting efflux-mediated drug resistance.58 The treatment of naturally pyrazinamide-resistant M. smegmatis with valinomycin showed an increase in the accumulation of pyrazinoic acid at neutral pH,63 illustrating the role of efflux activity in the pyrazinamide-resistant phenotype. Addition of valinomycin also stimulated isoniazid accumulation in M. smegmatis.64 However, the accumulated isoniazid was partially decreased by nigericin, an inhibitor of K+ transporters,64 suggesting that nigericin could be antagonistic to valinomycin's mechanism of action.
Plant-derived efflux pump inhibitors
In addition to inhibiting multidrug transporters, plant-derived alkaloids (efflux pump inhibitors) are emerging adjuvants with the potential ability to synergistically improve the potency of some anti-TB drugs. Such alkaloids include: the plant alkaloid reserpine (Figure 3a); piperine, the trans-trans isomer of 1-piperoyl-piperine from the Piperaceae family (Figure 3b); and berberine, from the Berberidaceae family (Figure 3c).65–68 Basic molecular and clinical research is required on these compounds, as limited data currently exist regarding their interaction in vitro and in vivo.

Chemical structures of efflux pump inhibitors derived from natural resources. (a) Reserpine. (b) Piperine. (c) Berberine. Obtained from PubChem; http://www.ncbi.nlm.nih.gov/pccompound.
Reserpine
Reserpine (Figure 3a) is a naturally occurring compound that was originally isolated from the roots of Rauwolfia vomitoria Afz.65,66,69 It acts by irreversibly blocking the uptake and storage of dopamine into (or in) synaptic vesicles, by means of inhibiting the vesicular monoamine transporters.66 It is used to treat hypertension and psychiatric disorders at daily doses that do not exceed 25 mg due to potential adverse effects.66,69 Reserpine is considered to be a promising efflux pump inhibitor that targets efflux pumps in the RND superfamily, MFS and membrane protein classes. Reserpine is reported to potentiate drug treatment by interacting directly with amino acids in the efflux protein transporters, as is characteristic of some efflux proteins.70 An example of the latter interaction is that of reserpine and the Bmr protein, a member of the MFS transporters that is responsible for tetracycline efflux in Bacillus subtilis.71 Of interest, it was observed in B. subtilis that addition of reserpine decreased the tetracycline MIC by 4-fold.72 Reserpine has been shown to influence the level of resistance to numerous anti-TB compounds in mycobacteria. This is exemplified by the observation that treatment of isoniazid-resistant strains with reserpine reversed high-level isoniazid resistance conferred by overexpression of the M. tuberculosis mmpL7 gene in M. smegmatis.73 Reserpine has also been shown to increase susceptibility to both isoniazid and ethidium bromide in M. bovis BCG susceptible strains and to isoniazid in M. tuberculosis susceptible strains.74,75 Additionally, reserpine partially restored susceptibility to ofloxacin in 15 MDR-TB isolates.9 This efflux pump inhibitor increased susceptibility to pyrazinamide in M. tuberculosis by blocking the pyrazinoic acid efflux pump responsible for pumping out pyrazinamide.63,76
Piperine
Piperine (Figure 3b) is present in black pepper and can be isolated from Piper nigrum.77 It was used in ancient times in some forms of traditional medicine. Piperine is a drug potentiator that inhibits the human P-glycoprotein,77 particularly cytochrome P450-mediated pathways, and mediates inhibition of glucuronidation activity in animal models.78 Piperine is used commercially as an inhibitor of enzymes that are important both in drug metabolism and in the transport of metabolites and xenobiotics. It simultaneously increases the bioavailability of various compounds and reduces the efficacy of some medications.68 Studies of piperine and piperidine suggest an inhibitory action against active bacterial efflux pumps, including those in mycobacteria.79,80 A study conducted in M. smegmatis showed that piperine (32 mg/L) could decrease the MIC of ethidium bromide by 2-fold, thus demonstrating piperine's ability to inhibit mycobacterial efflux pumps.80 These experiments have stimulated more studies focusing on the effect of this efflux pump inhibitor in M. tuberculosis. This includes a recent study in a mouse infection model of M. tuberculosis where the protective efficacy of piperine in combination with rifampicin (1 mg/kg) was tested. Piperine was synergistic with rifampicin treatment, resulting in a 6-fold decrease in lung cfu, thereby improving the efficacy of rifampicin.81 These findings have clinical implications for treatment of immunocompromised TB patients. Regardless of the limited number of studies in mycobacteria, piperine has been shown to be effective in other pathogenic bacteria. For example, the MIC of ciprofloxacin was reduced 2-fold for a NorA-overexpressing Staphylococcus aureus strain in the presence of piperine.82
Berberine
Berberine (Figure 3c) is a nucleic acid-binding isoquinolone alkaloid exhibiting broad-spectrum therapeutic properties that was isolated from Berberis fremontii.83 Studies on berberine have primarily focused on its beneficial effects on the cardiovascular system, its anti-inflammatory properties and its ability to suppress the growth of different tumour cells in cancer.84,85 It is also used to treat neuroinflammation-associated disorders.84 Berberine was previously suggested to have weak antibacterial activity when acting alone; however, it has been shown to have a synergistic effect when used in combination with other compounds such as 5′-methoxyhydnocarpin-D, norfloxacin and other NorA substrates in mycobacteria.86,87 It has been demonstrated that berberine binds to the TetR-like transcriptional repressor RamR. This interaction results in the expression of RamA and subsequent induction of the multidrug efflux system genes acrAB-tolC.88 Although no data exist on the role of this compound and its targets in mycobacterial resistance, published data support its further investigation in this context.
Efflux pump inhibitors as adjuvant therapy
While numerous in vitro studies have demonstrated the effect of efflux pump inhibitors on anti-TB treatment in mycobacteria, in vivo use of efflux pump inhibitors as an adjuvant to treatment regimens has only recently been explored. Investigation of efflux-mediated drug tolerance in a larval zebrafish model of infection suggested the potential advantages of inclusion of efflux pump inhibitors in treatment regimens.14,89 Follow-up studies showed the success of inclusion of verapamil in reducing macrophage-induced tolerance to numerous anti-TB compounds, including bedaquiline and moxifloxacin.15 Based on their findings, they subsequently hypothesized that verapamil may have success in shortening treatment periods. Treatment shortening by inclusion of verapamil into standard treatment regimens in a mouse model of infection was later demonstrated by Gupta et al.3 Coadministration of verapamil with standard chemotherapy resulted in treatment shortening, as well as a decrease in relapse rates in C3HeB/FeJ mice.41
Studies investigating the role of efflux activity in bedaquiline and clofazimine cross-resistance further determined the impact of the inclusion of verapamil into regimens containing these newer anti-TB compounds. Verapamil was found to decrease the MIC of bedaquiline and clofazimine by 8- to 16-fold and 8-fold, respectively.3 This decrease in MIC was irrespective of the drug resistance profile of the M. tuberculosis isolates tested. The authors hypothesize that their findings may hold true in in vivo studies, based on the previous studies.3,15 Gupta et al.3 also demonstrated that inclusion of verapamil may shorten treatment periods for bedaquiline and clofazimine and additionally may allow for lower doses of bedaquiline to be used in treatment regimens, thereby lowering dose-related bedaquiline toxicities. Nevertheless, these studies were conducted in mice and might not have taken into account the metabolism of verapamil by cytochrome P450 in humans.
Together, these findings demonstrate the potential of including efflux pump inhibitors in treatment regimens to improve and shorten anti-TB treatment. However, there are still numerous factors to be addressed to overcome limitations of adverse effects associated with high concentrations of efflux pump inhibitors in vivo.
New compounds: improvement of current efflux pump inhibitors
It has been noted that it is not known if the concentration of verapamil used in in vitro studies is attainable in patients.15 Verapamil is also known to have negative cardiac side effects.90 It is therefore vital to further investigate efflux pump inhibitors as a viable treatment option by improving current efflux pump inhibitors and searching for new compounds. One such example is norverapamil, a metabolite of verapamil that has less Ca2+ channel-blocking activity. Norverapamil exhibited the same activity as verapamil for inhibiting macrophage-induced drug tolerance in a macrophage infection model.15 Also of interest are two new, recently reported compounds that exhibit efflux pump inhibitor activity in mycobacteria. Farnesol (15-carbon isoprenoid component) (Figure 4a) is a natural plant metabolite that inhibits oxidation-reduction reactions and disrupts membrane integrity by causing K+ leakage from cytoplasm.21,91 In a chequerboard synergy assay conducted in M. smegmatis, the combination of farnesol and ethidium bromide at a concentration of 32 mg/L [fractional inhibitory concentration (FIC) = 0.625; an index range used to assess the interaction of different drugs] decreased the MIC of ethidium bromide by 8- and 4-fold, respectively, at 16 mg/L (FIC = 0.375). Furthermore, synergy was observed between farnesol and rifampicin. The potential clinical use of farnesol is highlighted by its potency, as well as its low toxicity in humans.91
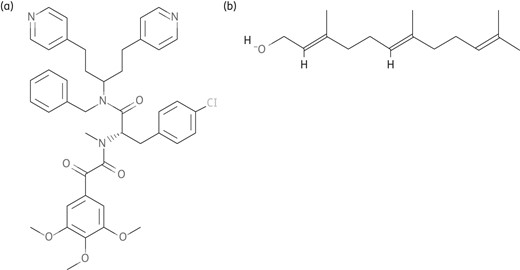
Structures of newly reported compounds. (a) Farnesol. (b) Timcodar. Obtained from PubChem; http://www.ncbi.nlm.nih.gov/pccompound.
Another compound demonstrating efflux pump inhibitor activity is a mammalian efflux pump inhibitor, timcodar (formerly VX-853) (Figure 4b). An adjuvant effect was demonstrated in combination with antimycobacterial drugs (rifampicin, bedaquiline and clofazimine) when M. tuberculosis was cultured in host macrophages.92 The same efflux pump inhibitor in combination with either rifampicin, bedaquiline or clofazimine resulted in an ∼10-fold increase (IC50 of 1.9 mg/L) in the growth inhibition of M. tuberculosis and synergy was observed in combination with rifampicin, moxifloxacin and clofazimine. Importantly, in a mouse model of infection, timcodar treatment resulted in 1.0 and 0.4 log10 reduction in bacterial burden when used in combination with rifampicin and isoniazid, respectively. This suggests its promise as an adjuvant treatment.92
Concluding remarks
Recent research has shown that multidrug resistance in M. tuberculosis is associated with constitutive or inducible expression of efflux systems. The complexity of resistance mechanisms such as those mediated by efflux pumps has allowed M. tuberculosis to evolve in such a way that it is now potentially resistant to most anti-TB drugs. This highlights the need for the development of new treatment regimens. Such regimens could potentially include the use of efflux pump inhibitors, in combination with existing or new drugs, to help increase the potency of current anti-TB drugs. Some promising in vitro and in vivo reports suggest the importance of using efflux pump inhibitors as adjuncts to improve TB chemotherapy. These efflux pump inhibitors (synthetic or derived from plant or bacterial sources) target various classes of efflux pumps (Figure 5) and can be grouped into broad-spectrum or selective inhibitors. Broad-spectrum inhibitors are ideal candidates for adjuvant therapy. However, these are difficult to develop and pose a greater risk of being more toxic to human cells. Selective inhibitors such as inhibitors of cytochrome bcd are good candidates for diagnostic purposes. Even though it is a potential advantage to use clinically approved efflux pump inhibitors in combination therapy, it is imperative to tailor the pharmacokinetics of the efflux pump inhibitor to the pharmacokinetics of the antibiotic in order to optimize synergy.
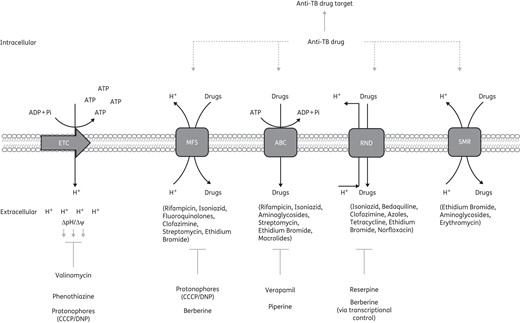
Efflux pump inhibitor mode of action and the effect on anti-TB therapy. Numerous efflux pump inhibitors have been shown to target specific efflux pump classes; however, some efflux pump inhibitors act indirectly to block efflux pump activity. Valinomycin, phenothiazine and the protonophores diminish the membrane potential generated by the PMF. The collapse in the PMF negatively impacts the activity of some efflux pumps. Berberine affects efflux pump activity via transcriptional control of efflux pump genes. Addition of efflux pump inhibitors has been shown to impact efficacy of anti-TB therapy, i.e. anti-TB drugs and other drugs may be extruded from the cell by efflux pumps; however, this efflux would be blocked by the presence of efflux pump inhibitors. This therefore allows anti-TB drugs/other drugs to remain in the cell and act on their drug targets. ETC, electron transport chain.
Promising advances have provided new hope for the design and efficacy of efflux pump inhibitor/antibiotic combination regimens, targeting efflux pump activity to improve TB treatment. The synthesis of efflux pump inhibitor analogues of known efflux pump inhibitors, such as verapamil, has also been explored recently and proven to be an interesting alternative route. These include certain natural sources (e.g. plants) that can be exploited for medicinal purposes, as seen with berberine, piperine and reserpine. The inhibitory compounds seem to enhance the activity of existing anti-TB drugs, either through synergism or through additive effects. This observation emphasizes the need for more advanced studies on this topic. An additional appealing characteristic of efflux pump inhibitors is their low mutation frequency, making them more potent against M. tuberculosis at therapeutically achievable concentrations. Certain naturally occurring compounds such as reserpine, piperine and berberine might have the potential to be exploited synergistically with current TB treatment regimens to improve the therapeutic efficacy. Furthermore, molecular docking analyses of well-studied putative efflux pumps could advance our knowledge regarding the potential use of efflux pump inhibitors as adjuncts to synergistically repurpose traditional anti-TB drugs.
In summary, it is evident from the present review that use of efflux pump inhibitors might offer an effective strategy to enhance the potency of current and newly emerging anti-TB drugs.
Funding
This work was sponsored by a Medical Research Council of South Africa Scholarship (to C. M. P.), a Claude Leon post-doctoral fellowship (to G. E. L.) and a National Research Foundation grant (CPR 81776) (to R. M. W.). S. L. S. is funded by the South African Research Chairs Initiative of the Department of Science and Technology and National Research Foundation of South Africa, award number UID 86539.
Transparency declarations
None to declare.
Disclaimer
The content is solely the responsibility of the authors and does not necessarily represent the official views of the funders.
Acknowledgements
We would like to thank the South African Medical Research Council and the National Research Foundation for their financial support.