-
PDF
- Split View
-
Views
-
Cite
Cite
Yang Yue, Yunbo Li, Pengcheng Li, Yuyi Xiao, Chunhong Piao, Xiujuan Wang, Comparison of physicochemical and functional characteristics of insoluble dietary fibre from okara by separate and co-fermentation with lactic acid bacteria and Kluyveromyces marxianus, International Journal of Food Science and Technology, Volume 58, Issue 10, October 2023, Pages 4992–5000, https://doi.org/10.1111/ijfs.16561
- Share Icon Share
Abstract
Okara, a natural source of dietary fibre (DF), is recognised as an edible and safe material. In this study, lactic acid bacteria (LAB) and Kluyveromyces marxianus were used to ferment okara in separately or in combination, and the physicochemical and functional characteristics of obtained insoluble dietary fibre (IDF) excluded from okara were compared. The result showed that compared with non-fermented okara, the content of IDF from co-fermented okara by LAB and K. marxianus increased by 6.8%, while separate fermentations were 2.4% and 4.0%. Scanning electron microscopy result showed that the structure of co-fermented okara IDF was looser and porous with the edge disappeared compared to separate and non-fermented okara. All the fermentation treatment groups effectively reduced the particle size and affected the cellulose and hemicellulose functional groups of IDF. Furthermore, compared with non-fermented okara, processing performance, and the activities of hypoglycaemic and hypolipidaemic of IDF were greatly improved in fermented okara.
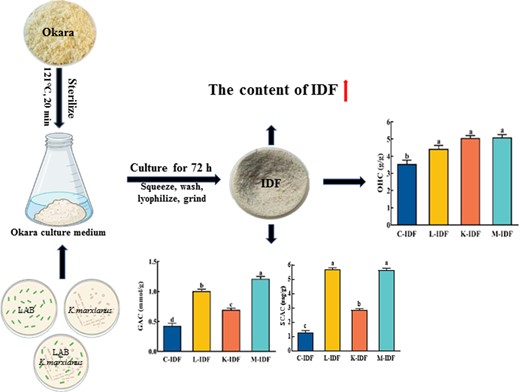
Introduction
Okara is a by-product of soybean milk, tofu, or other soybean products (Park et al., 2015). Although the therapeutic effects of dietary fibre (DF) from okara have been reported (So et al., 2018; Corpuz et al., 2019), okara has been usually utilised for animal feed or discarded owing to the rough taste and perishability. Insoluble dietary fibre (IDF) in okara is a kind of renewable biopolymer with abundant reserves (Dhingra et al., 2012), accounting for more than 90% of the total dietary fibre (TDF) in okara (Vong & Liu, 2016). Okara IDF has attracted much concern as a functional ingredient due to its excellent biological properties and promising applications in food manufacturing (Antonio et al., 2008; Corpuz et al., 2019). Therefore, to promote the utilisation of okara, the modification of okara has become an essential purpose to alleviate rough taste, strong beany, and anti-nutritional factors while improving the processing and functional ability of okara.
In recent years, plenty of modification methods of IDF including physical–chemical (Chen et al., 2019; Lin et al., 2020), enzymatic (Perez-Lopez et al., 2017), and microbial fermentation (Lin et al., 2020) have been investigated. Many studies on the manufacture of functional food additives through okara fermentation reported a great improvement in the quality of fermented okara (Liu et al., 2017; Zhang et al., 2019), which was favoured by consumers. Our previous research also confirmed that the nutritional value and processing properties of okara were remarkably enhanced after modification by Kluyveromyces marxianus fermentation (Hu et al., 2019). Lactic acid bacteria (LAB) are widely recognised as a kind of important microorganism used in food processing. In recent years, studies on improving the properties of DF by LAB fermentation are increasing (Lin et al., 2020; Sun et al., 2020). LAB produced a series of enzymes during fermentation that created an acidic environment to degrade fibre (Lin et al., 2020). Tu et al. (2014) reported that the microstructural and crystal structure of okara DF have been changed after fermentation with LAB and treatment with dynamic high-pressure micro-fluidisation (DHPM). Lin et al. (2020) reported that the availability and functional performance of okara DF were improved by co-fermentation with LAB and Neurospora crassa, indicating a new strategy for okara modification. Therefore, it is very curious to confirm the influence of co-fermentation by LAB and K. marxianus on okara DF.
The aim of this study is to modify IDF from okara in separate or co-fermentation by LAB and K. marxianus and to compare the influence on the physicochemical characteristics and functionality. This paper shows a greater application prospect for the co-fermentation by LAB and K. marxianus and provides foundation for the application of okara IDF in the foods industry.
Materials and methods
Materials and chemicals
Soybean was provided by Shengfeng Seed company (Shandong, China). LAB (Streptococcus thermophilus and Lactobacillus bulgaricus were used as combined LAB culture) was purchased from Ubitus Biotechnology Co., Ltd. (Qingdao, China). Kluyveromyces marxianus previously isolated from kefir grains was preserved at Jilin Province Innovation Center for Food Biological Manufacture (Changchun, China). Standards of cholesterol, glucose, and sodium cholate were purchased from Sigma Reagent Company (Shanghai, China).
Preparation of okara IDF
Okara preparation and activation of K. marxianus were performed according to Wang's method (Wang et al., 2020). The okara medium was sterilised for 20 min at 121 °C, cooled to room temperature, and then LAB, K. marxianus, and the two mixed strains (1:1, v/v) were inoculated into at a ratio of 1:1000 (v/v), respectively, and cultured at 34 °C and 120 r.p.m. for 72 h (Wang et al., 2020). The okara after fermentation was squeezed and washed twice with distilled water (70 °C). Then, the residue was lyophilised (FDU-7006, Oberon Co., Gimpo, Korea), superfine ground (FDV, Youqi, Co., Ltd, Japan), and screened with a 60-mesh sieve to obtain IDF, which was named L-IDF, K-IDF, and M-IDF (fermented by LAB, K. marxianus, and the two mixed strains, respectively). The IDF from non-fermented okara was used as a control, named C-IDF.
Proximate composition of okara IDF
The determination of moisture, ash, crude protein, crude fat, IDF, and SDF was carried out in accordance with 2016 Chinese national norms GB 5009.
Scanning electron microscopy (SEM)
The microstructure was observed as described by Wang's method (Wang et al., 2020). The samples were sputtered with gold and then watched using an SEM (TM3030plus, Hitachi Tech Desktop Microscope, Tokyo, Japan) at 100 kV under 500× and 1000× magnifications.
Particle size determination
Laser particle size analyser (BT-9300S, Dandong Baite Company, Liaoning, China) was used to measure the particle size distribution. IDF was prepared into 0.1% solution and ultrasonically dispersed with distilled water for analysis, and particle size distribution parameter was median diameter [D50 (μm)].
Fourier transform infrared spectroscopy (FT-IR)
Dry power of IDF and potassium bromide were pressed into small transparent flakes according to Sun's method (Sun et al., 2020) and then determined using an FT-IR equipped with an ATR accessory (IS50, Nicolet, Madison, WI, USA) at a scanning wave from 400 to 4000 cm−1 and scanning resolution of 4.0 cm−1, the scanning integrated spectrum was 32 times.
Chromaticity determination
The IDF powder was placed on the sample tray of a colorimeter (Colour Flex EZ, America) and observed according to Kurek' method (Kurek et al., 2018).
The capacity of water-holding (WHC), oil-holding (OHC), and water-swelling (WSC)
The WHC and OHC were measured by Mateos' method (Mateos-Aparicio et al., 2010), and the WSC was measured according to Xue' method (Xue et al., 2019).
The capacity of glucose adsorption (GAC) and the index of glucose dialysis retardation (GDRI)
The GAC was measured according to Chu' method (Chu et al., 2019), and the GDRI was measured according to Qi' method (Qi et al., 2016).
The capacity of cholesterol adsorption (CAC) and sodium cholate adsorption (SCAC)
The CAC was measured according to Yu' method (Yu et al., 2018), and the SCAC was measured according to Lin's method (Lin et al., 2020).
Statistical analysis
The data were processed by SPSS22.00 (SPSS Inc, Chicago, IL, USA) for analysis. One-way analysis of variance (Anova), LSD (least significant difference), and Duncan's multiple range test were used to analyse significant difference between the values, P < 0.05 was regarded as significant. The experiments were performed in triplicate, and the data were displayed as mean values ± SD.
Results
Proximate composition result of okara IDF
The water, ash, and SDF content of IDFs in fermented okara decreased significantly compared to C-IDF (Table 1), while the crude fat increased significantly (P < 0.05), probably due to the production of cell wall-bound and extracellular lipase by microbial fermentation (Vong et al., 2016; Hu et al., 2019). The significant decrease in crude protein content was accompanied by a significant increase in IDF content. The crude protein content of M-IDF decreased significantly by 24.9%, and the IDF content increased significantly by 6.8% compared to C-IDF; this positive result was mainly due to the β-glucosidase (Su et al., 2020) produced during okara fermentation by K. marxianus. The results indicated that high-purity IDF could be obtained by co-fermentation. Therefore, it is feasible to ferment okara using co-fermentation by LAB and K. marxianus to increase the DF content.
Samples . | Water (%) . | Crude fat(%) . | Crude protein(%) . | Ash (%) . | IDF (%) . | SDF (%) . |
---|---|---|---|---|---|---|
C-IDF | 8.60 ± 0.22a | 5.42 ± 0.25d | 12.00 ± 0.13a | 1.31 ± 0.11a | 70.53 ± 0.19d | 0.49 ± 0.16a |
L-IDF | 5.90 ± 0.26c | 7.75 ± 0.31c | 10.26 ± 0.30d | 1.21 ± 0.08b | 72.25 ± 0.24c | 0.21 ± 0.21d |
K-IDF | 5.56 ± 0.29d | 8.41 ± 0.17b | 9.95 ± 0.12c | 1.02 ± 0.12c | 73.38 ± 0.18b | 0.36 ± 0.15c |
M-IDF | 6.27 ± 0.31b | 8.83 ± 0.10a | 9.01 ± 0.21b | 0.53 ± 0.08d | 75.31 ± 0.11a | 0.38 ± 0.12b |
Samples . | Water (%) . | Crude fat(%) . | Crude protein(%) . | Ash (%) . | IDF (%) . | SDF (%) . |
---|---|---|---|---|---|---|
C-IDF | 8.60 ± 0.22a | 5.42 ± 0.25d | 12.00 ± 0.13a | 1.31 ± 0.11a | 70.53 ± 0.19d | 0.49 ± 0.16a |
L-IDF | 5.90 ± 0.26c | 7.75 ± 0.31c | 10.26 ± 0.30d | 1.21 ± 0.08b | 72.25 ± 0.24c | 0.21 ± 0.21d |
K-IDF | 5.56 ± 0.29d | 8.41 ± 0.17b | 9.95 ± 0.12c | 1.02 ± 0.12c | 73.38 ± 0.18b | 0.36 ± 0.15c |
M-IDF | 6.27 ± 0.31b | 8.83 ± 0.10a | 9.01 ± 0.21b | 0.53 ± 0.08d | 75.31 ± 0.11a | 0.38 ± 0.12b |
The value is the mean ± standard deviation of three independent repetitions (n = 3). Different letters between each column are significantly different (P < 0.05).
Samples . | Water (%) . | Crude fat(%) . | Crude protein(%) . | Ash (%) . | IDF (%) . | SDF (%) . |
---|---|---|---|---|---|---|
C-IDF | 8.60 ± 0.22a | 5.42 ± 0.25d | 12.00 ± 0.13a | 1.31 ± 0.11a | 70.53 ± 0.19d | 0.49 ± 0.16a |
L-IDF | 5.90 ± 0.26c | 7.75 ± 0.31c | 10.26 ± 0.30d | 1.21 ± 0.08b | 72.25 ± 0.24c | 0.21 ± 0.21d |
K-IDF | 5.56 ± 0.29d | 8.41 ± 0.17b | 9.95 ± 0.12c | 1.02 ± 0.12c | 73.38 ± 0.18b | 0.36 ± 0.15c |
M-IDF | 6.27 ± 0.31b | 8.83 ± 0.10a | 9.01 ± 0.21b | 0.53 ± 0.08d | 75.31 ± 0.11a | 0.38 ± 0.12b |
Samples . | Water (%) . | Crude fat(%) . | Crude protein(%) . | Ash (%) . | IDF (%) . | SDF (%) . |
---|---|---|---|---|---|---|
C-IDF | 8.60 ± 0.22a | 5.42 ± 0.25d | 12.00 ± 0.13a | 1.31 ± 0.11a | 70.53 ± 0.19d | 0.49 ± 0.16a |
L-IDF | 5.90 ± 0.26c | 7.75 ± 0.31c | 10.26 ± 0.30d | 1.21 ± 0.08b | 72.25 ± 0.24c | 0.21 ± 0.21d |
K-IDF | 5.56 ± 0.29d | 8.41 ± 0.17b | 9.95 ± 0.12c | 1.02 ± 0.12c | 73.38 ± 0.18b | 0.36 ± 0.15c |
M-IDF | 6.27 ± 0.31b | 8.83 ± 0.10a | 9.01 ± 0.21b | 0.53 ± 0.08d | 75.31 ± 0.11a | 0.38 ± 0.12b |
The value is the mean ± standard deviation of three independent repetitions (n = 3). Different letters between each column are significantly different (P < 0.05).
Apparent structure result of okara IDF
Remarkable changes occurred in the structure of IDFs from fermented okara compared to C-IDF (Fig. 1), and Wu (Wu et al., 2020) observed time-dependent changes in the structure of DF from okara after cavitation jet treatment compared to untreated. Figure 1a showed that C-IDF had a loose sheet structure, a corrugated surface, and a dense structure. The fibre structure of L-IDF was partially destroyed, resulting in a wrinkled surface and more loose flake structures, but its internal structure was relatively complete (Fig. 1b). Figure 1c showed that K-IDF had a rougher and uneven surface structure with more folds and grooves. As for M-IDF, the overall fibre structure was destroyed and the edges disappeared, presenting an irregular fragment with a more loose and dispersed structure (Fig. 1d). This is probably due to the cellulase (Ma & Mu, 2016) produced during okara fermentation degrading crude protein, starch, and cellulose. After fermentation, the changes in the microstructure would affect the physicochemical and functional characteristics of DF (Perez-Lopez et al., 2017) and its application in food (Wang et al., 2015; Li et al., 2019) and were imparted better water and oil retention ability (Zheng et al., 2018).
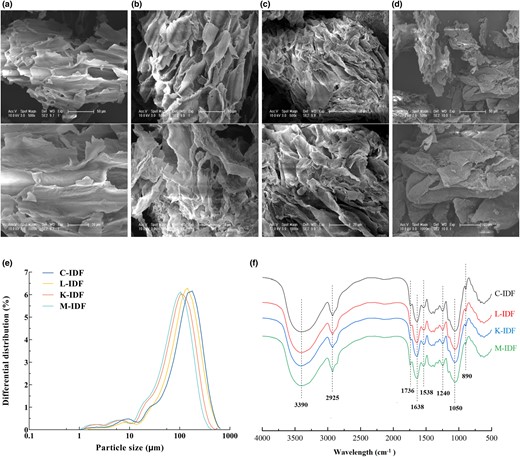
Structural properties of okara IDF. (a) Insoluble dietary fibre used as a control (C-IDF); (b) LAB fermented insoluble dietary fibre (L-IDF); (c) K. marxianus fermented insoluble dietary fibre (K-IDF); (d) Mixed fermented insoluble dietary fibre (M-IDF), the samples were systematically viewed at 500× and 1000× magnifications; (e) Particle size distribution; (f) Fourier transform infrared spectroscopy (FT-IR).
Particle size distribution result of okara IDF
The main peaks of IDFs from fermented okara moved to the left, and the particle size decreased compared with C-IDF (Fig. 1e), suggesting that fermentation could effectively reduce the particle size of IDF, while the M-IDF group had the best effect. This may be due to the enzymes produced during the fermentation process destroyed the part of the IDF with large particle diameters, and the texture became loose, which could effectively improve its adsorption capacity for water and oil. Hahn et al. (2015) regulated that the smaller fibre particle size provided the larger specific area, which in turn provided the fluid more fibres to make it resistant to flow. Thus, separate and co-fermentation reduced the particle size of okara IDF, which ultimately influenced the processing characteristics and functional properties of IDF in the food industry.
FT-IR result of okara IDF
The FT-IR spectra of all samples were similar distributed with approximately the same peak shape in the range of 4000–500 cm−1. Chemical bands at 3390, 2925, 1736, 1638, 1538, 1240, 1050, and 890 cm−1 were detected with the results in Fig. 1f. Strong absorption peaks located around 3390 cm−1 representing the O-H stretching vibration between hydrogen-bond and around 2925 cm−1 representing the C-H stretching vibration of methylene were typical structures of polysaccharides (Gao et al., 2017). But the absorption peaks of fermented IDFs at around 3390 cm−1 shifted slightly to the left compared with C-IDF, which may be caused by free -OH generated by cellulose and hemicellulose hydrolysis during the fermentation. The peaks around 1736 and 1538 cm−1 may be the C=O tensile oscillation of aromatic skeleton and aldehyde/ester group (Zhao et al., 2013), indicating that aldehyde or carboxyl groups and their derivatives may exist in IDF. The bands around 1638 and 1240 cm−1 may be attributed to the stretching of C-O and C=O, corresponding to the typical adsorption of carboxymethyl cellulose (Dai & Huang, 2017). The peak near 1050 cm−1 probably was C-O tensile oscillation in C-O-H and C-O-C of sugar rings in cellulose and hemicellulose (Chu et al., 2019; Jia et al., 2019). And the peak 890 cm−1 represented the tensile oscillation of β-glycosidic bonds in hemicellulose (Gao et al., 2017).
Colour result of okara IDF
Colour is an important index to evaluate DF application in the food industry (Gómez et al., 2010). The L*, a*, and b* colour values for IDFs from fermented and non-fermented okara are summarised in Table 2. An increase in L* value was observed in L-IDF, and a decline in L* value was observed in K-IDF and M-IDF compared to C-IDF, and the latter was in accordance with the results that reported for a decline in IDF from okara fermented by K. marxianus (Wang et al., 2020). All IDFs were characterised by whiter colour (higher L* values). Significant increase in a* value was obtained for IDFs from fermented okara compared to C-IDF, positive a* values indicated a shift towards red (a* > 0). Positive b* values for IDFs indicated a shift towards yellow (b*>0). ▵E values for IDFs from fermented okara ranged from 2.15 to 6.57. K-IDF and M-IDF exerted greater influence compared to C-IDF and L-IDF because ▵E values were >2.3 that is the minimum value at which colour differences could be detected (Djordjevic et al., 2023). The results showed the highest L* value (85.4), b* value (20.27), and a* value (2.20) occurred in L-IDF, K-IDF, and M-IDF, respectively, indicating favourable performance as a food ingredient.
Samples . | L* . | a* . | b* . | ▵E . |
---|---|---|---|---|
C-IDF | 83.78 ± 0.21b | 1.69 ± 0.13d | 17.26 ± 0.14c | 0.00 ± 0.00d |
L-IDF | 85.40 ± 0.11a | 1.73 ± 0.14c | 15.85 ± 0.12d | 2.15 ± 0.10c |
K-IDF | 77.94 ± 0.18d | 1.76 ± 0.14b | 20.27 ± 0.12a | 6.57 ± 0.10a |
M-IDF | 80.09 ± 0.11c | 2.20 ± 0.14a | 19.27 ± 0.12b | 4.23 ± 0.10b |
Samples . | L* . | a* . | b* . | ▵E . |
---|---|---|---|---|
C-IDF | 83.78 ± 0.21b | 1.69 ± 0.13d | 17.26 ± 0.14c | 0.00 ± 0.00d |
L-IDF | 85.40 ± 0.11a | 1.73 ± 0.14c | 15.85 ± 0.12d | 2.15 ± 0.10c |
K-IDF | 77.94 ± 0.18d | 1.76 ± 0.14b | 20.27 ± 0.12a | 6.57 ± 0.10a |
M-IDF | 80.09 ± 0.11c | 2.20 ± 0.14a | 19.27 ± 0.12b | 4.23 ± 0.10b |
The value is the mean ± standard deviation of three independent repetitions (n = 3). Different letters between each column are significantly different (P < 0.05).
Samples . | L* . | a* . | b* . | ▵E . |
---|---|---|---|---|
C-IDF | 83.78 ± 0.21b | 1.69 ± 0.13d | 17.26 ± 0.14c | 0.00 ± 0.00d |
L-IDF | 85.40 ± 0.11a | 1.73 ± 0.14c | 15.85 ± 0.12d | 2.15 ± 0.10c |
K-IDF | 77.94 ± 0.18d | 1.76 ± 0.14b | 20.27 ± 0.12a | 6.57 ± 0.10a |
M-IDF | 80.09 ± 0.11c | 2.20 ± 0.14a | 19.27 ± 0.12b | 4.23 ± 0.10b |
Samples . | L* . | a* . | b* . | ▵E . |
---|---|---|---|---|
C-IDF | 83.78 ± 0.21b | 1.69 ± 0.13d | 17.26 ± 0.14c | 0.00 ± 0.00d |
L-IDF | 85.40 ± 0.11a | 1.73 ± 0.14c | 15.85 ± 0.12d | 2.15 ± 0.10c |
K-IDF | 77.94 ± 0.18d | 1.76 ± 0.14b | 20.27 ± 0.12a | 6.57 ± 0.10a |
M-IDF | 80.09 ± 0.11c | 2.20 ± 0.14a | 19.27 ± 0.12b | 4.23 ± 0.10b |
The value is the mean ± standard deviation of three independent repetitions (n = 3). Different letters between each column are significantly different (P < 0.05).
Processing characteristics result of okara IDF
WHC represents the capability of DF to maintain water content under the external forces like centrifugal pressure. OHC assesses the ability of DF to adsorb fat (Ma & Mu, 2016). And WSC is the ratio of the volume of DF submerged in excess water to the initial weight of DF. Figure 2 showed that the WHC, OHC, and WSC of IDFs from fermented okara were improved compared to C-IDF, except that the WHC of L-IDF was reduced. It may be because the fibre structure of L-IDF was partially destroyed with a wrinkled surface and looser flake structures, but its internal structure was relatively complete without folds and grooves, which resulted in faster water loss, and the WHC was decreased. The WHC, OHC, and WSC of M-IDF were significantly increased by 15.6%, 60.6%, and 23.3% compared to C-IDF, which was better than that of Monascus anka fermentation (Sun et al., 2020). In general, the compact structure of IDF was disrupted by separate or co-fermentation into looser structure and smaller particle size, and more hydrophilic and lipophilic groups exposed (Chau et al., 2007), which led to easy penetration and absorption for water and oil particles (Luo et al., 2018; Sun et al., 2020). Furthermore, the loose structure and high porosity improved IDF's ability to contact and trap water and oil (Liu et al., 2016), thus increasing WHC, OHC, and WSC.
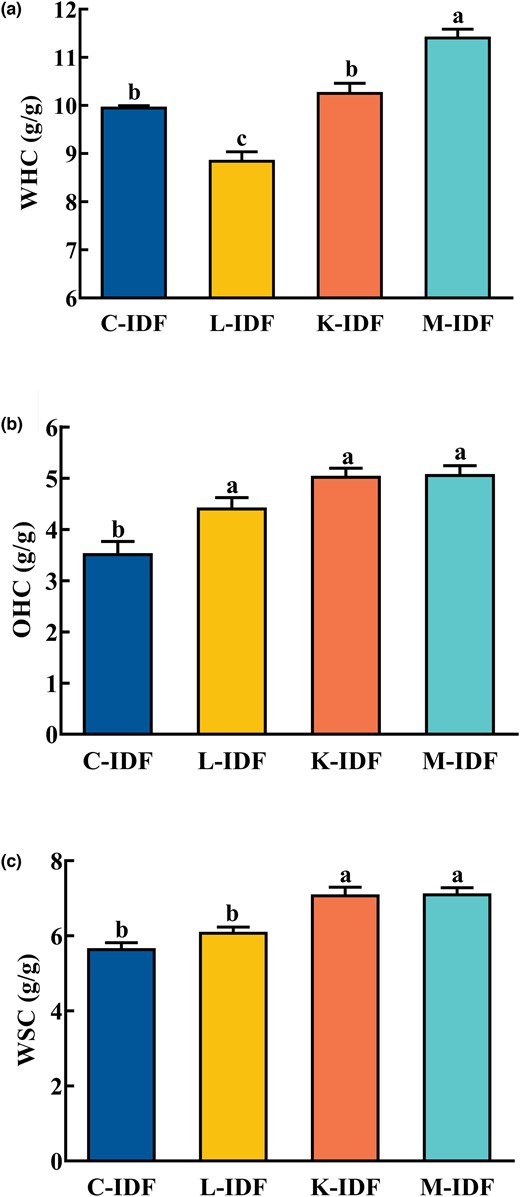
Processing characteristics of okara IDF. (a) The capacity of water-holding (WHC); (b) The capacity of oil-holding (OHC); (c) The capacity of water-swelling (WSC).
GAC and GDRI results of okara IDF
The ability of DF to absorb glucose in the gastrointestinal tract can be indirectly demonstrated by the ability to absorb glucose in vitro (Peerajit et al., 2012,), and the absorption of IDF on glucose can help lower postprandial blood sugar levels. Figure 3a showed that the GAC of L-IDF, K-IDF, and M-IDF were significantly increased by 138.1%, 64.3%, and 185.7% compared to C-IDF, indicating that fermentation could improve the GAC of IDF. It is possible that the porous structure and specific surface of modified IDF led to altered interactions between IDF and glucose (Benítez et al., 2017; Lin et al., 2020), and the formed network structure was easier to block the flow rate of glucose.
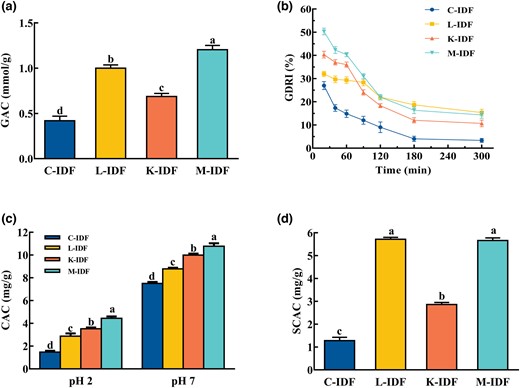
In vitro hypoglycaemic and hypolipidaemic activities of okara IDF. (a) The capacity of glucose adsorption (GAC); (b) The index of glucose dialysis retardation (GDRI); (c) The capacity of cholesterol adsorption (CAC); (d) The capacity of sodium cholate adsorption (SCAC).
The retardation effect of fibre on glucose adsorption in the gastrointestinal tract can be estimated by GDRI (Cheng et al., 2017). Figure 3b showed that the GDRI dropped over time, and the same result was shown by Ahmed (Ahmed et al., 2011). The GDRI of IDFs from fermented okara was always larger than that of C-IDF, indicating that fermentation could delay the diffusion of glucose to a certain extent. The GDRI may be affected by the physical barrier and entrapment ability of fibre particles to glucose particles (Qi et al., 2016; Cheng et al., 2017). Moreover, IDF from okara by separate or co-fermentation had porous structure, larger specific surface (Fig. 1a–d) and smaller particle diameters (Fig. 1e) that altering the interaction between DF and glucose, which facilitated the glucose adsorption on IDF. M-IDF had the best GAC and GDRI.
CAC and SCAC results of okara IDF
DF has been demonstrated to reduce the levels of serum cholesterol and the risk of cardiovascular disease (Nsor-Atindana et al., 2012). Figure 3c showed that the CACs of L-IDF, K-IDF, and M-IDF were 8.26, 9.78, and 10.21 mg g−1 at pH 7 and 2.91, 3.45, and 4.11 mg g−1 at pH 2, respectively, which were higher than those of C-IDF (7.38 mg/g at pH 7 and 1.75 mg g−1 at pH 2). It was obvious that all IDFs had lower CAC at pH 2 than at pH 7; this result was in agreement with Wang (Wang et al., 2020) who reported that the CAC of IDF from okara by K. marxianus fermentation was 8.50 mg g−1 at pH 7, which was higher than that at pH2. Furthermore, IDFs from fermented okara had porous structure and specific surface features (Lin et al., 2020), which facilitated the adsorption of IDF on cholesterol. And M-IDF group exhibited the best effect. The results showed that IDFs from fermented okara can act as a functional component to lower cholesterol levels in vivo.
Figure 3d showed that the SCAC of L-IDF, K-IDF, and M-IDF was significantly increased compared to C-IDF, which was 4.51, 2.29, and 4.44 times higher than that of C-IDF (1.26 mg g−1), among which L-IDF and M-IDF were more effective than K-IDF.
Conclusion
In this study, the physicochemical and functional properties of IDF from okara by separate or co-fermentation with LAB and K. marxianus were compared and analysed. The results showed that separate and co-fermentation significantly increased the content of IDF by 2.4%, 4.0%, and 6.8%, respectively, and led to changes in structure and processing characteristics, as well as a reduction in particle size of IDF compared to non-fermented okara. Furthermore, the GAC, CAC, and SCAC of IDF from okara by separate or co-fermentation were superior to those of non-fermented okara, and the GAC and CAC of co-fermentation were increased by 185.7% and 134.9% (pH 2) compared to non-fermented okara, indicating that the glucose-lowering and lipid-lowering activities of IDF from okara by co-fermentation were significantly increased. This study provides valuable information for exploring the potential applications of IDF.
Acknowledgments
The authors are grateful to Jilin Province Innovation Center for Biological Food and Manufacturing Technology for their excellent technical assistance. Financial support was provided by Department of Science and Technology of Jilin Province (20210202107NC) and Earmarked Fund for Modern Agro-industry Technology Research System (CARS-04).
Author contributions
Yang Yue: Data curation (lead); formal analysis (lead); writing – original draft (lead). Yunbo Li: Data curation (lead); software (lead); validation (equal); writing – review and editing (equal). Pengcheng Li: Formal analysis (supporting); methodology (supporting); software (supporting). Yuyi Xiao: Methodology (supporting); validation (supporting). Chunhong Piao: Funding acquisition (lead); investigation (lead); project administration (lead); resources (lead); validation (lead). Xiujuan Wang: Data curation (lead); funding acquisition (lead); project administration (lead); writing – original draft (lead); writing – review and editing (lead).
Conflict of interest
All authors declare there are no conflicts of interest.
Ethics statement
Ethics approval was not required for this research.
Peer review
The peer review history for this article is available at https://www.webofscience.com/api/gateway/wos/peer-review/10.1111/ijfs.16561.
Data availability statement
The data that support the findings of this study are available on request from the corresponding author.
References
As mentioned in our paper, “the compact structure of IDF was disrupted by separate or co-fermentation into looser structure and smaller particle size, and more hydrophilic and lipophilic groups exposed” was proven true by the literature.
The functions and properties of dietary fiber as well as its multiple functions were described in the literature, proving that DF is a renewable biopolymer.
The purpose of the literature was to prove the accuracy of the hypothesis that "the cellulase produced during okara fermentation degrading crude protein, starch and cellulose", and similar report was made.
Table 1 in the literature showed 42.4%–58.1% total dietary fiber and 40.2%–50.8% insoluble dietary fiber, demonstrating the accuracy of the IDF mentioned in our paper accounting for more than 90% of the total dietary fiber (TDF) in okara.
The preparation of okara and IDF, activation of K. marxianus, and observation of microstructure mentioned in the reference were referred in our paper, and the scientific validity of the methods involved in our paper was demonstrated by citing references.