-
PDF
- Split View
-
Views
-
Cite
Cite
Yanyan Chen, Xuqin Li, Fei Xu, Qing Yu, Qingqing Zhang, Effects of inulin with different polymerisation degrees on dough rheology and water distribution in the fresh noodles, International Journal of Food Science and Technology, Volume 58, Issue 10, October 2023, Pages 5418–5429, https://doi.org/10.1111/ijfs.16660
- Share Icon Share
Abstract
Over the past few years, the growing market demand for functional food leads to the development of inulin-containing staple food. Comparing to previous works, this study investigates the effect of inulin with different polymerisation degrees on dough rheology and water distribution in the fresh noodles. Rheological properties of gluten, either using empirical or fundamental methods illustrate the distinctly improved farinograph properties. The tensile properties of the noodles added with LCI increased. Both SCI and LCI reduced the gelatinisation temperature and viscosity of the mixed powder, inhibited the retrogradation of starch. The relaxation time of weakly bound water of fresh noodles with LCI was shortened, the proportion of tightly bound water increased by 3.33%, and the content of freezable water decreased by 1.97%. Results showed that LCI inhibits the fluidity of water and increases the toughness and stability of gluten protein network structure. Noodles with LCI were of higher quality than those in the control group and those with SCI. Both groups inhibit the fluidity of water in noodles and enhance the toughness and stability of the gluten protein network structure. These results show that comparing to SCI, LCI improve the quality of fresh noodles more effectively.
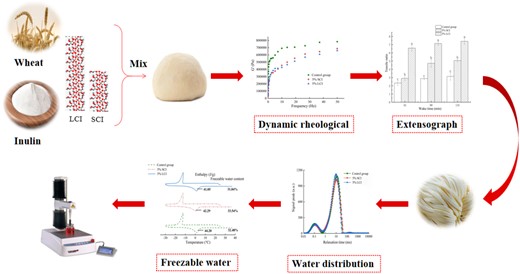
Introduction
As a functional food ingredient, inulin is widely used in steamed bread, biscuits, bread, and other flour products. In 2009, inulin was listed as a new food raw material in China, which can be added to all kinds of food except infant food (Sun & Kang, 2019). As a dietary fibre, inulin has better water solubility and the ability to form gel texture. Inulin can improve blood lipid status by reducing LDL cholesterol in the blood, thereby reducing the occurrence of cardiovascular disease and preventing obesity (Wang et al., 2019). The polymerisation degree of inulin is 2 ∼ 60, in which inulin with a degree of polymerisation ≤10 is referred to as short-chain inulin (SCI), and inulin with a degree of polymerisation ≥23 is referred to as long-chain inulin (LCI) (Luo et al., 2017b). In general, the water solubility of SCI is greater than that of LCI, while LCI has higher viscosity and gel properties.
Fresh noodles are one of the traditional staples of the Chinese diet. Quality characteristics of the dough have a crucial impact on the subsequent processing of fresh noodle products. Inulin can reduce the free water content in the product, for example, when inulin was used to make steamed bread, the hydrogen bond changed the water binding state between protein and starch.
Studies have shown that the stability of the dough increases when inulin is added, and the gluten network structure has good extensibility, high elasticity, and toughness (Rosell et al., 2010; Morris & Morris, 2012). Inulin can reduce the free water content in the product, for example, when inulin was used to prepare steamed bread, the hydrogen bond of inulin interacted with starch, which changed the water binding state between protein and starch. The presence of inulin reduces the content of free water in steamed bread and inhibits the migration of water during storage (Luo et al., 2017a). Chen & He (2014) also found that the relaxation time of noodles with 5% inulin increased after cooking, and the decrease of free water content slowed down significantly. Karolini-Skaradzinska et al. (2007) study the effect of LCI on the quality of dough made from different gluten flour, the results shows that inulin have a significant effect on flour with lower protein content than flour with higher protein content. Luo Denglin et al. (2015) explored the effect of adding SCI on plain (medium gluten) flour. The results confirmed that the kneading resistance of the dough improved and the gluten strength increased. In inulin-enriched durum wheat spaghetti, researchers also investigated the effect of polymerisation on the technological and nutritional characteristics. The results demonstrate that the inulin addition could bring prebiotic effect to the staple food without significantly affect the technological and sensorial properties (Garbetta et al., 2020).
In this paper, wheat flour without inulin was used as a control, 5% SCI or LCI were added to mixed flour. The texture, tensile properties, gelatinisation properties of mixed flour, elasticity, and viscosity of dough were determined. The texture property, cooking loss property, sensory property, internal water distribution, and freezable water content of fresh noodles with 5% SCI or LCI were determined. The effect of inulin with different degrees of polymerisation on the quality of fresh noodles was studied. It provides a new idea for the application of inulin to improve the quality of fresh noodles.
Materials and methods
Materials
The wheat flour was supplied by the company Zhengzhou Jinyuan Flour Co, LTD (Henan Province, China). The wheat flour components were analysed according to the methods of AACC (2000). It contained 13.47% moisture (AACC method 44–01), 0.34% ash (AACC method 08–12), and 9.89% protein (AACC method 39–11).
SCI and LCI (chicory inulin) were provided by Changzhou Yangsen Biotechnology Co., LTD (Jiangsu Province, China). The SCI (90.75%) proximal composition was 4.85% moisture, 0.03% ash, and 0.018% protein. The LCI (94.56%) proximal composition was 4.69% moisture, 0.04% ash, and 0.043% protein. SCI or LCI was added at 5% of wheat flour. Wheat flour without inulin was used as control. The dough for the experimental groups were prepared by mixing inulin with wheat flour and then adding distilled water.
Determination of wet gluten, dry gluten, and gluten index
Wet gluten, dry gluten, and gluten index was measured according to the method of AACC 38–12.02.
Farinograph properties
Mixing characteristics of dough were determined by a Farinograph (Brabender, Duisburg, Germany) on a 14% moisture basis according to GB/T14614-93. The flour samples (Control: wheat flour sample, SCI or LCI: 5% SCI-wheat or LCI-wheat mixed sample) were weighed and placed in the farinograph bowl, and the flour was mixed with the water from the burette. As the dough was mixed, the graph curve was recorded. A number of parameters were determined: dough development time, degree of softening, dough stability time, farinograph quality number, and water absorption of dough.
Determination of dynamic rheological properties
In the study, the dynamic rheological properties of fresh noodles were measured using Kim's method (Kim et al., 2014), with some modifications. Accurately weighed 100 g of the samples and an appropriate amount of distilled water was added according to 80% of the water absorption determined by the farinograph experiment. It is mixed with distilled water in a kneading machine and stirred for 4 min to obtain a smooth and homogeneous dough. A quantity of 5 g from the centre of the dough is taken and placed on the dynamic rheometer's test platform (DHR-15 TA, USA). Dynamic rheological properties were determined for dough by using a frequency sweep program in which the strain was 0.05%, the temperature was 25 °C, and the frequency range was 0.1 ∼ 50 Hz. The dough is placed between two layers of plates and when the spacing reaches 1 mm, the excess portions that have been squeezed out is scraped off. Before measuring, the dough is allowed to stand for 5 min to release the residual stress.
Determination of extensograph properties
With the aid of Extensograph-E (Brabender, Duisburg, Germany) for the extensibility determination of the dough samples, the AACC 54–10 and GB/T 14615–2006 method were adopted. The dough made by farinograph was divided into two parts and placed in extensograph. The finished face rod was rolled out of the shaper and falls in the centre of the bracket and was clamped with a clamp. Set the time to 45 min. The bracket should be placed on the balance arm of the stretcher. After adjusting the recording pen's zero position, the pull hook was started right away. The bracket was removed once the sample has been broken. The operation of kneading, forming, waking, and stretching with this block was repeated. The following parameters were determined: extensograph, tensile resistance, tensile ratio, and tensile energy.
Determination of gelatinisation properties
The gelatinisation properties of fresh noodles were measured using the method of Yang Saying's method (Yang, 2017) with minor modifications. Gelatinisation properties of the mixed powder were determined using a Rapid Viscosity Analyzer (RVA-4, Newport Scientific, Warriewood, Australia). The moisture content of the mixed powder was input, the mass was set to 3.5 g, and the correction was performed according to the 14% wet base. The mixed powder mass was corrected and weighed. Then, it was completely and accurately transferred into a dry and clean sample cylinder. 25 mL distilled water was added, and the sample was quickly stirred up and down with a stirrer ten times to fully disperse. The viscosity unit was expressed as cP.
Preparation of fresh noodles
A flour mixer (HM06, Jiangmen Yishang Technology Co., ltd., China) was used to mix wheat flour with 35% water and 1% salt. After the flour mixture was wrapped in plastic wrap and rested for 20 min at 25 °C, the dough was sheeted through a noodle machine (SK-1240, Solatek, Chengdu, China), adjusting the roller spacing to 2 mm thick. The sheet was then cut into fresh noodles of 3.0 mm width and 20.0 cm length.
Preparation of experimental group samples (5% SCI or LCI): Replace the corresponding proportion of wheat flour with 5% of SCI or LCI, respectively. First mixed in the dough machine for 2 min to prepare inulin-wheat mixed flour. Distilled water was added according to the amount of water added in the pre-test.
Determination of texture properties of fresh noodles
The texture properties of fresh noodles was measured referring to the method of (Luo, 2015). The texture properties of fresh noodles were measured by a texture analyser (TA-XT plus, Stable Micro System Company, UK). Twenty fresh noodles were weighed and boiled in 500 mL boiling water for 4 min, immediately taken out, quickly placed in a bowl containing cold water to cool for 1 min, and then soaked for 1 min. The noodles were covered with wet gauze to prevent water loss from affecting the test results. Each sample was tested at least six times. If the cooked noodles were placed for too long, it would harden and become sticky. Therefore, the experiment should be completed within 15 min to ensure the accuracy of the results.
Texture profile analysis
The texture profile analysis of fresh noodles were carried out according to the method of (Wang et al., 2020a). The probe of the HDP/PFS model was selected. The test mode was TPA. The calibration distance of the instrument was set to 30 mm. The speed parameters were set to 3.0 mm/s before the test, 1.0 mm/s during the test, and 1.0 mm/s after the test. The compression ratio was 75%, the time interval was 5 s, and the trigger force was 5 g. The probe was wiped clean during each measurement, and three noodles were selected to be placed flat and parallel on the stage.
Tensile resistance
The tensile resistance was measured by the method of (Xu et al., 2021) using the A/KIE test device equipped with the texture analyser. Place the prepared dough in the mould, press it firmly, remove the extruded spare parts on both sides, and press the dough into a dough strip 2 mm wide by 6 mm long, which is the sample to be tested. Placing the sample to be tested on the loading stand. The test parameters are: pre-test speed, 2.0 mm/s; test speed, 0.8 mm/s; post-test speed, 5 mm/s; tensile distance, 20.0 mm; trigger force, 5.0 g; time interval, 1 s.
Determination of cooking property of fresh noodles
Determination of water absorption
The water absorption of fresh noodles was measured according to the method of (Rombouts et al., 2014) with some modifications. Weigh about 20 g of fresh noodles (M1), and cook it in 500 mL boiling water for 4 min, remove with a spoon, and rinse with cold water for 1 min, drain for 1 min, then blot the surface with five sheets of filter paper. The mass of the noodles is recorded as M2, and the boiled noodle soup and washing water are retained. Noodle water absorption was calculated using eqn 1 under the same conditions.
where M1 is the weight of raw noodles (g) and M2 is the weight of cooked noodles.
Determination of cooking loss
Collect the boiled and rinsed water sample into a 500 mL volumetric flask and add distilled water to the mark. Accurately measure 50 mL of noodle soup with a measuring cylinder, and pour it into a 250 mL beaker with a mass of M3 that has been baked to a constant weight. After quickly evaporating most of the water in the beaker on the electric furnace, it is slowly dried to a constant weight in an oven at 105 °C. The mass of the beaker at this time was recorded as M4. The cooking loss rate was calculated using eqn 2:
where M1 is the weight of raw noodles (g), M3 is the beaker mass after constant weight (g), and M4 is the beaker after evaporation of most water (g).
Determination of moisture distribution of fresh noodles
A nuclear magnetic resonance analyser (LF-NMR, MicroMR-CL-I, Shanghai Niumai Electronic Technology Co., Ltd., Shanghai, China) was used to determine the water distribution of different samples using the method of (Bai et al., 2018) and slightly modified. About 1.0 g of noodles were accurately weighed and wrapped to prevent water loss during the test. The wrapped sample was plugged into a nuclear magnetic tube with a diameter of 1 cm with a glass rod, pressed to a scale line of 3 cm, and then put into the test tube into the test chamber. The specific parameter settings are as follows: sampling points (TD): 60000, sampling interval time (TW): 1500 ms, echo time (TE): 0.100 ms, magnification (PRG): 3, echo number (NECH): 3000, scanning times (NS): 32, sampling frequency (SW): 200 KHz.
Determination of freezable water content of fresh noodles
In this study, fresh noodles were measured with modifications to (He et al., 2019) method for measuring freezable water content. 10 mg were accurately weighed from the centre of the fresh noodles and placed in the DSC (DSC-Q20, TA company, USA). Firstly, it was balanced at 25 °C for 5 min, then cooled to −30 °C at a cooling rate of 10 °C/min and maintained for 10 min, and finally reheated to 40 °C at a rate of 10 °C/min. Record the enthalpy change ▵H of freezable water. Freezing water content is calculated according to eqn 3:
where F is freezable water content (%), ▵H is the melting enthalpy of fresh noodles (J/g), ▵H0 is the melting enthalpy of pure water freezing (335 J/g), and Wt is the water content of fresh noodles (%).
Sensory quality evaluation
Sensory quality evaluation of fresh noodles was performed to refer to the method of (Wang et al., 2020b) and (Pasqualone et al., 2019). Evaluation sessions were executed under artificial daylight conditions in isolated sensory booths (22 °C, %50 RH controlled environment) within the Sensory Evaluation Laboratory at the National Engineering Laboratory for Wheat and Corn Further Processing, Henan University of Technology, China. Sensory descriptors were entered into Compusense software (version 5.2; Guelph, ON, Canada) and assessors were asked to score each attribute. Twenty-eight trained panellists (fourteen males and fourteen females, 20–40 years old) from the School of Food Science and Technology participated in the sensory tests. Panellists were trained according to ISO standards for sensory analysis (ISO 8586:2012) and are subject to performance monitoring (ISO 11132:2012). The freshly cooked noodles were cooked to the optimum cooking time (AACC 66–50), and every panellist tasted 10 g recooked FCNs and scored on the basis of Table S1 (criteria adopted from GB/T 25005–2010). Panellists must finish the organoleptic evaluation within 20 min and rinsed their mouths with water between samples. The ethic application form (Haut015-2023) were reviewed and approved by the ethical committee of food science and technology college.
Determination of the brightness value of fresh noodles
At present, the commonly used method of colour representation is to use the CIE-ΔLΔaΔb colour space method (Xu & Chu, 2010), regarding the practice of (Jiao, 2018.) slightly modified, taking the appropriate amount of noodles, the same noodle determination site is upper, middle, and lower, do eight parallel, two repeated experiments. The L* value represents brightness, the greater the value, the brighter and whiter; +b* is the yellow direction, and −b* is the blue direction; +a* represents the reddish noodle, −a* represents the green noodle.
Statistical analysis
Experiments were repeated three times, analysed variance using SPSS19.0 software, and expressed results as mean ± standard deviation (SPSS Inc., IL, USA). Different lowercase letters indicated significant differences between samples (P < 0.05). Origin 2018 software was used to draw the figures (Origin Lab, Inc., Northampton, MA, USA).
Results and discussion
Inulin and water addition level determination
Considering the strong water absorption of inulin, the additional amount of SCI or LCI were determined to be 5% by the preliminary experiment.
The amount of water added to prepare noodles with SCI was 33%, and the amount of water added to prepare noodles with LCI was 37%.
Basic components of mixed powder
Basic composition of mixed powder measured by Near-infrared grain analyser (FOSS Infratec1241, Shanghai Zhanyi Scientific Instrument Co., Ltd. Shanghai, China). Table S2 shows that the addition of 5% SCI or LCI to the blended powder significantly reduced moisture, ash, and protein, and increased whiteness compared to the control group.
Gluten index
As shown in Table 1, wet gluten content was lower in both experimental groups compared to the control group, although there was no significant difference between the three groups.
Effect of Inulin with different polymerisation degrees on dry and wet gluten content, gluten index, farinograph properties, and gelatinisation characteristics of dough
Indicators . | Control group . | 5% SCI . | 5% LCI . |
---|---|---|---|
Wet gluten (g). | 29.63 ± 0.42a | 28.73 ± 0.49a | 29.02 ± 0.62a |
Dry gluten (g). | 10.63 ± 0.26a | 9.93 ± 0.31b | 10.09 ± 0.12b |
Gluten index | 90 ± 1.73b | 84 ± 2.08c | 93 ± 0.84b |
WA(%) | 60.05 ± 0.15a | 53.00 ± 0.31c | 54.50 ± 0.21b |
DDT (min) | 4.04 ± 0.04c | 4.46 ± 0.05b | 17.40 ± 0.14a |
DST (min) | 13.36 ± 0.16b | 10.14 ± 0.21c | 18.53 ± 0.27a |
DS (FU) | 26.00 ± 1.12b | 36.00 ± 1.06a | 27.00 ± 1.27b |
FQN | 167.67 ± 6.08b | 115.67 ± 5.64c | 283.33 ± 7.81a |
Gelatinisation temperature (°C) | 69.35 ± 0.07b | 88.48 ± 0.60a | 87.63 ± 0.53a |
Peak viscosity (cP) | 2914.50 ± 89.80a | 2358.00 ± 111.72b | 2352.00 ± 32.53b |
Trough viscosity (cP) | 2275.00 ± 141.42a | 1929.50 ± 67.18b | 1940.50 ± 26.16b |
Final viscosity (cP) | 3570.50 ± 115.26a | 3044.50 ± 106.77b | 2944.50 ± 21.92b |
Setback viscosity (cP) | 639.50 ± 51.62a | 428.50 ± 44.55b | 411.50 ± 6.36b |
Retrogradation (cP) | 1295.50 ± 26.16a | 1115.00 ± 4.24b | 1004.00 ± 39.60c |
Indicators . | Control group . | 5% SCI . | 5% LCI . |
---|---|---|---|
Wet gluten (g). | 29.63 ± 0.42a | 28.73 ± 0.49a | 29.02 ± 0.62a |
Dry gluten (g). | 10.63 ± 0.26a | 9.93 ± 0.31b | 10.09 ± 0.12b |
Gluten index | 90 ± 1.73b | 84 ± 2.08c | 93 ± 0.84b |
WA(%) | 60.05 ± 0.15a | 53.00 ± 0.31c | 54.50 ± 0.21b |
DDT (min) | 4.04 ± 0.04c | 4.46 ± 0.05b | 17.40 ± 0.14a |
DST (min) | 13.36 ± 0.16b | 10.14 ± 0.21c | 18.53 ± 0.27a |
DS (FU) | 26.00 ± 1.12b | 36.00 ± 1.06a | 27.00 ± 1.27b |
FQN | 167.67 ± 6.08b | 115.67 ± 5.64c | 283.33 ± 7.81a |
Gelatinisation temperature (°C) | 69.35 ± 0.07b | 88.48 ± 0.60a | 87.63 ± 0.53a |
Peak viscosity (cP) | 2914.50 ± 89.80a | 2358.00 ± 111.72b | 2352.00 ± 32.53b |
Trough viscosity (cP) | 2275.00 ± 141.42a | 1929.50 ± 67.18b | 1940.50 ± 26.16b |
Final viscosity (cP) | 3570.50 ± 115.26a | 3044.50 ± 106.77b | 2944.50 ± 21.92b |
Setback viscosity (cP) | 639.50 ± 51.62a | 428.50 ± 44.55b | 411.50 ± 6.36b |
Retrogradation (cP) | 1295.50 ± 26.16a | 1115.00 ± 4.24b | 1004.00 ± 39.60c |
Note: The results were reported as means ± standard deviation, in the same line with different letters were significant differences (P < 0.05).
Abbreviations: DDT, dough development time; DS, degree of softening; DST, dough stability time; FQN, farinograph quality number; WA, water absorption of dough.
Effect of Inulin with different polymerisation degrees on dry and wet gluten content, gluten index, farinograph properties, and gelatinisation characteristics of dough
Indicators . | Control group . | 5% SCI . | 5% LCI . |
---|---|---|---|
Wet gluten (g). | 29.63 ± 0.42a | 28.73 ± 0.49a | 29.02 ± 0.62a |
Dry gluten (g). | 10.63 ± 0.26a | 9.93 ± 0.31b | 10.09 ± 0.12b |
Gluten index | 90 ± 1.73b | 84 ± 2.08c | 93 ± 0.84b |
WA(%) | 60.05 ± 0.15a | 53.00 ± 0.31c | 54.50 ± 0.21b |
DDT (min) | 4.04 ± 0.04c | 4.46 ± 0.05b | 17.40 ± 0.14a |
DST (min) | 13.36 ± 0.16b | 10.14 ± 0.21c | 18.53 ± 0.27a |
DS (FU) | 26.00 ± 1.12b | 36.00 ± 1.06a | 27.00 ± 1.27b |
FQN | 167.67 ± 6.08b | 115.67 ± 5.64c | 283.33 ± 7.81a |
Gelatinisation temperature (°C) | 69.35 ± 0.07b | 88.48 ± 0.60a | 87.63 ± 0.53a |
Peak viscosity (cP) | 2914.50 ± 89.80a | 2358.00 ± 111.72b | 2352.00 ± 32.53b |
Trough viscosity (cP) | 2275.00 ± 141.42a | 1929.50 ± 67.18b | 1940.50 ± 26.16b |
Final viscosity (cP) | 3570.50 ± 115.26a | 3044.50 ± 106.77b | 2944.50 ± 21.92b |
Setback viscosity (cP) | 639.50 ± 51.62a | 428.50 ± 44.55b | 411.50 ± 6.36b |
Retrogradation (cP) | 1295.50 ± 26.16a | 1115.00 ± 4.24b | 1004.00 ± 39.60c |
Indicators . | Control group . | 5% SCI . | 5% LCI . |
---|---|---|---|
Wet gluten (g). | 29.63 ± 0.42a | 28.73 ± 0.49a | 29.02 ± 0.62a |
Dry gluten (g). | 10.63 ± 0.26a | 9.93 ± 0.31b | 10.09 ± 0.12b |
Gluten index | 90 ± 1.73b | 84 ± 2.08c | 93 ± 0.84b |
WA(%) | 60.05 ± 0.15a | 53.00 ± 0.31c | 54.50 ± 0.21b |
DDT (min) | 4.04 ± 0.04c | 4.46 ± 0.05b | 17.40 ± 0.14a |
DST (min) | 13.36 ± 0.16b | 10.14 ± 0.21c | 18.53 ± 0.27a |
DS (FU) | 26.00 ± 1.12b | 36.00 ± 1.06a | 27.00 ± 1.27b |
FQN | 167.67 ± 6.08b | 115.67 ± 5.64c | 283.33 ± 7.81a |
Gelatinisation temperature (°C) | 69.35 ± 0.07b | 88.48 ± 0.60a | 87.63 ± 0.53a |
Peak viscosity (cP) | 2914.50 ± 89.80a | 2358.00 ± 111.72b | 2352.00 ± 32.53b |
Trough viscosity (cP) | 2275.00 ± 141.42a | 1929.50 ± 67.18b | 1940.50 ± 26.16b |
Final viscosity (cP) | 3570.50 ± 115.26a | 3044.50 ± 106.77b | 2944.50 ± 21.92b |
Setback viscosity (cP) | 639.50 ± 51.62a | 428.50 ± 44.55b | 411.50 ± 6.36b |
Retrogradation (cP) | 1295.50 ± 26.16a | 1115.00 ± 4.24b | 1004.00 ± 39.60c |
Note: The results were reported as means ± standard deviation, in the same line with different letters were significant differences (P < 0.05).
Abbreviations: DDT, dough development time; DS, degree of softening; DST, dough stability time; FQN, farinograph quality number; WA, water absorption of dough.
Table 1 shows that the wet gluten content was lower in both experimental groups than in the control group, however, there was no significant difference in wet gluten content between the three groups (P > 0.05). When inulin is used to replace a part of the flour, the protein content in the mixed flour is correspondingly reduced. The wet gluten content formed in the system decreases, and the dry gluten content decreases. The gluten index reflected the strength of gluten. The gluten index of the 5% LCI group was 93, which was significantly higher than that of the other two groups (P < 0.05), indicating that LCI enhanced the gluten strength and improved the stability of the gluten protein network structure. The addition of SCI gluten index minimum, only 84.
Farinograph properties
It can be seen from Table 1 that the water absorption of the dough added with SCI or LCI was 11.74% or 9.24% lower than that of the control group, respectively. The possible explanation is that inulin molecules contain a large number of hydroxyl groups, which form hydrogen bonds with water, making it more difficult for free water to migrate and inhibiting the binding of starch to water, thus reducing water absorption (Monireh et al., 2014). The content of fructose in inulin with different degrees of polymerisation is different, which leads to the significant difference in water absorption of dough added with SCI or LCI.
Dough development time is proportional to quality. The DDT of adding LCI increased from 4.04 to 17.40 min, which was 4.3 times that of the control group. The DDT of adding SCI increased slightly compared with the control group. The stability time reflects the dough kneading resistance (Liu et al., 2018). DST and farinograph quality number of dough added with LCI increased by 38.70% and 68.98% compared with the control group, which was similar to that studied by (Zhao, 2017). DST and FQN of the addition of SCI were reduced by 24.10% and 31.01%, indicating that SCI weakens the shear resistance of dough. The gluten will not be resistant to stirring, and it is easy to collapse after awakening. The gluten network is not dense and easy to be destroyed.
Significantly low values of the degree of softening may indicate that the dough have higher capability of tolerating long mechanical processing. The DS of dough with SCI was 36 FU significantly greater than that of the control group, while the dough DS of adding LCI and the control group had no significant difference. This is consistent with (Peressini & Sensidoni, 2009) that inulin with high polymerisation degree determined large changes in linear viscoelastic properties of dough. Therefore, LCI is beneficial to improve flour farinograph.
Dynamic rheological properties
As a viscoelastic material, dough possesses both viscous and elastic properties. The viscous and elastic behaviours are represented by energy storage modulus G′ and loss modulus G″, respectively (Xu et al., 2021). From Fig. 1, it can be seen that elastic modulus G′ of dough added with SCI or LCI gradually increases with increasing scanning frequency, however, it was always significantly smaller than that of the control group. It is considered that the substitution of inulin reduces the protein content in flour and the gluten content formed, inhibits the cross-linking reaction of the gluten protein, and thus weakens the elasticity of gluten network structure.
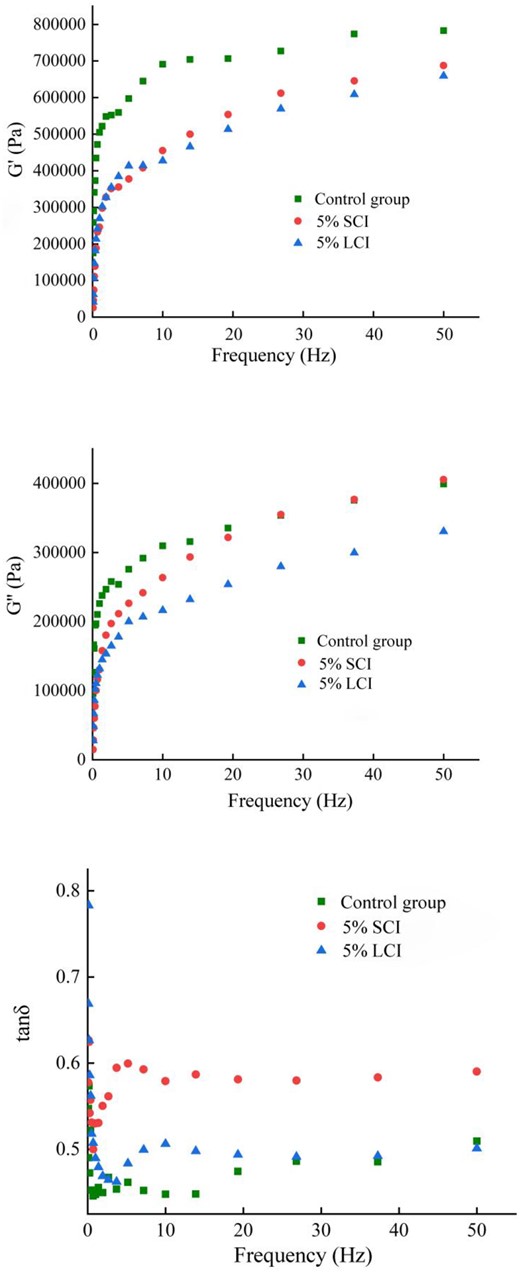
Effect of different polymerisation degrees of inulin on the elastic modulus (G′), loss modulus (G″), and tan delta (tanδ) of dough.
With the increase of scanning frequency, the viscosity modulus G″ of dough added with SCI increased greatly. When the scanning frequency was 0 ∼ 20 Hz, G″ was significantly lower than that of the control group. When the scanning frequency was 30 Hz, G″ was basically the same as that of the control group. When the scanning frequency reached 40 ∼ 50 Hz, G″ exceeded the control group. This is due to the interaction of fructose in the SCI and starch, which increases the viscosity of the dough system, showing a stronger fluid property. Although the dough G″ added with LCI also gradually increased, the growth rate was small. It shows that the gluten protein structure is compact.
In all three groups of dough, elastic modulus and viscous modulus increased with increasing scanning frequency, however, the elastic modulus was always greater than the viscous modulus, that is G′ > G″. The dough showed typical weak gel dynamic rheological spectra.
The tan delta indicates the ratio of viscosity to the elasticity of the system. The proportion of low polymerisation molecules is high, the fluidity is strong, and tanδ is large; the high degree of polymerisation of the high proportion of molecules, elastic, tanδ is small. Yuan et al. (2021) found that as the value of tanδ increases, the more energy lost and unrecoverable after deformation gradually increases, which indicates that the gluten protein network structure is seriously damaged, leading to poor dough rheological properties. When the frequency is 50 Hz, the tanδ of the dough added with SCI is about 0.6, the tanδ of the dough added with LCI is about 0.5, and the control group is slightly larger. This study indicates that adding SCI to dough could damage gluten protein and starch granules. In the oscillatory rheological test, the SCI gradually lost its gel solid properties, the elastic coefficient decreased, and the viscosity coefficient increased.
Extensograph properties
In dough production, farinograph properties are used to assess the quality of the flour, however, at the same time, the tensile index can accurately reflect the processing characteristics of the dough. As shown in Fig. 2, there were significant differences in the extensibility of the dough between the control group, and the addition of SCI or LCI at different wake times (P < 0.05). The extensibility of the dough added with SCI was 17.54% and 7.27% higher than that of the control group at 45 min and 90 min, respectively. The SCI contains small molecular fructose and has a high viscosity, so the dough is softer and the air-holding capacity is weak and difficult to shape, so the dough is tensiled more. Adding LCI makes the dough stronger and slightly harder, easier to shape, and the extensibility is stable at around 88 mm at 45, 90, and 135 min. This is partly due to the high degree of polymerisation, the binding force between inulin and gluten is strong. On the other hand, it is also due to the fact that LCI has certain gelling properties that can increase the gluten strength and elasticity of the dough (Nieto-Nieto et al., 2015).
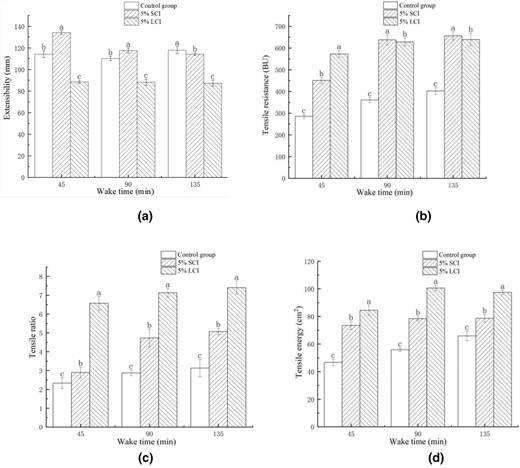
Effect of different polymerisation degrees of inulin on extensograph properties of dough. Different letters indicate significant differences between samples (P < 0.05).
Tensile resistance reflects the strength of the dough, and there is a significant positive relationship between tensile resistance and gluten index (Ba et al., 2019). Figure 2 shows that the tensile resistance of the three dough groups increases with the extension of wake time. When the wake time was 45, 90, and 135 min, the tensile resistance of the dough added with SCI increased by 42.31%, 70.73%, and 54.89%, respectively. The tensile resistance of dough added with LCI increased by 120%, 91.46%, and 73.64%, respectively. The tensile resistance of the dough is large, causing the air in the dough difficult to escape (Ba et al., 2019). During dough waking, the addition of two kinds of inulin could significantly improve the holding capacity of the dough, giving the dough good structure and texture, which was beneficial to the production of high-quality flour products. Compared with SCI, LCI is more beneficial to form a good gluten network in the dough.
The tensile ratio is the ratio of tensile resistance to extensograph, that is, the ratio of strength to expansion force of gluten network structure. A low tensile ratio means low tensile resistance and high extensibility so that the dough will become soft and runny quickly when fermenting, but a high tensile ratio means that the dough has too much resistance, resulting in a hard dough (Zhao, 2018). Figure 2 shows that the tensile ratio of each dough group increased gradually with increasing wake time. When the wake time was fixed, the dough tensile ratio of adding SCI and LCI was significantly greater than that of the control group. When the wake time was 45, 90, and 135 min, the tensile ratio of the dough added with SCI increased by 19.66%, 64.81%, and 61.98%, respectively. Compared with the control group, the tensile ratio of dough added with LCI increased by 181.97%, 148.43%, and 136.42%, respectively. Both groups help to increase the tensile ratio of dough, but the effect of LCI is more significant.
The gluten strength of flour increases with the tensile energy of the dough. Noodles with higher tensile energy have better quality. Figure 2 shows that when the wake time was 45, 90, and 135 min, the tensile energy of the dough added with SCI increased by 55.32%, 39.29%, and 18.18%, respectively. The tensile energy of dough added with LCI increased by 78.72%, 80.36%, and 46.97%, respectively. It can be seen that adding SCI and LCI can increase the tensile energy of dough, LCI is more conducive to the formation of gluten network structure and increase its stability, improve the processing characteristics of dough (Chen et al., 2014).
Starch gelatinisation
Starch gelatinisation occurs when water enters the crystalline region and the internal structure changes from order to disorder. The gelatinisation curves of the control group, 5% SCI or 5% LCI were observed in Table 1, and the addition of inulin had a significant effect on viscosity. Compared to control group, the mixed powder with SCI or LCI had a significant decrease.
In Table 1, we can see that the initial gelatinisation temperature of the mixed powder increased from 69.35 to 88.48 and 87.63 °C with the addition of SCI or LCI. Because the number of external hydroxyl inulin is more hydrophilic than starch, and thus competes with starch gelatinisation needs of water, making starch desalinisation caused by increased difficulty (Hager et al., 2011). The experiment of (Tester & Sommerville, 2003) also confirmed that non-starch polysaccharides can weaken the hydration ability of the amorphous region of starch, resulting in an increase in the gelatinisation temperature.
The peak viscosity, trough viscosity, and final viscosity of the mixed powder with SCI or LCI were significantly lower than those of the control group (P < 0.05). The starch gelatinisation increases the viscosity mainly because of the water absorption of amylopectin, and the crystal structure of inulin will expose more hydroxyl groups due to high-temperature damage above 80 °C, competing with amylopectin for water, making it unable to fully absorb water to form a complete viscous substance (Luo et al., 2017b). Alamri et al. (2012) also found that in the gelatinisation process of polysaccharide molecules will adhere to the surface of the starch, which will restrain starch granule expansion, and inhibit amylose leaching, so that the viscosity of the mixture will decrease.
The stability of starch granules during heating is negatively correlated with the setback viscosity. The setback viscosity of the mixed powder with SCI or LCI were reduced by 32.99% and 35.65%, respectively. This indicates that both groups were beneficial to enhance the stability of starch granules. The retrogradation is positively correlated with degree of recrystallisation after starch gelatinisation. The retrogradation value of the mixed powder with SCI or LCI decreased by 13.93% or 22.50%, respectively. This indicates that the effect of an appropriate amount of LCI on inhibiting starch retrogradation is significantly stronger than that of SCI.
Texture properties
It can be seen from Table 3 that compared with the control group, the hardness of fresh noodles added with SCI or LCI decreased by 5.11% or 2.48%, respectively. This is because inulin and protein compete for water, reducing gluten content and weakening the ability to resist external forces (Li et al., 2021). Chewiness decreased by 11.20% and 8.78%, respectively. In contrast, fresh noodles with SCI or LCI added did not differ significantly in their resilience (P > 0.05). The adhesiveness of fresh noodles added with SCI or LCI was 15.88% or 7.64% lower than that of fresh noodles added with control.
Tensile force reflects dough strength and flexibility, while tensile distance reflects dough extensibility and plasticity. As shown in Table 3, compared to the control group, the tensile force and rupture distance of fresh noodles added with SCI decreased by 7.36% and 7.40%, and the tensile force and rupture distance of fresh noodles added with LCI increased by 3.91% and 6.35%, respectively. This is because LCI increases the water absorption capacity of fresh noodles, allowing the gluten protein in wheat flour to fully absorb water and form a good gluten network structure, thus improving the tensile properties of fresh noodles and increasing the gluten strength of noodles. Because the molecular structure of SCI is small, it can easily escape from the inside and surface of noodles, competing with starch and protein for water absorption, reducing noodles water absorption, limiting gluten formation and weakening the tensile properties of fresh noodles.
Taking into account the extensograph properties above, the dough with SCI has extensibility resulting in noodles that break easily when cooked. The dough with LCI had less elongation, but the dough had the better tensile properties after cooking. This indicates that LCI can increase the resilience of raw noodles.
Cooking properties
The water absorption reflects the degree of expansion of the noodles after cooking, less water absorption may result in tough noodles with a poor texture, and too much water absorption may lead to insufficient elasticity and toughness of noodles (Feng, 2013). As can be seen from Table 2, compared with the control group, adding LCI to fresh noodles increased the water absorption by 5.15%; adding SCI to fresh noodles reduced water absorption by 4.63%. This is because LCI has more hydroxyl groups on the molecular chain and strong hygroscopicity. It can adsorb a large amount of water to the centre of the noodles during cooking, forming a good gluten network structure, and making noodles chewier.
Effects of different polymerisation degrees of inulin on cooking properties and moisture distribution of raw noodles
Indicators . | Control group . | 5% SCI . | 5% LCI . |
---|---|---|---|
Water absorption(%) | 51.64 ± 0.72b | 49.25 ± 0.54c | 54.30 ± 0.33a |
Cooking loss rate(%) | 4.77 ± 0.03c | 5.31 ± 0.01a | 5.09 ± 0.03b |
T21 | 0.11 ± 0.01a | 0.12 ± 0.01a | 0.11 ± 0.02a |
T22 | 11.94 ± 0.06a | 11.12 ± 0.03b | 9.80 ± 0.14c |
P21 | 12.92 ± 0.11b | 11.83 ± 0.17c | 13.35 ± 0.07a |
P22 | 86.84 ± 0.11b | 87.69 ± 0.17a | 86.35 ± 0.06c |
Indicators . | Control group . | 5% SCI . | 5% LCI . |
---|---|---|---|
Water absorption(%) | 51.64 ± 0.72b | 49.25 ± 0.54c | 54.30 ± 0.33a |
Cooking loss rate(%) | 4.77 ± 0.03c | 5.31 ± 0.01a | 5.09 ± 0.03b |
T21 | 0.11 ± 0.01a | 0.12 ± 0.01a | 0.11 ± 0.02a |
T22 | 11.94 ± 0.06a | 11.12 ± 0.03b | 9.80 ± 0.14c |
P21 | 12.92 ± 0.11b | 11.83 ± 0.17c | 13.35 ± 0.07a |
P22 | 86.84 ± 0.11b | 87.69 ± 0.17a | 86.35 ± 0.06c |
Note: The results were reported as means ± standard deviation, in the same line with different letters were significant differences (P < 0.05).
Effects of different polymerisation degrees of inulin on cooking properties and moisture distribution of raw noodles
Indicators . | Control group . | 5% SCI . | 5% LCI . |
---|---|---|---|
Water absorption(%) | 51.64 ± 0.72b | 49.25 ± 0.54c | 54.30 ± 0.33a |
Cooking loss rate(%) | 4.77 ± 0.03c | 5.31 ± 0.01a | 5.09 ± 0.03b |
T21 | 0.11 ± 0.01a | 0.12 ± 0.01a | 0.11 ± 0.02a |
T22 | 11.94 ± 0.06a | 11.12 ± 0.03b | 9.80 ± 0.14c |
P21 | 12.92 ± 0.11b | 11.83 ± 0.17c | 13.35 ± 0.07a |
P22 | 86.84 ± 0.11b | 87.69 ± 0.17a | 86.35 ± 0.06c |
Indicators . | Control group . | 5% SCI . | 5% LCI . |
---|---|---|---|
Water absorption(%) | 51.64 ± 0.72b | 49.25 ± 0.54c | 54.30 ± 0.33a |
Cooking loss rate(%) | 4.77 ± 0.03c | 5.31 ± 0.01a | 5.09 ± 0.03b |
T21 | 0.11 ± 0.01a | 0.12 ± 0.01a | 0.11 ± 0.02a |
T22 | 11.94 ± 0.06a | 11.12 ± 0.03b | 9.80 ± 0.14c |
P21 | 12.92 ± 0.11b | 11.83 ± 0.17c | 13.35 ± 0.07a |
P22 | 86.84 ± 0.11b | 87.69 ± 0.17a | 86.35 ± 0.06c |
Note: The results were reported as means ± standard deviation, in the same line with different letters were significant differences (P < 0.05).
Cooking loss is mainly caused by the leaching of amylose and soluble proteins during cooking of raw noodles, which makes the cooking water cloudy and the surface of the noodles sticky. Both groups significantly increased the cooking loss of the noodles. As inulin molecules are much smaller than wheat flour, and the partial degradation of starch granules in the high temperature, high pressure process allows them to escape more easily from the gluten protein network structure. The cooking loss rate of fresh noodles added with SCI was higher, mainly because SCI molecules were smaller and easier to lose during cooking. This also confirms that (Aravind et al., 2012) pointed out that LCI has less impact on the processing and sensory quality of the noodles, while even a 5% addition of SCI negatively impacts the noodles' cooking loss and organoleptic properties.
Water distribution
The relaxation time of fresh noodles was divided into three peaks, which were located at 0.1 ∼ 1, 10 ∼ 100, and 100 ∼ 1000 ms. It shows that there are three states of water. T21 represents the relaxation time of tightly bound water interacting with gluten protein, and the fluidity of water molecules is weak. T22 represents the relaxation time of weakly bound water connected to macromolecules such as starch. T23 denotes the relaxation time of free water. The ratio of each peak area to the total area was recorded as P21, P22, and P23, indicating the relative content of each component of water, of which P23 was generally ignored.
Figure 3 demonstrates that T22 is the main peak, indicating that the water distribution of fresh noodles is dominated by weakly bound water. The relaxation time T22 of fresh noodles added with LCI was significantly shortened, indicating that the fluidity of water was weakened.
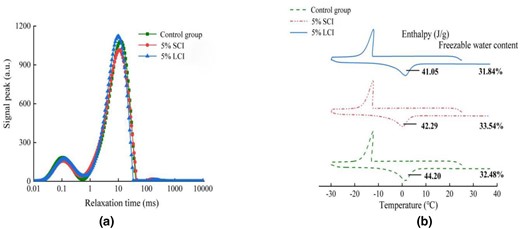
Relaxation time of fresh noodles with different polymerisation degrees of inulin (a). Effect of inulin with different degrees of polymerisation on the content of freezable water in fresh noodles (b).
A comparison of Table 2 with the control group shows an increase of 3.33% in tightly bound water P21 and an increase of 0.56% in weakly bound water P22. The number of hydroxyl groups on the molecular chain of LCI is higher, which binds to the gluten protein in the form of hydrogen bonds, increases the density and stability of the network structure, and promotes the migration of weakly bound water to tightly bound water. The P21 of fresh noodles with SCI decreased by 8.44% and P22 increased by 0.98%. SCI may have changed the water distribution state in the fresh noodles, converting the tightly bound water into weakly bound water.
Freezable water content
Fresh noodles contain about 35% ∼ 40% water, one part is freezable water, which expands when frozen and damages the gluten network structure; the other part is non-freezable water, which is firmly bound in the gluten protein or starch structure and generally does not freeze.
As can be seen from Fig. 3, compared with the control group, adding SCI or LCI of fresh noodles melting enthalpy values were significantly reduced. The freezable water content of fresh noodles added with SCI increased by 3.26%, and the freezable water content of fresh noodles added with LCI decreased by 1.97%. Results showed that LCI could inhibit the conversion of non-freezable water into freezable water, mainly due to two factors: on the one hand, the LCI is a linear straight-chain polysaccharide, which increases the viscosity of the system, making the molecular movement of freezable water in the fresh noodles restricted; on the other hand, the LCI has a stronger wrapping effect on the starch granules, blocking the interaction between starch and water molecules, thus inhibiting the migration of non-freezable water and leading to a decrease in the freezable water content, which is considered to have a better anti-freezable effect. This corresponds to the previous results of the water distribution state measured using low-field NMR.
Sensory quality
Using sensory evaluation to evaluate food quality directly reflects consumer acceptance of food. It can be seen from Table 3 that inulin makes the fresh noodles more white, slightly sweet, and has a unique fragrance. Adding SCI fresh noodles palatability and toughness score is low, so the total score is slightly lower than the control group. The addition of LCI fresh noodles results in higher viscosity and toughness scores, chewy taste, significantly increased flavour and the highest sensory score.
As shown in Table 3, after adding LCI and SCI, the brightness value L* increased significantly compared with the control group; yellowness value b* decreased significantly; a* increased significantly. Inulin is bright white and more transparent than wheat flour, which may increases acceptance of inulin fresh noodles for consumers with specific preference (Difonzo et al., 2022).
Effect of different polymerisation degrees of inulin on texture properties, sensory quality, and brightness of fresh noodles
Properties . | Indicators . | Control group . | 5% SCI . | 5% LCI . |
---|---|---|---|---|
Texture properties | Hardness (g) | 9291.52 ± 107.77a | 8816.31 ± 118.46b | 9061.45 ± 145.19ab |
Resilience | 0.94 ± 0.03a | 0.93 ± 0.03a | 0.95 ± 0.03a | |
Adhesiveness | 6036.81 ± 87.27a | 5078.22 ± 113.93c | 5575.77 ± 143.75b | |
Chewiness (g) | 5767.26 ± 150.93a | 5121.13 ± 164.04b | 5260.73 ± 52.03b | |
Tensile force (g) | 63.46 ± 0.69b | 58.79 ± 0.83c | 65.94 ± 0.85a | |
Rupture distance (mm) | 12.44 ± 0.38a | 11.52 ± 0.40b | 13.23 ± 0.79a | |
Sensory | External appearance | 9.1 | 9.3 | 9.3 |
Colour | 8.8 | 9.2 | 9.3 | |
Hardness | 19.2 | 18.9 | 19.5 | |
Stickiness | 20.8 | 20.5 | 21.2 | |
Tenacity | 21.3 | 20.7 | 21.8 | |
Smoothness | 4.8 | 4.8 | 4.9 | |
Aroma/Flavour | 4.6 | 4.6 | 4.7 | |
Total score | 88.6 | 88.0 | 90.7 | |
Brightness value | L* | 67.21 ± 0.58b | 68.05 ± 0.66a | 68.07 ± 0.61a |
a* | −3.88 ± 0.22c | −3.14 ± 0.18b | −2.87 ± 0.30a | |
b* | 20.31 ± 0.06a | 19.93 ± 0.09b | 19.67 ± 0.08b |
Properties . | Indicators . | Control group . | 5% SCI . | 5% LCI . |
---|---|---|---|---|
Texture properties | Hardness (g) | 9291.52 ± 107.77a | 8816.31 ± 118.46b | 9061.45 ± 145.19ab |
Resilience | 0.94 ± 0.03a | 0.93 ± 0.03a | 0.95 ± 0.03a | |
Adhesiveness | 6036.81 ± 87.27a | 5078.22 ± 113.93c | 5575.77 ± 143.75b | |
Chewiness (g) | 5767.26 ± 150.93a | 5121.13 ± 164.04b | 5260.73 ± 52.03b | |
Tensile force (g) | 63.46 ± 0.69b | 58.79 ± 0.83c | 65.94 ± 0.85a | |
Rupture distance (mm) | 12.44 ± 0.38a | 11.52 ± 0.40b | 13.23 ± 0.79a | |
Sensory | External appearance | 9.1 | 9.3 | 9.3 |
Colour | 8.8 | 9.2 | 9.3 | |
Hardness | 19.2 | 18.9 | 19.5 | |
Stickiness | 20.8 | 20.5 | 21.2 | |
Tenacity | 21.3 | 20.7 | 21.8 | |
Smoothness | 4.8 | 4.8 | 4.9 | |
Aroma/Flavour | 4.6 | 4.6 | 4.7 | |
Total score | 88.6 | 88.0 | 90.7 | |
Brightness value | L* | 67.21 ± 0.58b | 68.05 ± 0.66a | 68.07 ± 0.61a |
a* | −3.88 ± 0.22c | −3.14 ± 0.18b | −2.87 ± 0.30a | |
b* | 20.31 ± 0.06a | 19.93 ± 0.09b | 19.67 ± 0.08b |
Note: The above results are the average of ten sensory evaluations. The results were reported as means ± standard deviation, in the same line with different letters were significant differences (P < 0.05). Chinese national standard (GB/T 25005–2010) were used for training and evaluation of sensory evaluation attributes.
Effect of different polymerisation degrees of inulin on texture properties, sensory quality, and brightness of fresh noodles
Properties . | Indicators . | Control group . | 5% SCI . | 5% LCI . |
---|---|---|---|---|
Texture properties | Hardness (g) | 9291.52 ± 107.77a | 8816.31 ± 118.46b | 9061.45 ± 145.19ab |
Resilience | 0.94 ± 0.03a | 0.93 ± 0.03a | 0.95 ± 0.03a | |
Adhesiveness | 6036.81 ± 87.27a | 5078.22 ± 113.93c | 5575.77 ± 143.75b | |
Chewiness (g) | 5767.26 ± 150.93a | 5121.13 ± 164.04b | 5260.73 ± 52.03b | |
Tensile force (g) | 63.46 ± 0.69b | 58.79 ± 0.83c | 65.94 ± 0.85a | |
Rupture distance (mm) | 12.44 ± 0.38a | 11.52 ± 0.40b | 13.23 ± 0.79a | |
Sensory | External appearance | 9.1 | 9.3 | 9.3 |
Colour | 8.8 | 9.2 | 9.3 | |
Hardness | 19.2 | 18.9 | 19.5 | |
Stickiness | 20.8 | 20.5 | 21.2 | |
Tenacity | 21.3 | 20.7 | 21.8 | |
Smoothness | 4.8 | 4.8 | 4.9 | |
Aroma/Flavour | 4.6 | 4.6 | 4.7 | |
Total score | 88.6 | 88.0 | 90.7 | |
Brightness value | L* | 67.21 ± 0.58b | 68.05 ± 0.66a | 68.07 ± 0.61a |
a* | −3.88 ± 0.22c | −3.14 ± 0.18b | −2.87 ± 0.30a | |
b* | 20.31 ± 0.06a | 19.93 ± 0.09b | 19.67 ± 0.08b |
Properties . | Indicators . | Control group . | 5% SCI . | 5% LCI . |
---|---|---|---|---|
Texture properties | Hardness (g) | 9291.52 ± 107.77a | 8816.31 ± 118.46b | 9061.45 ± 145.19ab |
Resilience | 0.94 ± 0.03a | 0.93 ± 0.03a | 0.95 ± 0.03a | |
Adhesiveness | 6036.81 ± 87.27a | 5078.22 ± 113.93c | 5575.77 ± 143.75b | |
Chewiness (g) | 5767.26 ± 150.93a | 5121.13 ± 164.04b | 5260.73 ± 52.03b | |
Tensile force (g) | 63.46 ± 0.69b | 58.79 ± 0.83c | 65.94 ± 0.85a | |
Rupture distance (mm) | 12.44 ± 0.38a | 11.52 ± 0.40b | 13.23 ± 0.79a | |
Sensory | External appearance | 9.1 | 9.3 | 9.3 |
Colour | 8.8 | 9.2 | 9.3 | |
Hardness | 19.2 | 18.9 | 19.5 | |
Stickiness | 20.8 | 20.5 | 21.2 | |
Tenacity | 21.3 | 20.7 | 21.8 | |
Smoothness | 4.8 | 4.8 | 4.9 | |
Aroma/Flavour | 4.6 | 4.6 | 4.7 | |
Total score | 88.6 | 88.0 | 90.7 | |
Brightness value | L* | 67.21 ± 0.58b | 68.05 ± 0.66a | 68.07 ± 0.61a |
a* | −3.88 ± 0.22c | −3.14 ± 0.18b | −2.87 ± 0.30a | |
b* | 20.31 ± 0.06a | 19.93 ± 0.09b | 19.67 ± 0.08b |
Note: The above results are the average of ten sensory evaluations. The results were reported as means ± standard deviation, in the same line with different letters were significant differences (P < 0.05). Chinese national standard (GB/T 25005–2010) were used for training and evaluation of sensory evaluation attributes.
Conclusion
The addition of LCI increases the compactness of the gluten network, improves the kneading resistance of the dough, and greatly improves the farinograph properties; the tensile resistance and tensile energy are significantly improved, and the dough has good elasticity and toughness. The dough with the addition of SCI has poor kneading resistance and toughness, and the noodles have increased extensibility, little elasticity and high viscosity, making it difficult to shape. Compared with SCI, LCI has a more significant effect in increasing the stability of starch granules and inhibiting retrogradation. It was found that the tensile properties and water absorption of fresh noodles with LCI were increased, which gave fresh noodles good viscoelasticity and extensibility. The relaxation time of weakly bound water was shortened, the proportion of tightly bound water increased, and the content of freezable water was reduced. However, the tensile force and rupture distance of fresh noodles with SCI were reduced, the water absorption rate decreased, the cooking loss rate increased, and the palatability decreased. In this system, the proportion of tightly bound water is reduced, the quantity of freezable water increases, and the water fluidity is improved. This shows that the addition of LCI can inhibit the fluidity of water in frozen cooked noodles and enhance the toughness and stability of the gluten protein network structure. The research shows that the addition of LCI to flour can effectively improve the quality of the fresh noodles.
Acknowledgments
This study was supported by the National Key Research and Development Project of China (2019YFC1605800), the Doctoral Scientific Research Start-up Foundation (2019BS022) and Natural Science Innovation Fund Support Plan (2020ZKCJ13) from Henan University of Technology, and Henan Province Key Science and Technology Research Program from Education Department of Henan Province (22B550001).
Author contributions
yan yan chen: Data curation (equal); formal analysis (equal); investigation (equal); methodology (equal); software (equal); writing – original draft (equal); writing – review and editing (equal). Xueqin Li: Conceptualization (equal); funding acquisition (equal); methodology (equal); project administration (equal); supervision (equal); validation (equal); writing – review and editing (equal). Fei Xu: Conceptualization (equal); funding acquisition (equal); methodology (equal); project administration (equal); supervision (equal); validation (equal); writing – review and editing (equal). Qing Yu: Data curation (equal); formal analysis (equal); software (equal); writing – original draft (equal). Qingqing zhang: Data curation (equal); investigation (equal); software (equal).
Conflict of interest statement
All authors declare that they have no conflict of interest in the subject matter or materials discussed in this manuscript.
Data availability statement
The data presented in this study are available within the article.
References
This study exploited inulin with high degree of polymerisation as a replacement of durum wheat semolina for the production of functional fresh pasta. The results suggests that adding inulin to wheat products could enhance the functional properties of original product without compromise the quality characteristics.
In this study, the effects of the addition of inulin at different DP on the quality, sensory properties, and glycaemic index of four wholemeal spaghetti produces by ancient Sicilian cultivars of durum wheat was evaluated. The results suggests that high polymerisation degree inulin, demonstrate better effect on enhancing the functional property of the original food.
The aim of this work was to study the influences of three types of inulin with different degree of polymerisation on the water state of steamed bread during production and storage. The addition of inulin reduced the moisture content of the dough and facilitated the migration of free water to bound water. This study provided insights for the effect of inulin on water migration and redistribution and this theory could contribute to the wheat products quality management.
In this research, three types of inulin (short-chain inulin-DP <10, middle-chain inulin-DP >10, and long-chain inulin-DP >23) with different degrees of polymerisation were used, to investigate the effects of inulin on the pasting, thermal, and rheological properties of rice starch and to provide the foundation for the application of inulin in Rice Starch-based food. The results of this research revealed potential of inulin of being a valuable additive in rice starch-based products.