-
PDF
- Split View
-
Views
-
Cite
Cite
Ting Tang, Yifan Shi, Siran Yang, Jikeyi Li, Jiaying Chen, Xiaoqin Wu, Guangliang Xing, A comparative study on the physicochemical characteristics and protein digestibility of tofu induced by magnesium chloride or combined with transglutaminase, International Journal of Food Science and Technology, Volume 58, Issue 9, September 2023, Pages 4666–4674, https://doi.org/10.1111/ijfs.16571
- Share Icon Share
Abstract
In this study, tofu was prepared through the addition of transglutaminase (TGase) for pre-crosslinking at varying durations (1, 2 and 3 h), followed by the addition of magnesium chloride (MgCl2) to soymilk. The physicochemical properties and protein digestibility were analysed. Results indicated that, with the prolongation of TGase pre-crosslinking time, the zeta-potential absolute value and the average particle size of soymilk increased gradually. In contrast, the pH value remained unchanged (P > 0.05), suggesting that the colloidal stability of soymilk improved with TGase treatment. Both the water-holding capacity (WHC) and gel strength of tofu gels exhibited a first increasing and then decreasing trend with prolonged pre-crosslinking time. Notably, the highest WHC and hardness values of tofu gels prepared by adding MgCl2 after pre-crosslinking with TGase for 1 h were 96.5 ± 0.5% and 363.9 ± 28.3 g, respectively. Subsequently, in vitro simulated gastrointestinal digestion of tofu samples prepared under this condition was conducted to determine changes in soluble protein content, small peptide content and protein hydrolysis spectrum. Gastric and intestinal digestion phases were the primary stages of protein hydrolysis, and the bioavailability of proteins in TGase+MgCl2-induced tofu was higher than in MgCl2-coagulated tofu. Therefore, tofu prepared through TGase pre-treatment was more conducive to soybean protein digestion and absorption.
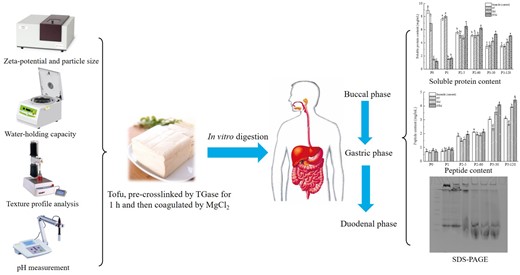
Introduction
Soybean (Glycine max) is one of the essential crops in China, which contains about 30%–40% (w/w) protein and is a good source of various nutrients in the daily diet. As a standard soybean product, tofu has been widely accepted and highly regarded among the Chinese people and enjoys the reputation of ‘plant-based meat.’ The quality and yield of tofu are significantly influenced by the type of coagulants employed. In general, coagulants are classified into three categories: salt coagulants (e.g., gypsum and magnesium chloride [MgCl2]), acidic coagulants (e.g., glucono-δ-lactone [GDL]), and enzymatic coagulants (e.g., transglutaminase [TGase]) (Zheng et al., 2020).
However, tofu produced by a single coagulant may not meet the desired quality standards, a mix of two or more coagulants can be added to achieve the expected texture (Mefleh et al., 2022). For instance, MgCl2-induced tofu exhibits high stiffness but with a coarse texture and poor water-holding capacity (WHC). This is primarily due to the rapid reaction between MgCl2 and soy proteins, which causes quick shrivelling and dehydration of protein web structures, resulting in the rough texture of the final tofu product (Zhu et al., 2016). Using only TGase as a coagulant to prepare tofu resulted in low-gel strength (Gao et al., 2021). In an earlier study, Zhu et al. (2019) found that the addition of TGase to brine tofu and GDL tofu not only improved their springiness, chewiness and microstructure in comparison to single coagulant tofu without TGase, but also resulted in a higher yield and a more uniform structure. Hence, compound coagulants, created through the artificial processing of two or more components, have become a promising research direction in response to the pressing need for improved tofu quality and modern production requirements.
Currently, research on the use of coagulants in tofu production mainly focuses on the principles of gel formation and manufacturing processes (Hsieh et al., 2012; Shi et al., 2020), with little attention paid to the impact of different types of coagulants on the digestion and absorption of protein in tofu. A previous study has shown that the type of coagulant affects not only the external quality of soybean protein gel but also its intrinsic protein bioavailability (Rui et al., 2016). Therefore, in this study, TGase combined with MgCl2 was used as a compound coagulant to produce tofu. An analysis of the physicochemical properties of pH value, water retention and gel strength were done to assess the quality of the resultant tofu. In addition, an in vitro simulated human gastrointestinal digestion model was used to explore the differences in soy protein digestibility among tofu produced by using MgCl2 only, TGase solely and their combination. The analysis was performed via measurements of the peptide content, the amount of soluble protein and electrophoresis. By comparing and analysing the results of the physicochemical and nutritional indices, we aimed to determine the optimal processing parameters to improve tofu quality and promote protein digestion and absorption, providing scientific guidance for tofu production.
Materials and methods
Materials
TGase (enzyme activity: 100 U g−1) was provided by the Yiming Biological Technology Co., Ltd., Jiangsu. MgCl2 was obtained from Hubei Angel Yeast Co., Ltd. α-amylase (G8290), pancreatin powder (P7340), pepsin (P8390) and porcine bile salts (G8310) were purchased from Solarbio Science & Technology Co., Ltd., Beijing. Soybeans were bought from supermarkets. Unless otherwise specified, all other chemicals were analytical grade.
Preparation of tofu curd
After selection, the soybeans were soaked in distilled water overnight using a 1:3 (m/v) ratio at 25 °C. The soaked soybeans were drained, and the water absorption mass of soybeans was calculated. Then, an appropriate amount of water based on a dry soybean to water ratio of 1:8 (m/v) was added and ground by a high-speed blender. The mixture underwent filtration using a 120-mesh cheesecloth to eliminate okara, and the resulting filtrate was subjected to boiling for 5 min. Utilising the Kjeldahl method, the protein content in the cooked soymilk was determined to be 3.6 g/100 mL. After cooling down to 50 °C, TGase (3.0 U g−1 protein) was added, and the mixture was divided into three equal parts, which were separately incubated at 50 °C for different durations (1, 2, 3 h). Soymilk without adding TGase was set as the blank control. Then, MgCl2 was added to the blank control and pre-crosslinked soymilk to a final concentration of 30 mM. The samples were then kept in an 85 °C water bath for 30 min. The resulting curd was relocated to a cheesecloth that was positioned in a mould of 14 cm (length) × 10 cm (width) × 9 cm (height). To eliminate whey from the curd to obtain tofu, it was compressed at a force of 20 g cm−2 for 30 min. Three replicates were performed for the tofu production.
Determination of zeta-potential and particle size
After being cross-linked by TGase for different durations, the soymilk samples were diluted with distilled water to achieve a protein concentration of 1.0 mg mL−1. According to Xing et al. (2019), the diluted samples' particle size and zeta-potential were assessed. To promote accuracy, all the tests were carried out three times.
pH value determination
The pH values of the soymilk and the final tofu samples were measured using an FE20 laboratory pH meter (Shanghai Mettler-Toledo Instrument Co., Ltd.). All assays are repeated in triplicates.
Gel properties determination
The high-speed centrifugation method specified by Peng & Guo (2015) was employed to determine the WHC of tofu gels that crosslinked with TGase for varying durations and coagulated through the addition of MgCl2. The tofu gel was cut into cylindrical shapes (diameter: 1.5 cm × height: 3.0 cm) and centrifuged for about 10 min at 480 × g. The ratio of the post-centrifugation weight of the tofu to its pre-centrifugation weight was used to derive the WHC. The gel strength of tofu was evaluated through texture profile analysis (TPA), following the methodology of an earlier study (Rui et al., 2016). Triplicate determinations were performed.
In vitro simulated gastrointestinal digestion
The samples subjected to in vitro simulated gastrointestinal digestion were classified into four groups: (1) Soymilk (the control): soymilk without the addition of TGase and MgCl2; (2) ST: soymilk with 1 h of pre-crosslinking using TGase; (3) SM: tofu gel prepared solely by adding MgCl2 and (4) STM: tofu gel prepared by pre-crosslinking with TGase for 1 h followed by adding MgCl2. The in vitro simulated gastrointestinal digestion model established by Hui & Xing (2022) was used for the experiment, which includes the oral digestion stage (P1), gastric digestion stage (P2) and intestinal digestion stage (P3). Samples were collected at the following time points: prior to digestion (numbered P0), after oral digestion (numbered P1), after 5 and 60 min of gastric digestion (numbered P2–5 and P2–60), after 30 and 120 min of duodenal digestion (numbered P3–30 and P3–120).
Soluble protein content determination
Soluble protein content in digested samples was determined by the bicinchoninic acid (BCA) method according to Li et al. (2013). A standard curve was produced in accordance with the BCA assay kit (PC0020, Solarbio, Beijing, China) procedure. The supernatant of samples from various digestion stages was diluted 40 times after being centrifuged (10 000 r min−1, 5 min), and then added into a 96-well plate. After adding BCA working solution to each well, the plate was incubated at 37 °C for approximately 30 min. The absorbance was detected at 562 nm with a microplate reader (ReadMax 1900, Shanghai Shanpu Biotechnology Co. LTD., China), and the protein concentration of each well was calculated based on the standard curve. Triplicate determinations were performed.
Determination of peptide content
Two millilitres of digested samples from each digestion stage were transferred to a Millipore ultrafiltration tube with a molecular weight cutoff of 10 kDa. The tube was then centrifuged at 5000 r min−1 for 5 min, and the resulting protein permeate was collected. Fifty microliters of the permeate were then added to 2 mL of the reaction mixture and incubated for exactly 2 min at room temperature, followed by measuring at 340 nm (Perez-Miguez et al., 2019). To prepare the reaction mixture, 25 mL of 100 mm borate buffer, 2.5 mL of 20% (w/w) SDS, 40 mg of OPA (dissolved in 1 mL methanol) and 100 μL of β-mercaptoethanol were combined, then the mixture was adjusted to 50 mL with distilled water. Casein tryptone was used as a standard. All the analyses were performed in triplicate.
Electrophoresis
Sodium dodecyl sulfate polyacrylamide gel electrophoresis (SDS-PAGE) was used to detect changes in protein molecular weight of soymilk treated by TGase alone and in combination with MgCl2 for different time. Also including the digested samples at different stages, as mentioned above section 2.6. Twenty microliters of each sample were mixed with 5 μL of loading buffer (BL502A, Biosharp, China), and then boiled at 100 °C for 5 min, and 10 μL of the resulting solution was used for loading. Discontinuous vertical slab gel electrophoresis was performed using 12% separation gel and 4% stacking gel.
Statistical analysis
SPSS 16.0 version (SPSS Inc., Chicago, IL, USA) was employed to conduct one-way analysis of variance and the Duncan's multiple comparison tests in order to identify significant differences among the means (P < 0.05). Graphs were created using Origin 9.0 software (OriginLab Corporation, Northampton, MA, USA).
Results and discussion
Particle average size and zeta-potential analysis
Table 1 presents the particle average size and zeta-potential measurements for soymilk samples with addition of TGase after being incubated for various times (1, 2 and 3 h). As depicted in Table 1, all samples exhibited zeta-potential values greater than 20 mV in absolute terms, indicating their colloidal stability (Schmitt et al., 2007; Zhang et al., 2021a), and the absolute value increased as time extended. This suggests that TGase pre-crosslinking treatment resulted in an improved colloidal stability for the soymilk samples. It has been proposed that multiple aspects, such as protein type, salt concentrations, pH and temperature, can impact the zeta-potential of proteins (Ryan et al., 2012). Particle size measurement is a useful tool for monitoring and assessing the degree of protein aggregation. Additionally, it was observed that particle average size distribution of soymilk increased gradually with prolonged pre-crosslinking time under the catalytic influence of TGase, which indicated that the cross-linking reaction occurred. TGase possesses the ability to facilitate the formation of covalent isopeptide bonds between lysyl side chains and glutaminyl residues of soy protein, culminating in ε-(γ-glutamyl) lysine covalent isopeptide bond creation (Gaspar & de Góes-Favoni, 2015). For instance, even after merely 1 h of pre-crosslinking, particle average size exhibited a significant increase (P < 0.05) compared to soymilk devoid of TGase addition (the control, 362.9 ± 3.2 nm), increasing specifically to 474.4 ± 26.1 nm. The result corroborated with another study, which discovered that the mean diameter of TGase-treated soy protein isolates gradually increased over time (Liu et al., 2021).
Zeta-potential and particle average size of soymilk samples incubated with TGase for different time points
Time . | Zeta-potential (mV) . | Average Size (d nm−1) . |
---|---|---|
Control | −26.5 ± 0.7a | 362.9 ± 3.2c |
1 h | −35.3 ± 1.6b | 474.4 ± 26.1b |
2 h | −37.7 ± 1.2bc | 516.3 ± 55.5b |
3 h | −39.8 ± 3.1c | 581.8 ± 12.0a |
Time . | Zeta-potential (mV) . | Average Size (d nm−1) . |
---|---|---|
Control | −26.5 ± 0.7a | 362.9 ± 3.2c |
1 h | −35.3 ± 1.6b | 474.4 ± 26.1b |
2 h | −37.7 ± 1.2bc | 516.3 ± 55.5b |
3 h | −39.8 ± 3.1c | 581.8 ± 12.0a |
Note: Lowercase letters (a–c) that differ within the same column indicate statistically significant dissimilarities (P < 0.05).
Zeta-potential and particle average size of soymilk samples incubated with TGase for different time points
Time . | Zeta-potential (mV) . | Average Size (d nm−1) . |
---|---|---|
Control | −26.5 ± 0.7a | 362.9 ± 3.2c |
1 h | −35.3 ± 1.6b | 474.4 ± 26.1b |
2 h | −37.7 ± 1.2bc | 516.3 ± 55.5b |
3 h | −39.8 ± 3.1c | 581.8 ± 12.0a |
Time . | Zeta-potential (mV) . | Average Size (d nm−1) . |
---|---|---|
Control | −26.5 ± 0.7a | 362.9 ± 3.2c |
1 h | −35.3 ± 1.6b | 474.4 ± 26.1b |
2 h | −37.7 ± 1.2bc | 516.3 ± 55.5b |
3 h | −39.8 ± 3.1c | 581.8 ± 12.0a |
Note: Lowercase letters (a–c) that differ within the same column indicate statistically significant dissimilarities (P < 0.05).
Electrophoresis of soymilk samples with different treatment
The electrophoresis of soymilk treated by TGase alone and in combination with MgCl2 for varying durations can be observed in Fig. 1. In the soymilk sample without adding TGase (lane 1), the major soy protein bands, 7S (β-conglycinin) and 11S (glycinin) were visible. The 7S protein (150–200 kDa) is a trimeric glycoprotein consisting of three subunits, i.e., α, α′ and β. Comprising acidic subunits (ASs) and basic subunits (BSs) linked by disulfide bonds, the 11S protein (~360 kDa) is a non-glycosylated, hexameric protein (Zheng et al., 2020). After pre-treatment with TGase for 1 h (lane 2), most protein bands disappeared, leaving only three weak bands visible. Based on the subunit distribution in the control, these were predicted to be 7S β subunit (band 1), 11S AS (band 2) and 11S BS (band 3). A similar phenomenon was observed in the sample pre-crosslinked with TGase for 1 h and then added MgCl2 (lane 5). As a result, cross-linked polymers emerged with a molecular weight exceeding 130 kDa, which gradually increased at the intersection of the stacking gel and the resolving gel. Additionally, owing to their significant size, it was difficult for certain high-molecular-weight polymers to enter the stacking gel. So, many proteins piled up at the base of the loading well. These findings indicated that TGase could promote intermolecular covalent bond formation, creating substantial aggregates from the subunits of 7S and 11S (Gaspar & de Góes-Favoni, 2015). With increasing cross-linking time, almost all bands disappeared except for the faint 11S BS in lane 4 and lane 7. This is consistent with numerous previous reports (Ojukwu et al., 2020; Xing et al., 2020; Zhang et al., 2021b), which found that 11S BSs are not easily cross-linked by TGase, possibly due to their location within the 11S globulin and lower content of glutamine/lysine residues in the 11S BS.
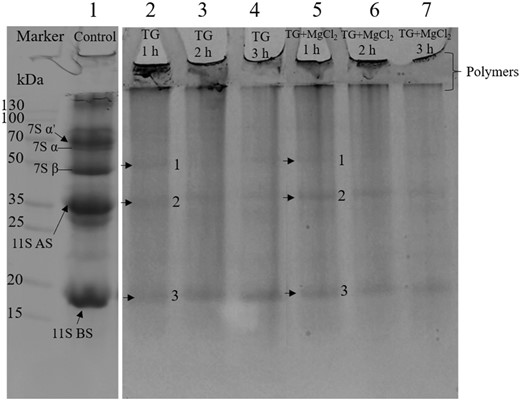
Electrophoresis of soymilk pre-incubated with TGase or combined with MgCl2.
Analysis of pH
Table S1 summarises the effects of TGase pre-crosslinking time on pH value. When only TGase was added, the pH values of soymilk with different pre-crosslinking time were not dramatically different from the blank control (P > 0.05). When soymilk was pre-crosslinked with TGase for a certain period (2 and 3 h), followed by the addition of MgCl2 and incubation at 85 °C for 30 min, the pH of tofu samples decreased significantly (P < 0.05). This is possibly due to the ionisation of MgCl2 in soymilk, releasing Mg2+ that can shield the negative charge on the protein molecule's surface, disrupt the hydration shell and double electron layer, and interact with H+ on the carboxyl group to form a magnesium bridge, reducing the electrostatic repulsion between protein molecules and enhancing hydrophobic interaction forces and hydrogen bonds, resulting in a decrease in pH (Lu et al., 2021; Yu et al., 2022).
WHC and gel strength
The quality and marketability of tofu largely depend on its texture indices, which can be demonstrated through two primary measures: gel strength and WHC (Liu et al., 2017; Wee et al., 2018). The impact of TGase pre-treatment time on these two indexes of tofu is illustrated in Fig. 2. With the increase of TGase pre-crosslinking duration, the WHC and hardness of tofu showed a trend of initial increase followed by a decrease. Tofu incubated with TGase for 1 h exhibited the highest WHC and hardness, with gel hardness increasing significantly from 160.9 ± 23.8 g (the control) to 363.9 ± 28.3 g (1 h) and WHC increasing significantly from 90.2 ± 2.3% (the control) to 96.5 ± 0.5% (1 h) (P < 0.05). The reduction in WHC is possibly caused by the formation of coarse networks and destruction of hydrophobic bonds, as stated by Luo et al. (2019), which is a result of excessive cross-linking of TGase. In other words, if the pre-crosslinking time is too long (2–3 h), the proteins in the soymilk can become overly crosslinked and result in a reduced WHC and gel strength (Yang et al., 2021). Previous research has suggested that structural integrity and strength are key factors affecting gel WHC. A uniform and robust structure is more effective in trapping water within the protein network (Wee et al., 2017).
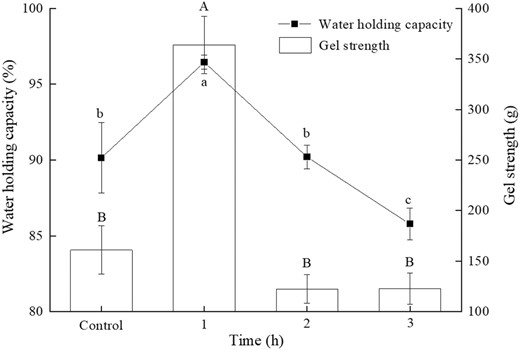
The effects of TGase pre-crosslinking time (1, 2, 3 h) on water retention and gel strength of tofu coagulated by MgCl2.
Based on the data presented in Table 1 and Fig. 1, it can be concluded that the pre-treatment of soymilk with TGase for 1 h generated strong cross-linking, resulting in an increase in the average particle size of protein aggregates. Ultimately, tofu produced from this TGase treated 1-h soymilk followed the addition of MgCl2 had a more compact protein structure, leading to enhanced gel strength and WHC. Conversely, prolonged cross-linking time (2–3 h) caused the tofu network to become rough, making it difficult to effectively retain moisture within the gel matrix, resulting in substantial reductions in the hardness and WHC. This could be attributed to the fact that the TGase catalytic reaction became saturated, as the optimal number of covalent bonds required to maintain the stability of the protein gel network has a limit (Chen et al., 2023). Excessive protein cross-linking resulted in large aggregated clusters that were disadvantageous for the further action of MgCl2 coagulant, leading to uneven final gel structure. Luo et al. (2019) also drew similar conclusions, reporting that excessive cross-linking of soy protein isolate with TGase resulted in the formation of excessively large polymers or aggregates, impeding the formation of an effective protein network structure. Therefore, it is more appropriate to choose an enzyme pre-reaction time of around 1 h.
Soluble protein content
Determining the amount of soluble protein present in the supernatant of a digestive mixture is crucial for assessing the bioavailability of proteins (Rui et al., 2019). During gastrointestinal digestion, the changes of soluble protein content in different samples are shown in Fig. 3. Before in vitro digestion (P0 phase), the soluble protein content in soymilk (control) was the highest (8.9 mg mL−1), followed by ST (6.9 mg mL−1), and SM and STM had the lowest soluble protein content (around 1.5 mg mL−1), mainly due to different gelation mechanisms of TGase and MgCl2. The formation of bridges between the negatively charged groups (-COO-) of proteins through the binding or cross-linking action of divalent cations (Mg2+) leads to water entrapment within the gel. The major driving forces behind this process are hydrophobic interactions and ionic binding that occurs due to the addition of MgCl2 (Marinea et al., 2021). In contrast, as a coagulant, the negative charges on the surface of soy protein cannot be neutralised by TGase, but instead of being cross-linked with each other via catalysis to form covalent bonds (Rui et al., 2016). Therefore, ST sample kept in liquid state, and most of the proteins were still soluble. After oral digestion (P1), the soluble protein content in SM and STM gels remained significantly lower than the control and ST (P < 0.05), indicating that the simulated chewing action and α-amylase in saliva had almost no effect on the soluble protein content.

Soluble protein contents in different samples during in vitro gastrointestinal simulated digestion. Statistically significant differences (P < 0.05) are denoted by lowercase letters that vary within the same digestive phase.
Compared with the P0 and P1 stages, during the gastric digestion period (P2), both the control and ST exhibited a decrease trend in soluble protein content with no significant difference (P > 0.05). However, the soluble protein content in SM and STM increased considerably, especially after 5 min of gastric digestion. The soluble protein content in SM significantly increased from 1.53 mg mL−1 in the P1 stage to 4.73 mg mL−1, while that in STM increased dramatically from 1.69 mg mL−1 in the P1 stage to 6.54 mg mL−1, and there was a significant difference between the two (P < 0.05). In the subsequent intestinal digestion stage (P3), the soluble protein content in all samples showed a decreasing trend compared to that in the P2 stage. This may be because proteins are further hydrolyzed into smaller molecular weight peptides under the combined action of pepsin, pancreatin and bile salts, making them undetected by the BCA method, leading to a decreasing trend in experimental results. However, it is worth noting that at different time points during gastrointestinal digestion, the soluble protein content measured in STM was dramatically higher than in SM (P < 0.05). In our previous research, it was found that using lactic acid bacteria in conjunction with TGase as coagulant to treat mixed soy and cow milk for 5 h results in a lower release of soluble protein compared to using only lactic acid bacteria fermentation (Xing et al., 2016). We speculate that the type of coagulant, TGase cross-linking time, and TGase dosage may all affect the protein digestion process. Proteins that persist in soluble form in duodenal environment are more likely to be further absorbed, while those remain in the precipitate might imply the existence of large protein aggregates that could be too big to pass through the intestinal epithelium (Rahaman et al., 2016). Therefore, the use of TGase and MgCl2 as a composite coagulant for tofu production can promote the hydrolysis and release of soybean protein during gastrointestinal digestion, which is more conducive to be absorbed.
Bioaccessible peptides
Figure 4 presents the results of small peptide content below 10 kDa in four groups of samples during different digestion stages. Throughout the digestion process, the small peptide content of all samples showed an increasing trend. The content of small peptides in all samples was very low (less than 1.0 mg mL−1) at P0 and P1 digestion stages, indicating that the hydrolysis of soy protein was minimally impacted by chewing and salivary amylase, and most of soy proteins still existed in the form of macromolecules in the samples. As gastrointestinal digestion progressed, the small peptide content in the samples gradually increased. In the gastric digestion phase (P2), the small peptide content of the control and STM was significantly higher than that of ST and SM (P < 0.05). In the intestinal digestion stage (P3), the small peptide content of all four groups of samples was significantly higher than that in the P2 stage, mainly due to the joint action of digestive enzymes in gastric and intestinal juice, breaking down protein into small peptides. Among them, the small peptide content of tofu gel made by STM was the highest, reaching 4.44 mg mL−1 at the end of intestinal digestion (P3–120), which was observably higher than that of the other three groups of samples (P < 0.05). This further indicates that tofu cross-linked by TGase and MgCl2 is more likely to release small active peptides and is more suitable for digestion and absorption by the human body. This result is consistent with our previous study that the probability of bioactive peptide release was more remarkable in tofu prepared by first treating with TGase for 2 h, followed by the addition of glucono-δ-lactone, than in soymilk (Hui & Xing, 2022). Different factors can modify protein microstructures, leading to changes in their enzymatic susceptibility and influencing the release of peptides from the food matrix (Bhat et al., 2021).
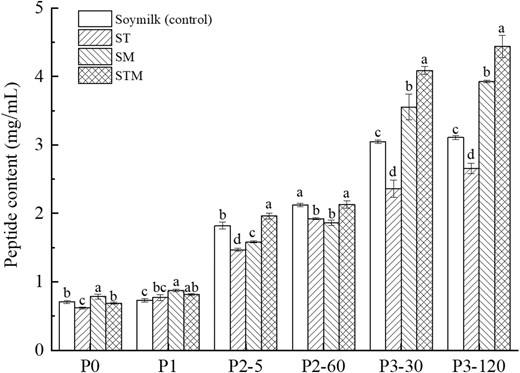
Peptide contents in different samples during in vitro gastrointestinal simulated digestion. Statistically significant differences (P < 0.05) are denoted by lowercase letters that vary within the same digestive phase.
Electrophoresis of the digesta
The protein degradation at different digestion stages of each sample was analysed by SDS–PAGE, and the results are shown in Fig. S1. The major components of soy protein were visible in the P0 and P1 lanes of the soymilk samples. However, only one major band (11S BS) was observed in the ST sample. The disappearance of the 7S and 11S AS bands in the ST sample indicates that protein aggregation occurred under TGase action, and the concentration of high-molecular-weight protein aggregates (>130 kDa) at the top of the concentrated gel also confirmed this. Hsieh et al. (2014) obtained similar results indicating that 7S α′, 7S α, 7S β and 11S ASs were suitable substrates for TGase, whereas 11S BSs were less susceptible to TGase crosslinking. The bands visible in the SM and STM samples were fewer in number and had a low-density value in the P0 and P1 stages. This may be due to most proteins being trapped in the tofu gel, which is in line with the lower soluble protein content during the early stage of digestion, as shown in Fig. 3. As gastric digestion proceeded under the action of pepsin, the bands corresponding to the 7S and 11S proteins in all samples almost disappeared, and some fuzzy low-molecular-weight protein bands could only be observed in the range of 20–35 kDa. In ST and STM samples, there were no intact bands throughout the entire gastrointestinal digestion, indicating that digestive enzymes hydrolyzed most high-molecular-weight protein aggregates into peptides and amino acids.
Conclusion
In this study, TGase combined with MgCl2 was used as a compound coagulant to produce tofu. Results showed that the hardness and WHC of tofu significantly increased (P < 0.05) when soymilk was pre-treated with TGase for 1 h. However, when the crosslinking time exceeded 1 h, the gel strength and WHC of tofu decreased observably (P < 0.05). By simulating gastric and intestinal digestion under this condition, it was found that the protein bioaccessibility of STM gel was higher than that of SM gel, indicating that tofu prepared by adding TGase pre-treatment is more conducive to the digestion and absorption of soy proteins. These results help to better understand the effect of TGase pre-crosslinking on soy protein bioaccessibility and provide fundamental knowledge for the compound coagulant (TGase combined with MgCl2) in the tofu industry to enhance its nutritional value.
Acknowledgments
The “Golden Seed” Program for Innovation and Entrepreneurship of Changshu Institute of Technology (grant number JXJ202222) provided financial support for this study. This research was also supported by the college students’ innovation training program project of Changshu Institute of Technology in 2023 and the Research Start-up Fund of Changshu Institute of Technology (grant number KYZ2019036Q).
Author contributions
Ting Tang: Data curation (equal); formal analysis (equal); investigation (equal); methodology (equal); writing – original draft (equal). Yifan Shi: Data curation (equal); formal analysis (equal); investigation (equal); software (equal); writing – original draft (equal). Siran Yang: Data curation (equal); formal analysis (equal); investigation (equal). Jikeyi Li: Data curation (equal); formal analysis (equal); methodology (equal). Jiaying Chen: Methodology (equal); software (equal). Xiaoqin Wu: Formal analysis (equal); resources (equal); software (equal); supervision (equal). Guangliang Xing: Conceptualization (equal); data curation (equal); formal analysis (equal); methodology (equal); software (equal); supervision (equal); writing – original draft (equal); writing – review and editing (equal).
Conflict of interest
The authors declare that there are no conflicts of interest.
Peer review
The peer review history for this article is available at https://www.webofscience.com/api/gateway/wos/peer-review/10.1111/ijfs.16571.
Data availability statement
The data that support the findings of this study are available from the corresponding author upon reasonable request.
References
Author notes
Yifan Shi is the co-first author.