-
PDF
- Split View
-
Views
-
Cite
Cite
Aliza Zulfiqar, Muhammad Imran, Muhammad Haseeb Ahmad, Shyam S Sablani, Muhammad Kamran Khan, Ultrasound-assisted conjugation of Moringa proteins with gallic acid: characterisation of structural and techno-functional properties, International Journal of Food Science and Technology, Volume 59, Issue 10, October 2024, Pages 7102–7112, https://doi.org/10.1111/ijfs.17427
- Share Icon Share
Abstract
In this study, ultrasound-assisted covalent binding method, free radical-induced method, was used to prepare Moringa protein isolate (MPI) – gallic acid conjugates (MPI-GAC) to examine the improvement of the sonication application. Additionally, the influence of polyphenol conjugation on functional and structural attributes of MPI was assessed thru spectral analysis, scanning electron microscopy, antioxidant activity, emulsification, foaming, and water and oil holding properties. The enhanced value of grafted polyphenol species was observed after introducing ultrasound treatment during free radical mediation process. The structural changes in protein by gallic acid conjugation were confirmed by using FT-IR which illustrated the modification in MPI-GAC with modified peak width of the amide-I band. Scanning electron microscopy also showed a significant difference in the structure of MPI and MPI-GAC. The grafting of gallic acid onto MPI directed under ultrasound-assisted conditions conferred distinctive functional characteristics, containing elevated emulsification capacity (51.4 mg mL−1) along with enhanced water and oil holding capacity by 2.07 and 5.11 g g−1, respectively. Furthermore, conjugation also increased the protein solubility 89.3–97.6% and significant trend (68.04–88.06%) of antioxidant capacity was observed by increasing the concentration. The findings of this research work offer new insights on the effective fabrication of MPI-GAC with multifaceted functionalities, thus broadening the scope and possibilities of high-value Moringa seed protein.
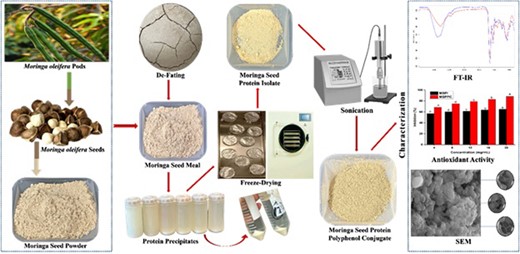
Introduction
Proteins constitute vital amino acids essential for the sustaining functions of human body across all ages and extensively utilised in food industry to develop healthy food products to address nutrition demand. A billion animals are raised and consumed to satisfy the meat eating of the world population. Excessive meat consumption is not only associated with higher costs but also causes detrimental effects on health, for instance obesity and cardiovascular problems (Godfray et al., 2018). In developing countries, protein malnutrition or inadequacy of protein energy is one of the significant issues that is directly related to people's affordability, about 95 million Pakistanis now live in poverty and cannot afford protein from animal sources. To address these challenges, alternative protein sources including plant proteins can be used as excellent substitutes for animal proteins which offering nutritional advantages, environmental sustainability, and affordability (Jain et al., 2019).
Conspicuously plant protein isolates from wheat, soy, and pea are commonly utilised in food industry owing to their worthy functional characteristics, like gel formation and emulsification but there are limitations owing to lesser protein content and quality (Schreuders et al., 2019). Researchers now expanded the horizon to find novel resources with high-quality protein. Moringa oleifera is renowned for its adaptability to challenging environmental conditions such as drought and extreme temperatures. This resilience may allow it to be cultivated in different tropical and subtropical regions across Asian and African countries, Moringa oleifera commonly known as “sohanjna” is a popular plant. It is a nutrient-dense plant, and all of its parts are being used and known for many nutritional benefits, and extremely fast-growing plant at affordable cost which makes it the best alternative protein source with sustainable resources (Tang et al., 2021).
Moringa oleifera seeds are the richest in protein compared to other parts of the plant and are also known as plant diamonds. Seed is comprised of 37–40% protein including the essential amino acid which makes it the best option for protein alternative sources (Liang et al., 2019). To accomplish comparable functional properties of plant proteins regarding industrial use, several modifications are being applied. Sonication and conjugation using polyphenols can indeed be used at the industrial level for modifying plant proteins to improve their functional properties. It is effective for both batch and continuous processing, depending on the specific application needs. High-power ultrasound has only become an efficient tool, recently, for commercial applications, like emulsification, homogenisation, extraction, crystallisation, dewatering, low-temperature pasteurisation, degassing, defoaming, activation and inactivation of enzymes, particle size reduction and viscosity alteration. The process of polyphenol conjugation with proteins using sonication can be scaled up to the industrial level. They offer ways to improve the functional properties of proteins to meet the diverse needs of industries ranging from food and beverage to pharmaceuticals and cosmetics (Xu et al., 2021). Due to consumer demand for safe, clean labels and toxin-free products now methods for the modification of protein have also been upgraded. Novel physical techniques are preferred to chemical and enzymatic modifications due to the toxicity associated with them (Pojić et al., 2018).
Among the various novel techniques ‘plant protein-polyphenol conjugation’ is one of the best techniques that aligns with both environmental sustainability and the growing demand for healthy food (Gong et al., 2023). Polyphenols are also extracted from the plants as they are the by-products of plant reactions. Protein-phenols reaction along with ultra-sonication allows unfolding, disintegration, and rearrangement of structure which results in modification of functional properties (Dzah et al., 2020; Kim et al., 2024). Interaction between plant proteins and phenols is generally the method that oxidised the hydroxyl groups of polyphenols to quinones which are then attached to nucleophilic groups of proteins through grafting. Several studies on protein-polyphenols conjugation resulted in enhanced functional and antioxidant properties being reported in recent years, for instance, hemp protein isolate-polyphenol conjugates (Liu et al., 2023), soy protein isolates-cyanidin-3-galactoside conjugates (Xue et al., 2020), perilla seed meal proteins-different polyphenols conjugates (Wang et al., 2024) and there is a lack of moringa protein isolates and polyphenol conjugates. Moreover, coupling conjugation utilising polyphenols with ultrasound-assisted sonication offers several advantages, particularly in the context of modifying plant proteins for food or nutraceutical applications. The combined effect of ultrasound and polyphenol conjugation allows for precise control over the modification process. Sonication can be adjusted to achieve specific structural changes in proteins, while polyphenol conjugation provides a method to tailor functional properties such as emulsification, foaming, and gelation. Induction of polyphenol group in protein improves overall stability and antioxidant properties of protein and these modified proteins have good lipophilic and hydrophilic properties and are therefore used as a carrier for control release of bioactive compounds, improve the texture and stability of beverages, as well as enhance the nutritional profile of fortified foods. Besides, it offers meat and dairy alternatives along with allergen-friendly products (Benjakul et al., 2021).
This research was designed to modify the protein structure by conjugating Moringa oleifera seed protein with gallic acid polyphenol and determine the changes in functional and structural properties. Functional properties comprising protein solubility, water holding and oil holding capacity, emulsion and foaming properties were investigated. The antioxidant potential of conjugates was also studied. These changes, endorsed by structural changes were examined using FT-IR and scanning electron microscopy.
Material and method
Materials
Seeds of Moringa oleifera were picked up during first spring harvesting from Virsa farm, Faisalabad, Pakistan and stored in a clean plastic bag at 15 °C to avoid the humidity. Gallic acid was purchased from Merck, USA. Petroleum ether (95% purity) and other chemicals were procured from Lab Alley, Texas, USA.
Preparation of Moringa seed powder
Dried seeds of Moringa were dehulled and cleaned. Dehulled Moringa seeds were grounded to powder by using a lab scale grinder (Golden wall 700 g Electric grain grinder mill) at a pilot processing plant, School of Food Sciences, Washington State University, USA. This grounded powder was subsequently sifted by a 100 mesh size sieve to achieve a fine seed powder.
Proximate analysis
Proximate analysis of Moringa seed powder, including moisture content, protein, ash, crude fat, and fibre was determined by adopting the protocols prescribed by the Association of Official Analytical Chemists (AOAC, 2005).
Preparation of defatted Moringa seed meal
Ground Moringa seed powder was defatted by using petroleum ether until the fat content was reduced <0.5%. Moringa seed powder was mixed with petroleum ether, the quantity of petroleum ether was three times the flour. The mixture was stirred continuously for 1 h by following sedimentation and filtration process. The process was repeated one more time for 1 h by dispersing in the same volume of petroleum ether. After that petroleum ether was decanted and the remaining in powder was evaporated by placing it in a fume hood. This defatted Moringa seed powder was again grounded to obtain a fine Moringa seed meal (MSM).
Isolation of Moringa seed protein
Moringa seed protein isolates were obtained following the method outlined by Aderinola et al. (2018) and represented in Fig. 1. In order to obtain the Moringa seed protein isolates, MSM was dispersed in 0.1 M NaOH solution at a ratio of 1:20 (w/v). The initial pH of dispersion was 12, it was stirred for 60 min at room temperature continuously for greater solubility. After 1 h of stirring the dispersion was added in 50 mL centrifugation tubes and centrifuged at 6000 rpm for 1 h at ambient temperature. After centrifugation, there was supernatant S1 and precipitates P1. P1 was discarded and S1 was shifted into a separate beaker. The pH of the supernatant was adjusted while stirring with a magnetic stirrer to an acidic, pH of 3.5. This acidified supernatant was then placed in the refrigerator at 4 °C overnight to get the precipitate. On the next day, it was centrifuged at 6000 rpm for 60 min at ambient temperature, centrifugation resulted in supernatant S2 and Precipitates P2. S2 was discarded and P2 was the protein. P2 was transferred to a beaker after 2 times washing with distilled water. The pH of the extract was then fixed to 7 (Neutral). The obtained extract was ultra-filtered to get the Moringa protein isolates (MPI). An ultrafiltration process was carried out for extraction purposes by using a stirred membrane cell unit (Amicon 8050, Millipore, USA) equipped with a 25–30 kDa (MWCO) membrane at 0.4 MPa. The obtained extract was diluted (sixfold) with chilled water and resulted solution was then centrifuged for 10 min at 10 °C at 10 000 g. Finally, the resulting protein precipitates (MPI) were subjected to freeze-drying (8624-FDU, Operon, South Korea) for 48 h at −85 °C and then stored at 4 °C for further investigation.
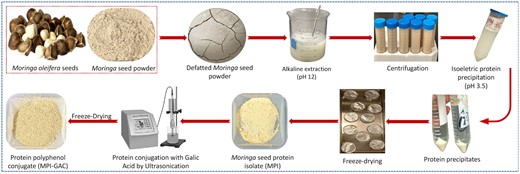
Protein estimation and precipitate yield
Protein content in MSM and MPI was assessed by adopting the AOAC micro-Kjeldahl technique (AOAC, 2005). Protein content was quantified by multiplying nitrogen content by 6.25. Precipitation yield was calculated to check the efficiency of the process. After the freeze-drying of samples, the yield was calculated by using the formula:
Formation of Moringa seed protein polyphenol conjugates (MPI-GAC)
Moringa seed protein polyphenol conjugate (MPI-GAC) formation was performed using the method described by Wang et al. (2024). The 1 g of MPI was mixed with 15 mg of gallic acid and dissolved in distilled water (100 mL). The solution was homogenised for 1 min at 10 000 rpm by operating a homogeniser (Ultra-Turrax TP 18/10S1, Germany), and the pH of the solution was adjusted to 9.00 by adding 1 M NaOH. To make protein-polyphenol conjugate, the dispersion was sonicated at 50% amplitude by using a sonicator (200 Watt, UP200S, Heischler, Germany) with a 0.636 cm diameter titanium probe for 20 min and sonication was applied for 4 s on 4 s off pulsing in the ice bath. The pH was adjusted to 7.00, which allowed to freeze-dried and the samples were stored at 6 °C for further investigation (Fig. 1).
Analysis of Moringa oleifera seed protein isolates and Moringa seed protein polyphenol conjugates
Protein solubility
The solubility of protein was measured in accordance with the protocols prescribed by Zou et al. (2017). 10 mg of each sample was mixed with distilled water (8 mL) and pH was adjusted under the range of 2–10 with the help of 0.1 M NaOH. The prepared solution was stirred at ambient temperature for 30 min through a magnetic stirrer and the volume was then fixed at 10 mL adding pH solution. Additionally, the sample was centrifuged at 6000 rpm for 20 min and protein in supernatant was measured by adopting Bradford procedures. The solubility was calculated using the mentioned formula:
Water holding capacity
The Water holding capacity (WHC) was determined by dispersing 1 g of each sample into deionised water (10 mL) and mixing vigorously at ambient temperature. The sample was left for 30 min and centrifuged at 3000 rpm for 13 min at ambient temperature. Finally, the supernatant was removed and the weight of the tube was noted Yılmaz and Hüriyet (2017). WHC was calculated using the mentioned formula:
where W0 = Weight of sample in grams, W1 = Weight of sample and tube, W2 = Weight of remnants and tube.
Oil holding capacity
The oil holding capacity (OHC) was measured by adopting the protocols prescribed by Yılmaz and Hüriyet (2017) with some modifications. The 1 g of both samples was added to 10 mL of corn oil and mixture was mixed properly. The sample was left for 30 min and centrifuged at 3000 rpm for 13 min. The tube was weighed after removing the supernatant. OHC was calculated by using the given formula:
where W0 = Weight of sample in grams, W1 = Weight of sample and tube, W2 = Weight of residues and tube.
Emulsion capacity and emulsion stability
Emulsion capacity (EC) and stability (ES) were assessed by following the procedure given by Jiang et al. (2009). 1 g of each sample was dissolved in 50 mL 0.1 N NaOH in a beaker (250 mL) and 50 mL of sunflower oil was added to the mixture. The resulting mixture was homogenised by using a homogeniser (Ultra-Turrax TP 18/10S1, Janke & Kunkel, Saufen, Germany) at a speed of 10 000 rpm for 2–3 min to make an emulsion. The resulting emulsion was shifted into a 100 mL measuring cylinder. The emulsion capacity was measured through the formula given below:
Emulsion stability was measured by observing the emulsion after 48 h by using the formula given below:
Foaming capacity and stability
Foaming capacity (FC) and stability (FS) were assessed by using the procedures given by Phongthai et al. (2016). To measure foaming capacity and foaming stability 50 mL of 0.1% (w/v) from each sample solution was placed into a beaker (150 mL). Then, it was homogenised at a speed of 20 000 rpm for 1–2 min. The volume was calculated at 0 and 30 min and both values were measured by using the formulas:
Where, FC = Foaming capacity, FS = Foaming stability, V1 = Volume of protein solution after 0-min of homogenisation, V0 = Volume of protein solution before homogenisation, V2 = Volume of protein solution after 30-min of homogenisation.
Antioxidant activity
Antioxidant activity was measured using the following methodology outlined by Sharma and Bhat (2009), and 1 g of each powdered sample was taken and mixed in 25 mL of 99% methanol and sealed the sample by using aluminium foil and Para film. To prepare 1 M DPPH solution 4 mg of DPPH and 100 mL of 99% methanol were taken in a 100 mL volumetric flask covered with aluminium foil and placed at cool conditions. Samples were placed in a shaking water bath at 100 rpm at ambient temperature for 150 min. Then, the samples were centrifuged at 6000 rpm for 15 min and supernatant was collected to make solution series by using supernatant and methanol. A final volume of 10 mL was made for five different series by taking 1, 2, 3, 4, and 5 mL from supernatant and remaining 99% methanol. 1 mL of prepared solution was taken in a 10 mL volumetric flask from five different sample series and mixed it with 3 mL of DPPH solution to make 10 mL volume by using 99% methanol and keeping them in a dark place for 30 min. Absorbance was analysed by using a Shimadzu UV 160 U spectrophotometer under the wavelength range of 517 nm. Antioxidant activity was calculated by using the following formula:
Fourier-transform infrared spectroscopy
The Attenuated Total Reflectance-Fourier Transform Infrared (ATR FT-IR) Spectroscopy was applied to analyse the pattern of powdered samples of MPI and MPPC after freeze-drying. The spectra of each sample were obtained by using the FT-IR Shimadzu IR Prestige-21 spectrophotometer, with an ATR accessory having in MIR region 4000–600 cm−1. The analysis was performed at ambient temperature and spectra of each sample were carried out in triplicates. Before taking the spectra of the sample, the spectra of the empty Diamond ATR accessory were taken to define the background. Spectra of samples were acquired through 64 scans at 10 kHz speed. ATR was cleaned after every sample with cellulose tissue (Mir et al., 2019).
Scanning electron microscopy
Scanning electron microscopy was performed by adopting the protocols outlined by (Zhou et al., 2020). Scanning electron microscopy is operated to analyse the detailed structure of Moringa seed protein isolate and Moringa protein-polyphenol conjugates. The powder sample in a small amount was placed on conductive glue and the excess sample was removed from the top. The samples were examined under the microscope at 26 kV and selected magnifications for pictures were 5, 10 and 30 k.
Statistical analysis
All analysis was conducted three times for each sample and data for each sample was calculated as mean value ± standard deviation. One-way analysis of variance (ANOVA) was performed using Minitab Statistical Software 19 and differences between samples means were compared using the Duncan's multiple range test, taking statistically significant at P < 0.05.
Results and discussion
Protein estimation and precipitate yield
Extracted protein as MPI was further concentrated by using membrane processing to reduce the water amount required for protein precipitation which leads to efficiency gain. Protein content in MSM was (35.5 ± 0.67b g/100 g, db) and in MPI was (91.2 ± 0.48a g/100 g, db). The result of MSM was consistent with a study previously reported by Bakwo Bassogog et al. (2022), wherein effect of heat on Moringa oleifera seed protein studied but the protein content of MPI was relatively high compared to studies by the same process. The significant difference in protein content might be due to the selection of salt, in related studies NaCl was used for alkaline extraction but in this study, NaOH was used as an alkali, it increased the protein extraction by breaking down the cell membrane and facilitated the release of protein. The precipitation yield after membrane processing was 54% which was in line with the outcomes published by Jain et al. (2019).
Protein solubility
Protein solubility refers as the protein concentration in saturated solution that is in equilibrium with the solid phase under particular conditions and considered as thermodynamic property. Solubility can be increased or decreased depending on extrinsic or intrinsic properties (Kramer et al., 2012). Protein solubility of MPI-GAC increased significantly after conjugation with gallic acid, this increase may be owing to a small particle size of MPI-GAC. As shown in Table 1, at neutral pH, the solubility of MPI was 89.3 ± 0.71 and after conjugation, the solubility of MPI-GAC was 98 ± 0.97. This phenomenon may possibly be attributed to cavitation influence induced by ultra-sonication that results in the breakage of hydrophobic interactions that result in increased protein water affinity and an increased number of soluble protein aggregates. Conjugation also affects solubility significantly (P < 0.05) by reducing particle size, and covalent and non-covalent bonding between MSP and gallic acid. The protein solubility of Moringa protein isolate was in accordance with Cattan et al. (2022) and similar results have been reported in prior research study where protein isolate-polyphenol conjugates were subjected to ultrasound treatment increased the solubility of hemp protein Liu et al. (2023). Xue et al. (2020) found a significant enhancement in the solubility of soy protein after conjugating with cyanidin-3-galactoside was observed by using a sonication method.
Functional properties of Moringa oleifera seed protein isolate and Moringa oleifera seed protein isolate-polyphenol conjugate
Functional properties . | MPI . | MPI-GAC . |
---|---|---|
Water holding capacity (g g−1) | 0.79 ± 0.02b | 2.07 ± 0.03a |
Oil holding capacity (g g−1) | 1.50 ± 0.03b | 5.11 ± 0.05a |
Emulsion capacity (mg mL−1) | 28.1 ± 0.62b | 51.4 ± 0.43a |
Emulsion stability (%) | 22.2 ± 0.60b | 42.0 ± 0.86a |
Foaming capacity (%) | 70.4 ± 0.30b | 94.3 ± 0.32a |
Foaming stability (%) | 82.7 ± 0.43b | 93.2 ± 0.21a |
Protein solubility (%) | 89.3 ± 0.71b | 97.6 ± 0.46a |
Functional properties . | MPI . | MPI-GAC . |
---|---|---|
Water holding capacity (g g−1) | 0.79 ± 0.02b | 2.07 ± 0.03a |
Oil holding capacity (g g−1) | 1.50 ± 0.03b | 5.11 ± 0.05a |
Emulsion capacity (mg mL−1) | 28.1 ± 0.62b | 51.4 ± 0.43a |
Emulsion stability (%) | 22.2 ± 0.60b | 42.0 ± 0.86a |
Foaming capacity (%) | 70.4 ± 0.30b | 94.3 ± 0.32a |
Foaming stability (%) | 82.7 ± 0.43b | 93.2 ± 0.21a |
Protein solubility (%) | 89.3 ± 0.71b | 97.6 ± 0.46a |
Letters a and b in superscript are showing statistical significance in row wise.
MPI, Moringa oleifera seed protein isolate; MPI-GAC, Moringa oleifera seed protein isolate-polyphenol conjugate.
Functional properties of Moringa oleifera seed protein isolate and Moringa oleifera seed protein isolate-polyphenol conjugate
Functional properties . | MPI . | MPI-GAC . |
---|---|---|
Water holding capacity (g g−1) | 0.79 ± 0.02b | 2.07 ± 0.03a |
Oil holding capacity (g g−1) | 1.50 ± 0.03b | 5.11 ± 0.05a |
Emulsion capacity (mg mL−1) | 28.1 ± 0.62b | 51.4 ± 0.43a |
Emulsion stability (%) | 22.2 ± 0.60b | 42.0 ± 0.86a |
Foaming capacity (%) | 70.4 ± 0.30b | 94.3 ± 0.32a |
Foaming stability (%) | 82.7 ± 0.43b | 93.2 ± 0.21a |
Protein solubility (%) | 89.3 ± 0.71b | 97.6 ± 0.46a |
Functional properties . | MPI . | MPI-GAC . |
---|---|---|
Water holding capacity (g g−1) | 0.79 ± 0.02b | 2.07 ± 0.03a |
Oil holding capacity (g g−1) | 1.50 ± 0.03b | 5.11 ± 0.05a |
Emulsion capacity (mg mL−1) | 28.1 ± 0.62b | 51.4 ± 0.43a |
Emulsion stability (%) | 22.2 ± 0.60b | 42.0 ± 0.86a |
Foaming capacity (%) | 70.4 ± 0.30b | 94.3 ± 0.32a |
Foaming stability (%) | 82.7 ± 0.43b | 93.2 ± 0.21a |
Protein solubility (%) | 89.3 ± 0.71b | 97.6 ± 0.46a |
Letters a and b in superscript are showing statistical significance in row wise.
MPI, Moringa oleifera seed protein isolate; MPI-GAC, Moringa oleifera seed protein isolate-polyphenol conjugate.
Water holding capacity
One of the paramount properties required in food systems for gel formation is water-holding capacity, considered as capacity of protein that hold the water. It decreases the dehydration time and increases the storage and stability of specific food by binding the water (Walayat et al., 2022). The water holding capacity of both MPI and MPI-GAC are shown in Table 1, for MPI 0.79 ± 0.02 and MPI-GAC 2.07 ± 0.03. The modified protein after conjugation with gallic acid exhibited an improved water-holding capacity than the unmodified protein. It might be due to gallic acid which changes the surface charge on protein and increases the binding sites to retain and hold the water in protein structure and the conjugation by ultrasonication that resulted in the rearrangement of protein molecule. The agglomerated structure of protein shows more hydrophilic properties due to which the capacity of protein to retain water increased; hence solubility also increased. Zhang et al. (2020) found significant increase in WHC of whey protein after the covalent conjugation of protein.
Oil holding capacity
The oil-holding property of protein refers to its capacity to retain oil in molecules by non-polar chain. Good oil-holding capacity proteins are needed in food systems, for instance, used as meat extenders. The oil holding capacity of both MPI and MPI-GAC are shown in Table 1, for MPI OHC (g g−1) 1.50 ± 0.03 and MPI-GAC 5.11 ± 0.05. The conjugated proteins show increased oil-holding capacity than unmodified protein, it could be owing to the stability of protein structure after ultrasonication treatment which decreased the surface tension between oil and protein molecules and increased oil retention in structure. Conjugation also resulted in hydrophobic pockets in the structure of protein during the rearrangement of molecules that can hold oil and improve water holding capacity of protein. The same trends were perceived by Pham et al. (2019) and Xue et al. (2020) in recent studies of conjugate formation between cyanidines and soy protein.
Emulsion capacity and stability
Protein type, structure, and polyphenol selected for conjugation affect the emulsion capacity and stability. Emulsion is also one of the paramount properties required within a food system (Djuardi et al., 2020). The result in Table 1, shows that MPI had an emulsion capacity of 28.1 ± 0.62 and MPI-GAC 51.4 ± 0.43 mg mL−1. It indicates that the emulsion capacity of conjugated protein is significantly (P < 0.05) greater as compared to unconjugated protein, which might be due to covalent grafting that exposes more hydrophilic ends and increases hydrophobicity that as a result lowers the surface tension and enhances the emulsion stability. Phenolic group attachment with protein molecules increases the hydrophobicity and creates a more favourable environment for oil-in-water emulsion. The final obtained emulsions were held in reserve at ambient temperature for 30 min to estimate the emulsion stability. However, marginal improvement was observed in the emulsion stability of the MPI-GAC 22.2 ± 0.60 as compared to MPI 42.0 ± 0.86. This improvement might be associated with an enlarged oil droplets in the water emulsion, that is due to ultra-sonication effect and hydrophobic interaction between phenol and protein create a protective barrier around oil droplets in emulsion (Omura et al., 2021). Furthermore, it has been perceived that enhanced solubility of protein leads to an optimal emulsifying capability. Moreover, enhanced emulsifying capacity of MPI-GAC could be linked to changes in aggregation, secondary structure, and solubility (Jiang et al., 2009). Analogous findings were reported in prior study conducted by Zhou et al. (2020), SPI-EGCG conjugates also showed increased emulsifying properties of the protein.
Foaming capacity and stability
In food production, foaming plays a significant role for example in the making of ice cream. Foaming properties are measured by calculating foaming capacity (FS) and foaming stability (FC) Foaming capacity is defined as foam produced by whipping of protein under controlled conditions and stability is capacity of foam to resist the collapse under storage period. Other functional properties of proteins like solubility, flexibility, and protein interactions are directly related to FC and FS (Yang et al., 2024). Table 1 shows the values of MPI-GAC were increased by 24% after conjugation with gallic acid. This modification increased the FC in MAPPC due to a variation in the secondary structure of protein (Huang et al., 2022). Compared to native or unmodified protein MPI, a marginal increase was noted in FS of MPI-GAC after conjugation with polyphenol gallic acid, which might have linked to the introduction of more hydroxyl groups in protein to enhance resistance of the foam to collapse. Pi et al. (2023) also reported similar results in soybean protein-proanthocyanidins conjugates.
Antioxidant activity
Antioxidant activity is one of the important properties of protein-polyphenol conjugates. Moringa protein-polyphenol conjugates show higher antioxidant properties than unmodified Moringa protein. Modification of protein into conjugates significantly (P < 0.05) affects the antioxidant property, due to the high antioxidant activity of gallic acid (Table 2). The higher antioxidant level of conjugates may be attributed to abundant hydroxyl groups existent in the phenolic structure. Earlier studies also proved that the binding of lactoferrin protein with gallic acid has increased the antioxidant level of the protein (Liu et al., 2019). Figure 2 shows the antioxidant value of both MPI and MPI-GAC at different sample concentrations which indicates that after conjugation antioxidant capacity of protein has been increased. At 4 mg per mL antioxidant capacity of unmodified protein, MPI was 57.0 ± 0.09 and of modified protein, MPI-GAC was 68.0 ± 0.14. With increasing concentration, a significant (P < 0.05) increase in antioxidant capacity is also observable within each group and between the groups at each concentration as well (Table 3). Various studies reported that polyphenol conjugation with proteins enhances the antioxidant activity of proteins (Yin et al., 2014; Gu et al., 2017; Fan et al., 2018; Feng et al., 2018). Liu et al. (2023) also reported related results in which hemp protein isolates conjugated with polyphenol through ultrasound exhibited higher antioxidant level than native protein and hemp protein isolates conjugated with polyphenol by alkali treatment.
Response . | Levene's test for equality of variances . | t-Test for equality of means . | |||||||
---|---|---|---|---|---|---|---|---|---|
F . | Sig. . | t . | df . | Sig. (2-tailed) . | Mean difference . | Std. error difference . | 95% Confidence interval of the difference . | ||
Lower . | Upper . | ||||||||
Equal variances assumed | 9.169 | 0.005 | −8.678 | 28 | 0.000 | −17.29000 | 1.99240 | −21.37124 | −13.20876 |
Equal variances not assumed | −8.678 | 18.632 | 0.000 | −17.29000 | 1.99240 | −21.46572 | −13.11428 |
Response . | Levene's test for equality of variances . | t-Test for equality of means . | |||||||
---|---|---|---|---|---|---|---|---|---|
F . | Sig. . | t . | df . | Sig. (2-tailed) . | Mean difference . | Std. error difference . | 95% Confidence interval of the difference . | ||
Lower . | Upper . | ||||||||
Equal variances assumed | 9.169 | 0.005 | −8.678 | 28 | 0.000 | −17.29000 | 1.99240 | −21.37124 | −13.20876 |
Equal variances not assumed | −8.678 | 18.632 | 0.000 | −17.29000 | 1.99240 | −21.46572 | −13.11428 |
Response . | Levene's test for equality of variances . | t-Test for equality of means . | |||||||
---|---|---|---|---|---|---|---|---|---|
F . | Sig. . | t . | df . | Sig. (2-tailed) . | Mean difference . | Std. error difference . | 95% Confidence interval of the difference . | ||
Lower . | Upper . | ||||||||
Equal variances assumed | 9.169 | 0.005 | −8.678 | 28 | 0.000 | −17.29000 | 1.99240 | −21.37124 | −13.20876 |
Equal variances not assumed | −8.678 | 18.632 | 0.000 | −17.29000 | 1.99240 | −21.46572 | −13.11428 |
Response . | Levene's test for equality of variances . | t-Test for equality of means . | |||||||
---|---|---|---|---|---|---|---|---|---|
F . | Sig. . | t . | df . | Sig. (2-tailed) . | Mean difference . | Std. error difference . | 95% Confidence interval of the difference . | ||
Lower . | Upper . | ||||||||
Equal variances assumed | 9.169 | 0.005 | −8.678 | 28 | 0.000 | −17.29000 | 1.99240 | −21.37124 | −13.20876 |
Equal variances not assumed | −8.678 | 18.632 | 0.000 | −17.29000 | 1.99240 | −21.46572 | −13.11428 |
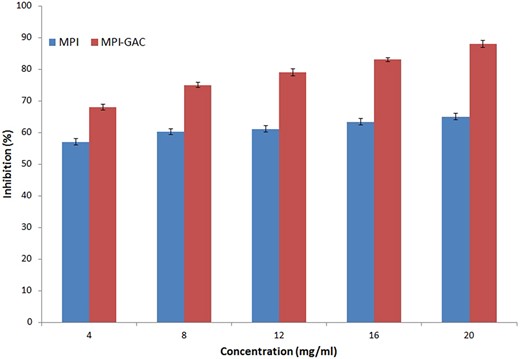
Antioxidant activity of Moringa oleifera seed protein isolate and Moringa oleifera seed protein isolate-polyphenol conjugate (MPI, Moringa seed protein isolates; MPI-GAC, Moringa seed polyphenol conjugate; Lettering (a–h) showing the statistical difference between different concentrations).
Treatment . | Source . | Sum of squares . | df . | Mean square . | F . | Sig. . |
---|---|---|---|---|---|---|
MPI (R2 = 0.931, adjR2 = 0.879) | Corrected model | 112.851 | 6 | 18.809 | 17.903 | 0.000 |
Intercept | 56475.744 | 1 | 56475.744 | 53757.240 | 0.000 | |
Concentration | 112.677 | 4 | 28.169 | 26.813 | 0.000 | |
Replicates | 0.174 | 2 | 0.087 | 0.083 | 0.921 | |
Error | 8.405 | 8 | 1.051 | |||
Total | 56597.000 | 15 | ||||
Corrected total | 121.256 | 14 | ||||
MPI-GAC (R2 = 0.985, adjR2 = 0.973) | Corrected model | 701.555 | 6 | 116.926 | 86.498 | 0.000 |
Intercept | 92787.337 | 1 | 92787.337 | 68640.857 | 0.000 | |
Concentration | 701.526 | 4 | 175.381 | 129.741 | 0.000 | |
Replicates | 0.029 | 2 | 0.015 | 0.011 | 0.989 | |
Error | 10.814 | 8 | 1.352 | |||
Total | 93499.707 | 15 | ||||
Corrected total | 712.369 | 14 |
Treatment . | Source . | Sum of squares . | df . | Mean square . | F . | Sig. . |
---|---|---|---|---|---|---|
MPI (R2 = 0.931, adjR2 = 0.879) | Corrected model | 112.851 | 6 | 18.809 | 17.903 | 0.000 |
Intercept | 56475.744 | 1 | 56475.744 | 53757.240 | 0.000 | |
Concentration | 112.677 | 4 | 28.169 | 26.813 | 0.000 | |
Replicates | 0.174 | 2 | 0.087 | 0.083 | 0.921 | |
Error | 8.405 | 8 | 1.051 | |||
Total | 56597.000 | 15 | ||||
Corrected total | 121.256 | 14 | ||||
MPI-GAC (R2 = 0.985, adjR2 = 0.973) | Corrected model | 701.555 | 6 | 116.926 | 86.498 | 0.000 |
Intercept | 92787.337 | 1 | 92787.337 | 68640.857 | 0.000 | |
Concentration | 701.526 | 4 | 175.381 | 129.741 | 0.000 | |
Replicates | 0.029 | 2 | 0.015 | 0.011 | 0.989 | |
Error | 10.814 | 8 | 1.352 | |||
Total | 93499.707 | 15 | ||||
Corrected total | 712.369 | 14 |
Treatment . | Source . | Sum of squares . | df . | Mean square . | F . | Sig. . |
---|---|---|---|---|---|---|
MPI (R2 = 0.931, adjR2 = 0.879) | Corrected model | 112.851 | 6 | 18.809 | 17.903 | 0.000 |
Intercept | 56475.744 | 1 | 56475.744 | 53757.240 | 0.000 | |
Concentration | 112.677 | 4 | 28.169 | 26.813 | 0.000 | |
Replicates | 0.174 | 2 | 0.087 | 0.083 | 0.921 | |
Error | 8.405 | 8 | 1.051 | |||
Total | 56597.000 | 15 | ||||
Corrected total | 121.256 | 14 | ||||
MPI-GAC (R2 = 0.985, adjR2 = 0.973) | Corrected model | 701.555 | 6 | 116.926 | 86.498 | 0.000 |
Intercept | 92787.337 | 1 | 92787.337 | 68640.857 | 0.000 | |
Concentration | 701.526 | 4 | 175.381 | 129.741 | 0.000 | |
Replicates | 0.029 | 2 | 0.015 | 0.011 | 0.989 | |
Error | 10.814 | 8 | 1.352 | |||
Total | 93499.707 | 15 | ||||
Corrected total | 712.369 | 14 |
Treatment . | Source . | Sum of squares . | df . | Mean square . | F . | Sig. . |
---|---|---|---|---|---|---|
MPI (R2 = 0.931, adjR2 = 0.879) | Corrected model | 112.851 | 6 | 18.809 | 17.903 | 0.000 |
Intercept | 56475.744 | 1 | 56475.744 | 53757.240 | 0.000 | |
Concentration | 112.677 | 4 | 28.169 | 26.813 | 0.000 | |
Replicates | 0.174 | 2 | 0.087 | 0.083 | 0.921 | |
Error | 8.405 | 8 | 1.051 | |||
Total | 56597.000 | 15 | ||||
Corrected total | 121.256 | 14 | ||||
MPI-GAC (R2 = 0.985, adjR2 = 0.973) | Corrected model | 701.555 | 6 | 116.926 | 86.498 | 0.000 |
Intercept | 92787.337 | 1 | 92787.337 | 68640.857 | 0.000 | |
Concentration | 701.526 | 4 | 175.381 | 129.741 | 0.000 | |
Replicates | 0.029 | 2 | 0.015 | 0.011 | 0.989 | |
Error | 10.814 | 8 | 1.352 | |||
Total | 93499.707 | 15 | ||||
Corrected total | 712.369 | 14 |
Fourier-transform infrared spectroscopy
Fourier-transform infrared was employed to investigate secondary structure of Moringa seed protein isolates and Moringa seed protein polyphenol conjugates. The infrared spectra of proteins commonly exhibited secondary structural modifications, with stretching and bending vibrations of peptide backbones in amide I and II bands may potentially delineating diverse secondary structures (Chen et al., 2010). The amide I band (1600–1700 cm−1) is most sensitive and responsive in assessing the changes in protein secondary structural analysis (Yang et al., 2014). The regions and positions of distinctive absorption peaks of MPI-GAC differed from those observed in native MPI. As shown in Fig. 3, the spectrum of pure Moringa seed protein isolate exhibited band at 3500–3000 cm−1. Moringa seed protein polyphenol conjugates displayed the band towards the shorter wavelength around 3300 due to the absorption of water after the protein and polyphenol conjugates formation owing to –OH stretching vibration of phenolic group (Jia et al., 2016). This implies that there is a potential interaction between MPI and polyphenol through C–N covalent bonds formation. Furthermore, spectrum of MPI-GAC showed the vibration peak corresponding to the N–H bending of primary amine at 1647 cm−1 and –CH bending at 1432 cm−1, whereas MPI exhibited the vibration peak at 1599 cm−1, which linked to the bending vibration of amino (N–H) (Li et al., 2023). The absorption peaks noted at 1641 and 1431 cm−1 were attributed to the C–O stretching of the amide I band and the symmetrical stretching of COO−, respectively. It was noticed that after protein and polyphenol conjugation, there was stretching of peaks 1400–1500 cm−1, which indicated after polyphenol attachment the secondary structure of protein did not destroy. The new wave peaks in the MPI-GAC confirmed the introduction of phenolic hydroxyl groups through covalent bonds using certain groups in MPI. The findings supported the functional and microstructure results as mentioned in the above section. A similar type of pattern was seen in the secondary structure of whey protein isolate conjugated with different polyphenols (Liu et al., 2021).
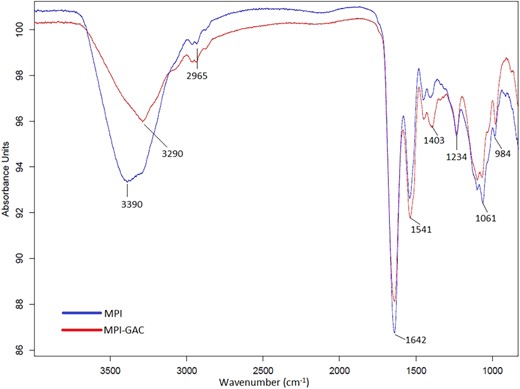
Fourier-transform infrared (FT-IR) spectra of Moringa oleifera seed protein isolate (MPI) and Moringa oleifera seed protein isolate-polyphenol conjugate (MPI-GAC).
Scanning electron microscopy
The surface morphology of MPI and MPI-GAC were characterised by scanning electron microscopy at 5, 10 and 30 K magnification. Figure 4 shows the morphological structure of the native Moringa protein isolate, at 5000 magnifications it shows a smooth structure and for MPI-GAC it shows a spherical or nearly spherical structure with a smaller particle size. There is a visible alteration of the structure before and after conjugation with polyphenol. Compared with native Moringa protein isolate, Moringa protein-polyphenol conjugates exhibited the particles with consistent and smaller sizes, which suggest that covalently grafted polyphenols may have changed the range and strength of attractive and repulsive interactions between the protein and polyphenol molecules and higher content of hydroxyl group in gallic acid, which make protein polyphenol interaction easier (Liu et al., 2021). The smaller particle size is due to the cavitation effect of ultrasound and the linkage between phenols and proteins. In MPI-GAC the structure is like agglomerates which can be explained by the fact that the reaction of protein and polyphenols and covalent and non-covalent grafting between molecules and rearrangement of molecules resulted in this structure which added in increased solubility of protein. Similar results were obtained by scanning electron microscopy of the soy protein isolate-EGCG covalent complexes (Zhou et al., 2020).
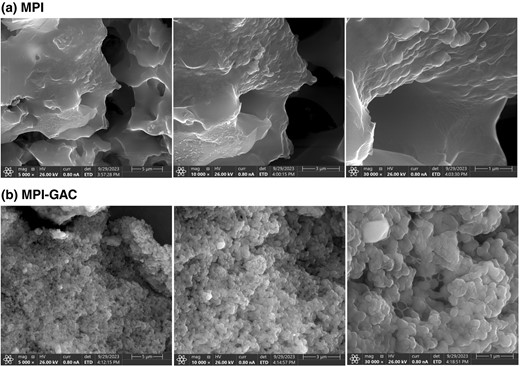
The scanning electron micrographs (SEM) of Moringa oleifera seed protein isolate (MPI) and Moringa oleifera seed protein isolate-polyphenol conjugate (MPI-GAC).
Conclusion
Sonication induced conjugation of Moringa protein isolate with gallic acid improved the functional properties of the final conjugated product. The conjugated MPI had higher solubility, foaming and emulsifying properties, and water and oil holding capacities due to protein in the isolate that was unfold, higher charge density, and particle size reduction. The change in conformation and reduced particle size were evident from FT-IR spectra and SEM images. The SEM images were also showing the linkage of phenolic compounds with protein isolates. This linkage also increased the antioxidant capacity of protein isolates. These results suggest that ultrasound technology can be used to improve the functional properties proteins.
Acknowledgments
The authors thank the Higher Education Commission, Pakistan (HEC) for financial support and Washington State University, USA, for providing research facilities under International Research Support Initiative Program.
Author contributions
Aliza Zulfiqar: Methodology; formal analysis; writing – original draft. Muhammad Imran: Software; validation. Muhammad Haseeb Ahmed: Methodology. Shyam S. Sablani: Conceptualization; writing – review and editing. Muhammad Kamran Khan: Supervision; conceptualization; writing – review and editing.
Conflict of interest
The authors declare no conflicts of interest.
Ethical approval
Ethics approval was not required for this research.
Data availability statement
All the data related to this article is available in this manuscript.
References
The paper provides a detailed analysis of both the amino acid profile and the antioxidant properties, offering a holistic view of the Moringa oleifera seed protein. This comprehensive approach supports pressent research in understanding the composition and antioxidant properties of Moringa oleifera seed protein.
The study by Jain et al. (2019) examines the preparation, characterisation, and functional properties of Moringa oleifera seed protein isolate, which is directly related to current research on the properties and applications of Moringa oleifera seed proteins. This reference provides an in-depth analysis of the physicochemical and functional properties of the protein isolate. Understanding these properties is essential for my study as it helps in evaluating the potential use of Moringa oleifera proteins in various food applications.
This reference provides a thorough review of the current state of research on phenolic–protein conjugates. It covers various aspects such as the methods of synthesis, detailed characterisation techniques, and the resulting biological activities. This comprehensive overview is valuable for my research. It discusses innovative approaches to enhancing the properties of proteins through phenolic conjugation. These insights are crucial for current study, which aims to explore novel methods for improving the functional properties of plant proteins for food applications.
This research investigates the improved functionality of soybean protein-proanthocyanidins conjugates prepared by alkali treatment. This reference provides an in-depth analysis of the functional improvements achieved through the conjugation process. The detailed characterisation of these improvements, such as enhanced solubility, emulsifying properties, and stability, is critical for current study as it helps in understanding how modifications can optimize the use of plant proteins in food applications.
This study investigates the physicochemical properties of soy protein isolate-cyanidin-3-galactoside conjugates produced using free radicals induced by ultrasound. This research is directly relevant to present research, the use of ultrasound to induce free radicals for the conjugation process is an innovative method that can potentially improve the functionality of plant proteins.